-
PDF
- Split View
-
Views
-
Cite
Cite
Muhammad M Hossain, Ganeshraj Sivaram, Jason R Richardson, Regional Susceptibility to ER Stress and Protection by Salubrinal Following a Single Exposure to Deltamethrin, Toxicological Sciences, Volume 167, Issue 1, January 2019, Pages 249–257, https://doi.org/10.1093/toxsci/kfy238
- Share Icon Share
Abstract
Endoplasmic reticulum (ER) stress is a significant contributor to neurodegeneration and cognitive dysfunction. Recently, we reported that repeated exposure to the pyrethroid insecticide deltamethrin caused ER stress in the hippocampus of adult mice, which was accompanied by deficits in learning (Hossain et al., 2015). Here, we investigated regional susceptibility to ER stress and the ability of salubrinal, an inhibitor of ER stress, to reduce apoptosis following a single oral administration of deltamethrin (6 mg/kg). Deltamethrin significantly increased the ER stress marker C/EBP-homologous protein (CHOP) in the hippocampus by 148% at 24 and 48 h compared with age-matched controls. In contrast, CHOP was increased by 146% in the frontal cortex only at 48 h after deltamethrin exposure. Similarly, the level of GRP-78 was increased by 314% and 262% in the hippocampus at 24 and 48 h, whereas the same factors were increased by 178% at 24 h and 139% at 48 h in the frontal cortex. These changes were accompanied by increased levels of activated caspase-12, caspase-3, and TUNEL-positive cells in both brain regions, with the hippocampus showing a more robust response. Pre-treatment of mice with the eIf2α inhibitor salubrinal prevented deltamethrin-induced caspase-3 activation and attenuated the number of TUNEL-positive cells. These data demonstrate that the hippocampus appears to be particularly vulnerable to deltamethrin exposure in adult animals, which may contribute to observed effects of deltamethrin on cognitive function.
Pyrethroids are synthetic insecticides similar to the natural pyrethrins found in chrysanthemum flowers, which now constitute the majority of commercial household and agricultural insecticides. The use of pyrethroids has increased worldwide over the past decade with declining use of organochlorine and organophosphate pesticides (Morgan, 2012). Deltamethrin is a potent type II pyrethroid used extensively in agriculture and residential applications leading to public health and commercial concerns. It has also been used for mosquito control following outbreaks of the West Nile, Ross River, and Dengue viruses, malaria, and bed bug infestations (ATSDR, 2003; van den Berg et al., 2012; WHO, 2011), and most recently for Zika. There is concern that because humans lack serum carboxylesterases (CES) (Crow et al., 2007), one of the main enzymes involved in the detoxication of pyrethroids, humans may be at increased risk based on reduced ability to detoxify pyrethroids. Recent data demonstrate the potential for neurotoxic effects following pyrethroid exposure, particularly in sensitive and vulnerable populations such as children (Richardson et al., 2015; Wagner-Schuman et al., 2015). In addition, neurological effects, including cognitive impairment, have been reported in adults occupationally exposed to higher levels of pyrethroids (Calvert et al., 2003; Kamel et al., 2005; Kimata et al., 2009; Muller-Mohnssen, 1999).
The primary mechanism by which pyrethroids exert neurotoxicity is delaying the closing of neuronal voltage-gated sodium channels (VGSC), resulting in persistent depolarization of the nerve membrane (Soderlund, 2012). In addition, voltage-gated calcium channels and voltage- and ligand-gated chloride channels have been implicated as alternative action sites of pyrethroids (Clark and Symington, 2012). More recently, attention has focused on the potential cellular mechanisms that may be involved in pyrethroid neurotoxicity associated with neurodegeneration, including disruption of mictochondrial function (Kumar et al., 2016), microglial activation (Hossain et al., 2017), and ER stress (Hossain et al., 2015; Hossain and Richardson, 2011).
Previously, we reported that repeated exposure to deltamethrin (3 mg/kg every 3 days for 2 months) caused ER stress and apoptosis in the hippocampus of adult mice that was accompanied by learning deficits (Hossain et al., 2015). The ER is an essential multifunctional cellular organelle that facilitates protein synthesis, folding, modification, and transportation and maintains intracellular calcium homeostasis (Oslowski and Urano, 2011). Various intracellular and extracellular insults can disturb ER function leading to excessive calcium homeostasis and misfolded protein in the ER, which then triggers ER stress (Asada et al., 2011; Ron and Walter, 2007; Tabas and Ron, 2011). Thus, cells employ a highly specific ER quality control system termed the unfolded protein response (UPR) to re-establish normal ER function (Kim et al., 2008; Szegezdi et al., 2006). However, prolonged or unresolved ER stress can result in cell death through the activation of multiple apoptotic signaling cascades (Tabas and Ron, 2011).
