-
PDF
- Split View
-
Views
-
Cite
Cite
Gustavo S de Miranda, Siddharth S Kulkarni, Jéssica Tagliatela, Caitlin M Baker, Alessandro P L Giupponi, Facundo M Labarque, Efrat Gavish-Regev, Michael G Rix, Leonardo S Carvalho, Lívia Maria Fusari, Mark S Harvey, Hannah M Wood, Prashant P Sharma, The Rediscovery of a Relict Unlocks the First Global Phylogeny of Whip Spiders (Amblypygi), Systematic Biology, Volume 73, Issue 3, May 2024, Pages 495–505, https://doi.org/10.1093/sysbio/syae021
- Share Icon Share
Abstract
Asymmetrical rates of cladogenesis and extinction abound in the tree of life, resulting in numerous minute clades that are dwarfed by larger sister groups. Such taxa are commonly regarded as phylogenetic relicts or “living fossils” when they exhibit an ancient first appearance in the fossil record and prolonged external morphological stasis, particularly in comparison to their more diversified sister groups. Due to their special status, various phylogenetic relicts tend to be well-studied and prioritized for conservation. A notable exception to this trend is found within Amblypygi (“whip spiders”), a visually striking order of functionally hexapodous arachnids that are notable for their antenniform first walking leg pair (the eponymous “whips”). Paleoamblypygi, the putative sister group to the remaining Amblypygi, is known from Late Carboniferous and Eocene deposits but is survived by a single living species, Paracharon caecusHansen (1921), that was last collected in 1899. Due to the absence of genomic sequence-grade tissue for this vital taxon, there is no global molecular phylogeny for Amblypygi to date, nor a fossil-calibrated estimation of divergences within the group. Here, we report a previously unknown species of Paleoamblypygi from a cave site in Colombia. Capitalizing upon this discovery, we generated the first molecular phylogeny of Amblypygi, integrating ultraconserved element sequencing with legacy Sanger datasets and including described extant genera. To quantify the impact of sampling Paleoamblypygi on divergence time estimation, we performed in silico experiments with pruning of Paracharon. We demonstrate that the omission of relicts has a significant impact on the accuracy of node dating approaches that outweighs the impact of excluding ingroup fossils, which bears upon the ancestral range reconstruction for the group. Our results underscore the imperative for biodiversity discovery efforts in elucidating the phylogenetic relationships of “dark taxa,” and especially phylogenetic relicts in tropical and subtropical habitats. The lack of reciprocal monophyly for Charontidae and Charinidae leads us to subsume them into one family, Charontidae, new synonymy.
A phylogenetic relict represents the remnant of a previously more diverse and more widespread fauna that has undergone faunal turnover and extinction events (Simpson 1955; Darlington 1957; Holmquist 1962; Gould et al. 1977). The evidence for this condition often consists of an ancient appearance of a lineage in the fossil record and a historically broad distribution of extinct taxa, with the retention of only a small number of geographically restricted extant species (Grandcolas et al. 2014). Typically, phylogenetic relicts represent an example of extreme phylogenetic attenuation, either as a result of extinction, low speciation rate, or a combination of these two processes. Relicts are often the sister taxa of diverse clades, which confers special status upon these small groups for evolutionary and genomic studies, as well as conservation priority. Renowned examples of phylogenetic relicts include coelocanths, lungfishes, tuatara, monotremes, nautiloids, horseshoe crabs, ginkgo, and Amborella trees (Grandcolas et al. 2014, 2016).
Recapitulating a historical fascination with the causal mechanisms that create relicts, modern investigations of phylogenetic relicts have focused on evidence of bradytelic evolution at the level of genes and genome architecture, as well as the signatures of extinction and faunal turnover (Soltis et al. 2008; Amemiya et al. 2010, 2013; Combosch et al. 2017; Meyer et al. 2021; Nong et al. 2021). Regardless of the cause of their taxonomic attenuation, the significance of phylogenetic relicts is two-fold. First, as the established sister groups of more diverse clades, such relicts play an outsized role in polarizing character states and reconstructing patterns of morphological evolution and genome organization. Second, as an extension of both topological placement and their fossil record, phylogenetic relicts play indispensable roles in molecular dating, specifically through the provision of calibration points for node dating. Lastly, relicts are critical in biogeographic analyses as they can have distinct ranges that differ from their sister-groups.
