-
PDF
- Split View
-
Views
-
Cite
Cite
Hannah M Wood, Jörg Wunderlich, Burma Terrane Amber Fauna Shows Connections to Gondwana and Transported Gondwanan Lineages to the Northern Hemisphere (Araneae: Palpimanoidea), Systematic Biology, Volume 72, Issue 6, November 2023, Pages 1233–1246, https://doi.org/10.1093/sysbio/syad047
- Share Icon Share
Abstract
Burmese amber is a significant source of fossils that documents the mid-Cretaceous biota. This deposit was formed around 99 Ma on the Burma Terrane, which broke away from Gondwana and later collided with Asia, although the timing is disputed. Palpimanoidea is a dispersal-limited group that was a dominant element of the Mesozoic spider fauna, and has an extensive fossil record, particularly from Burmese amber. Using morphological and molecular data, evolutionary relationships of living and fossil Palpimanoidea are examined. Divergence dating with fossils as terminal tips shows timing of diversification is contemporaneous with continental breakup.Ancestral range estimations show widespread ancestral ranges that divide into lineages that inherit different Pangean fragments, consistent with vicariance. Our results suggest that the Burmese amber fauna has ties to Gondwana due to a historical connection in the Early Cretaceous, and that the Burma Terrane facilitated biotic exchange by transporting lineages from Gondwana into the Holarctic in the Cretaceous.
Burmese amber, from the Hukawng Valley in Myanmar, dated 98.79 ± 0.62 Ma (Shi et al. 2012), is a significant source of plant and animal fossils that documents the mid-Cretaceous biome of southeastern Asia. With 1908 species described thus far (Ross 2021), the preserved biota shows evidence of a warm, tropical ecosystem (Xing et al. 2018), and contains both terrestrial and marine species, suggesting that the resin-producing trees were in close proximity to estuarine and freshwater habitats (Yu et al. 2019). The amber deposit is on the Burma Terrane (BT), or West Burma Block, a fragment that broke off from northeastern Australia and drifted northward. However, the timing of when the BT broke off is disputed, with suggestions that it occurred in the Devonian (Metcalfe 2017), preceding Pangean breakup, or the Late Jurassic (Heine and Müller 2005; Seton et al. 2012), but the preserved biota suggests it could not have occurred before the Early Cretaceous (Poinar 2018). It is also disputed when the BT collided with Asia, for example, some studies suggest collision was in the Late Cretaceous (Seton et al. 2012), and others suggest the Eocene or Oligocene (Westerweel et al. 2019), and see Morley et al (2021) that details inconsistencies within the geologic evidence. Either way, when the amber deposits were formed 99 Ma, the BT was likely an isolated island, possibly with an endemic biota due to being separated from Gondwana for large periods of time. Because of this history, the BT may have contributed to biological exchange by ferrying Gondwanan lineages to Asia.
Documentation of Burmese amber fossils has mostly supported that the BT has Gondwanan affinities (e.g., Zhang et al. 2018; Liu et al. 2020; Wriedt et al. 2021; Chitimia-Dobler et al. 2022; De Francesco Magnussen et al. 2022; Lepeco and Melo 2022), including evidence based on evolutionary relationships (Yamamoto et al. 2019), and often these studies call for further research to explain the puzzling appearance of Gondwanan fauna in Southeast Asia. There are many different scenarios that could explain this distribution, for example, a formerly widespread group may have undergone extensive extinction in the Northern Hemisphere, giving a false impression of a Gondwanan distribution, or alternatively, the Burmese amber fossils may reflect cosmopolitism if taxa from the Holarctic dispersed onto the BT when it was in close proximity to Asia, as suggested by Westerweel et al. (2019). To properly address these questions necessitates a dated phylogeny to examine whether divergence is consistent with the order and timing of continental breakup, which to date has not been done for many Burmese amber taxa.
One group particularly well-suited for studying patterns of continental drift are the Palpimanoidea spiders. Today, palpimanoids consist of 290 described species in 5 extant families (Archaeidae, Huttoniidae, Mecysmaucheniidae, Palpimanidae, and Stenochilidae), overall making an insignificant contribution to total spider species richness (over 50,000 spider species have been documented thus far; World_Spider_Catalog 2020). However, palpimanoids were seemingly at their zenith in the Mesozoic, when together with the Synspermiata, they were a dominant spider group until major faunal turnover in the Cenozoic (Magalhães et al. 2020). Using modern sequencing methods, palpimanoids consistently fall as the sister group to the Entelegynes (Fernández et al. 2018; Kulkarni et al. 2020; Kallal et al. 2021), which contains the bulk of modern spider species richness and contains the dominant Cenozoic groups (Magalhães et al. 2020). Using molecular clock methodology, studies have shown that palpimanoids likely emerged in the Permian or Triassic (Wood et al. 2013; Magalhães et al. 2020; Kallal et al. 2021). This body of research suggests palpimanoids existed prior to the breakup of Pangea—a necessary precondition to be a useful group for examining vicariance.
