-
PDF
- Split View
-
Views
-
Cite
Cite
Ana Clara B Menezes, Sebastião C V. Filho, Pauliane Pucetti, Marcos V C. Pacheco, Letícia A Godoi, Diego Zanetti, Herlon M Alhadas, Mário F Paulino, Joel S Caton, Oscillating and static dietary crude protein supply: II. Energy and protein requirements of young Nellore bulls, Translational Animal Science, Volume 3, Issue 4, July 2019, Pages 1216–1226, https://doi.org/10.1093/tas/txz139
- Share Icon Share
Abstract:
The objective of this study was to evaluate whole body chemical composition and energy and protein nutrient requirements for maintenance and gain of Nellore bulls. Fifty young bulls, with an average age of 7 ± 1 mo and initial body weight (BW) of 260.0 ± 8.1 kg, were used in this experiment. Four bulls were used as baseline reference animals and were slaughtered at the beginning of the experiment. Four bulls were fed at maintenance (12 g dry matter [DM]/kg of BW), whereas 42 bulls were divided into six groups (n = 7/group) and were randomly assigned to the following dietary treatments 105 (low [LO]), 125 (medium [MD]), or 145 (high [HI]) g crude protein (CP)/kg DM, LO to HI (LH), LO to MD (LM), or MD to HI (MH) oscillating CP at a 48-h interval for 140 d. At the end of the experiment, bulls were slaughtered and samples of the whole body were collected. All samples were lyophilized, ground, and composed as percentage of component of empty body weight (EBW) from each bull. A power model was used to estimate carcass, non-carcass components, and gastrointestinal content of the shrunk body weight (SBW), and CP and water present in the empty body, whereas an exponential model was used to estimate adipose tissue and ether extract (EE) present in the EBW. Nonlinear regression equations were developed to predict heat production from metabolizable energy (ME) intake and retained energy (RE). The net energy requirements for maintenance and ME for maintenance were 77 and 122.75 kcal/EBW0.75/d, respectively. The efficiency of ME utilization for maintenance was 62.7%. The equation obtained for net energy for gain (NEg) was: NEg (Mcal/EBW0.75/d) = 0.0535 × EBW0.75 × EBG0.7131, where EBG is the empty body gain, and the efficiency was 24.25%. Net protein requirement for growth (NPg) was: NPg (g/d) = 227.372 × EBG – 19.479 × RE. There was a linear increase for carcass, CP, and water present in the EBW as the animal grew. The EE deposition exponentially increased as EBW increased.
INTRODUCTION
Bos indicus represent a large portion of the global cattle herd as more than half of the cattle in the world are maintained in tropical environments (Cundiff et al., 2012). Bos indicus are predominant in the Brazilian herd, the world’s largest commercial herd (ANUALPEC, 2017); furthermore, about 40% of the beef cows in the United States are located in relatively hot and humid subtropics of the Southeast or more arid subtropics of the Southwest, where the Bos indicus genetics play a critical role in providing heterosis for beef production (Cundiff et al., 2012). Bos indicus cattle have metabolic and physiologic differences compared with Bos taurus, implying potential differences in nutrient utilization, which is supported by currently available separate requirement systems (the BR-CORTE system; Valadares Filho et al., 2016; NASEM, 2016, respectively).
Appropriate formulation of diets to meet the nutritional requirements of zebu cattle during growing and finishing phases will result in reduced excretion of polluting compounds, like N, without decreasing cattle performance (Menezes et al., 2016). It is known that animal requirements change over time (Robertson et al. 1970), therefore, considering the adoption of feed systems, like oscillation of dietary CP levels, that adjust diets according to the animals’ growth stage is an essential tool to improve production systems from both economic and environmental perspectives. A more effective diet formulation to optimize the use of protein can reduce dietary costs, since protein is considered the most expensive nutrient in beef cattle diets (Appuhamy et al., 2014), and the reduction in N excretion by meeting the ruminally degradable protein and metabolizable protein requirements of animals, without decreasing performance, has great potential to reduce the environmental impact of beef cattle production and increase economic returns for producers.
Despite this interest, to the best of our knowledge, there are limited data about metabolizable energy (ME) and protein requirements of feedlot young Nellore bulls. Therefore, this article calls into question the need to improve estimates of ME and protein requirements to accurately formulate diets to Bos indicus cattle, considering the global importance of this genetic. The objectives of this study were to evaluate the whole body chemical composition and establish the energy and protein requirements for maintenance and gain of young Nellore bulls.
MATERIALS AND METHODS
Animals, Experimental Design, and Treatments
The experiment was conducted at the Experimental Feedlot of the Animal Science Department at the Universidade Federal de Viçosa (UFV), Viçosa, Minas Gerais, Brazil. Animal care and handling followed guidelines set by the UFV (process 59/2016). Dietary crude protein (CP) levels were determined according to the protein requirements for Nellore bulls suggested by the BR-CORTE system (Valadares Filho et al., 2016), where 125 g CP/kg dry matter (DM) was established as the adequate CP concentration for bulls in this age and weight category. Therefore, we used 125 g CP/kg DM as our medium, or average, treatment.