Here, we investigated regional differences in ER stress and apoptosis following acute oral administration of a single dose (6 mg/kg) of deltamethrin. We further assessed whether the inhibition of ER stress would prevent induction of apoptosis caused by deltamethrin.
The data reveal that a single exposure to deltamethrin leads to ER stress and activation of apoptotic signaling, as indicated by increased levels of CHOP and GRP-78 along with increased caspase-12 and caspase-3 activation. The hippocampus appears to be more sensitive to deltamethrin-induced ER stress and apoptosis compared with the cortex, as earlier and more pronounced effects were observed in this region. Pre-treatment of mice with ER stress factor eIf2α inhibitor salubrinal completely abolished the induction of apoptosis caused by deltamethrin. Taken in concert, these data demonstrate the unique sensitivity of the hippocampus to deltamethrin-induced ER stress and provides support for inhibition of ER stress in preventing deltamethrin-induced apoptotic signaling and, perhaps cognitive dysfunction.
MATERIALS AND METHODS
Animals
Ten-week-old male C57BL/6J mice were housed 4 per cage in a temperature-controlled animal care barrier facility under a 12 h light/dark cycle with access to food and water ad libitum. Breeder males and females were originally obtained from Jackson Laboratories (Bar Harbor, Maine) and mice for these experiments were generated through our in-house breeding colony in an AALAC-accredited facility. Experiments were performed in compliance with the NIH Guide for the Care and Use of Laboratory Animals and all procedures were approved by the Institutional Animal Care and Use Committee of Northeast Ohio Medical University.
Treatment
A total of 56 mice were randomly divided into 14/group for 4 groups (control, salubrinal, deltamethrin, and salubrinal + deltamethrin). Deltamethrin (Cat No. N-11579, Chem Service, Inc, West Chester, Pennsylvania) was dissolved in corn oil, and salubrinal (Cat No. SML0951, Sigma, St. Louis, Missouri) was prepared in saline (0.9% NaCl, Hospira, Lake Forest, Illinois). Mice were administered two intraperitoneal (i.p.) injections of 1 mg/kg salubrinal 24 h and 30 min before the administration of deltamethrin. Animals were administered a single dose of deltamethrin (6 mg/kg) via oral gavage. The dose of deltamethrin used in this study is 1/10th of LD50 (Sharma et al., 2014) and did not cause any classic signs of toxicity, including tremor, salivation, choreoathetosis, and ataxia in mice. Control animals received the same volume (1 ml/kg) of corn oil as deltamethrin-treated animals. Animals (n = 5/group) were sacrificed at 24 and 48 h following deltamethrin administration. Brains were removed and the cortex and hippocampus were dissected on ice and stored at −80°C for biochemical assays. The remaining 16 animals (4/groups) were anesthetized with ketamine/xylazine (100 mg/kg/10 mg/kg, i.p.) mixture, transcardially perfused with phosphate buffered saline (PBS) followed by 4% paraformaldehyde in PBS (pH 7.4). Brains were removed and post-fixed in 4% paraformaldehyde at 4°C for 72 h, and then transferred into 30% sucrose with 0.1% sodium azide in PBS. Brains were cut at 30 μm coronal sections through the entire hippocampus on a freeze sliding microtome (MH 450, Thermo Fisher Scientific) and stored at −20°C in cryoprotectant solution (25% sucrose and 25% ethylene glycol in PBS).
Western blot analysis
Protein extraction and Western blot analysis were performed as previously described in Hossain et al. (2015). Briefly, cortical and hippocampal tissues were homogenized and protein concentrations were determined using a BCA kit (Cat No. 23225, Thermo Fisher Scientific). Twenty micrograms of protein from each sample were electrophoresed on 4%–12% Bis-Tris Midi gels (Invitrogen, Carlsbad, California) and separated protein bands were then transferred onto PVDF membranes. The membranes were then blocked in 7.5% non-fat milk followed by incubation with appropriate primary and secondary antibodies. Protein bands were detected with SuperSignal West Dura Extended Duration Substrate (Thermo Scientific, Rockford, Illinois) and images were visualized and captured using an Alpha Innotech Fluorochem imager (San Leandro, California).