Given the attention and research focus that many phylogenetic relicts command, obtaining genomic and phylogenetic representation for such groups is often prioritized, even in cases where these taxa are geographically restricted (e.g., Amborella; tuataras; Cryptocercus cockroaches; Inward et al. 2007; Soltis et al. 2008; Gemell et al. 2020). One notable exception to this trend is the monotypic family Paracharontidae, the putative sister group to the remaining Amblypygi, commonly known as whip spiders (Weygoldt 1996; Garwood et al. 2017). The order Amblypygi is comprised of ca. 260 species, with a broad global distribution across tropical and subtropical habitats (Weygoldt, 1996; Harvey 2003; Miranda et al. 2022). Whip spiders are notable for the fearsome appearance of their hypertrophied raptorial pedipalps, as well as the modification of the first walking leg pair into antennal analogs—these arachnids are functionally hexapodous (Fig. 1). Amblypygi has also featured prominently in studies of arachnid behavior and communication, as they exhibit complex behaviors such as homing, learning, and aggregation as a function of kinship groups (Fowler-Finn and Hebets 2006; Wiegmann et al. 2016; Flanigan et al. 2021).
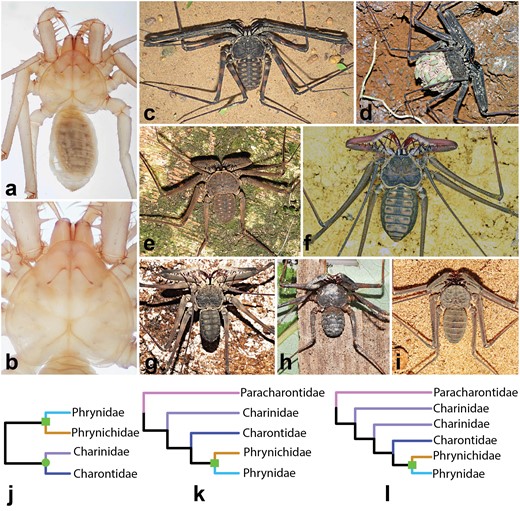
Exemplars of whip spider diversity and overview of morphology-based classification schema. a) Habitus of a preserved adult Paracharon sp. from Colombia. Note orthognathous articulation of the pedipalps. b) Magnification of the prosoma of Paracharon sp., highlighting the absence of eyes. c) Trichodamon princeps. d) Adult female of Damon medius, carrying juveniles on the dorsal opisthosoma. e) Paraphrynus aztecus. f) Heterophrynus batesii. g) Catageus sp. h) Sarax sp. i). Charinus cearensis j) Cladogram from Quintero (1986). Genera have been replaced with current familial assignments. k) Interfamilial relationships inferred by Weygoldt (1996). l) Interfamilial relationships inferred by Garwood et al. (2017). Square and circle represent the superfamilies Phryonidea and Charontoidea, respectively.
Whereas higher-order (and often, genome-scale) molecular phylogenies are now available for almost all extant chelicerate orders (Klompen et al. 2007; Giribet et al. 2014; Clouse et al. 2017; Fernández et al. 2017; Wheeler et al. 2017; Klimov et al. 2018; Benavides et al. 2019, 2021; Ballesteros et al. 2021; Santibáñez-López et al. 2022; Kulkarni et al. 2023a, b), a global molecular phylogeny for Amblypygi remains unavailable (Miranda et al. 2022). This gap is specifically attributable to the elusive nature of the putative sister group of the remaining extant whip spiders; the family Paracharontidae was known from a single species, Paracharon caecus, described one century ago from two localities in modern-day Guinea Bissau (Hansen 1921) based on materials discovered in the years 1899 and 1900. Morphological phylogenetic analyses have suggested that the oldest known whip spider fossils, from Carboniferous deposits in North America and the British Isles, constitute stem groups of Paracharontidae (Garwood et al. 2017). A more recent discovery of this lineage in Cambay Basin amber in India supports a historically broader distribution of Paracharontidae across the Paleotropics by the Eocene (Engel and Grimaldi 2014). Fossils of the remaining extant Amblypygi taxa (Euamblypygi) are comparatively recent, dating between the Cretaceous and the Miocene (Engel and Grimaldi 2014). This fossil record suggests that Paracharon is the result of broad extirpation across much of its former range because the Carboniferous, in tandem with a lower rate of cladogenesis than the remaining whip spiders.
