-
PDF
- Split View
-
Views
-
Cite
Cite
Taylor D Harrison, Elizabeth M Chaney, Kiernan J Brandt, Taylor B Ault-Seay, Liesel G Schneider, Lew G Strickland, F Neal Schrick, Kyle J McLean, The effects of differing nutritional levels and body condition score on scrotal circumference, motility, and morphology of bovine sperm, Translational Animal Science, Volume 6, Issue 1, January 2022, txac001, https://doi.org/10.1093/tas/txac001
- Share Icon Share
Abstract
Bulls often experience various levels of nutrient availability throughout the year. Nutritional management is a critical factor on overall ejaculate composition and the ability to get females pregnant. We hypothesized that differing nutritional levels and body condition score (BCS) affect reproductive fertility parameters in bulls. Mature Angus bulls (n = 11) were individually housed and randomly assigned to one of two dietary regimens: 1) over-fed (n = 5) or 2) restricted (n = 6). Bulls were fed the same ration at different volumes to achieve desired effects resulting in eight individual treatments: gain to an over-fed body condition score ([BCS]; GO), gain after nutrient restriction (GR), loss after an over-fed BCS (LO), loss from nutrient restriction (LR), maintenance at ideal adiposity (BCS = 6) after overfeeding (IMO), maintenance at ideal adiposity after nutrient restriction (IMR), maintenance at an over-fed BCS (BCS = 8; MO), and maintenance at a restricted BCS (BCS = 4; MR). Body weight (BW) and BCS were recorded every 2 wk to monitor bull weight and BCS changes. Scrotal circumference was measured every 28 d. Body fat and sperm motility and morphology were evaluated every 84 d. Scrotal circumference, motility, and morphology were normalized to the initial value of each bull. Thus, allowing the individual bull to serve as a control. Statistical analyses were conducted with PROC GLIMMIX of SAS as a complete randomized design to determine if treatment influenced BW, BCS, scrotal circumference, motility, morphology, and adipose thickness. Scrotal circumference (P < 0.001) had the least amount of deviation from initial during the LR (0.29 ± 0.44) treatment and the greatest during the MO (3.06 ± 0.44), LO (2.28 ± 0.44), MR (2.43 ± 0.44), GR (3.03 ± 0.44), and IMR (2.91 ± 0.44) treatments. Sperm motility was not affected by nutritional treatments (P = 0.55). Both head and total defects of sperm differed (P = 0.02) due to nutritional treatments. Increased head abnormalities occurred during the LO (37.60 ± 8.61) treatment, with no differences between the other treatments. Total defects increased during the LO (43.80 ± 9.55) treatment with similar increases in bulls during the GR (29.40 ± 9.55) and IMR (35.60 ± 9.55) treatments. In conclusion, male fertility was impacted when a deviation from a BCS of 6 occurred which could be detrimental to reproductive and beef production efficiency.
INTRODUCTION
There is a critical need for efficient beef production due to the predicted exponential growth of the world’s human population (Reynolds et al., 2015). As countries become more developed, diets change from a larger proportion of starches to more protein, causing an estimated increase of 200 million tons of meat to be needed by 2050 (FAO, 2009). Efforts to increase cattle production have largely been focused on the female due to the long-term interaction and influence on the offspring (Funston et al., 2012; Endecott et al., 2013; Diskin and Kenny, 2014). However, the paternal contribution to production efficiency may be greater than previously anticipated due to a sire having multiple offspring per breeding season. Bull fertility can be negatively impacted by many factors including the environment and nutrition (Parkinson, 1987; NRC, 2000; Thomas, 2009). Sire nutrition is a major limiting factor for male reproductive performance (Short and Adams, 1988) which can affect overall fertility (Singh et al., 2018) and potentially lower pregnancy rates (Coulter et al., 1999; Singh et al., 2018). Bulls can lose a considerable amount of weight (45 to 135 kg) during the breeding season due to mating and reduced feed intake (NRC, 2000; Barth, 2018). Therefore, continued evaluation of the interaction between diet and semen quality is necessary to maximize reproductive efficiency through nutritional optimization.
To aid in the production of increased semen quality, proper nutritional management should be adjusted depending on bull age and time of year (Leathem, 1975; NRC, 2000). Nutrient restriction can negatively affect male fertility via poor testicular development, diminished libido, reduced progressive forward motility, and increased morphological defects (Mwansa and Makarechian, 1991; Singh et al., 2018). Prolonged nutritional restriction has also influenced the interstitial, Sertoli, and Leydig cell populations which alters testicular steroidogenesis and spermatogenesis (NRC, 2000; Bollwein et al., 2017). Interestingly, over nutrition can also impact reproductive performance. Greater intake levels can decrease ejaculate volume, sperm concentration, motility, and morphology of the sperm (Coulter et al., 1997; Perkovic S, 2001) as well as create a hormonal imbalance (Tremellen et al., 1998; Selvaraju et al., 2012; Singh et al., 2018), all of which can impact male fertility. Pre-pubertal bulls (8 to 25 wk of age) receiving elevated concentrate levels (37% concentrate) had increased GnRH-stimulated testosterone production compared to bulls on a diet without concentrate (Barth et al., 2008). Increasing energy levels by 20% has been reported to improve sperm velocity and motility as well as mitochondrial membrane potential and integrity in rams (Selvaraju et al., 2012). Thus, nutritional management of the sire prior to the breeding season is a critical factor for reproductive efficiency. Therefore, the hypothesis of the current study is that prolonged alterations to nutritional plane and body condition score (BCS) impact industry standard fertility measurements of mature bulls.