TUNEL assay
Terminal deoxynucleotidyl transferase-mediated dUTP nick end labeling (TUNEL) was used to detect apoptotic cells. To visualize DNA fragmentation of apoptotic cells, we performed TUNEL staining using an in situ BrdU-Red DNA Fragmentation assay kit (Cat No. ab66110, Abcam, Cambridge, Massachusetts) according to the manufacturer's instructions with minor modifications. Every sixth section throughout the frontal cortex and hippocampus was used for TUNEL analysis and each section was 180 µm apart. Briefly, free-floating sections were rinsed with PBS and then treated with 20 μg/ml proteinase K for 5 min at room temperature. Then, endogenous peroxidase activity was blocked by incubation with 3% hydrogen peroxide in 100% methanol for 5 min. The sections were rinsed with PBS and incubated with the DNA Labeling Solution at 37°C for 1 h in the dark. The sections were then rinsed and incubated with anti-BrdU-red antibody solution in dark for 30 min at room temperature, followed by incubation in 7-AAD/RNase A staining buffer in the dark for 30 min at room temperature. Sections were then rinsed, mounted onto slides (VWR, West Chester, Pennsylvania), dried and cover slipped with Prolong Gold containing 4′,6-diamidino-2-phenylindole (DAPI, Cat No. P36935, Life Technologies).
Following staining, red TUNEL-positive cells from every sixth coronal section containing hippocampus and cortex were visualized using an FSX 100 microscope (Olympus, Tokyo, Japan). The positive cells from bilateral hippocampi and cortex were manually counted in a blinded fashion at higher magnification (×40) from a total of 6 sections per animal and 5 animals per group. The mean for each animal (from the 6 sections) then was averaged to obtain the group mean.
Statistical analysis
Statistical analysis was performed on raw data using Prism 5.01 software (San Diego, California), with data presented as mean ± SEM. Where appropriate, one- or two-way ANOVA and Bonferroni’s post hoc multiple comparison test was used to compare the difference among regions or treatment groups. Statistical significances were determined at level of p < .05.
RESULTS
Deltamethrin Toxicity
We observed no overt toxicity or behavioral changes in mice following a single oral exposure to 6 mg/kg of deltamethrin. This was evidenced by the lack of classic signs of pyrethroid toxicity, including tremor, salivation, choreoathetosis, and ataxia.
Activation of the ER Stress Pathway in the Cortex and Hippocampus Following Acute Exposure to Deltamethrin
To determine whether acute single exposure deltamethrin induces ER stress in vivo, ER stress-related proteins in the cortex and hippocampus were determined by Western blot analysis. Deltamethrin exposure (6 mg/kg) caused ER stress as evidenced by significantly increased levels of CHOP and GRP-78 in both the hippocampus and frontal cortex. CHOP was increased by 148% in the hippocampus at 24 and 48 h following deltamethrin treatment (Figure 1A), but in the cortex, CHOP was increased by 146% at 48 h (Figure 1B). Similarly, the level of GRP-78 was increased by 314% and 262% in the hippocampus at 24 and 48 h (Figure 1C), whereas they were increased by 178% at 24 h and 139% at 48 h in the frontal cortex (Figure 1D). Compared with cortex, the hippocampus showed a more robust effect to ER stress (Table 1).
Comparison of ER Stress and Apoptotic Markers Between Hippocampus and Cortex Following a Single Oral Exposure to 6 mg/kg of Deltamethrin in Mice
Time Point . | Brain Regions . | Caspase-12 . | CHOP . | GRP-78 . | Caspase-3 . |
---|---|---|---|---|---|
24 h | Hippocampus | 668.67 ± 11.82** | 144.99 ± 4.69* | 314.53 ± 34.44** | 102.21 ± 3.09 |
Cortex | 177.85 ± 9.99 | 101.17 ± 3.71 | 178.82 ± 25.59 | 100.64 ± 5.85 | |
48 h | Hippocampus | 313.51 ± 92.31** | 147.94 ± 4.46 | 262.12 ± 40.69** | 249.09 ± 11.96** |
Cortex | 171.68 ± 7.71 | 146.72 ± 5.46 | 139.19 ± 12.05 | 171.97 ± 15.11 |
Time Point . | Brain Regions . | Caspase-12 . | CHOP . | GRP-78 . | Caspase-3 . |
---|---|---|---|---|---|
24 h | Hippocampus | 668.67 ± 11.82** | 144.99 ± 4.69* | 314.53 ± 34.44** | 102.21 ± 3.09 |
Cortex | 177.85 ± 9.99 | 101.17 ± 3.71 | 178.82 ± 25.59 | 100.64 ± 5.85 | |
48 h | Hippocampus | 313.51 ± 92.31** | 147.94 ± 4.46 | 262.12 ± 40.69** | 249.09 ± 11.96** |
Cortex | 171.68 ± 7.71 | 146.72 ± 5.46 | 139.19 ± 12.05 | 171.97 ± 15.11 |
Protein levels of CHOP, GRP-78, caspase-12, and caspase-3 in the hippocampus and frontal cortex were determined by Western blot analysis at 24 and 48 h after deltamethrin exposure and presented as relative pixel density. Statistical analyses were performed on raw data using one-way ANOVA followed by Bonferroni’s post hoc test to compare differences between hippocampus and cortex. The values represent mean ± SEM from 5 animals per group. Asterisks indicate significant differences from cortex when compared with hippocampus (*p < .05, **p < .01).
