-
PDF
- Split View
-
Views
-
Cite
Cite
Miriam C Poirier, Letizia Marsili, Maria Cristina Fossi, Céline A. J Godard-Codding, Elena E Hernandez-Ramon, Nancy Si, Kathyayini V Divi, Rao L Divi, Iain Kerr, John Pierce Wise, Catherine F Wise, Sandra S Wise, Abou El-Makarim Aboueissa, James T. F Wise, John Pierce Wise, Polycyclic Aromatic Hydrocarbon-DNA Adducts in Gulf of Mexico Sperm Whale Skin Biopsies Collected in 2012, Toxicological Sciences, Volume 181, Issue 1, May 2021, Pages 115–124, https://doi.org/10.1093/toxsci/kfab016
- Share Icon Share
Abstract
The northern Gulf of Mexico has a long history of polycyclic aromatic hydrocarbon (PAH) contamination from anthropogenic activities, natural oil seepages, and the 2010 Deepwater Horizon explosion and oil spill. The continental shelf of the same area is a known breeding ground for sperm whales (Physeter macrocephalus). To evaluate PAH-DNA damage, a biomarker for potential cancer risk, we compared skin biopsies collected from Gulf of Mexico sperm whales in 2012 with skin biopsies collected from sperm whales in areas of the Pacific Ocean in 1999–2001. All samples were obtained by crossbow and comprised both epidermis and subcutaneous blubber. To evaluate exposure, 7 carcinogenic PAHs were analyzed in lipids extracted from Pacific Ocean sperm whale blubber, pooled by sex, and location. To evaluate PAH-DNA damage, portions of all tissue samples were formalin-fixed, paraffin-embedded, sectioned, and examined for PAH-DNA adducts by immunohistochemistry (IHC) using an antiserum elicited against benzo[a]pyrene-modified DNA, which crossreacts with several high molecular weight carcinogenic PAHs bound to DNA. The IHC showed widespread epidermal nuclear localization of PAH-DNA adducts in the Gulf of Mexico whales (n = 15) but not in the Pacific Ocean whales (n = 4). A standard semiquantitative scoring system revealed significantly higher PAH-DNA adducts in the Gulf of Mexico whales compared to the whales from the Pacific Ocean study (p = .0002).
The burden of PAH-DNA damage in Gulf of Mexico sperm whale epidermis demonstrates exposure to high molecular weight carcinogenic PAHs, and suggests that these whales may have an elevated risk of cancer induction.
The northern Gulf of Mexico has substantial polycyclic aromatic hydrocarbon (PAH) contamination. Anthropogenic sources include agriculture runoff, fossil fuel burns, vehicle emissions, marine traffic, commercial oil exploration, and refinery loss (MacDonald et al., 1993; Mitra and Bianchi, 2003). In addition, gas hydrates (ice crystals trapping gasses) drilled from the continental shelf contain oil (Brooks et al., 1984,1986), and natural oil seepage is frequent in the Gulf of Mexico. The magnitude of oil leaked naturally is predicted to be approximately 120 000 barrels per year (MacDonald et al., 1993). Median PAH values in continental shelf sediment at depths up to 3000 m were 76 ng total PAHs/g dry weight (Wade et al., 2008), a value considered nontoxic to local biota. However, the Deepwater Horizon (DWH) explosion (April 2010), resulted in the addition of approximately 5 million barrels of light sweet crude (MC252) oil to the northern Gulf of Mexico between the Florida Keys and the Texas coast (Berenshtein et al., 2020; MacDonald et al., 2015; McNutt et al., 2012). MC252 was composed mainly of n-alkanes, pristine, phytane, BTEX, C3 benzenes and alkylated, and nonalkylated PAHs (Liu et al., 2012). The PAHs were mostly low molecular weight (LMW, 2–3 benzene rings), but included low levels of high molecular weight (HMW, 4–6 benzene rings) PAHs. HMW PAHs found environmentally are of concern because they are chemically stable and include the known carcinogens: benzo[a]pyrene, benzo[a]anthracene, benzo[b]-fluoranthene, benzo[k]fluoranthene, chrysene, dibenzo[a, h]anthracene, and indeno[123-c, d]pyrene (Nisbet and LaGoy, 1992; USEPA, 1993).
After the DWH explosion, studies were initiated to evaluate toxicants, including PAHs, in the affected waters (DWH NRDA—Trustees, 2016; McNutt et al., 2012; Sammarco et al., 2013; Wade et al., 2016; Wise et al., 2018). In one study, the sum of 33 (Σ33)bioavailable PAHs, including HMW and LMW PAHs, in coastal waters from the 4 Gulf states, ranged from 5 to 10 ng/L before the spill, to 15–170 ng/L during the spill, to 5–15 ng/L by the spring of 2011 (Allan et al., 2012). Comparison of PAH levels in coastal waters with those in shoreline sediments consistently revealed that HMW PAH content was higher in the shoreline and wetland sediments, than in the waters and sediments of the continental shelf (Evans et al., 2017; Hong et al., 2015; Kirman et al., 2016; Wang et al., 2014; Yin et al., 2015). The presence of HMW PAHs in shoreline areas, which may be from multiple sources (anthropogenic, oil seepage, and oil weathering), are considered to increase the risk of carcinogenic PAH dispersal into the waters of the northern Gulf of Mexico as a result of hurricanes and tropical storms. Furthermore, Kolian et al. (2015) observed fresh oil leaking from the DWH well up to 22 months after the July 2010 capping, suggesting that this may also contribute to the shoreline PAH deposits.