MATERIALS AND METHODS
All experimental procedures were approved by the University of Tennessee Institutional Animal Care and Use Committee protocol no. 2713-0819.
Experimental Design and Sample Collection
Mature Angus bulls (n = 12; body weight [BW] = 738 kg; BCS = 6; Age = 4 yr) were purchased from Jorgensen Land & Cattle (Ideal, SD). One bull developed bovine leucosis and was required to be euthanized during the study; thus, it was excluded from all analyses. All bulls were individually housed in a 2.44- by 12.19-m paddock with ad libitum access to water and provided a 100 g mineral supplement daily (CO-OP Supreme Cattle Mineral; Tennessee Farmers Cooperative; Lavergne, TN). Bulls were provided feed to target intakes to meet BW and BCS goals as per experimental design. The diet consisted of 35% ground hay, 35% cracked corn, 20% dried distillers’ grain, and 10% soybean meal. Prior to the initiation of treatments, a breeding soundness exam (BSE) was conducted to assess fertility status. All bulls were required to pass a BSE with pre-treatment average scrotal circumference 38.52 ± 1.45 cm (34 cm minimum), forward progressive motility averaged 45.8 ± 7.3% (30 % minimum), and morphological defects were 19.9 ± 5.9%, 5.9 ± 3.9%, 0.64 ± 1.02%, and 26.5 ± 6.1% for head, mid piece, tail, and total (70 % minimum), respectively. Following a 21-d adaptation period, each bull was randomly assigned to one of two dietary regimens, either restricted or over-fed initial diets (Figure 1), to achieve eight individual treatments: gain to an over-fed BCS (GO), maintenance at an over-fed BCS (BCS = 8; MO), loss after an over-fed BCS (LO), maintenance at ideal adiposity (body condition score [BCS] = 6) after overfeeding (IMO), loss from nutrient restriction (LR), maintenance at a restricted BCS (BCS = 4; MR), gain after nutrient restriction (GR), and maintenance at ideal adiposity after nutrient restriction (IMR). On day 21, restricted bulls began with the LR treatment, targeting a decrease of 2 BCS over 84 d. On day 105, restricted bulls began adaptation to diet intake adequate for maintenance at an abnormal BCS of 4 over 10 d and began the MR treatment. On day 189, restriction bulls began re-alimentation (GR treatment) back to initial basal BW and BCS with intakes targeting a BCS increase of 2. Bulls assigned to the over-fed regimen were subjected to inverse dietary changes of the restricted regimen. Following the 21 d adaptation, over-fed bulls started with the GO treatment and received an intake volume to support ~1.25 kg/d to increase BCS by 2 in 84 d. On day 105, over-fed bulls were acclimated to the abnormal BCS of 8 during the MO treatment with intakes adequate for maintenance at the new BW and remained on this treatment for 84 d. On day 189, over-fed bulls began the return to basal BW and BCS by targeting a 2 BCS decrease in 84 d during the LO treatment. On day 273, all bulls received intake levels to support maintenance at an ideal (BCS = 6) condition level, IMO or IMR, depending on if the bull was over-fed (IMO) or restricted (IMR) over 84 d to complete the objectives.
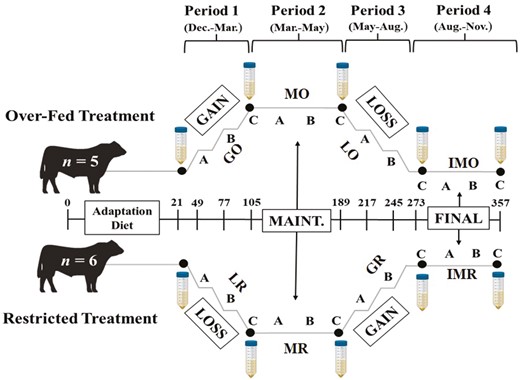
Project timeline with two dietary regimens: Over-fed and Restricted, with four respective nutritional periods per regimen. Sample collections followed a 21-d diet adaptation period prior to treatments. The treatments include Gain to an Over-fed BCS (GO), Maintenance at an Over-fed BCS (MO), Loss after an Over-fed BCS (LO), Maintenance at Ideal Adiposity after Overfeeding (IMO), Loss from Nutrient Restriction (LR), Maintenance at Restriction BCS (MR), Gain after Nutrient Restriction (GR), and Maintenance at Ideal Adiposity after Nutrient Restriction (IMR). Each treatment includes semen collection for morphology and motility for initial and every 84 d (large falcon tubes), and scrotal circumference measurements for days 28, 56, and 84 per nutritional period, respectively (A, B, and C).