Comparison of ER Stress and Apoptotic Markers Between Hippocampus and Cortex Following a Single Oral Exposure to 6 mg/kg of Deltamethrin in Mice
Time Point . | Brain Regions . | Caspase-12 . | CHOP . | GRP-78 . | Caspase-3 . |
---|---|---|---|---|---|
24 h | Hippocampus | 668.67 ± 11.82** | 144.99 ± 4.69* | 314.53 ± 34.44** | 102.21 ± 3.09 |
Cortex | 177.85 ± 9.99 | 101.17 ± 3.71 | 178.82 ± 25.59 | 100.64 ± 5.85 | |
48 h | Hippocampus | 313.51 ± 92.31** | 147.94 ± 4.46 | 262.12 ± 40.69** | 249.09 ± 11.96** |
Cortex | 171.68 ± 7.71 | 146.72 ± 5.46 | 139.19 ± 12.05 | 171.97 ± 15.11 |
Time Point . | Brain Regions . | Caspase-12 . | CHOP . | GRP-78 . | Caspase-3 . |
---|---|---|---|---|---|
24 h | Hippocampus | 668.67 ± 11.82** | 144.99 ± 4.69* | 314.53 ± 34.44** | 102.21 ± 3.09 |
Cortex | 177.85 ± 9.99 | 101.17 ± 3.71 | 178.82 ± 25.59 | 100.64 ± 5.85 | |
48 h | Hippocampus | 313.51 ± 92.31** | 147.94 ± 4.46 | 262.12 ± 40.69** | 249.09 ± 11.96** |
Cortex | 171.68 ± 7.71 | 146.72 ± 5.46 | 139.19 ± 12.05 | 171.97 ± 15.11 |
Protein levels of CHOP, GRP-78, caspase-12, and caspase-3 in the hippocampus and frontal cortex were determined by Western blot analysis at 24 and 48 h after deltamethrin exposure and presented as relative pixel density. Statistical analyses were performed on raw data using one-way ANOVA followed by Bonferroni’s post hoc test to compare differences between hippocampus and cortex. The values represent mean ± SEM from 5 animals per group. Asterisks indicate significant differences from cortex when compared with hippocampus (*p < .05, **p < .01).
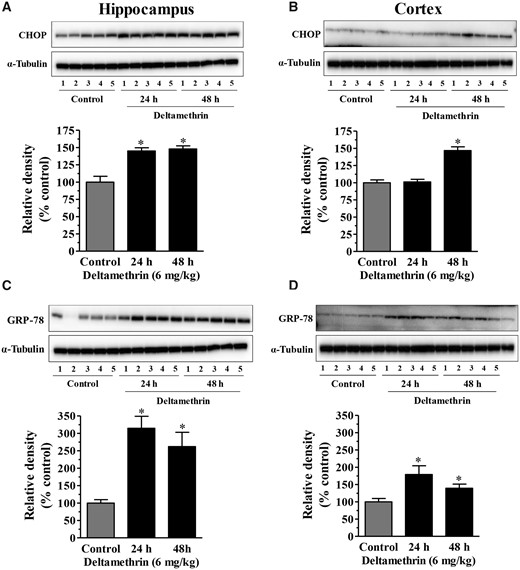
Oral administration of a single dose of deltamethrin (6 mg/kg) causes ER stress in the hippocampus and cortex of adult mice. Levels of CHOP in hippocampus (A) and frontal cortex (B). Levels of GRP-78 in hippocampus (C) and frontal cortex (D). Induction of CHOP and GRP-78 proteins were determined by Western blot analysis at 24h and 48h following deltamethrin exposure. α-Tubulin was used as a loading control. Relative densities are presented in bar graphs and representative blots are provided above the graphs. The values represent mean ± SEM from 5 animals per group. Asterisks indicate significantly different from control (Hippocampus: f = 19.25; df = 4; p < .0001 for CHOP and f = 10.26; df = 4; p < .003 for GRP-78. Cortex: CHOP: f = 35.04; df = 4; p < .0001 for CHOP and f = 5.24; df = 4; p < .02).