The northern Gulf of Mexico is home to 600–700 sperm whales (Physeter macrocephalus) (Whitehead, 2003; DWH NRDA Rosel and Mullin). They are the longest of the toothed whales, and have the largest brain of any species on earth. They dive for 40–50 min to a depth of more than 1000 ft to consume squid and fish, but must come to the surface for 8–9 min at a time for oxygen and socialization (Whitehead, 2003). Males have a global migration range, but females, who breed every several years, spend 10 years raising a calf and remain in breeding areas located in warm climates. The continental shelf region of the northern Gulf of Mexico, is a sperm whale breeding ground (Waring et al., 2011), and largely superimposes the area impacted by the DWH oil spill (Figure 1). Photographic evidence of sperm whales swimming in oil has been provided by the Deepwater Horizon Natural Resource Damage Assessment (DWH NRDA Dias 2016). Sperm whales are listed as “endangered” under the Endangered Species Act and “strategic” under the Marine Mammal Protection Act (DWH NRDA Rosel and Mullin).
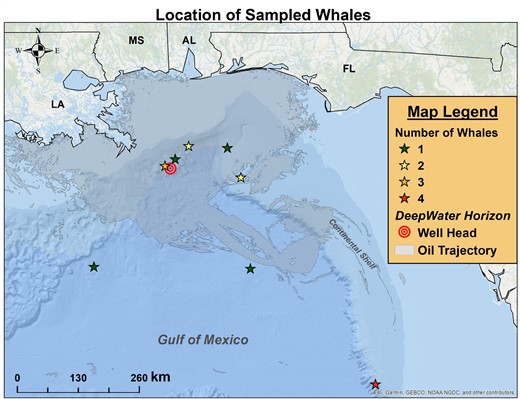
Map of the northern Gulf of Mexico where stars show the sampling locations of the sperm whales biopsied in 2012. The star colors change with number of whales sampled at each site: green = (1); yellow = (2); orange = (3); and red = (4). The location of the Deepwater Horizon oil rig is shown by the concentric red circles. The oil trajectory, as mapped by NOAA (see Materials and Methods), is shaded in grey.
In 2010–2012, the Wise Laboratory conducted research voyages in the northern Gulf of Mexico, taking biopsies consisting of epidermis and blubber from the local sperm whales for analysis of multiple toxic end-points (Wise et al., 2018). Here, biopsies taken during the 2012 voyage were examined for epidermal nuclear localization of carcinogenic PAH-DNA adducts using an antiserum specific for a family of carcinogenic HMW PAHs bound to DNA (Pratt et al., 2011). For comparison, we examined samples of sperm whale epidermis taken in areas of the Pacific Ocean where PAH levels were determined to be low at the time of biopsy (Godard-Codding et al., 2011).
MATERIALS AND METHODS
Whale biopsies and tissue processing
Skin biopsies were collected from 8 male and 7 female free-ranging adult or subadult sperm whales in the northern Gulf of Mexico in the summer of 2012 (Wise et al., 2018). Ocean Alliance’s research vessel Odyssey, a 93-ft motor-sailer ketch, was equipped to acoustically track echolocating whales. Once the whale was sighted, biopsies were collected from the left flank of the whale’s back using a crossbow with a modified bolt, as described (Wise et al., 2018). The bolt was constructed of a hydrostatic buoy attached behind a stainless-steel tip, which was approximately 20 mm in length and 6 mm in diameter. The buoy prevented deep penetration of the stainless-steel tip and provided a means of finding the biopsy once it was released from the whale. Along with the sample, parameters recorded included: species; suspected age (subadult, adult); Global Positioning System (GPS) coordinates of the encounter; any identifying markings (scars, flukes); whale’s reaction (tail flick); and where on the whale’s body the biopsy was taken (the left flank of the whale’s back). All animal procedures complied with approved institutional animal care protocols, and the whale samples were collected under National Marine Fisheries Services permits #1008-1637-03 and #0751-1614-03. Once retrieved, portions of 15 biopsies (from 8 males and 7 females numbered WO136-WO150) were placed immediately into standard buffered formalin for 48 h and then removed to vials containing ethanol. Postvoyage, the fixed samples were embedded in paraffin and stored at room temperature.
Whales were classified as adults or juveniles based on a clear and obvious size discrepancy. Adult males were obviously based on pronounced sexual dimorphism, and their male sex was confirmed with PCR-based amplification of the SRY gene on the y chromosome. Subadults were not determined in the field, as subadult males are about the same size as a female. Subadult males were separated from females in the laboratory using PCR amplification of the SRY gene on the y chromosome. Females were not classified into adults and subadults as there is no way to distinguish based on external appearance or PCR.
During Ocean Alliance’s global voyage of the Odyssey between 1999 and 2001, skin and blubber biopsies were collected from the right or left flank of adult or subadult sperm whales in several areas of the Pacific Ocean using crossbow and arrow, under US National Marine Fisheries Service permits #1004 and #751-1614 to Ocean Alliance, and according to standard procedures (Godard-Codding and Fossi, 2018). Details for multiple biomarkers were published in Godard et al. (2004) and Godard-Codding et al. (2011). The 2011 paper included analysis of 15 PAHs, but did not present a separate analysis of 7 carcinogenic PAHs shown here for the same whales. Samples were collected in Papua New Guinea (66 females, 10 males), Kiribati Island (16 females), Pacific Crossing (14 females, 9 males), Galapagos (19 males), and Sea of Cortez (55 females, 21 males).