Semen Collection and Analysis
Individual non-shrunk BW and BCS (1 = emaciated and 9 = obese [Wagner et al., 1988]) was taken every 14 d to monitor changes and ensure treatments met experimental goals. Two trained individuals collected BCS at each sampling and the average of these values were utilized during analyses. Scrotal circumference was measured using the Reliabull scrotal tape (Lane Manufacturing Inc., Denver, CO) every 28 d during each nutritional treatment, yielding three measurements per treatment at days 28, 56, and 84 corresponding to samples A, B, and C, respectively (Figure 1). Sperm, hip height, back fat, rump fat, and scrotal fat were quantified every 84 d. Body fat was measured by a single Centralized Ultrasound Processing lab certified ultrasound technician via ultrasonography with an IBEX EVO II (E.I. Medical Imaging, Loveland, CO).
Back fat thickness was quantified from images taken over the 11th, 12th, and 13th ribs. Rump fat thickness was quantified from images taken from the right side of the animal with the ultrasound probe placed on the caudal end of the hook bone and extending towards the tail head. Scrotal fat thickness was quantified from images taken at the widest area of the scrotum. All fat thickness measurements were calculated by averaging three distinct measurements quantified in Image-J. Prior to electro-ejaculation, trans-rectal palpation was conducted to ensure normal internal tract morphology. To collect the ejaculate, a semen collection handle with a saline bag was connected to a disposable cone and vial. The attached saline bag was kept in a heated water bath (~37 °C) to keep the ejaculate at a constant temperature during and after collection to eliminate environmental temperature effects on the sperm. The progressively forward motile sperm was subjectively evaluated in 5% increments with a Fischer Scientific Light microscope under 400× magnification after dilution with a drop of warm saline. A sample of each ejaculate was stained with Eosin Nigrosin morphology stain (Lane Manufacturing Inc., Denver, CO) to assess sperm morphology. Percentage of abnormal sperm was quantified by Diplomate of American College of Theriogenologists, by counting individual sperm with defects out of a total of 100 sperm under 1000× magnification. Individual sperm were assessed from head to tail; thus, sperm with more than one defect would be classified as the defect occurring closest to the head of the sperm. The same bottle of morphology stain was used throughout the entire study to avoid variation in morphological measurements due to osmolality issues between bottles.
Statistical Analyses
Bulls were randomly assigned to either the overfed or the restricted regimen. Within each dietary regimen, every bull underwent every treatment. Statistical analyses were performed using GLIMMIX SAS 9.4 (SAS Institute, Cary, NC) to determine the fixed effect of nutritional treatment on motility, morphology, scrotal circumference, back fat, rump fat, and scrotal fat. The experimental unit was each individual bull. Experimental model confirmation was done by evaluating BW and BCS to assess if predicted BW and BCS were reached through dietary volume adjustments for each designated treatment. This statistical model of BW and BCS included date and nutritional treatment for BW, BCS, and scrotal circumference. A covariate of hip height was included when evaluating BW and BCS. The initial value for scrotal circumference, motility, and morphology was subtracted from each subsequent value to determine deviation from that animals’ starting value prior to the onset of treatments, allowing for each bull to serve as their own respective control. Means were determined to be different when P ≤ 0.05 and a tendency when P ≤ 0.10.
RESULTS
BW and BCS
The covariate, hip height, had no effect on BCS; however, there was an (P < 0.0001) effect of hip height on BW. Both BW and BCS were influenced (P < 0.001) by a treatment × date interaction. Body weight tended to differ at the beginning of the study, but both BW and BCS followed the predicted experimental design model (Table 1). However, neither dietary regimen fully achieved the desired BCS predicted (over-fed = 8; restricted = 4). The four maintenance periods, MO (BW = 855.42 ± 19.27 kg; BCS = 6.17 ± 0.16), MR (BW = 718.44 ± 17.59 kg; BCS = 4.84 ± 0.15), IMO (BW = 811.64 ± 19.27 kg; BCS = 5.22 ± 0.16), and IMR (BW = 845.64 ± 17.59 kg; BCS = 5.84 ± 0.15), were successful in maintaining bulls at a steady BW and BCS as desired by the model (Table 1). Bulls on the restricted dietary regimen decreased to a BW of 695.34 ± 17.59 kg and BCS of 4.09 ± 0.15 on the LR treatment, whereas the over-fed dietary regimen increased BW to 888.14 ± 19.27 kg and BCS to 6.84 ± 0.16 on the GO treatment (Table 1). Bulls on the GR treatment gained to a BW of 833.97 ± 17.59 kg and a BCS of 6.09 ± 0.15, and bulls on the LO treatment decreased BW to 812.51 ± 19.27 kg and BCS to 5.24 ± 0.16 (Table 1).