Such patterns of early branching phylogenetic relicts are common within Chelicerata (Supplementary Table S1, available on Dryad at https://doi.org/10.5061/dryad.8sf7m0ctp). For examples, mesothele spiders (ca. 0.36% of described spider diversity) once had a broad geographic range including Western Europe in the Carboniferous, but are presently restricted to parts of east and southeast Asia (Selden 1996, Kallal et al., 2021). Cyphophthalmi, the cryptic sister group of the remaining Opiliones (ca. 3.1% of described harvestman diversity), exhibit distributions, internal relationships, and molecular divergence times that nearly mirror the sequence and timing of supercontinental fragmentation (Boyer et al. 2007; Giribet et al. 2012; Baker et al. 2020), which is also the case for the Palpimanoidea spiders (Wood and Wunderlich 2023), a group that was more abundant and widespread in the Mesozoic (Magalhaes et al. 2020). But in the case of Amblypygi, the absence of the “living fossil” Paracharon in any recent historical collections has effectively barred any higher-level molecular phylogenetic assessment of this group, as well as molecular dating that leverages the availability of fossil data. A handful of morphological cladistic analyses have tackled whip spider relationships, but these exhibit incongruence in higher-level nodes (Quintero 1986; Weygoldt 1996; Garwood et al. 2017; Fig. 1). This incongruence is partly attributable to the morphological conservatism of extant whip spiders, which incurs a paucity of discrete variable characters in the group.
Propitiously, we discovered an undescribed species of Paracharon from a collection at a cave in Colombia, consisting of 1 adult and 2 juvenile specimens. The rediscovery of this lineage after 123 years opens the door to the first higher-level molecular phylogeny of this poorly understood arachnid group. Here, we present a global molecular phylogeny of Amblypygi using ultraconserved element sequencing in tandem with Sanger datasets and sampling all extant genera. To quantify the impact of this relict lineage on evolutionary analyses, we performed multiple divergence time estimations with and without Paracharon sequence data, as well as with and without Amblypygi fossils. We show that the sampling of Paracharon plays a more significant role in the accuracy of divergence time estimation than the use of ingroup calibration fossils. Additionally, we perform an ancestral area reconstruction to understand distribution shifts over time when a relict is included. Our results underscore the significance of phylogenetic relicts in evolutionary analyses.
Materials and Methods
Species Sampling
Specimens sequenced for this study were hand-collected from field sites or contributed by museum collections (listed in Supplemental Material). We selected exemplars of each of the 5 described extant families, and all 17 described genera: 20 specimens of Charinidae (133 described species), 2 Charontidae (15 described species), 1 Paracharontidae (1 described species), 2 Phrynichidae (35 described species), and 10 Phrynidae (77 described species). Outgroup sampling leveraged previous phylogenomic works that have established Amblypygi as the sister group of vinegaroons (Thelyphonida), and short-tailed whip scorpions (Schizomida) (the clade Pedipalpi; Sharma et al. 2014; Ballesteros and Sharma 2019; Ballesteros et al. 2019). Pedipalpi in turn is understood to form a clade with spiders (Araneae), scorpions (Scorpiones), and pseudoscorpions (Pseudoscorpiones), a relationship supported by rare genomic changes (e.g., Ontano et al. 2021, 2022; Ballesteros et al. 2022). We therefore included 2 representatives of spiders, 1 vinegaroon, 3 short-tailed whip scorpions, and rooted the tree with 3 scorpions. Accession data for all specimens in this study are provided in Supplementary Table S2.
Ultraconserved Element Sequencing
Ultraconserved element (UCE) sequences generated in this study were augmented with published UCE and RNASeq datasets (Supplementary Table S3). We analyzed 47 terminals with UCE data including 39 Amblypygi. For newly sequenced specimens, one leg was used for DNA extractions from one specimen using the DNeasy™ Tissue Kit (Qiagen Inc., Valencia, CA). Libraries were prepared and enriched following protocols in Faircloth et al. (2015), but following the modifications detailed in the Supplementary Material. All pools were enriched with the Arachnida probe set (Starrett et al. 2017) except Heterophrynus and Charon, which were enriched using the Spider2Kv1 probe set (Kulkarni et al. 2020) following the myBaits protocol 4.01 (Arbor Biosciences).
Inclusion of Sanger-Sequenced Terminals
Although UCE datasets have demonstrated great potential for leveraging historical collections, the compatibility of these data with legacy datasets in Sanger-sequencing studies is often not evaluated. We integrated six standard Sanger-sequenced loci from historical datasets and through query raw files from the UCE dataset (see Supplemental Methods for details).