Biopsy tissue samples were divided into 2 parts: one was fixed in 10% neutral-buffered formalin and paraffin blocks were prepared; the other was frozen for toxicological analyses (Godard et al., 2004). For analytical chemistry of carcinogenic PAHs, blubber samples from each region were pooled based on gender and location (weight range 3.7–184 mg) and lipids were extracted according to Griest and Caton (1983) with modifications (Marsili et al., 2001). Analysis was with High Performance Liquid Chromatography (HPLC) and fluorescence detection (Marsili et al., 2001). The sum of 7 (Σ7)carcinogenic PAHs was expressed as nanograms PAH/g lipid weight. The 7 carcinogenic PAHs included: benzo[a]anthracene, chrysene, benzo[b]fluoranthene, benzo[k]fluoranthene, benzo[a]pyrene, dibenzo(a, h)anthracene, and indeno(1,2,3-cd)pyrene (Godard-Codding et al., 2011).
ArcGIS mapping of Gulf of Mexico whale biopsy locations
The whale samples were mapped using GPS coordinates collected at the time of sampling with ArcGIS version 10.8.1 [Environmental Systems Research Institute (ESRI), Redlands, CA]. The specific map used was the Ocean basemap provided in the program, which was developed for the program using data from General Bathymetric Chart of the Oceans (GEBCO), National Oceanic and Atmospheric Administration (NOAA), National Geographic, DeLorme, and ESRI. Additionally, the basemap layer for the state outlines was used from the ArcGIS program and is SpatialData.GIS.states. The following digital coordinates were used for the Deepwater Horizonoil rig: 28.7381400 and -88.3659400. The oil trajectory was converted by the basemap data from DWH oil spill dataset compiled for Gulf of Mexico Ocean Observation (GCOOS) which compiled the data from NOAA/NESDIS found through ArcGIS’ online database. Their website is: https://gcoos.org/disclaimer/. All mapping was carried out with Geographic Coordinate System: GCS_WGS_1984.
Immunohistochemical (IHC) localization of PAH-DNA adducts
Sections (5 µm-thick) were cut from paraffin blocks of whale tissue, and stained using the Ventana (Benchmark XT, Roche Diagnostics Corp., Indianapolis, IN) with rabbit r7, t8-dihydroxy-t-9,10-epoxy-7,8,9,10-tetrahydrobenzo[a]pyrene (BPDE)-DNA antiserum diluted 1:18 000 (Poirier et al., 1980). This antiserum was elicited against DNA modified with the major adduct of benzo[a]pyrene (BP), the r7, t8, t9-trihydroxy-10-(N2-deoxy-guanosyl)-7,8,9,10-tetrahydrobenzo[a]pyrene (BPdG), and was shown to crossreact with at least 8 carcinogenic PAHs bound to DNA, but not with DNA alone or individual PAH-DNA adducts hydrolyzed from DNA (Pratt et al., 2011). Antirabbit Fast Red-conjugated secondary antiserum was used to reveal pink-colored nuclei containing DNA damage caused by covalent DNA binding of carcinogenic PAHs (Pratt et al., 2011; vanGijssel et al., 2002). Paraffin blocks were cut in pairs, so that one section was stained with the specific BPDE-DNA antiserum, and an adjacent section was stained with BPDE-DNA antiserum absorbed with the immunogen BPDE-DNA. The absorbed serum section served as a negative control, demonstrating the specificity of the PAH-DNA signal. Subsequently, the section stained with absorbed serum was stained with hematoxylin to reveal the location of nuclei. Sections from all blocks were stained at least once, and selected sections from several blocks were stained on 2 or more separate occasions, to verify reproducibility. Two whale blocks that showed very strong positive staining were repeatedly stained to serve as positive internal controls for the consistency of the staining.
Slides stained with specific and absorbed BPDE-DNA antiserum, and absorbed BPDE-DNA antiserum plus hematoxylin, were scanned into the Aperio system at 20× magnification (AperioScanScope AT2 digital slide scanner, Leica Biosystems, Inc., Buffalo Grove, IL) to create whole-slide image data files at 0.5 µm/pixel resolution. Image files were stored as “svs” files in an eSlide Manager Image Management System, and viewed using Aperio ImageScope software (Leica Biosystems, Inc., Buffalo Grove, IL). By Aperio, it was possible to identify the same region in each image, allowing simultaneous comparison of staining of a single region with specific antiserum, absorbed antiserum, and hematoxylin.
Standard curve for benzo[a]pyrene (BP)-DNA in sperm whale fibroblasts
Skin fibroblasts, isolated, and cultured from a biopsy obtained from a free-ranging sperm whale off the coast of North Carolina (Wise et al., 2011), were exposed to increasing concentrations of BPDE (Poirier et al., 2019), and used here as a positive control for the IHC staining of the sperm whale epidermal samples. Exposure of the cells, IHC of formalin-fixed cells, and quantitative ELISA of the BPdG adduct in DNA extracted from these cells, are described in detail, with photographs (Poirier et al., 2019), and will not be repeated here. Briefly, cultured sperm whale skin fibroblasts were grown to semiconfluence and exposed to 0, 0.05, 0.15, and 0.30 µM BPDE for 1 h. Half of the pelleted cells were formalin-fixed and made into paraffin blocks for immunohistochemistry (IHC), as described above. Half of the pelleted cells were used for DNA extraction and the BPdG adduct quantified by BPDE-DNA chemiluminescence immunoassay as described (Poirier et al., 2019). Both methods showed similar dose–response profiles for the standard curve. In this study, the standard curve blocks were stained simultaneously with the whale biopsy sample to validate the consistency of the staining.