Fifty weaned Nellore bulls, average 7 ± 1 mo of age and with an average initial body weight (BW) of 260.0 ± 8.1 kg were used in this trial. The experiment was conducted in a completely randomized design, where the experimental units (bulls) were assigned to treatments at random, allowing every bull equal probability of receiving a treatment. Four bulls were randomly selected as the baseline reference group, being slaughtered at the beginning of the experiment to evaluate initial body composition. Four bulls were fed at a maintenance level (12 g DM/kg initial BW), housed in individual pens equipped with concrete feeders and received a 125 g CP/kg DM-based diet. The remaining 42 bulls were fed ad libitum and were randomly assigned to receive one of the six diets (n = 7 bulls per treatment) with different CP concentrations for 140 d, either: 1) Low (LO; 105 g CP/kg DM), 2) Medium (MD; 125 g CP/kg DM), 3) High (HI; 145 g CP/kg DM), 4) Low to High (LH; Oscillating CP concentration of 105–145 g CP/kg DM at a 48-h interval), 5) Low to Medium (LM; oscillating CP concentration of 105 to 125 g CP/kg DM at a 48-h interval), and 6) Medium to High (MH; Oscillating CP concentration of 125–145 g CP/kg DM at a 48-h interval). The chemical composition of the three diets used in this experiment is presented in Table 1. Briefly, the Low diet (105 g CP/.kg DM) provided 673.2 g rumen degradable protein (RDP)/kg CP, and 326.8 g rumen undegradable protein (RUP)/kg CP; the Medium diet (125 g CP/kg DM) provided 696.7 g RDP/kg CP, and 303.3 g RUP/kg CP; and the High diet (145 g CP/kg DM) provided 713.7 g RDP/kg CP, and 286.3 g RUP/kg CP.
Proportion of ingredients and nutrient composition of the experimental diets
Item . | Experimental diets1 . | ||
---|---|---|---|
. | Low . | Medium . | High . |
Proportion | |||
Corn Silage | 50.0 | 50.0 | 50.0 |
Ground corn | 39.4 | 39.4 | 39.4 |
Soybean meal | 2.38 | 4.92 | 7.46 |
Wheat bran | 6.09 | 3.05 | 0.00 |
Urea | 0.47 | 0.98 | 1.49 |
Salt | 0.30 | 0.30 | 0.30 |
Limestone | 0.06 | 0.06 | 0.06 |
Mineral mix2 | 0.29 | 0.29 | 0.29 |
Sodium bicarbonate | 0.75 | 0.75 | 0.75 |
Magnesium oxide | 0.25 | 0.25 | 0.25 |
Total | 100 | 100 | 100 |
Chemical composition | |||
Dry matter, g/kg as-fed | 406.0 | 406.1 | 406.2 |
Organic matter, g/kg DM | 944.1 | 943.8 | 943.5 |
Crude protein, g/kg DM | 102.7 | 122.3 | 141.9 |
Rumen degradable protein, g/kg CP | 673.2 | 696.7 | 713.7 |
Rumen undegradable protein, g/kg CP | 326.8 | 303.3 | 286.3 |
Ether extract, g/kg DM | 42.8 | 42.1 | 41.4 |
Neutral detergent fiber, g/kg DM | 321.3 | 314.2 | 307.1 |
Indigestible neutral detergent fiber, g/kg DM | 98.09 | 95.7 | 93.4 |
Non-fiber carbohydrates, g/kg DM | 480.6 | 476.2 | 471.8 |
Item . | Experimental diets1 . | ||
---|---|---|---|
. | Low . | Medium . | High . |
Proportion | |||
Corn Silage | 50.0 | 50.0 | 50.0 |
Ground corn | 39.4 | 39.4 | 39.4 |
Soybean meal | 2.38 | 4.92 | 7.46 |
Wheat bran | 6.09 | 3.05 | 0.00 |
Urea | 0.47 | 0.98 | 1.49 |
Salt | 0.30 | 0.30 | 0.30 |
Limestone | 0.06 | 0.06 | 0.06 |
Mineral mix2 | 0.29 | 0.29 | 0.29 |
Sodium bicarbonate | 0.75 | 0.75 | 0.75 |
Magnesium oxide | 0.25 | 0.25 | 0.25 |
Total | 100 | 100 | 100 |
Chemical composition | |||
Dry matter, g/kg as-fed | 406.0 | 406.1 | 406.2 |
Organic matter, g/kg DM | 944.1 | 943.8 | 943.5 |
Crude protein, g/kg DM | 102.7 | 122.3 | 141.9 |
Rumen degradable protein, g/kg CP | 673.2 | 696.7 | 713.7 |
Rumen undegradable protein, g/kg CP | 326.8 | 303.3 | 286.3 |
Ether extract, g/kg DM | 42.8 | 42.1 | 41.4 |
Neutral detergent fiber, g/kg DM | 321.3 | 314.2 | 307.1 |
Indigestible neutral detergent fiber, g/kg DM | 98.09 | 95.7 | 93.4 |
Non-fiber carbohydrates, g/kg DM | 480.6 | 476.2 | 471.8 |
1Low = 105 g CP/kg DM; Medium = 125 g CP/kg DM; High =145 g CP/kg DM.