Body weight and body condition score for each nutritional treatment per individual sampling date through the designated dietary regimens
Nutritional periods . | Sample, d . | Nutritional treatments . | BW, kg1 . | BCS1 . |
---|---|---|---|---|
Initial | 21 | Over-fed | 718.32w,2 | 5.89a |
Restricted | 762.61x | 6.09a | ||
Period 1 | 49 | GO | 801.96a,4 | 6.04a |
LR | 742.31b | 4.96b | ||
77 | GO | 854.32a | 6.49a | |
LR | 723.22b | 4.67b | ||
105 | GO | 888.14a | 6.84a | |
LR | 695.34b | 4.09b | ||
Period 2 | 133 | MO | 855.23a | 6.29a |
MR | 720.94b | 4.76b | ||
161 | MO | 856.69a | 6.04a | |
MR | 718.67b | 4.96b | ||
189 | MO | 855.60a | 6.04a | |
MR | 715.94b | 4.92b | ||
Period 3 | 217 | LO | 840.32w | 5.79a |
GR | 791.55x | 5.51a | ||
245 | LO | 824.14a | 5.49a | |
GR | 810.34a | 5.76a | ||
273 | LO | 812.51a | 5.24a | |
GR | 833.97a | 6.09b | ||
Period 4 | 301 | IMO | 807.05a | 5.24a |
IMR | 836.85a | 6.05b | ||
329 | IMO | 809.78a | 5.44a | |
IMR | 833.22a | 5.88b | ||
357 | IMO | 816.32a | 5.20a | |
IMR | 854.43a | 5.63b |
Nutritional periods . | Sample, d . | Nutritional treatments . | BW, kg1 . | BCS1 . |
---|---|---|---|---|
Initial | 21 | Over-fed | 718.32w,2 | 5.89a |
Restricted | 762.61x | 6.09a | ||
Period 1 | 49 | GO | 801.96a,4 | 6.04a |
LR | 742.31b | 4.96b | ||
77 | GO | 854.32a | 6.49a | |
LR | 723.22b | 4.67b | ||
105 | GO | 888.14a | 6.84a | |
LR | 695.34b | 4.09b | ||
Period 2 | 133 | MO | 855.23a | 6.29a |
MR | 720.94b | 4.76b | ||
161 | MO | 856.69a | 6.04a | |
MR | 718.67b | 4.96b | ||
189 | MO | 855.60a | 6.04a | |
MR | 715.94b | 4.92b | ||
Period 3 | 217 | LO | 840.32w | 5.79a |
GR | 791.55x | 5.51a | ||
245 | LO | 824.14a | 5.49a | |
GR | 810.34a | 5.76a | ||
273 | LO | 812.51a | 5.24a | |
GR | 833.97a | 6.09b | ||
Period 4 | 301 | IMO | 807.05a | 5.24a |
IMR | 836.85a | 6.05b | ||
329 | IMO | 809.78a | 5.44a | |
IMR | 833.22a | 5.88b | ||
357 | IMO | 816.32a | 5.20a | |
IMR | 854.43a | 5.63b |
BW SEM for Over-fed and Restricted was 19.27 and 17.59 and BCS SEM was 0.16 and 0.15, respectively.
Within a column, means without a common letter differ for BW and BCS (P < 0.10).
Period 1: Over-fed regimen = GO treatment; Restricted regimen = LR treatment.
Within a column, means without a common letter differ for BW and BCS (P < 0.05).
Both treatments per dietary regimen—Period 2: MO and MR treatments; Period 4: IMO and IMR treatments.
Period 3: Over-fed regimen = LO treatment; Restricted regimen = GR treatment.