Single Acute Exposure to Deltamethrin Induces Apoptosis in the Mouse Cortex and Hippocampus
We next measured protein levels of active caspases in the cortex and hippocampus, as ER stress-mediated apoptotic signaling can occur through the sequential activation of caspase-12 and caspase-3. Deltamethrin treatment resulted in a significant increase in activated caspase-12 (Figure 2) and caspase-3 (Figure 3) in both brain regions. The levels of caspase-12 were increased by 668% at 24 and 313% at 48 h in the hippocampus (Figure 2A). In contrast, caspase-12 was increased by 177% at 24 h and 171% at 48 h in the frontal cortex following deltamethrin exposure (Figure 2B). These data demonstrate that deltamethrin-treated animals exhibited a 491% greater increase in activated caspase-12 in the hippocampus compared with the cortex at the 24 h time point (Table 1). Deltamethrin increased the activation of caspase-3 by 249% in the hippocampus (Figure 3A) and by 171% in the cortex (Figure 3B) at 48 h after deltamethrin exposure, representing a 78% greater effect in hippocampus compared with the cortex (Table 1).
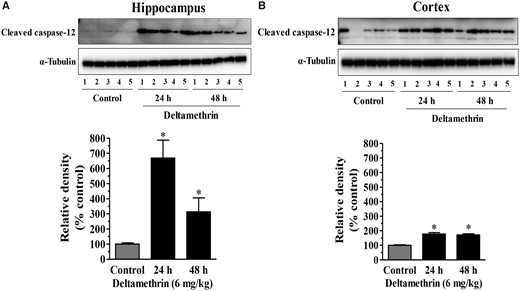
Time course activation of caspase-12 following a single oral exposure to deltamethrin (6 mg/kg). Protein levels of caspase-12 in the hippocampus (A) and frontal cortex (B) were determined by Western blot analysis. Relative densities are presented in bar graphs and representative blots are provided above the graphs. α-Tubulin was used as a loading control. The values represent mean ± SEM from 5 animals per group. Asterisk indicates significantly different from control (Hippocampus: f = 10.90; df = 4; p < .002 and Cortex: f = 32.77; df = 4; p < .0001).

Single oral exposure to deltamethrin (6 mg/kg) activates caspase-3 in the hippocampus and cortex of adult mice. Western blot analysis was used to assess cleaved caspase-3 protein levels in the hippocampus and prefrontal cortex at 24 and 48h after deltamethrin exposure. Protein levels of activated caspase-3 in hippocampus (A) and frontal cortex (B). α-Tubulin was used as a loading control. Relative densities are presented in bar graphs and a representative blot is provided above the graph. The values represent mean ± SEM from 5 animals per group. Asterisk indicates significantly different from control (Hippocampus: f = 139.9; df = 4; p < .001 and Cortex: f = 17.29; df = 4; p < .0003).
Salubrinal Prevents Activation of the ER Stress and Caspase Pathway Following Exposure to Deltamethrin
Salubrinal selectively inhibits dephosphorylation of the α subunit of eukaryotic translation initiation factor 2 (eIF2α), which is an integral component of the ER stress pathway (Boyce et al., 2005). To determine whether inhibition of eIF2α prevents the induction of ER stress by deltamethrin, animals were treated with salubrinal (1 mg/kg, i.p.) prior to deltamethrin administration. Western blot analysis revealed the induction of CHOP by deltamethrin was completely prevented by salubrinal (Figs. 4A and 4B).
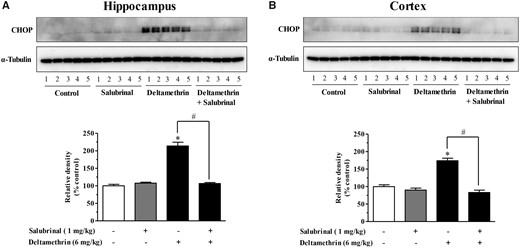
Salubrinal pretreatment prevents activation of the ER stress pathway following a single oral exposure to deltamethrin (6 mg/kg) in adult mice. Mice were given two i.p. injections of 1 mg/kg salubrinal 24 h and 30 min before the administration of deltamethrin. Levels of CHOP in hippocampus (A) and frontal cortex (B) were measured 48 h after deltamethrin administration. Protein levels of CHOP in hippocampus (A) and frontal cortex (B) were measured by Western blot analysis. α-Tubulin was used as loading control. Relative densities are presented in bar graphs and a representative blot is provided above the graph. The values represent mean ± SEM from 5 animals per group. * indicates significantly different from control and # indicates significant differences from deltamethrin when compared with deltamethrin + salubrinal (Hippocampus: f = 79.98; df = 4; p < .0001 and Cortex: f = 46.78; df = 4; p < .0001).