2Mineral mix = 7.83 g S/kg; 5,950 mg Co/kg; 10,790 mg Cu/kg; 1,000 mg Mn/kg; 1,940 mg Se/kg; 1,767.4 mg Zn/kg.
Proportion of ingredients and nutrient composition of the experimental diets
Item . | Experimental diets1 . | ||
---|---|---|---|
. | Low . | Medium . | High . |
Proportion | |||
Corn Silage | 50.0 | 50.0 | 50.0 |
Ground corn | 39.4 | 39.4 | 39.4 |
Soybean meal | 2.38 | 4.92 | 7.46 |
Wheat bran | 6.09 | 3.05 | 0.00 |
Urea | 0.47 | 0.98 | 1.49 |
Salt | 0.30 | 0.30 | 0.30 |
Limestone | 0.06 | 0.06 | 0.06 |
Mineral mix2 | 0.29 | 0.29 | 0.29 |
Sodium bicarbonate | 0.75 | 0.75 | 0.75 |
Magnesium oxide | 0.25 | 0.25 | 0.25 |
Total | 100 | 100 | 100 |
Chemical composition | |||
Dry matter, g/kg as-fed | 406.0 | 406.1 | 406.2 |
Organic matter, g/kg DM | 944.1 | 943.8 | 943.5 |
Crude protein, g/kg DM | 102.7 | 122.3 | 141.9 |
Rumen degradable protein, g/kg CP | 673.2 | 696.7 | 713.7 |
Rumen undegradable protein, g/kg CP | 326.8 | 303.3 | 286.3 |
Ether extract, g/kg DM | 42.8 | 42.1 | 41.4 |
Neutral detergent fiber, g/kg DM | 321.3 | 314.2 | 307.1 |
Indigestible neutral detergent fiber, g/kg DM | 98.09 | 95.7 | 93.4 |
Non-fiber carbohydrates, g/kg DM | 480.6 | 476.2 | 471.8 |
Item . | Experimental diets1 . | ||
---|---|---|---|
. | Low . | Medium . | High . |
Proportion | |||
Corn Silage | 50.0 | 50.0 | 50.0 |
Ground corn | 39.4 | 39.4 | 39.4 |
Soybean meal | 2.38 | 4.92 | 7.46 |
Wheat bran | 6.09 | 3.05 | 0.00 |
Urea | 0.47 | 0.98 | 1.49 |
Salt | 0.30 | 0.30 | 0.30 |
Limestone | 0.06 | 0.06 | 0.06 |
Mineral mix2 | 0.29 | 0.29 | 0.29 |
Sodium bicarbonate | 0.75 | 0.75 | 0.75 |
Magnesium oxide | 0.25 | 0.25 | 0.25 |
Total | 100 | 100 | 100 |
Chemical composition | |||
Dry matter, g/kg as-fed | 406.0 | 406.1 | 406.2 |
Organic matter, g/kg DM | 944.1 | 943.8 | 943.5 |
Crude protein, g/kg DM | 102.7 | 122.3 | 141.9 |
Rumen degradable protein, g/kg CP | 673.2 | 696.7 | 713.7 |
Rumen undegradable protein, g/kg CP | 326.8 | 303.3 | 286.3 |
Ether extract, g/kg DM | 42.8 | 42.1 | 41.4 |
Neutral detergent fiber, g/kg DM | 321.3 | 314.2 | 307.1 |
Indigestible neutral detergent fiber, g/kg DM | 98.09 | 95.7 | 93.4 |
Non-fiber carbohydrates, g/kg DM | 480.6 | 476.2 | 471.8 |
1Low = 105 g CP/kg DM; Medium = 125 g CP/kg DM; High =145 g CP/kg DM.
2Mineral mix = 7.83 g S/kg; 5,950 mg Co/kg; 10,790 mg Cu/kg; 1,000 mg Mn/kg; 1,940 mg Se/kg; 1,767.4 mg Zn/kg.