Phylogenomic Analyses
The assembly, alignment, trimming, and concatenation of data were done using the PHYLUCE pipeline (publicly available at https://phyluce.readthedocs.io/en/latest/). UCE contigs were extracted using the Spider2Kv1 probe set (Kulkarni et al. 2020) and the Blended probe set (Maddison et al. 2020). We applied gene occupancies of 10%, 25%, and 40% on the UCE dataset. Additionally, we also analyzed the 1% occupancy of the UCE dataset to allow the inclusion of all loci in the reconstruction of the phylogeny. We screened for orthologous and duplicate loci with minimum identity and coverage parameters of 65 and 65 matches. Protocols to reconcile the UCE dataset with RNASeq datasets are provided in the Supplemental Material.
Phylogenetic analyses were performed on two types of datasets: an unpartitioned UCE nucleotide dataset (at difference thresholds of occupancy); and a matrix of UCE paired with the partitioned six-locus Sanger matrix. Maximum likelihood analyses were performed using IQ-TREE (Nguyen et al. 2015) version 2. Model selection was allowed for each unpartitioned dataset using the TEST function (Hoang et al. 2018; Kalyaanamoorthy et al. 2017). Nodal support was estimated via 1000 ultrafast bootstrap (UFBoot) replicates (Hoang et al. 2018) and Shimodaira-Hasegawa approximate likelihood ratio testing (SH-aLRT) with 1000 iterations. We used the flag-bnni which reduces the risk of overestimating branch support with UFBoot due to model violations. We used gene (gCF) and site concordance factors (sCF) to evaluate the percentage of gene trees and decisive alignment sites containing a given branch in the maximum likelihood tree implemented in IQ-TREE (Minh et al. 2020).
To evaluate the topological robustness of selected nodes, we evaluated the signal for alternative placements using quartet likelihood mapping (Strimmer and Von Haeseler 1997) in IQ-TREE. Likelihood mapping was performed against the complete dataset, representing all UCE loci (i.e., not filtered for any occupancy threshold).
Phylogenomic Dating
Divergence time estimation was performed using a Bayesian inference approach, as implemented in codeml and MCMCTree (both part of PAML v.4.8; Yang 2007; dos Reis and Yang 2019). Separately, we inferred divergence times under LSD2, which uses a least-squares approach based on a Gaussian model and is robust to uncorrelated violations of the molecular clock (To et al. 2016). To investigate the influence of the relict Paracharon on divergence time estimation (i.e., to simulate the effect of not having discovered the Colombian specimens), we performed a separate family of analyses for both dating approaches (MCMCTree and LSD2), wherein we removed the terminal Paracharon sp.; the MRCA of the remaining Amblypygi (Euamblypygi) was therefore calibrated with the minimum age constraint reflecting the age of the Carboniferous fossil Weygoldtina anglica. Details on age priors and parameters are provided in the Supplementary Material.
We compared the median age and variance of nodes between runs to assess which parts of the chronogram were most prone to node age overestimation. In addition, to compare the significance of sampling Paracharon versus ingroup fossil calibrations, we performed a third family of analyses wherein we retained all terminals but removed the three ingroup fossil node calibrations and kept only the five calibration points for outgroups.
Ancestral Area Reconstruction
We reconstructed ancestral areas on internal nodes of the dated preferred tree, the combined tree with and without Paracharon using the package BioGeoBEARS (Matze, 2013) implemented in RASP 4.0 (Yu et al., 2020). The biogeographic areas and their justification are provided in the supplementary text and listed in Supplementary Table S5.
Results
Phylogenomics
We reconstructed an Amblypygi phylogeny using UCE datasets, as well as a combination of UCEs and six Sanger loci. Phylogenetic relationships obtained from UCE datasets with varying levels of missing data (10%, 25%, and 40% occupancy) across the two probe sets were grossly similar (Fig. 2; Supplementary Fig. S1). The 25% occupancy dataset targeted using the Blended probe set contained 415 loci and was therefore combined with the six-locus Sanger dataset for dating analyses. Six-locus alignments were combined with 44 out of 48 UCE alignments; we were unable to retrieve sequences for the remaining four taxa (Supplementary Table S4).