Scoring based only on nuclear pink color intensity
In order to compare pink color intensity, indicating the presence of PAH-DNA adducts, in the different IHC samples each complete tissue section was visualized by Aperio and representative photomicrographs were taken at 1:100 magnification. The scoring was performed in a blinded fashion by 3 of the authors (MCP, RLD, and KVD). Each tissue from a single block was examined visually and scored either 1, 2, 3, or 4, where: [1] indicated no nuclear pink color (no PAH-DNA adducts); [2] indicated faint pink nuclear staining; [3] indicated moderately positive pink nuclear staining; and [4] indicated strongly positive, dark pink nuclear staining. The final intensity score was an average of the values obtained by the 3 authors. Examples of sperm whale epidermis with scores 1–4 are shown in Figure 2.
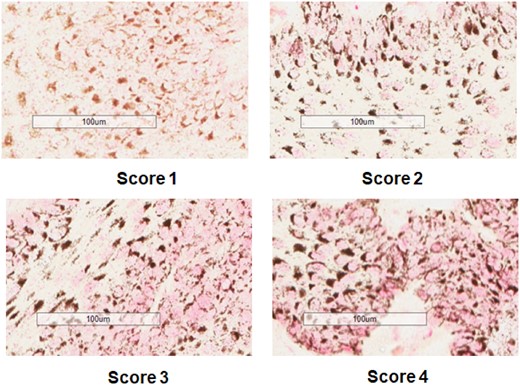
Examples of scoring 1–4 for intensity of nuclear pink color, indicating PAH-DNA damage. Whales shown here are: PM99-454 (Sea of Cortez) for Score 1; WO141 (Gulf of Mexico) for Score 2; WO148 (Gulf of Mexico) for Score 3; and WO139 (Gulf of Mexico) for Score 4.
Standardized semiquantitative scoring system
The method, from Godard et al. (2004), will be described briefly here. For each whale, a representative section was scored 0–3 for staining occurrence, where a 0 indicates no staining and 3 indicates staining in all cells. The scoring for staining intensity was 1–4, and is described above. The final score for each whale was determined to be the product of the staining occurrence multiplied by the staining intensity.
Statistics
The distribution-free bootstrap test was used to perform the comparison between PAH-DNA staining of Gulf of Mexico and Pacific Ocean whales. A p value <.05 provides statistical evidence that the average of the values from the Gulf of Mexico whales is greater than the average of the values from the Pacific Ocean whales. This analysis was conducted using R 4.0.3 software, and was applied to both the staining intensity scoring and the semiquantitative scoring.
RESULTS
Analysis of Carcinogenic PAHs in Pacific Ocean Sperm Whale Blubber
Between 1999 and 2001 scientists from Ocean Alliance cruised various destinations in or adjacent to the Pacific Ocean and obtained samples of sperm whale skin, which contained both blubber and epidermis (Godard-Codding et al., 2011). For analysis of the 7 carcinogenic HMW PAHs, whale blubber samples from each location were combined, but separated by sex. The data for each compound and the Σ7 HMW PAHs are presented in Table 1. The table shows that the Pacific Ocean sperm whales were exposed to all 7 of the HMW PAHs, and that Galapagos and Sea of Cortez were among the locations where the lowest levels of carcinogenic PAHs were found in blubber. Consequently, whales from these areas were chosen as low-level controls for IHC analysis of PAH-DNA adducts.
Concentrations (ng/gLipid Weight) of 7 Carcinogenic HMW PAHsa in Blubber of Male (M) and Female (F) Sperm Whales in the Pacific Ocean (1999–2001) Pooled by Location and Sex.