Each treatment was group-housed in a feedlot pen (48.0 m2) with one electronic feeder (model AF-1000Master; Intergado Ltd., Contagem, Minas Gerais, Brazil) and one electronic waterer per pen (model WD-1000 Master; Intergado Ltd.). Before the experiment, each bull was fitted with an ear tag (left ear) containing a unique radio frequency transponder (FDX-ISO 11784/11785; Allflex, Joinville, Santa Catarina, Brazil). The young bulls were allowed a 21-d acclimation period to the experimental conditions and treated for the control of internal and external parasites by administration of ivermectin (Ivomec; Merial, Paulinia, Brazil). The bulls were weighed at the beginning and end of the experiment after undergoing 16 h of fasting to measure initial and final BW and weighed every 28 d to evaluate and monitor average daily gain and BW. The diets (50:50 forage to concentrate ratio) were formulated according to the BR-CORTE system (Valadares Filho et al., 2016) to achieve an ADG of 1.1 kg and consisted of corn silage and a concentrate that was formulated with ground corn, wheat bran, soybean meal, urea, ammonium sulfate, sodium bicarbonate, salt, and mineral mix (Table 1).
The total mixed rations were provided twice a day, at 0700 and 1600 h. Feed delivery was adjusted daily to maintain minimum refusals the next day and ad libitum intake. The appropriate feed delivery for each group was based on refusal weight every morning. Electronic feeders were evaluated at 0600 h each day to quantify orts and to adjust daily feed delivery to a maximum of 2.5% orts. According to the amount of refusals, the total mixed ration was reduced (more than 2.5% orts at morning evaluation) or increased (less than 2.5% orts at morning evaluation) to reach ad libitum intake. Each treatment was delivered to an electronic feeder that measured daily individual feed intake using the electronic identification tags (model AF-1000 Master; Intergado Ltd.; Chizzotti et al., 2015).
Slaughter and Samplings
The bulls were slaughtered at the end of the experimental trail, after 140 d. Before slaughter, all bulls were fasted from feed 16 h to estimate shrunk body weight (SBW). Bulls were slaughtered via captive bolt stunning followed by exsanguination. The gastrointestinal tract contents (i.e., rumen, reticulum, omasum, abomasum, and small and large intestines) were removed and washed. The weights of the heart, lungs, liver, spleen, kidneys, KPH fat, diaphragm, mesentery, tails, trimmings, and the washed gastrointestinal tract were added to the other parts of the body (i.e., carcass, head, hide, limbs, and blood) to determine empty body weight (EBW). The rumen, reticulum, omasum, abomasum, small and large intestines, KPH fat, mesentery, liver, heart, kidneys, lungs, tongue, spleen, diaphragm, esophagus, trachea, and reproductive tract were ground for 20 min using an industrial cutter to create a homogeneous sample of organs + viscera. The head and all limbs, after removal of the hide, were ground by using a grinding machine (LUNASA, TOL10 model, Araguari, Brazil) to reduce the size of the bones. The hide was manually chopped and sampled. A sample of blood was obtained during the course of bleeding.
After slaughter, the carcass of each bull was separated into two halves that were chilled at 4 ºC for 18 h. Next, half-carcasses were removed from the cold chamber for weighing and the hot and cold carcass yields were calculated. Subcutaneous fat thickness was measured using a digital caliper in the region between 11th and 12th rib cut. The section between the 9th and 11th ribs was collected from the left half-carcass according to procedures described by Hankins and Howe (1946). This section was dissected into muscle, fat, and bone, and each portion was weighed separately. The muscle and fat from the section between the 9th and 11th ribs of each bull were homogenized and ground to obtain a composite sample of muscle and fat. Bones from the same rib section were sliced with a band saw (Skymsen, model SFL-315HD, Santa Catarina, Brazil) in subsections of 1.5-cm length to obtain a representative sample of the bones. The composite sample of muscle and fat and the sample of rib bones were lyophilized and then were ground in a knife mill (Fortinox, Piracicaba, São Paulo, Brazil) with a 1-mm mesh sieve to evaluate the DM, organic matter (OM), N, and ether extract (EE) contents.
Laboratory Analysis
All samples (blood, organs and viscera, head and limbs, hide, muscle and fat, and bones) were quantified in terms of DM, N, and EE according to the AOAC (AOAC, 2012; methods number 934.01, and 981.10 and AOAC, 2006; method number 945.16, respectively). The moisture content was assessed by drying the samples at 105 °C in a hot-air oven until constant weight was achieved. The CP content (N × 6.25) was determined by the Kjeldahl method (Jacobs, 1951), and the assay comprised acid digestion and alkali distillation with an auto Kjeldahl System (2200 Kjeltech auto distillation; Foss Tecator, Hoganas Sweden) followed by titration. The EE was estimated by the solvent extraction method (Socsplus, SCS-08-As, Pelican equipment, Chennai, India) and the assay comprised extraction of lipid with an organic solvent (petroleum ether) at 40–60 °C temperature using a soxhlet apparatus. Digestible energy (DE) intake was obtained by multiplying digestible nutrients by their respective energy values (NRC, 2001): DE = (5.6 × CPIdigestible) + (9.4 × EEIdigestible) + (4.2 × NDFIdigestible) + (4.2 × NFCIdigestible), where the respective digestibility values can be find in Menezes et al. (2019, submitted). The concentration of metabolizable energy intake (MEI) was estimated according to the following equation, MEI = (0.9455 × DE) – 0.3032, as proposed by Detmann et al. (2016).