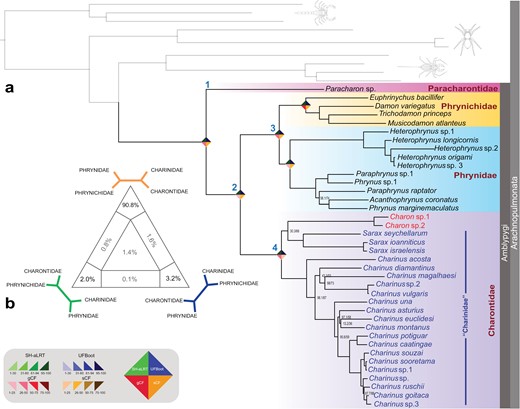
a) Phylogenomic relationships of Amblypygi based on 415 UCE loci (25% occupancy). Numbers on nodes correspond to SH-aLRT/ UFBoot, respectively; unmarked nodes are maximally supported. Numbers at nodes indicate higher-level taxa: 1, Paleoamblypygi; 2, Euamblypygi; 3, Phrynoidea; 4, Charontoidea. Rhombus icons on selected nodes reflect nodal support values, corresponding to the legend in (b). b) Four-cluster likelihood mapping assessing support for Charontoidea (Charontidae + Charinidae), based on the supermatrix of all UCE loci.
All datasets recovered Paracharontidae as the sister group of all other extant Amblypygi (Euamblypygi), as proposed by Weygoldt (1996) (Figs. 2 and 3). Together with some extinct taxa, Paracharontidae forms Paleoamblypygi (Weygoldt, 2000; Garwood et al., 2017), distinguished from the remaining whip spiders (i.e., Euamblypygi) by the dorso-ventral articulation of the pedipalps and the anterior projection of the carapace (Weygoldt, 1996; Garwood et al. 2017). Phrynichidae and Phrynidae (Phrynoidea) were recovered in a clade sister to Charinidae plus Charontidae (Charontoidea). Phrynoidea and Charontoidea are similar to the traditional groups Pulvillata and Apulvillata, respectively, as conceived by Quintero (1986). However, these names were abandoned by Weygoldt (1996, 2000) and Harvey (2003), so we follow Weygoldt (1996) and Harvey (2003) in naming Phrynichidae + Phrynidae as Phrynoidea, and Charontoidea for the remaining Euamblypygi.
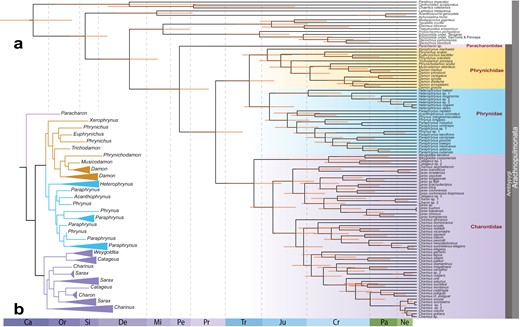
a) Divergence time estimation for Amblypygi. Data matrix is derived from a fusion of UCEs (40% occupancy) and 6 Sanger loci. b) Simplified maximum likelihood tree of the UCE and Sanger dataset, showing intergeneric relationships.
UCE matrices (Fig. 2; Supplementary Fig. S1) recovered the monophyly of Heterophryninae and Phryninae within the phrynids. Charon, the type genus of Charontidae, was nested within Charinidae (Fig. 2; Supplementary Fig. S1), regardless of occupancy and taxon composition, and contradicting recent morphological interpretations (Weygoldt 1996, 2000; Garwood et al. 2017). When support for (Charinidae + Charontidae) was assessed, quartet-based likelihood mapping strongly favored the placement of Charinidae and Charontidae as sister groups (90.8% of quartets; Fig. 2b).
Analyses of the 109-terminal UCE + Sanger loci dataset also recovered Paracharon sp. as the sister group to Euamblypygi (Fig. 3), a clade formed by Phrynoidea and Charontoidea. Derived relationships largely accorded with morphological analyses (Weygoldt, 1996; Miranda et al. 2018) (Fig. 3). Damon was recovered as polyphyletic due to the nested placement of Musicodamon and Phrynichodamon. Phrynidae was recovered in two main branches, Heterophryninae and Phryninae. Phrynus and Paraphrynus were also recovered as polyphyletic. Within Charontoidea, Charontidae (represented by three Charon and three Catageus; Miranda et al. 2018) was recovered as polyphyletic, and all four exemplars were nested within Charinidae with robust support. Two species identified as Catageus by Weygoldt (in Arabi et al. 2012) were recovered as the sister group to Weygoldtia and together formed the sister group to the remaining Charontoidea. The third species identified as Catageus by Weygoldt (in Arabi et al. 2012) was recovered in a clade with Charon that was nested within Sarax. The nonmonophyly of various genera supports the need for systematic revision of shallow-level taxa (e.g., Miranda et al. 2022). As a first step toward a simplified classification that is consistent with this phylogeny, we regard Charinidae as a junior synonym of Charontidae, new synonymy. The diagnosis of Charinida sensuQuintero (1986) is treated as the definition of the redefined Charontidae.