Body weight and body condition score for each nutritional treatment per individual sampling date through the designated dietary regimens
Nutritional periods . | Sample, d . | Nutritional treatments . | BW, kg1 . | BCS1 . |
---|---|---|---|---|
Initial | 21 | Over-fed | 718.32w,2 | 5.89a |
Restricted | 762.61x | 6.09a | ||
Period 1 | 49 | GO | 801.96a,4 | 6.04a |
LR | 742.31b | 4.96b | ||
77 | GO | 854.32a | 6.49a | |
LR | 723.22b | 4.67b | ||
105 | GO | 888.14a | 6.84a | |
LR | 695.34b | 4.09b | ||
Period 2 | 133 | MO | 855.23a | 6.29a |
MR | 720.94b | 4.76b | ||
161 | MO | 856.69a | 6.04a | |
MR | 718.67b | 4.96b | ||
189 | MO | 855.60a | 6.04a | |
MR | 715.94b | 4.92b | ||
Period 3 | 217 | LO | 840.32w | 5.79a |
GR | 791.55x | 5.51a | ||
245 | LO | 824.14a | 5.49a | |
GR | 810.34a | 5.76a | ||
273 | LO | 812.51a | 5.24a | |
GR | 833.97a | 6.09b | ||
Period 4 | 301 | IMO | 807.05a | 5.24a |
IMR | 836.85a | 6.05b | ||
329 | IMO | 809.78a | 5.44a | |
IMR | 833.22a | 5.88b | ||
357 | IMO | 816.32a | 5.20a | |
IMR | 854.43a | 5.63b |
Nutritional periods . | Sample, d . | Nutritional treatments . | BW, kg1 . | BCS1 . |
---|---|---|---|---|
Initial | 21 | Over-fed | 718.32w,2 | 5.89a |
Restricted | 762.61x | 6.09a | ||
Period 1 | 49 | GO | 801.96a,4 | 6.04a |
LR | 742.31b | 4.96b | ||
77 | GO | 854.32a | 6.49a | |
LR | 723.22b | 4.67b | ||
105 | GO | 888.14a | 6.84a | |
LR | 695.34b | 4.09b | ||
Period 2 | 133 | MO | 855.23a | 6.29a |
MR | 720.94b | 4.76b | ||
161 | MO | 856.69a | 6.04a | |
MR | 718.67b | 4.96b | ||
189 | MO | 855.60a | 6.04a | |
MR | 715.94b | 4.92b | ||
Period 3 | 217 | LO | 840.32w | 5.79a |
GR | 791.55x | 5.51a | ||
245 | LO | 824.14a | 5.49a | |
GR | 810.34a | 5.76a | ||
273 | LO | 812.51a | 5.24a | |
GR | 833.97a | 6.09b | ||
Period 4 | 301 | IMO | 807.05a | 5.24a |
IMR | 836.85a | 6.05b | ||
329 | IMO | 809.78a | 5.44a | |
IMR | 833.22a | 5.88b | ||
357 | IMO | 816.32a | 5.20a | |
IMR | 854.43a | 5.63b |
BW SEM for Over-fed and Restricted was 19.27 and 17.59 and BCS SEM was 0.16 and 0.15, respectively.
Within a column, means without a common letter differ for BW and BCS (P < 0.10).
Period 1: Over-fed regimen = GO treatment; Restricted regimen = LR treatment.
Within a column, means without a common letter differ for BW and BCS (P < 0.05).
Both treatments per dietary regimen—Period 2: MO and MR treatments; Period 4: IMO and IMR treatments.
Period 3: Over-fed regimen = LO treatment; Restricted regimen = GR treatment.
Scrotal Circumference and Body Fat
Scrotal circumference was affected (P < 0.001) by nutritional treatments (Figure 2). The LR treatment exhibited the smallest change in scrotal circumference (0.29 ± 0.44 cm) from the initial sample prior to treatment. The MO (3.06 ± 0.44 cm), LO (2.28 ± 0.44 cm), MR (2.43 ± 0.44 cm), GR (3.03 ± 0.44 cm), and IMR (2.91 ± 0.44 cm) were all similar in scrotal circumference change from initial. Yet the GO (2.13 ± 0.44 cm) and IMO (1.74 ± 0.44 cm) treatments were intermediate. All scrotal circumference changes were positive which may indicate that even in mature bulls, scrotal size continues to grow with age (Figure 2). In contrast to scrotal circumference measurements, the scrotal fat measurements had no significant treatment period or interaction effects (P > 0.05). Back fat (Figure 3) and rump fat (Figure 4) thicknesses were impacted by nutritional treatments (P < 0.001). Like BW and BCS, back fat had increased (P < 0.001; Figure 3) thickness during the GO and GR treatment (0.77 ± 0.04 cm), the least during the MR treatment (0.41 ± 0.04 cm), the LR treatment (0.44 ± 0.04 cm), and IMO treatment (0.49 ± 0.04 cm), and MO, LO, and IMR being intermediate in back fat thickness. Rump fat thickness (P < 0.001; Figure 4) was greater during the GO (1.13 ± 0.09 cm), MO (0.88 ± 0.09 cm), GR (0.97 ± 0.09 cm), and IMR (1.13 ± 0.09 cm) treatments compared with all others. The MR treatment (0.27 ± 0.09 cm) had less rump fat than LO (0.53 ± 0.09 cm) and IMO and LR were intermediate between the two (0.45 ± 0.09 cm and 0.39 ± 0.09 cm, respectively). Both back and rump fat followed the experimental model according to the treatments of each respective dietary regimen.
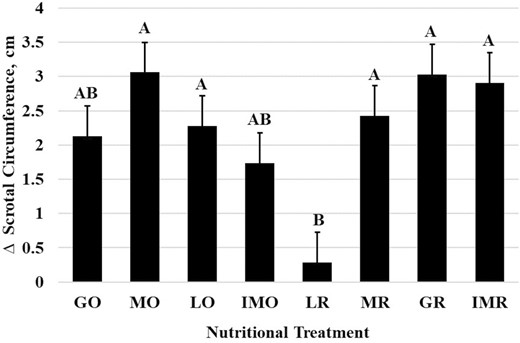
The effects of nutritional treatment on the change of scrotal circumference. Initial scrotal circumference measurements were subtracted from each nutritional treatment period for each bull. ABBars (arithmetic means ± SEM) that do not share a letter denote differences at P ≤ 0.05.