Calculations
Empty body chemical composition was estimated using the equations described by Marcondes et al. (2012) for Nellore bulls, which were validated by Costa e Silva et al. (2013a):
where CPCor = % CP in the 9th to 11th rib section; EBW = empty body weight; VF = % visceral fat (renal, pelvic, cardiac, and mesenteric fat) per unit of EBW; EECor = % EE in the 9th to 11th rib section; WCor = % water in the 9th to 11th rib section; and OV = % organs and viscera per unit of EBW.
Models were generated from the EBW and body compositions of all animals used in this study. For water and CP, the models used were as follows: Ci = a × EBWb, where Ci is the i body component of the bull, which is the water or CP content in the empty body weight (kg), and a and b are the regression parameters. The EE content in the EBW was estimated by the exponential model: Ci = a × e (b × EBW), in which Ci is the i body component of the bull, which was EE in the empty body weight (kg) and e is the Euler number.
The relationship between SBW and EBW was calculated for all bulls to convert SBW to EBW, whereas the relationship between the ADG and empty body gain (EBG) was calculated to convert ADG to EBG. Body energy content was obtained from body protein and fat contents and their respective caloric equivalents were determined according to the ARC (1980):
The heat production (HP) was calculated based on the difference between MEI and energy content in the body, by using the equation above. Then, the net energy requirement for maintenance (Mcal/EBW0.75/d) was estimated to be the intercept (β0) of the exponential regression between HP and MEI. The following model was used:
where HP = heat production (Mcal/EBW0.75/d), MEI = metabolizable energy intake (MJ/EBW0.75/d), β0 and β1 are regression parameters, and “e” is the Euler number (2.718281). The ME requirement for maintenance (MEm, Mcal/EBW0.75/d) was estimated by the iterative method, with MEm considered to be the value where MEI equals HP.
The efficiency of ME utilization for maintenance was calculated as the ratio between the net energy and ME for maintenance. The net energy requirement for growth (NEg) was estimated from the regression between NEg, EBG, and metabolic EBW by using the following model:
where NEg = the net energy for growth represented as the energy retained in the body (Mcal/d), EBW0.75 = metabolic empty body weight, and EBG = empty body gain (kg/d). Metabolizable protein for maintenance was obtained based on the linear regression between metabolizable protein intake and EBG divided by average EBW0.75, whereas the net protein requirement for growth (NPg) was estimated by a model involving EBG and retained energy in the body:
where NPg = retained protein or the net protein requirement for growth (g/d), EBG = empty body gain (kg/d), RE = retained energy (Mcal/d), and b1 and b2 are regression parameters. The metabolizable protein for gain was estimated by dividing the NPg by the efficiency of metabolizable protein utilization for growth, according to the equation proposed by Valadares Filho et al. (2016).
Statistical Analyses
Statistical procedures were performed using SAS (SAS Inst. Inc., Cary, NC). Data were analyzed in a completely randomized design, with the bull being the experimental unit. Data of reference and maintenance animals were used to estimate energy and protein requirements for young Nellore bulls. A linear regression model between metabolizable protein intake and EBG was analyzed to estimate the net protein requirements for maintenance by using PROC REG (SAS Inst. Inc.). To estimate the net protein requirements for gain and the net energy requirement for maintenance and gain, data were analyzed using nonlinear models through PROC NLIN (SAS Inst. Inc.) and were adjusted by the Gauss–Newton method.
RESULTS AND DISCUSSION
Body Composition
During the course of this study, the bulls had changes in their body composition (Figure 1). Thus, it became necessary to evaluate growth composition throughout the experiment. The growth composition data were used to develop equations to estimate the chemical body composition of young Nellore bulls from their EBW (Table 2).
Equations to estimate chemical body composition from empty body weight (EBW)
Item . | Equation . | R2 . |
---|---|---|
Crude protein, kg | 0.3413 × EBW 0.8882 | 95.56 |
Ether extract, kg | 16.2886 × e(0.0041 × EBW) | 90.44 |
Water, kg | 1.0058 × EBW 0.9017 | 98.86 |
Item . | Equation . | R2 . |
---|---|---|
Crude protein, kg | 0.3413 × EBW 0.8882 | 95.56 |
Ether extract, kg | 16.2886 × e(0.0041 × EBW) | 90.44 |
Water, kg | 1.0058 × EBW 0.9017 | 98.86 |
Equations to estimate chemical body composition from empty body weight (EBW)
Item . | Equation . | R2 . |
---|---|---|
Crude protein, kg | 0.3413 × EBW 0.8882 | 95.56 |
Ether extract, kg | 16.2886 × e(0.0041 × EBW) | 90.44 |
Water, kg | 1.0058 × EBW 0.9017 | 98.86 |
Item . | Equation . | R2 . |
---|---|---|
Crude protein, kg | 0.3413 × EBW 0.8882 | 95.56 |
Ether extract, kg | 16.2886 × e(0.0041 × EBW) | 90.44 |
Water, kg | 1.0058 × EBW 0.9017 | 98.86 |
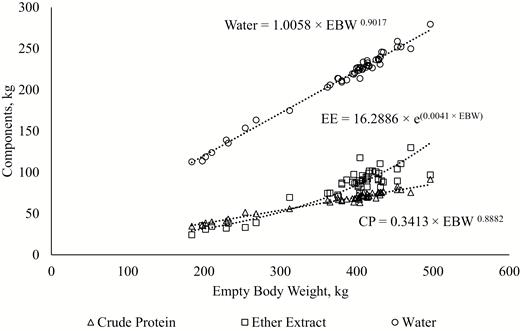
Relationship between the amount of crude protein (CP), ether extract (EE), and water and empty body weight (EBW) of young Nellore bulls.