Molecular Dating and Ancestral Area Reconstruction
Divergence time estimation using five outgroup and three ingroup fossil calibrations in MCMCTree recovered a 330 Ma age for the crown group of Amblypygi (95% highest posterior density [HPD] interval: 374–295 Ma), with diversification of family-level taxa occurring in the late Permian and Triassic (Fig. 3). Removal of Paracharon resulted in node age overestimates for the deepest nodes in Ambypygi, but negligible effects on shallow nodes (Supplementary Fig. S2). The estimated age of Charontidae accorded closely with previous estimates based on two fossil calibrations and a reduced representation of Amblypygi higher-level diversity (Miranda et al. 2022).
By comparison to MCMCTree, divergence time estimation using LSD2 exhibited a more pronounced impact upon the removal of Paracharon. When Paracharon sp. was removed and a node calibration from the oldest known whip spider fossil was instead applied to the most recent common ancestor of Euamblypygi, the resulting analysis recovered a 314 Ma age for crown-group Euamblypygi (95% HPD interval: 353–314 Ma), and divergences of the family-level taxa in the Jurassic. All node ages were overestimated by LSD2 when Paracharon sp. was excluded, with the magnitude of overestimation correlating with phylogenetic depth (Supplementary Fig. S2). This result suggests that the sampling of Paracharontidae strongly influences divergence time estimation within Amblypygi, as a function of the estimation method.
To compare the relative influence of denser sampling of extant lineages versus the use of ingroup fossil calibrations, we performed a third analysis wherein we retained all terminals, but removed the three ingroup fossil calibrations. Divergence time analyses using only outgroup node calibrations resulted in broader variance (HPD intervals) for all node age estimates of interest, regardless of the use of MCMCTree or LSD2 (Supplementary Fig. S5), but median age estimates did not significantly change as a result of removing the ingroup fossil calibrations.
Historical biogeographic area optimizations were analyzed using RASP on our combined dataset which yielded DEC + j (AICc = 175.9, AICc weight = 0.89) as the best-fitting model. (Fig. 4; Supplementary Table S6). A combination of Oriental, African and Neotropical (OAN) regions was optimized as the most likely ancestral area of Amblypygi, as well as for Euamblypygi. Exclusion of the jump parameter rendered the inclusion of the Mediterranean region to the OAN regions as the ancestral area for Amblypygi (Supplementary Fig. S4). The most likely ancestral areas for individual families were as follows. Phrynichidae: Africa, Phrynidae: Neotropical, and Charontidae: Oriental region. The most likely ancestral area for Phrynoidea was Africa. The divergence of Paleoamblypygi (based on the Colombian Paracharon) and Euamblypygi from a widespread Amblypygi ancestor to the Neotropical region from the OAN region (however see Discussion and Supplementary Table S7). On coding the area of Paracharon as Neotropical + Africa, the latter based on an unsequenced record from Guinea-Bissau, the ancestral area for Amblypygi was recovered as a combination of Neotropical + Africa and Africa for Euamblypygi. Alternatively, the exclusion of Paracharon rendered a combination of Oriental, Africa, and Mediterranean as the Amblypygi ancestral area (Supplementary Fig. S6). Multiple dispersal events were inferred during the Cretaceous and early Paleogene periods, prominently between Africa and the Neotropical regions and out of the Oriental regions.