To determine the effects of salubrinal on deltamethrin-induced caspase signaling, Western blot analysis was used and revealed the significant induction of activated caspase-3 in the both hippocampus (Figure 5A) and frontal cortex (Figure 5B). Inhibition of ER stress by salubrinal completely prevented deltamethrin-induced activation of caspase-3 (Figs. 5A and 5B).
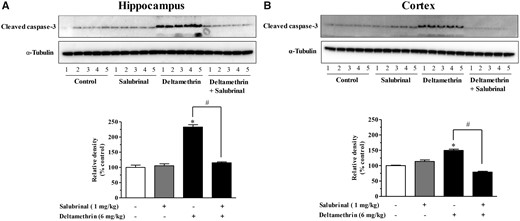
Inhibition of ER stress with salubrinal prevents activation of caspase-3 in the brain of adult mice following exposure to deltamethrin. Mice were given two i.p. injections of 1 mg/kg salubrinal 24 h and 30 min before the administration of deltamethrin. Protein levels of activated caspase-3 in hippocampus (A) and frontal cortex (B) were measured by Western blot analysis at 48 h following deltamethrin administration. α-Tubulin was used as loading control. Relative densities are presented in bar graphs and a representative blot is provided above the graph. The values represent mean ± SEM from 5 animals per group. * indicates significantly different from control and # indicates significant differences from deltamethrin when compared with deltamethrin + salubrinal (Hippocampus: f = 38.03; df = 4; p < .0001 and Cortex: f = 69.34; df = 4; p < .0001).
To determine whether activated caspase signaling resulted in apoptosis, we visualized DNA fragmentation of apoptotic cells with TUNEL staining (Figure 6). We found that deltamethrin significantly increased the number of TUNEL-positive cells in the hippocampus (Figure 6A) and the frontal cortex (Figure 6B) when compared with the control animals. There was an 880% increase in the number of TUNEL-positive cells observed in the dentate gyrus area of the hippocampus when compared with the cortex. Inhibition of ER stress with salubrinal significantly reduced the number of TUNEL-positive cells in the both hippocampus (Figure 6A) and frontal cortex (Figure 6B) of deltamethrin-treated mice, indicating that deltamethrin-induced apoptosis associated with activation of the ER stress pathway.
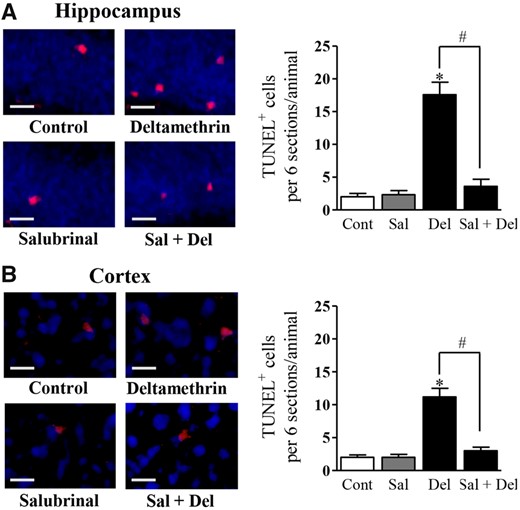
Single oral exposure to deltamethrin (6 mg/kg) causes neuronal apoptosis and salubrinal preventens deltamethrin-induced apoptotic cell death in the brain of adult mice. Mice were given two i.p. injections of 1 mg/kg salubrinal 24 h and then 30 min before the administration of deltamethrin. Apoptotic cells (red) were visualized with TUNEL staining in the dentate gyrus (DG) of hippocampus (A) and prefrontal cortex (B) at 48 h after deltamethrin administration. Scale bar = 200 µM. The values represent mean ± SEM from 4 animals per group. * indicates significantly different from control and # indicates significant differences from deltamethrin when compared with deltamethrin + salubrinal (Hippocampus: f = 46.33; df = 3; p < .0001 and Cortex: f = 38.11; df = 3; p < .0001).