CP and water content increased with increasing EBW (Figure 1). This is evidenced by the respective equations coefficient, close to 1, as reported in Table 2. On the other hand, EE content increased as the animal reached maturity, indicating that the animal starts to deposit more adipose tissue in proportion to the other tissues thereby resulting in an exponential equation of EE content in the EBW. Costa e Silva et al. (2013b) reported that the chemical composition of growing and finishing Nellore bulls specifically the CP, EE, and mineral content, presented a similar pattern of deposition as that of muscle, adipose, and bone tissues, respectively. This similarity in deposition can be explained because these are the prevailing components of each corresponding tissue. In addition, according to Robelin and Geay (1984), the chemical composition of skeletal muscle tissue varies during animal growth, in which, after birth, protein content increases greatly and then remains constant, and afterward fat increases. The data of the current study agree with the results of Costa e Silva et al. (2013b) and Robelin and Geay (1984).
Relationship Between EBW and SBW, and EBG and ADG
EBW is the most accurate index of energy and nutrient content in the body (Owens et al., 1995). According to Gionbelli et al. (2016), there is a gain in precision and accuracy with the use of a variable EBG/ADG ratio, obtained from the nonlinear model. The equation obtained for the ratio between EBW and SBW was EBW (kg) = 1.3620 × SBW0.9365 (kg). For conversion of ADG in EBG, this study found: EBG = 1.0119 × ADG0.8315. Pacheco (2018) described the relationship between EBG and ADG of young Nellore bulls as EBG = 1.0120 × ADG0.8299, where 1.020 is the EBG/ADG ratio, value similar to 1.0119 found in this study.
Considering a 450 kg Nellore bull gaining 1.2 kg/d raised in a feedlot, the EBW and EBG estimated by these equations were 415.83 kg and 1.18 kg/d, respectively. Although the values estimated by the following equations proposed by Valadares Filho et al. (2016), EBW = 0.8126 × SBW1.0134 and EBG = 0.963 × ADG1.0151 were 396.86 kg and 1.16 kg/d. These data suggest a reduction in the contribution of intestinal fill (gastrointestinal tract contents) in this study compared to Valadares Filho et al. (2016). This reduction can be explained by dietary characteristics, or the proportion of concentrate, which reduces the DM intake and consequently increases the EBW:SBW ratio. Considering the following equations proposed by NASEM (2016), EBW = 0.891 × SBW and EBG = 0.956 × ADG and the same animal used in the previous example, the estimated EBW and EBG were 400.95 kg and 1.14 kg/d, respectively.
For this study, the quantity of digesta (g/kg SBW) was compared with SBW at the time of slaughter (Figure 2), and the carcass and non-carcass components (blood, organs and viscera, head and limbs, and hide) were compared with the SBW for each bull (Figure 3). The equations obtained are reported in Table 3. As SBW increases, there is a decrease in the digesta content that is represented by the negative exponent linked to SBW, which results from a reduction in the contribution of intestinal fill, as suggested in this study by the ratio obtained between EBG and ADG. The correlation coefficient among carcass and SBW was greater than 1.00 and the regression equation had high determination coefficient (R2 = 98.95); thus, with increases in SBW, there is a greater proportion of the carcass component. The equation derived between non-carcass components and SBW additionally shows a positive relationship between these variables (correlation coefficient = 0.9539 and R2 = 94.98). At this point, these correlations have been scarcely studied, so no comparisons could be made with previous studies.
Equations to estimate the contribution of the carcass, non-carcass and gastrointestinal content from shrunk body weight (SBW)
Item1 . | Equation . | R2 . |
---|---|---|
Carcass, kg | 0.3821 × SBW 1.0753 | 98.95 |
Non-carcass, kg | 0.4213 × SBW 0.9539 | 94.98 |
Gastrointestinal content, g/kg SBW | 2228.4 × SBW -0.5537 | 35.65 |
Item1 . | Equation . | R2 . |
---|---|---|
Carcass, kg | 0.3821 × SBW 1.0753 | 98.95 |
Non-carcass, kg | 0.4213 × SBW 0.9539 | 94.98 |
Gastrointestinal content, g/kg SBW | 2228.4 × SBW -0.5537 | 35.65 |
1Carcass = muscle, fat, and bones; Non-carcass = blood, organs and viscera, head, limbs, and hide.