Location/# Sex . | Chrysene . | B[a]A . | B[k]F . | B[b]F . | BP . | DB[a, h]A . | I[1,2,3-cd]P . | Total (Σ7) . |
---|---|---|---|---|---|---|---|---|
Sea of Cortez/55 F | 42.35 | 7.37 | 15.87 | 1.20 | 4.15 | 5.63 | 1.64 | 78.21 |
Sea of Cortez/21 M | 13.63 | 1.59 | 16.55 | 0.95 | 2.11 | 0.86 | 0.23 | 35.92 |
Galapagos/19 M | 34.95 | 8.62 | 16.25 | 2.94 | 4.33 | 2.08 | 0.56 | 69.74 |
Pacific Crossing/14 F | 233.89 | 3.61 | 21.05 | 47.12 | 1.69 | 8.63 | 5.46 | 321.44 |
Pacific Crossing/9 M | 43.93 | 5.99 | 32.67 | 5.10 | 6.34 | 8.44 | 0.22 | 102.69 |
Kiribati/16 F | 65.93 | 0.81 | 13.52 | 1.42 | 3.86 | 1.80 | 1.79 | 89.12 |
Papua New Guinea/66 F | 65.95 | 3.54 | 47.48 | 3.38 | 4.34 | 3.13 | 3.92 | 131.73 |
Papua New Guinea/10 M | 74.25 | 6.58 | 70.02 | 10.09 | 13.36 | 15.42 | 25.16 | 214.88 |
Location/# Sex . | Chrysene . | B[a]A . | B[k]F . | B[b]F . | BP . | DB[a, h]A . | I[1,2,3-cd]P . | Total (Σ7) . |
---|---|---|---|---|---|---|---|---|
Sea of Cortez/55 F | 42.35 | 7.37 | 15.87 | 1.20 | 4.15 | 5.63 | 1.64 | 78.21 |
Sea of Cortez/21 M | 13.63 | 1.59 | 16.55 | 0.95 | 2.11 | 0.86 | 0.23 | 35.92 |
Galapagos/19 M | 34.95 | 8.62 | 16.25 | 2.94 | 4.33 | 2.08 | 0.56 | 69.74 |
Pacific Crossing/14 F | 233.89 | 3.61 | 21.05 | 47.12 | 1.69 | 8.63 | 5.46 | 321.44 |
Pacific Crossing/9 M | 43.93 | 5.99 | 32.67 | 5.10 | 6.34 | 8.44 | 0.22 | 102.69 |
Kiribati/16 F | 65.93 | 0.81 | 13.52 | 1.42 | 3.86 | 1.80 | 1.79 | 89.12 |
Papua New Guinea/66 F | 65.95 | 3.54 | 47.48 | 3.38 | 4.34 | 3.13 | 3.92 | 131.73 |
Papua New Guinea/10 M | 74.25 | 6.58 | 70.02 | 10.09 | 13.36 | 15.42 | 25.16 | 214.88 |
HMW PAHs (ng carcinogenic PAH/g lipid weight) in lipid extracted from sperm whale blubber biopsies pooled from 55 females and 21 males in the Sea of Cortez, 19 males in the Galapagos, 14 females and 9 males in the Pacific crossing, 16 females in Kiribati, and 66 females and 10 males in Papua New Guinea. The PAHs included: chrysene; benzo[a]anthracene (B[a]A); benzo[b]fluoroanthene (B[b]F); benzo[k]fluoroanthene (B[k]F); benzo[a]pyrene (BP); dibenzo[a, h]anthracene (DB[a, h]A); and indeno[1,2,3-cd]pyrene (I[1,2,3-cd]P).
Concentrations (ng/gLipid Weight) of 7 Carcinogenic HMW PAHsa in Blubber of Male (M) and Female (F) Sperm Whales in the Pacific Ocean (1999–2001) Pooled by Location and Sex.
Location/# Sex . | Chrysene . | B[a]A . | B[k]F . | B[b]F . | BP . | DB[a, h]A . | I[1,2,3-cd]P . | Total (Σ7) . |
---|---|---|---|---|---|---|---|---|
Sea of Cortez/55 F | 42.35 | 7.37 | 15.87 | 1.20 | 4.15 | 5.63 | 1.64 | 78.21 |
Sea of Cortez/21 M | 13.63 | 1.59 | 16.55 | 0.95 | 2.11 | 0.86 | 0.23 | 35.92 |
Galapagos/19 M | 34.95 | 8.62 | 16.25 | 2.94 | 4.33 | 2.08 | 0.56 | 69.74 |
Pacific Crossing/14 F | 233.89 | 3.61 | 21.05 | 47.12 | 1.69 | 8.63 | 5.46 | 321.44 |
Pacific Crossing/9 M | 43.93 | 5.99 | 32.67 | 5.10 | 6.34 | 8.44 | 0.22 | 102.69 |
Kiribati/16 F | 65.93 | 0.81 | 13.52 | 1.42 | 3.86 | 1.80 | 1.79 | 89.12 |
Papua New Guinea/66 F | 65.95 | 3.54 | 47.48 | 3.38 | 4.34 | 3.13 | 3.92 | 131.73 |
Papua New Guinea/10 M | 74.25 | 6.58 | 70.02 | 10.09 | 13.36 | 15.42 | 25.16 | 214.88 |
Location/# Sex . | Chrysene . | B[a]A . | B[k]F . | B[b]F . | BP . | DB[a, h]A . | I[1,2,3-cd]P . | Total (Σ7) . |
---|---|---|---|---|---|---|---|---|
Sea of Cortez/55 F | 42.35 | 7.37 | 15.87 | 1.20 | 4.15 | 5.63 | 1.64 | 78.21 |
Sea of Cortez/21 M | 13.63 | 1.59 | 16.55 | 0.95 | 2.11 | 0.86 | 0.23 | 35.92 |
Galapagos/19 M | 34.95 | 8.62 | 16.25 | 2.94 | 4.33 | 2.08 | 0.56 | 69.74 |
Pacific Crossing/14 F | 233.89 | 3.61 | 21.05 | 47.12 | 1.69 | 8.63 | 5.46 | 321.44 |
Pacific Crossing/9 M | 43.93 | 5.99 | 32.67 | 5.10 | 6.34 | 8.44 | 0.22 | 102.69 |
Kiribati/16 F | 65.93 | 0.81 | 13.52 | 1.42 | 3.86 | 1.80 | 1.79 | 89.12 |
Papua New Guinea/66 F | 65.95 | 3.54 | 47.48 | 3.38 | 4.34 | 3.13 | 3.92 | 131.73 |
Papua New Guinea/10 M | 74.25 | 6.58 | 70.02 | 10.09 | 13.36 | 15.42 | 25.16 | 214.88 |
HMW PAHs (ng carcinogenic PAH/g lipid weight) in lipid extracted from sperm whale blubber biopsies pooled from 55 females and 21 males in the Sea of Cortez, 19 males in the Galapagos, 14 females and 9 males in the Pacific crossing, 16 females in Kiribati, and 66 females and 10 males in Papua New Guinea. The PAHs included: chrysene; benzo[a]anthracene (B[a]A); benzo[b]fluoroanthene (B[b]F); benzo[k]fluoroanthene (B[k]F); benzo[a]pyrene (BP); dibenzo[a, h]anthracene (DB[a, h]A); and indeno[1,2,3-cd]pyrene (I[1,2,3-cd]P).