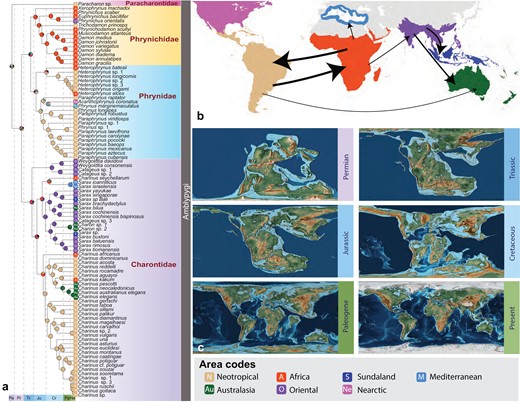
A. Biogeographic hypothesis obtained from the Dispersal-Extinction-Cladogenesis + jump parameter model of RASP analyses on the fossil-calibrated LSD2 analysis using the 25% occupancy dataset. B. A schematic representation of dispersal events optimized by the DEC + j model. The thickness of arrows corresponds to the frequency of events. C. Graphics for Pangean breakup from Scotese, C.R., 2016. PALEOMAP Project, https://www.earthbyte.org/paleomap-paleoatlas-for-gplates/. Ancestral areas optimized for each family and higher-level nodes are marked at the respective MRCA nodes. Pie charts at nodes indicate probability of individual or a combination of ancestral areas.
Discussion
Considerable strides have been achieved in deciphering the relationships of chelicerate arthropods in the past decade. An emphasis on denser taxonomic sampling, together with the availability of new sequencing technologies, has revolutionized modern investigations of relationships within poorly studied groups, such as mites, pseudoscorpions, and sea spiders (e.g., Klimov et al. 2018; Benavides et al. 2019; Ballesteros et al. 2021). Such datasets have facilitated greater precision in hypothesis-testing with regard to diversification dynamics, biogeographic history, and both morphological and molecular evolution (e.g., Giribet et al. 2012; Bond et al. 2014; Wheeler et al. 2017; Benavides et al. 2019; Santibáñez-López et al. 2022). Surprisingly, there remains a handful of higher-level taxa within arthropods for which molecular phylogenies still do not exist, as exemplified by Amblypygi. Here, our rediscovery of a long-lost relict breaks an erstwhile phylogenetic impasse, greatly expands the known range of the relictual lineage Paracharontidae, and significantly improves the precision of molecular dating efforts.
Our analyses of whip spider relationships recovered a well-resolved topology that placed Paracharontidae as the sister group of the remaining Amblypygi, consistent with the interpretations of Weygoldt (1996) and Garwood et al. (2017). Paracharontids closely resemble Carboniferous fossils of Amblypygi, such as Weygoldtina anglica (315 Ma). The articulation of the paracharontid pedipalp is parallel to the body axis, in contrast to the remaining Amblypygi. This feature is shared by the Paleozoic fossil whip spiders, as well as by nonwhip spider arachnopulmonates, such as Schizomida and spiders. All analyses additionally recovered the clades Phrynidae + Phrynichidae, as well as Charontidae (as redefined herein) with robust support. These results are partly congruent with each of the preceding morphological analyses of Amblypygi (Quintero 1986; Weygoldt 1996; Garwood et al. 2017).
The undescribed species of Paracharon that we identified from the Neotropics is morphologically similar to Paracharon caecus from Guinea Bissau, including in its extreme reduction of eyes and its troglobitic habitus, which likely reflects a case of convergence (Hansen 1921). Together with the Indian amber species Paracharonopsis cambayensis, as well as the Nearctic Carboniferous taxa (Weygoldtina; Graeophonus), these localities suggest a broad former distribution of Paleoamblypygi that underwent significant range contraction or extinction. Given the comparatively large size and visibility of whip spiders, we postulate that the remaining species of Paracharontidae may be restricted to caves and other microrefugia of Tropical Gondwana. Our biogeographic analysis is consistent with this inference, in that the ancestral area from the late Carboniferous period for Paracharontidae is reconstructed as Gondwanan. We interpret the Neotropical-Africa ancestral area around Permian the (alternate coding for Paracharon) to reflect regionalization within West Gondwana because these regions were separated only later, although when taking the Northern Hemisphere stem fossils into account, it is plausible that Paracharontidae might have been widespread. Although the exact mechanisms that create evolutionary relicts are multifactorial and complex, demonstrably broad historical distribution of Paleoamblypygi fossils in northern Europe and south Asia, support the characterization of Paracharon as a taxonomic and biogeographic relict. Extant records of Amblypygi from isolated regions such as Fiji and New Caledonia suggest that whip spiders may be capable of dispersal. Ree and Sanmartín (2018) cautioned that the jump parameter may inflate likelihood, optimize more jump dispersals, and mask signals of anagenetic events. Our exploration rendered fewer dispersals (more vicariance) with DEC models in comparison with the DEC + j models.