Equations to estimate the contribution of the carcass, non-carcass and gastrointestinal content from shrunk body weight (SBW)
Item1 . | Equation . | R2 . |
---|---|---|
Carcass, kg | 0.3821 × SBW 1.0753 | 98.95 |
Non-carcass, kg | 0.4213 × SBW 0.9539 | 94.98 |
Gastrointestinal content, g/kg SBW | 2228.4 × SBW -0.5537 | 35.65 |
Item1 . | Equation . | R2 . |
---|---|---|
Carcass, kg | 0.3821 × SBW 1.0753 | 98.95 |
Non-carcass, kg | 0.4213 × SBW 0.9539 | 94.98 |
Gastrointestinal content, g/kg SBW | 2228.4 × SBW -0.5537 | 35.65 |
1Carcass = muscle, fat, and bones; Non-carcass = blood, organs and viscera, head, limbs, and hide.

Relationship between the gastrointestinal content and shrunk body weight (SBW) of young Nellore bulls.
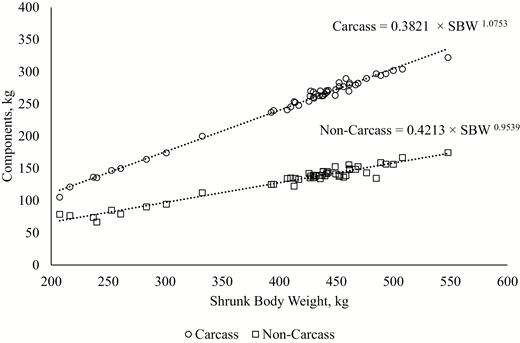
Relationship between the carcass and non-carcass components and shrunk body weight (SBW) of young Nellore bulls.
Energy Requirements
Energy requirements can be estimated by long-term feeding experiments, respirometric techniques, and comparative slaughter. This trial used the comparative slaughter method where we measured directly the MEI and RE, and HP was determined as the difference between the other variables. The NEm can be understood as total HP of the animal in a state of absolute fasting (Valadares Filho et al., 2016). For this experiment, the relationship between HP and MEI was described by the following equation: HP = 0.077 × e (3.7992 × MEI) (Figure 4) where NEm was estimated as 77 kcal/kg EBW0.75/d for young Nellore bulls. This estimated value was similar to the 74.9 kcal/kg EBW0.75/d proposed by Valadares Filho et al. (2016) for Nellore bulls. Maintenance energy expenditures vary with BW, breed or genotype, sex, age, season, temperature, physiological state, and previous nutrition (NASEM, 2016).
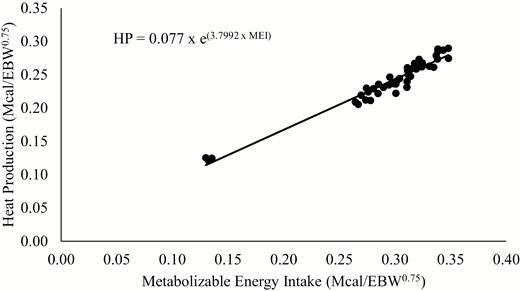
Relationship between heat production (HP) and metabolizable energy intake (MEI) of young Nellore bulls.
The use of the value found for NEm is limited and has no practical application in diet formulation because producing animals are not found in a fasting state. Therefore, the maintenance requirement was calculated in a more applicable form, as ME. The ME requirement for maintenance (MEm) was 122.75 kcal/EBW0.75/d, this value was considered the point where HP and MEI are equal and was obtained by applying an iterative process to the exponential model of HP as a function of the MEI. This value was superior to the 107 and 106.79 kcal/EBW0.75/d reported by Prados (2016) and Pacheco (2018) who also worked with growing Nellore bulls. A possible explanation for the high MEm value in this study is that the average final SBW of the animals (444.64 kg) was superior than the values reported by Prados (2016) and Pacheco (2018), 397.85 and 440.0, respectively. In addition, Prados (2016) worked with a 40:60 sugar-cane:concentrate ratio, and Pacheco with 28:72 corn silage:concentrate ratio, whereas in this study the forage:concentrate ratio was 50:50, thus, dietary composition and proportion of concentrate can contributed to the high MEm of the Nellore bulls in this study.
The efficiency of utilization of ME for maintenance was estimated from the NEm and MEm ratio, resulting in 62.7%. The efficiency can be affected by several factors, for instance sex, genetic group, age, environment, and the ME concentration in the diet (AFRC, 1993; NRC, 2000; CSIRO, 2007). In addition, there is strong evidence that the efficiency of utilization of ME for maintenance is also affected by characteristics linked to animal performance, such as rate of weight gain and feed intake (Williams and Jenkins, 2003; Marcondes et al., 2010).