DISCUSSION
Emerging evidence indicates that chronic and sustained ER stress plays a pivotal role in the pathogenesis of neurodegeneration in many neurological diseases (Tsujii et al., 2015; Zhao et al., 2015) and metabolic disorders (Zhang et al., 2013). Previously, we reported that the pyrethroid pesticide deltamethrin causes ER stress and apoptosis in vitro (Hossain and Richardson, 2011), and recently, we found that repeated exposure to low levels of deltamethrin causes hippocampal ER stress as well as deficits in learning in adult mice (Hossain et al., 2015). In this study, we investigated regional differences in ER stress and apoptosis following oral administration of a single dose of deltamethrin. Our results demonstrate that a single exposure to deltamethrin leads to ER stress in both the hippocampus and cortex, but that the hippocampus displays a much more robust response. Furthermore, we demonstrate the ability of salubrinal, an ER stress inhibitor, to reduce ER stress and subsequent apoptosis following deltamethrin exposure.
The effect of environmental exposure on human health is a significant concern, as exposure to toxicants is associated with increased risk of chronic diseases (Baldi et al., 2003; Baltazar et al., 2014; Cannon and Greenamyre, 2011). Numerous epidemiological studies and laboratory-based experiments have demonstrated that pesticides exposure contributes to neurodegeneration (Cannon and Greenamyre, 2011; Tanner et al., 2014). ER stress has recently been identified as a potential common mechanism of neurotoxicity in a variety of neurodegenerative diseases, including both Alzheimer’s and Parkinson’s diseases (Hoozemans et al., 2009; Lindholm et al., 2006; Salminen et al., 2009; Tsujii et al., 2015; Zhang et al., 2013). Furthermore, persistent activation of the ER stress pathway is linked to progressive loss of neurons leading to cognitive dysfunction (Hotamisligil, 2010; Salminen et al., 2009), which may be the result of reduced synaptic plasticity and neuronal viability in the hippocampus (Hotamisligil, 2010). A recent study from our laboratory demonstrated that long-term repeated deltamethrin (3 mg/kg every 3 days for 2 months) exposure causes impairment of hippocampal neurogenesis and deficits in learning in adult mice that are associated ER stress (Hossain et al., 2015). Here, we found that a single dose of deltamethrin (6 mg/kg) was capable of eliciting acute ER stress in the cortex and hippocampus of adult male mice, as evidenced by increased levels of GRP-78 and CHOP 24 and 48 h following deltamethrin treatment.
Induction of GRP-78 and CHOP are classic molecular markers of ER stress (Zheng et al., 2014). These proteins are typically expressed at very low basal levels under physiological conditions and are increased when ER experiences stressful conditions (Li et al., 2014; Nishitoh, 2012; Oyadomari and Mori, 2004). GRP-78 is a major ER chaperone protein critical for protein folding, as well as regulating activation of the ER-transmembrane signaling molecules, including protein kinase R (PKR)-like ER kinase (PERK) to restore ER function. Following ER stress, induction of GRP-78 results in activation of PERK and activated PERK then phosphorylates and inactivates eIF2α, which inhibits mRNA translation to prevent further protein synthesis in an attempt to reduce ER stress (Cai et al., 2014). Conversely, excessive phosphorylation of eIF2α leads to activation of CHOP, a key player initiating apoptosis in ER stress (Marciniak et al., 2004) through suppression of the anti-apoptotic molecule Bcl-2 (Kim et al., 2008; Xu et al., 2005) and deletion of CHOP protects it against lethal consequences (Ji et al., 2005). Here, we observed that inhibition of eIF2α with salubrinal abolished the increases in CHOP, demonstrating that activation of the ER stress pathway by deltamethrin works through eIF2α. This finding is consistent with our previously reported in vitro data demonstrating that deltamethrin exposure, through interaction with neuronal Na+ channels, resulting in a secondary increase in intracellular Ca++ and activation of the ER stress-apoptotic pathway (Hitomi et al., 2004; Hossain and Richardson, 2011).