Beyond testing the validity of phylogenetic relationships inferred on the basis of morphology, there are two critical benefits to phylogenetic analyses that hinge upon sampling phylogenetic relicts with molecular data. First, we demonstrate that the omission of relicts has a significant impact on node dating approaches. When Paracharon sp. is artificially excluded from the dataset to simulate the effect of nonsampling (or assuming that the relict has gone extinct), the next available crown group node is treated as the most recent common ancestor of Amblypygi. The effect of this incorrect calibration is that the crown age of Amblypygi (= Euamblypygi, on exclusion of Paracharon) is overestimated, particularly by penalized likelihood approaches. This overestimation of node ages has downstream implications for any hypothesis-testing that relies upon divergence time estimation (e.g., historical biogeography; assessing diversification dynamics; Miranda et al. 2022). Second, phylogenetic relicts such as Paracharon have the effect of breaking branches that subtend crown group taxa (Inward et al. 2007; Soltis et al. 2008). The sampling of such basally branching groups is critical to phylogenetic accuracy when unstable relationships are driven by heterogeneous evolutionary rates across taxa (Ontano et al. 2021).
Our results therefore underscore the imperative for biodiversity discovery and continued campaigns to survey and document the diversity of tropical invertebrates, with the goal of finding taxa that represent phylogenetically and evolutionarily significant groups, including relicts (Harvey 1998; Griswold et al. 2012; Cruz-López et al. 2016; Aharon et al. 2019). Future efforts to advance invertebrate systematics must emphasize the completion of higher-level phylogenies for dark parts of the Tree of Life, in tandem with recognition of the scientific value of depauperate groups of phylogenetic significance.
Supplementary Material
Supplementary Material available from the Dryad Digital Repository: https://doi.org/10.5061/dryad.8sf7m0ctp.
Note Added in Proof
During the two-year review of this manuscript, which was previously made available as a preprint online, another group of researchers undertook the taxonomic description of the paracharontid rediscovered by us in this study and placed it in a new monotypic genus. To reflect the correct chronological order of this taxon's discovery, as well as our position on the practice of unnecessary taxonomic over-splitting, we maintain the use of "Paracharon sp." throughout this paper, consistent with the peer-reviewed draft.
Acknowledgements
This work is dedicated to the legacy of Peter Weygoldt. Access to natural history collections was provided by Jairo Moreno González, Lorenzo Prendini, Gonzalo Giribet, Lauren Esposito, Joachim Holstein, Adriano Kury, and Nikolaj Scharff, and access to recently collected material from Australia was provided by Kieran Aland. Siegfried Huber provided the photograph of Damon medius. Sample preparation for sequencing was performed at the Laboratory of Analytical Biology facility of the National Museum of Natural History (https://ror.org/05b8c0r92) and at the BioTechnology Center, University of Wisconsin- Madison. Scientific computation was performed on the Smithsonian High Performance Cluster (SI/ HPC), Smithsonian Institution (https://doi.org/10.25572/SIHPC), and High Performance Computing Cluster at The George Washington University.
Data Availability
All supplementary materials: data including sequence alignments, supplementary methods, tables, and figures can be downloaded from Dryad (https://doi.org/10.5061/dryad.8sf7m0ctp.)
Funding
G.S.M. was supported by a Buck Postdoctoral Fellowship and Global Genome Initiative Postdoctoral Fellowship from the National Museum of Natural History, Smithsonian Institution. S.S.K. and P.P.S. were supported by National Science Foundation IOS-2016141 to P.P.S. Sequencing was supported by the Global Genome Initiative grants (GGI-Peer-2018-179 and GGI-Rolling-2018-200 to G.S.M. and H.M.W.) from the National Museum of Natural History. J.T. was supported by grants from the Coordenação Aperfeiçoamento de Pessoal de Nível Superior (CAPES; 88882.426372/2019-01; 88887.631058/2021-00) at the “Programa de Pós-Graduação em Ecologia e Recursos Naturais,” Universidade Federal do São Carlos, and Programa de Pós-Graduação em Biologia Comparada, Universidade de São Paulo. Fieldwork in Israel and some UCE sequencing was additionally supported by Binational Science Foundation 2019216 to E.G.R. and P.P.S.
References
Author notes
Gustavo S. de Miranda and Siddharth S. Kulkarni contributed equally to this article.