IHC Analysis of PAH-DNA Adducts in Sperm Whale Epidermis from the Gulf of Mexico and the Pacific Ocean
Figure 1, a map of the northernGulf of Mexico, shows the location of the DWH oil platform, a NOAA projection of the oil spread, and the locations where biopsies were taken from the 15 sperm whales in the Gulf of Mexico in 2012. For the IHC analysis, we also examined 2 whales from the Galapagos taken in 2000, and 2 whales from the Sea of Cortez taken in 1999.
Sections of whale epidermis incubated with an antiserum specific for a family of carcinogenic PAHs bound to DNA were subsequently stained with Fast Red to localize PAH-DNA adducts (pink color). Figure 2 shows varying levels of PAH-DNA staining intensity in sperm whale epidermis. Parallel slides were stained with hematoxylin to localize nuclei, and antigen-absorbed serum to serve as negative controls. This approach allowed simultaneous examination of the same tissue area in 3 photographs. Representative examples are shown in Figure 3, where photos (a) and (b) show specific PAH-DNA antiserum, photos (c) and (d) show localization of nuclei with hematoxylin, and photos (e) and (f) show the absorbed serum controls. The pink color, indicating the presence of PAH-DNA adducts, was localized primarily in the epidermal basal layers of the sperm whale epidermis, and was found in all 15 of the Gulf of Mexico sperm whales, but not in the 4 whales from the Pacific Ocean study.
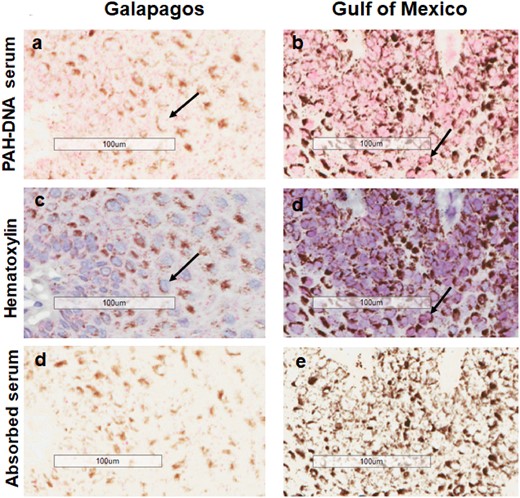
Immunohistochemistry showing PAH-DNA adduct formation (pink nuclei) in sperm whales from Galapagos (a) and Gulf of Mexico (b). Hematoxylin staining of nuclei is shown in (c) and (d) and absorbed serum controls are shown in (e) and (f). The pink color intensity scoring values for (a) and (b), are 1 and 4, respectively. Black arrows indicate PAH-DNA adducts in nuclei. Brown perinuclear areas show localization of melanin.
Scoring for PAH-DNA Adducts in Epidermis by Both Nuclear Color Intensity and a Semiquantitative Scoring System
In order to compare the intensity of PAH-DNA nuclear pink color among samples, coded photomicrographs were scored blind by 3 of the authors and subjected to both: scoring of only nuclear pink color intensity on a scale of 1–4 (Figure 2; Table 2); and scoring using a standard semiquantitative scoring method (Godard et al., 2004) (Table 2). Figures 2 and 3 show representative samples for color intensity levels 1–4. Table 2 shows scoring values and statistics for 15 Gulf of Mexico whales and 4 Pacific Ocean whales using both scoring methods. Using the distribution-free bootstrap test, the p values of .0004 and .0002, indicated that the mean of the values from the Gulf of Mexico whales was greater than the mean of the values from the Pacific Ocean whales using both the nuclear color intensity scoring and semiquantitative scoring, respectively.
Scoring Method . | Number of Whales . | Location . | Mean ± SD . | p Valuec . |
---|---|---|---|---|
Color intensity Scoringa | 4 | Sea of Cortez/Galapagos | 1.16 ± 0.16 | |
15 | Gulf of Mexico | 3.02 ± 0.74 | 0.0004 | |
Semiquantitative Scoringb | 4 | Sea of Cortez/Galapagos | 1.00 ± 0.27 | |
15 | Gulf of Mexico | 8.87 ± 2.64 | 0.0002 |
Scoring Method . | Number of Whales . | Location . | Mean ± SD . | p Valuec . |
---|---|---|---|---|
Color intensity Scoringa | 4 | Sea of Cortez/Galapagos | 1.16 ± 0.16 | |
15 | Gulf of Mexico | 3.02 ± 0.74 | 0.0004 | |
Semiquantitative Scoringb | 4 | Sea of Cortez/Galapagos | 1.00 ± 0.27 | |
15 | Gulf of Mexico | 8.87 ± 2.64 | 0.0002 |
Blinded scoring by 3 of the authors taking into account nuclear color intensity only.
Semiquantitativeblinded scoring by 3 of the authors taking into account both the occurrence of pink nuclei and intensity of pink color (Godard et al., 2004).