The net energy requirement for gain is defined as all the energy that is retained in the EBW of the animals in the form of protein or fat (Garrett et al., 1959). Therefore, what determines the composition of the EBG is the weight relative to the weight at maturity of the animal (NASEM, 2016). In this experiment, the NEg (daily Mcal/EBW0.75) was estimated by the following equation: RE = 0.0535 × EBW0.75 × EBG0.7131, where RE = retained energy or net energy requirement for weight gain (Mcal/d), EBW = empty body weight (kg), EBG = empty body gain (kg/d).
The value of the efficiency of the use of ME for gain was obtained based on the linear regression between RE and MEI, and this value was 24.25% (Figure 5). The efficiency for gain depends on the proportions of energy retained in form of protein and fat (Costa e Silva et al., 2012). In this study, the proportion of energy retained as protein (REp) was 0.2265, and this value was calculated according to the following model proposed by Marcondes et al. (2013): REp = 1.140 × (RE/EBG)-1,137. According to the same author, the efficiency for gain and EBG were the most important variables that affected km.
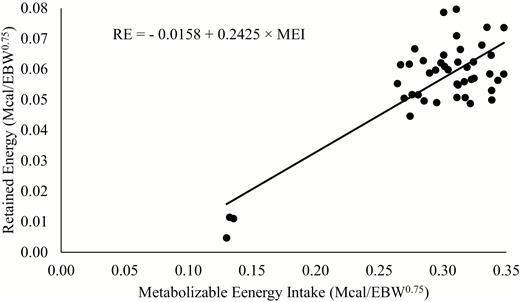
Relationship between retained energy (RE) and metabolizable energy intake (MEI) of young Nellore bulls.
Protein Requirements
Metabolizable protein for maintenance was 3.83 g/SBW0.75. This value was obtained based on a linear regression between metabolizable protein intake and EBG divided by the average metabolic EBW (Figure 6). This value is similar to the 3.6 and 3.8 g/SBW0.75 suggested by the BR-CORTE system (Valadares Filho et al., 2016) and NASEM (2016), respectively.
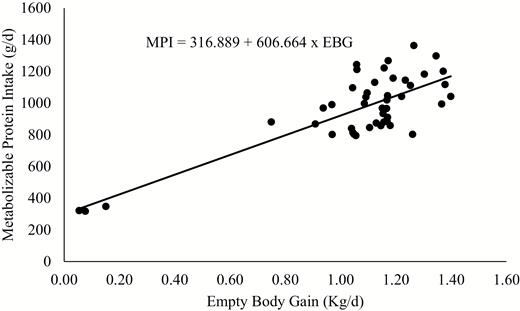
Relationship between metabolizable protein intake (MPI) and empty body gain (EBG) of young Nellore bulls.
Net protein requirement for growth (g/d) was estimated by the following equation: NPg = 227.372 × EBG – 19.479 × RE, whereas the model proposed by the BR-CORTE system (Valadares Filho et al., 2016) is NPg = 210.09 × EBG – 10.01 × RE. The NASEM (2016) adopted the following equation to estimate net protein requirement for growth: NPg = SWG × {268 – [29.4 × (RE/SWG)]}. Considering a 400 kg Nellore bull with SWG of 1 kg/d, EBG of 0.963 kg/d, and RE of 4.69 Mcal/d in a feedlot system, the net protein requirements for gain are 128, 155, and 130 g/d according to this study, Valadares Filho et al. (2016) and NASEM (2016), respectively. The protein required for animal growth depends on body composition (Boin, 1995), thus, protein requirements vary based on mature size, sex, and nutrition. The net protein requirements for gain are lower for bulls that are late maturing rather than early maturing because bulls deposit more lean tissue than steers (Vanderwert et al., 1985). The efficiency of the use of metabolizable protein for gain was 24.43%, which was obtained based on the linear regression between RP and MPI (Figure 7). Several factors such as age, body composition, and feeding condition can affect the efficiency of the use of protein for growth (Marcondes et al., 2013).

Relationship between retained protein (RP) and metabolizable protein intake (MPI) of young Nellore bulls.
There is a constant need for updating nutrient requirements aiming to reduce nutrient excretion, decrease production costs, and improve performance at the same time. So, improving the nutritional requirements of the Brazilian national herd is best accomplished by offering Brazilian producers technology that is generated under Brazilian conditions. In addition, this study provides useful information about nutritional requirements to help diet formulation in tropical countries, where predominantly Bos indicus cattle are used for meat production. The current data partially agree with previously published nutrient requirements; however, improvements in estimates of energy and protein requirements for Nellore bulls contained herein should be consider in production settings using similar animals and considered by the next committee assessing energy and protein requirements for Nellore bulls in Brazilian production scenarios.
ACKNOWLEDGMENTS
This study was made possible by grants from CNPq-INCT/Ciência Animal and FAPEMIG.
Conflict of interest statement. None declared.
LITERATURE CITED