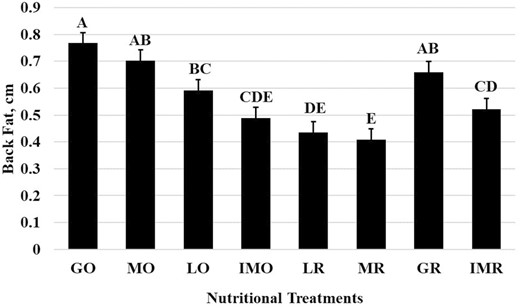
Back fat measurements for each nutritional treatment (Raw Mean ± SEM; P < 0.001). ABCDEBars (raw means ± SEM) that do not share a letter denote differences at P ≤ 0.05.
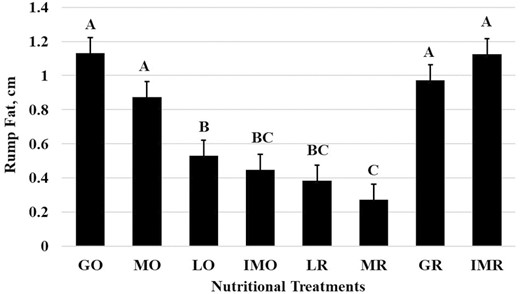
Rump fat measurements (Raw Mean ± SEM; P < 0.0001) for the nutritional treatments. ABCBars (raw means ± SEM) that do not share a letter denote differences at P ≤ 0.05.
Motility and Morphology
Forward progressive motility was not impacted by nutritional treatments (P = 0.55, Table 2). The change in morphological head abnormalities when compared with the initial morphology prior to treatments was influenced (P = 0.02; Table 2) by nutritional treatments. The greatest increase in head abnormalities occurred during the LO (37.60 ± 8.61). The treatments including the GO (−7.67 ± 8.61), MO (1.00 ± 8.61), IMO (1.80 ± 8.61), LR (−2.40 ± 8.61), MR (−4.50 ± 8.61), GR (12.00 ± 8.61), and IMR (10.60 ± 8.61) were all similar. The sperm defects for midpiece (P = 0.47) and tail (P = 0.84) were not influenced by nutritional treatments (Table 2). In contrast, when evaluating the change in total sperm abnormalities, there was a nutritional treatment effect (P = 0.02; Table 2). The greatest increase for total defects occurred during the LO (43.80 ± 9.55) treatment, whereas no change occurred during the LR (0.00 ± 9.55) treatment. Similarly, the GO (−3.33 ± 9.55) treatment for the OVER bulls had a decrease in total defects. The MO (17.20 ± 9.55), IMO (14.60 ± 9.55), MR (11.67 ± 9.55), GR (29.40 ± 9.55), and IMR (35.60 ± 9.55) had fluctuating intermediate values according to treatment.
Change in motility (%) and morphology (#/100) from the initial collection prior to treatments for the designated nutritional treatments according to dietary regimens1
. | Nutritional treatments2,3 . | Pooled SE . | P-Value . | |||||||
---|---|---|---|---|---|---|---|---|---|---|
GO . | MO . | LO . | IMO . | LR . | MR . | GR . | IMR . | |||
∆ Motility, % | −6.00a | −13.00a | −12.00a | 4.00a | −9.17a | −0.83a | −14.17a | −2.50a | ± 7.02 | 0.55 |
∆ Head Defects | −7.67b | 1.00b | 37.60a | 1.80b | −2.40b | −4.50b | 12.00b | 10.60b | ± 8.61 | 0.02 |
∆ Midpiece Defects | 3.00a | 13.60a | 9.00a | 12.60a | 2.20a | 18.33a | 18.00a | 22.60a | ± 7.29 | 0.47 |
∆ Tail Defects | 1.33a | 7.20a | 1.40a | 4.60a | 3.60a | 2.33a | 4.40a | 5.40a | ± 2.93 | 0.84 |
∆ Total Defects | −3.33c | 17.20abc | 43.80a | 14.60bc | 0.00c | 11.67bc | 29.40ab | 35.60ab | ± 9.55 | 0.02 |
. | Nutritional treatments2,3 . | Pooled SE . | P-Value . | |||||||
---|---|---|---|---|---|---|---|---|---|---|
GO . | MO . | LO . | IMO . | LR . | MR . | GR . | IMR . | |||
∆ Motility, % | −6.00a | −13.00a | −12.00a | 4.00a | −9.17a | −0.83a | −14.17a | −2.50a | ± 7.02 | 0.55 |
∆ Head Defects | −7.67b | 1.00b | 37.60a | 1.80b | −2.40b | −4.50b | 12.00b | 10.60b | ± 8.61 | 0.02 |
∆ Midpiece Defects | 3.00a | 13.60a | 9.00a | 12.60a | 2.20a | 18.33a | 18.00a | 22.60a | ± 7.29 | 0.47 |
∆ Tail Defects | 1.33a | 7.20a | 1.40a | 4.60a | 3.60a | 2.33a | 4.40a | 5.40a | ± 2.93 | 0.84 |
∆ Total Defects | −3.33c | 17.20abc | 43.80a | 14.60bc | 0.00c | 11.67bc | 29.40ab | 35.60ab | ± 9.55 | 0.02 |
Within a row, means without a common letter differ for motility and morphology (P < 0.05).