The distribution free “bootstrap test” was used to compare the 2groups, and by both scoring methods there were significant differences in PAH-DNA adducts between the mean values for the Gulf of Mexico sperm whales and the Sea of Cortez/Galapagos sperm whales.
Scoring Method . | Number of Whales . | Location . | Mean ± SD . | p Valuec . |
---|---|---|---|---|
Color intensity Scoringa | 4 | Sea of Cortez/Galapagos | 1.16 ± 0.16 | |
15 | Gulf of Mexico | 3.02 ± 0.74 | 0.0004 | |
Semiquantitative Scoringb | 4 | Sea of Cortez/Galapagos | 1.00 ± 0.27 | |
15 | Gulf of Mexico | 8.87 ± 2.64 | 0.0002 |
Scoring Method . | Number of Whales . | Location . | Mean ± SD . | p Valuec . |
---|---|---|---|---|
Color intensity Scoringa | 4 | Sea of Cortez/Galapagos | 1.16 ± 0.16 | |
15 | Gulf of Mexico | 3.02 ± 0.74 | 0.0004 | |
Semiquantitative Scoringb | 4 | Sea of Cortez/Galapagos | 1.00 ± 0.27 | |
15 | Gulf of Mexico | 8.87 ± 2.64 | 0.0002 |
Blinded scoring by 3 of the authors taking into account nuclear color intensity only.
Semiquantitativeblinded scoring by 3 of the authors taking into account both the occurrence of pink nuclei and intensity of pink color (Godard et al., 2004).
The distribution free “bootstrap test” was used to compare the 2groups, and by both scoring methods there were significant differences in PAH-DNA adducts between the mean values for the Gulf of Mexico sperm whales and the Sea of Cortez/Galapagos sperm whales.
DISCUSSION
The data presented here show that epidermal nuclei from 15 sperm whales biopsied in the Gulf of Mexico in 2012 contained DNA adducts of carcinogenic HMW PAHs. Furthermore, skin samples from Pacific Ocean sperm whales (n = 4) taken in 1999–2001, had significantly lower levels of epidermal PAH-DNA adducts than the skin samples from the Gulf of Mexico sperm whales (p = .0002) using a standardized semiquantitative scoring system. Investigations in whales are challenging because of the difficulty in obtaining subject material, and this study has some weaknesses. For example, it was not possible to obtain pre-DWH Gulf of Mexico whale biopsy samples, and there were no PAH analyses performed on the blubber samples from the Gulf of Mexico whales. In addition, the group of Pacific Ocean whales was small. However, the presence of DNA adducts formed by carcinogenic PAHs in epidermal nuclei from Gulf of Mexico sperm whales indicates that these whales were exposed to carcinogenic HMW PAHs. Values for PAH-DNA adducts in opportunity samples from animals in the wild are reflective of the steady-state balance between the process of DNA adduct formation, a function of exposure, and the processes of DNA adduct removal, largely a function of DNA repair and epidermal turnover. The significant steady-state PAH-DNA levels in the Gulf of Mexico sperm whale epidermis suggest that HMW PAH exposure is an ongoing process in whales frequenting this area. Despite their small number, the lack of PAH-DNA damage in the Galapagos and Sea of Cortez whales indicates that the HMW PAH exposure levels of these whales (Table 1) are insufficient to induce PAH-DNA adduct levels detectable by the IHC method used here.
The basic mechanistic paradigm of chemical carcinogenesis comprises chronic carcinogen exposure, consequent carcinogen-DNA damage (DNA adduct formation), subsequent mutagenesis in critical (driver, tumor suppressor) genes, and eventual tumor formation (Beland and Poirier, 1993; IARC, 2012; Maher and McCormick, 1984; Phillips, 2018; Poirier, 2004; 2016; Vogelstein and Kinzler, 2004). This paradigm has been demonstrated to apply to cancer induction by carcinogenic PAHs in many species, including humans, rodents, and fish (IARC, 2010; 2012). Previous studies in beluga have shown the presence of PAH-DNA adducts in liver, brain, and kidney of beluga from the Arctic and the St. Lawrence Estuary (SLE) (Martineau et al., 1988; Mathieu et al., 1997). Furthermore, PAH-DNA adduct formation in SLE beluga small intestine has been linked the high rate of gastrointestinal cancers observed in these same whales. The intestinal cancers were attributed to PAH contamination in the Saguenay and St. Lawrence rivers caused by industrial waste. Between 1926 and 1976 (Lair et al., 2016; Martineau et al., 2002) unaltered PAHs were deposited directly into the beluga habitat, the Saguenay river. By IHC, PAH-DNA damage was found localized in intestinal crypt cells of the SLE beluga, the cells from which the intestinal tumors are thought to arise (Poirier et al., 2019). In addition, several beluga developing cancers also had the highest levels of PAH-DNA damage, suggesting that observations of PAH-DNA damage in the Gulf of Mexico sperm whales may also indicate a potential for cancer. Currently, cancers reported for sperm whales in the wild are rare (Newman and Smith, 2006), and include genital papillomas, tumor of the cervix, uterine leiomyoma, hemangioma, and fibromas of the skin and jaw. However, because PAH-DNA damage is necessary but not sufficient for cancer induction, it is possible that sperm whales carrying PAH-DNA adducts may have an increased cancer risk.