Apoptosis plays a crucial role during normal development and maintains proper function of organisms throughout life (Fuchs and Steller, 2011). However, dysregulation of apoptosis can lead to pathological cell death in a wide ranges of diseases, including neurodegenerative diseases (Arduino et al., 2009). The ER plays a crucial role in the storage of Ca2+ to regulate intracellular Ca2+ homeostasis (Verkhratsky, 2005) and release of calcium stores from the ER can lead to activation of calpain and a caspase cascade, leading to DNA fragmentation and apoptosis in vitro following exposure to deltamethrin (Hossain and Richardson, 2011; Morishima et al., 2002). Among the number of caspases, activation of caspase-3 is the most critical event of DNA fragmentation leading to apoptotic cell death, which can occur independently without mitochondrial cytochrome c release via activation of the ER (Hossain and Richardson, 2011; Sano and Reed, 2013). ER stress can directly initiate and execute apoptotic cell death by activating caspase-12 (Hitomi et al., 2004), as we and others previously observed (Hitomi et al., 2004; Hossain and Richardson, 2011). In this study, mice administered a single oral administration of deltamethrin displayed an early and robust increase in active caspase-12, which was followed by increases in active caspase-3 in both the cortex and hippocampus of adult mice. Similar to the ER stress markers, active caspase-3 levels were significantly higher in the hippocampus of deltamethrin-treated mice than in the cortex. To determine the relationship between ER stress and apoptosis, we pre-treated mice with salubrinal, a specific inhibitor of eIF2α, which resulted in prevention of caspase-3 activation in both the cortex and hippocampus. Although activation of caspase-3 is necessary for induction of apoptosis, it does not provide information on whether cells are actually undergoing apoptosis. To address this issue, we performed TUNEL staining and confirmed the active caspase-3 data and that salubrinal pre-treatment significantly reduced the number of TUNEL-positive cells. Collectively, these data provide mechanistic confirmation that single acute exposure to deltamethrin causes apoptosis in the adult mouse brain through activation of the ER stress pathway, as suggested by our previous in vitro (Hossain and Richardson, 2011) and in vivo studies (Hossain et al., 2015).
An important finding of our studies is the identification that the adult hippocampus appears to be more vulnerable to deltamethrin compared with other brain regions, specifically the cortex. This vulnerability is evidenced by greater induction of ER stress markers and caspase activation in the hippocampus compared with the cortex 24 and 48 h following deltamethrin treatment. The mechanisms responsible for the apparent sensitivity of the hippocampus to deltamethrin are currently not known. Anadon et al. (1996) reported that rats administered a single oral dose of 26 mg/kg deltamethrin exhibited higher levels of deltamethrin in the hippocampus compared with the cortex. However, whether pharmacokinetic or pharmacodynamic mechanisms contribute to this effect remains to be established, as well as whether or not a similar relationship would be observed in mice and at lower doses. From a pharmacokinetic standpoint, differences in regional brain lipid content are known, with the hippocampus containing roughly 20% more lipids than the cortex (Chavko et al., 1993), which might contribute to greater accumulation of deltamethrin. From a pharmacodynamic standpoint, it has been reported that the Na+ current density and number of Na+ channels are higher in the hippocampus than the cortex (Xia et al., 2003), which might contribute to significant regional differences in ER stress response and apoptosis following deltamethrin exposure. This apparent greater sensitivity of the hippocampus to deltamethrin-induced ER stress and apoptosis has significant implications for potential adverse behavioral effects previously observed following deltamethrin exposure (Hossain et al., 2015). The hippocampus plays integral roles in learning and various types of memory formation, consolidation and retrieval, particularly as it relates to spatial and contextual information (Jarrard, 1993). Furthermore, eIF2α plays an important role in learning and memory (Costa-Mattioli et al., 2007) and genetic or pharmacological inhibition of eIF2α has been shown to restore synaptic function and learning and memory in a variety of neurodegenerative disease models, including prion diseased mice and tauopathy mice (Freeman and Mallucci, 2016). Thus, additional studies are required to determine the relationship between deltamethrin-induced ER-stress and neurobehavioral dysfunction. Likewise, additional studies are required to determine the relative susceptibility of additional brain regions and whether or not the effects persist following a single exposure to deltamethrin.
In conclusion, this study identifies the critical role of ER stress in causing apoptosis in the cortex and hippocampus of adult mice following a single exposure to deltamethrin, with the hippocampus apparently being much more sensitive. Furthermore, the finding that the ER stress inhibitor salubrinal could significantly reduce or prevent deltamethrin-induced ER stress and apoptosis provides a potential pharmacological intervention that may reduce or prevent the effects of deltamethrin on ER stress and cognitive function (Hossain et al., 2015).
FUNDING
This work was supported in part by National Institutes of Health (1R01ES027481-01A1 (to M.M.H.) and R01ES021800, R01ES026057, and U01NS079249 (to J.R.R.)). The content of this article is solely the responsibility of the authors and does not necessarily represent the official views of the National Institutes of Health.
Comments