GO, Gain to an over-fed BCS; MO, Maintenance at an over-fed BCS; LO, Loss after an over-fed BCS; IMO, Maintenance at ideal adiposity after overfeeding.
LR, Loss from nutrient restriction; MR, Maintenance after nutrient restriction; GR, Gain after nutrient restriction; IMR, Ideal maintenance condition after nutrient restriction.
Change in motility (%) and morphology (#/100) from the initial collection prior to treatments for the designated nutritional treatments according to dietary regimens1
. | Nutritional treatments2,3 . | Pooled SE . | P-Value . | |||||||
---|---|---|---|---|---|---|---|---|---|---|
GO . | MO . | LO . | IMO . | LR . | MR . | GR . | IMR . | |||
∆ Motility, % | −6.00a | −13.00a | −12.00a | 4.00a | −9.17a | −0.83a | −14.17a | −2.50a | ± 7.02 | 0.55 |
∆ Head Defects | −7.67b | 1.00b | 37.60a | 1.80b | −2.40b | −4.50b | 12.00b | 10.60b | ± 8.61 | 0.02 |
∆ Midpiece Defects | 3.00a | 13.60a | 9.00a | 12.60a | 2.20a | 18.33a | 18.00a | 22.60a | ± 7.29 | 0.47 |
∆ Tail Defects | 1.33a | 7.20a | 1.40a | 4.60a | 3.60a | 2.33a | 4.40a | 5.40a | ± 2.93 | 0.84 |
∆ Total Defects | −3.33c | 17.20abc | 43.80a | 14.60bc | 0.00c | 11.67bc | 29.40ab | 35.60ab | ± 9.55 | 0.02 |
. | Nutritional treatments2,3 . | Pooled SE . | P-Value . | |||||||
---|---|---|---|---|---|---|---|---|---|---|
GO . | MO . | LO . | IMO . | LR . | MR . | GR . | IMR . | |||
∆ Motility, % | −6.00a | −13.00a | −12.00a | 4.00a | −9.17a | −0.83a | −14.17a | −2.50a | ± 7.02 | 0.55 |
∆ Head Defects | −7.67b | 1.00b | 37.60a | 1.80b | −2.40b | −4.50b | 12.00b | 10.60b | ± 8.61 | 0.02 |
∆ Midpiece Defects | 3.00a | 13.60a | 9.00a | 12.60a | 2.20a | 18.33a | 18.00a | 22.60a | ± 7.29 | 0.47 |
∆ Tail Defects | 1.33a | 7.20a | 1.40a | 4.60a | 3.60a | 2.33a | 4.40a | 5.40a | ± 2.93 | 0.84 |
∆ Total Defects | −3.33c | 17.20abc | 43.80a | 14.60bc | 0.00c | 11.67bc | 29.40ab | 35.60ab | ± 9.55 | 0.02 |
Within a row, means without a common letter differ for motility and morphology (P < 0.05).
GO, Gain to an over-fed BCS; MO, Maintenance at an over-fed BCS; LO, Loss after an over-fed BCS; IMO, Maintenance at ideal adiposity after overfeeding.
LR, Loss from nutrient restriction; MR, Maintenance after nutrient restriction; GR, Gain after nutrient restriction; IMR, Ideal maintenance condition after nutrient restriction.
DISCUSSION
The role of the sire is often underestimated, since females are responsible for large nutrient investments and long-term interactions with the calf. The bull does not provide any nutrients to the offspring but is responsible for half of the genetic information and most of the postnatal performance in numerous calves per breeding season. Semen quality of the sire can be hindered through many external and environmental factors such as age, season, nutrition, collection frequency, and injuries (Almquist, 1982; Senger, 2012; Kastelic, 2013; Murphy et al., 2018; Tank and Monke, 2020). Specifically, factors causing temperature changes within the scrotum such as injuries and diseases from inflammation and fevers as well as season and nutrition will decrease semen quality in response to elevated body temperature (Vogler et al., 1993). Bulls often experience various nutritional planes throughout the year due to a short and extreme period of increased activity during the breeding season, with most of the year spent with relatively low activity levels. Nutrition is generally accepted as the limiting factor of sire fertility; thus, any fluctuations can be detrimental to reproductive herd efficiency (Short and Adams, 1988). Since diet has been shown to impact fertility parameters within the ejaculate, we evaluated the motility and morphology of bulls undergoing different levels of nutrition. Even though neither dietary regimen fully achieved the desired BW and BCS, both BW and BCS were different, confirming the success of the nutritional treatment and experimental design. However, BCS during the IMR treatment was increased compared to the IMO treatment, potentially due to changes in maintenance requirements after undergoing restricted treatment (NRC, 2000). Changes in nutrient requirements after different planes of nutrition should be considered so that overcompensation does not occur, specifically following the breeding season in bulls.