Direct comparison of the pollution levels in the post-DWH Gulf of Mexico and areas of the Pacific Ocean in 1999–2001 is not straightforward, however, some general information may be useful. Sammarco et al. (2013) reported a mean level of 29 parts per million (ppm, dry weight basis), with a maximum value of 554 ppm, for total PAHs in 21 pooled samples of flora and fauna taken in the Gulf of Mexico near the mouth of the Mississippi river during the first several months after the DWH spill. In the Pacific Ocean study,Godard-Codding et al. (2011) examined sperm whale blubber concentrations for Σ15 PAHs, 7 of which are the carcinogenic PAHs presented separately here in Table 1. Values for Σ15 PAHs extracted from blubber ranged between 0.08 and 0.31 ppm (lipid basis) or 800–5800 ng total PAHs/g extracted organic material.
The effects of oil exposure on cetaceans have been studied for at least 50 years and typical routes of oil exposure include inhalation (aspiration), oral exposure, and dermal exposure (Godard-Codding and Collier, 2018a; Peterson et al., 2003). Toxic consequences of oil exposure impact the integrity of the lungs, skin, and gastrointestinal systems. Many of the sequelae, including inflammatory response, different types of lung diseases, central nervous system effects, adrenal cortex issues, reproductive compromise, and death, are similar in both humans (IARC, 2010,2012; Sramet al., 2017) and cetacean species (Godard-Codding and Collier, 2018b). The first major US oil spill, the 1989 grounding of the oil tanker Exxon Valdez in Prince William Sound Alaska, provided an opportunity to observe both short-term and long-term effects of oiling on the natural environment (Matkin et al., 2008; Peterson et al., 2003). Short term, there were massive mortalities of sea otters, harbor seals, and birds (Peterson et al., 2003), and 2 pods of killer whales (Orcinus orca) suffered 33 and 41% mortality in the first year after the spill (Matkin et al., 2008). The same killer whales were followed for 16 years, at which time 1 pod still had fewer individuals than before the spill, while the other pod was judged depleted under the Marine Mammal Protection Act, and likely to become extinct (Matkin et al., 2008). In the aftermath of the DWH explosion, there was evidence of oil exposure in 21 cetacean species inhabiting the affected area (DWH NRDATrustees). A great deal is known about oil toxicity in the bottlenose dolphins in Barataria Bay through a capture-release health assessment program (Schwacke et al., 2014). This species was particularly hard hit, as about half of the 29 dolphins evaluated in 2011 were given a poor prognosis, and 17% were not expected to live (Schwacke et al., 2014). For obvious reasons, capture-release programs are not possible with the Gulf of Mexico sperm whales, however they are at the top of the food chain and may be particularly susceptible to PAHs and other toxicants collecting in the fish and squid that constitute the major fraction of their diet.
The conclusions of this study are as follows: (1) all 15 of the sperm whales biopsied in the Gulf of Mexico and examined for PAH-DNA adducts showed evidence of PAH-DNA adduct formation in epidermal nuclei, showing exposure to carcinogenic HMW PAHs; (2) all 4 sperm whales from the Pacific Ocean showed no evidence of nuclear PAH-DNA adduct formation in epidermis, and low levels of HMW PAHs in blubber, which suggested low PAH exposure; (3) because of ongoing HMW PAH exposures the Gulf of Mexico sperm whales may also have an increased risk of cancer.
ACKNOWLEDGMENTS
We would like to thank Amie Holmes, Shouping Huang, Selma Botman, Timothy Stevens, and all the Wise Laboratory/Odyssey science team volunteers for their help with technical support, whale spotting, and sample collection. We thank Bob Wallace, Ian Glass, and all of the Odyssey boat crew for their help with sample collection and logistics. The content of this manuscript is solely the responsibility of the presenters and does not necessarily represent the official views of the National Institute of Environmental Health Sciences, the National Institutes of Health, the Army Research Office, or the Department of Defense Logistics. The critical comments of Dr. Daniel Martineau are very much appreciated.
FUNDING
The immunohistochemical staining and statistical analyses were supported by the Intramural Research Program of the Center for Cancer Research, National Cancer Institute, NIH; grant number Z01 BC 005177. The work in the Gulf of Mexico was supported by grants from the National Institute of Environmental Health Sciences [P30ES030283 and ES016893 (J.P. Wise Sr., PI)] and [T32ES011564 (J. Wise Jr, J.T.F. Wise and J.P. Wise Sr. )]; the Army Research Office [W911NF-09-1-0296 (J. Wise Sr., PI)]; and funding from University of Southern Maine, the Maine Center for Toxicology and Environmental Health; The Albemarle Corporation; the Campbell Foundation; the Ocean Foundation; Quiznos; Ocean Alliance, and the many individual and anonymous Wise Laboratory donors. Work was conducted under National Marine Fisheries Service permit #1008-1637-03 (J. Wise Sr., PI) and permit #751-1614 (Iain Kerr, PI). For the work in the Pacific Ocean study, funding was provided by: National Institute of Environmental Health Sciences grant P42-ES-0469; Superfund Basic Research Program grant P42-ES-007381; NOAA Sea Grant NA86RG0075R/B-162; and the Ocean Alliance.
DECLARATION OF CONFLICTING INTERESTS
The authors declare no competing financial interests.
REFERENCES
Deepwater Horizon Natural Resource Damage Assessment (DWH NRDA),
Deepwater Horizon Natural Resource Damage Assessment (DWH NRDA).
Deepwater Horizon Natural Resource Damage Assessment (DWH NRDA) Trustees. (
IARC (
IARC (
USEPA. (
Comments