Scrotal circumference is a heritable trait that is an essential fertility marker due to increased testicular size correlating to an increase in sperm output and also carries over into heifer fertility by reducing age at puberty (Martin et al., 1992; Youngquist and Threlfall, 2006; Latif et al., 2009). Scrotal circumference is a valued and important trait on bull fertility that can be influenced by nutrition (Barth et al., 2008). This agrees with the results of the current dataset where nutritional plane affected scrotal circumference even though the bulls in these data were mature and not growing bulls. Elevated energy and protein diets have been reported to increase scrotal circumference (Mwansa and Makarechian, 1991; Coulter et al., 1997; Coulter et al., 1999; Barth et al., 2008). The increase in adipose deposition from overfeeding can have negative effects (Coulter et al., 1997; Coulter et al., 1999) or no effect (Schrick, 1998; Lemaster, 1999) on spermatogenesis. Scrotal fat in our study was not influenced by nutritional treatments and changes in scrotal circumference measurements only occurred during the LR period of the restricted dietary regimen. Therefore, mature bulls may not readily deposit fat in the scrotum until extreme BCS is achieved.
The increase in morphological abnormalities associated with overfeeding is speculated to be related to adipose deposition within the scrotum (Coulter and Kozub, 1984). The current data show that morphology was influenced the greatest during the LO treatment for bulls returning to ideal BCS; however, no scrotal fat differences were observed from ultrasonography. In contrast, prolonged nutritional deficiencies can hinder spermatogenesis and decrease testes size (Hurley and Doane, 1989). This result also agrees with this current study, where decreases in scrotal circumference were observed during the LR treatment. Moreover, data within demonstrate that deviating from and back to ideal BCS of 6 do not negatively impact motility. Similar to these data, developing bulls on different types of nitrogen from natural or purified urea or isolated soy protein did not affect fertility parameters like motility (Johnson et al., 1971). In other studies, motility was affected by nutrition but seemed to recover in bull that underwent different nutritional periods (Flipse and Almquist, 1961; Pakenas, 1966).
Seminal plasma (SP) can enhance sperm motility (Graham, 1994); however, certain components of SP including inflammatory cytokines like tumor necrosis factor-α have decreased the motility of human sperm during malnutrition (Eisermann et al., 1989). Increases in cytokines could have occurred during abnormal nutritional periods due to response to inflammation and stress (Eckel and Ametaj, 2016). Thus, a possible association between nutritional stress and motility may exist in bulls (Flipse and Almquist, 1961), boars (Stevermer et al., 1961), rodents (Ghanayem et al., 2010), and humans (Skoracka et al., 2020).
Even though forward progressive motility is vital for sperm to reach the egg, morphological defects are one of the greatest causes for pregnancy failures (Thundathil et al., 2002; Walters et al., 2005). As the sperm head contains the genetic material and key effectors of fertilization, most abnormalities of the head are associated with fertility impairment (Wilmington, 1981). The fact that head defects were greatest during the LO treatment may indicate that returning to ideal BCS after being overfed is more detrimental to morphology than when losing BW from a BCS of 6. Furthermore, the midpiece defects, which potentially occurred from nutritional stress within the epididymis, were highest in the IMR period. Similarly, other research found bulls decreased from elevated nutritional periods, continued abnormal production of sperm potentially due to impaired heat exchange mechanisms (Coulter and Kozub, 1984). Furthermore, the total sperm defects also increased the greatest during the LR treatment potentially due to the acquired fat deposition from nutritional stress of overfeeding (Skinner, 1981).
In conclusion, sperm morphology fluctuated throughout nutritional periods, whereas motility was not affected. Nutritionally preparing bulls for the breeding season is vital to ensure optimal fertility by increasing or maintaining slightly elevated BCS. Within the current study, the MO, LO, and LR treatments resembled the nutritional levels and potentially the morphological and motility effects that could occur during the breeding season. Overall, nutritional treatments influenced semen quality at different adiposity and BCS levels that bulls undergo in normal production scenarios. Further studies are need in order to fully understand the long-term impacts on the ejaculate due to sire diet and reproductive efficiency.
ACKNOWLEDGMENTS
We thank Hugh Moorehead, Matt Backus, Kevin Thompson, and the Middle Tennessee Research and Education Center at Lewisburg for animal care and aide collecting samples. Additionally, we thank the state of Tennessee through UT AgResearch, the Department of Animal Science, and the USDA National Institute of Food and Agriculture, Hatch Project No. 1019048 for providing funding and support for this research.
Conflict of interest statement
Authors declare no conflict of interest exists.