Palpimanoids have an extensive fossil record, arguably one of the best among spiders, occurring in 12 different deposits (Table 1), consisting of fossil members that are close relatives to the extant families, and with 5 extinct families that are known only from the fossil record (†Lagonomegopidae, †Micropalpimanidae, †Planarchaeidae, †Spatiatoridae, and †Vetiatoridae). The oldest known palpimanoid fossils are from the Early to Late Jurassic and are from Europe and Asia. A specimen from the Early Cretaceous of Brazil has been documented, and there are fossil specimens from Burmese, New Jersey, and Canadian ambers, all dated Late Cretaceous. In the Eocene, palpimanoids are documented from ambers of Europe and Cambay amber from India. The most recent fossils are from Dominican amber of Miocene age. To date, there are over 25 described species (Dunlop et al. 2023) from Burmese amber alone, most described by Wunderlich (e.g., 2004, 2015).
List of fossil deposits containing palpimanoids, and their location, age and time period
Deposit . | Location . | Age . | Citation for age . | Geologic period . |
---|---|---|---|---|
Burmese amber | Hukawng Valley, Myanmar | 98.79 ± 0.62 Ma (mean = 98.8) | Shi et al. (2012) | Late Cretaceous, Cenomanian |
Baltic amber | Baltic sea southern coastline | 43–48.5 Ma (mean = 45.8) | Ritzkowski et al. (1997) | Middle Eocene, Lutetian |
Bitterfeld amber | Bitterfeld, Germany | likely 47.8–38 Ma (mean = 42.9) | Wolfe et al. (2016); Dunlop et al. (2018) | Middle Eocene, Bartonian to Lutetian |
Cambay amber | Gujarat state, western India | 50–52 Ma (mean = 51) | Rust et al. (2010) | Early Eocene, Ypresian |
Crato Formation | Araripe Plateau, Brazil | 115–120 Ma (mean = 117.5) | Heimhofer and Hochuli (2010); Downen and Selden (2021) | Early Cretaceous, Late Aptian to Early Albian |
Dominican amber | Hispaniola island, Dominican Republic | 15–20 Ma (mean = 17.5) | Iturralde-Vinent and MacPhee (2019) | Early to Middle Miocene, Burdigalian to Langhian |
Grassy Lake amber | Grassy Lake, Alberta, Canada | 76.5–79.5 Ma (mean = 78) | Poinar et al. (2000) | Late Cretaceous, Campanian |
New Jersey amber | Atlantic coast of New Jersey, USA | 89.8–93.9 Ma (mean = 91.9) | Grimaldi et al. (1997) | Late Cretaceous, Turonian |
Jiulongshan Formation, rock deposit | Daohugou Village, Inner-Mongolia, China | 162–163 Ma (mean = 162.5) | Huang (2019) | Late Jurassic, Oxfordian |
Klein Lehmhagen clay pit | Grimmen, Germany | 182.7–174.1 Ma (mean = 178.4) | Selden and Dunlop (2014) | Early Jurassic, Toarcian |
Karabastau Formation sedimentary deposit | Karatau Mountains, Kazakhstan | 163.5–157.3 Ma (mean = 160.4) | Doludenko et al. (1990) | Late Jurassic, Oxfordian to Kimmeridgian |
Oise amber, Le Quesnoy | Oise River, Paris basin, France | 55–53 Ma (mean = 54) | Nel et al. (1999) | Early Eocene, Ypresian |
Deposit . | Location . | Age . | Citation for age . | Geologic period . |
---|---|---|---|---|
Burmese amber | Hukawng Valley, Myanmar | 98.79 ± 0.62 Ma (mean = 98.8) | Shi et al. (2012) | Late Cretaceous, Cenomanian |
Baltic amber | Baltic sea southern coastline | 43–48.5 Ma (mean = 45.8) | Ritzkowski et al. (1997) | Middle Eocene, Lutetian |
Bitterfeld amber | Bitterfeld, Germany | likely 47.8–38 Ma (mean = 42.9) | Wolfe et al. (2016); Dunlop et al. (2018) | Middle Eocene, Bartonian to Lutetian |
Cambay amber | Gujarat state, western India | 50–52 Ma (mean = 51) | Rust et al. (2010) | Early Eocene, Ypresian |
Crato Formation | Araripe Plateau, Brazil | 115–120 Ma (mean = 117.5) | Heimhofer and Hochuli (2010); Downen and Selden (2021) | Early Cretaceous, Late Aptian to Early Albian |
Dominican amber | Hispaniola island, Dominican Republic | 15–20 Ma (mean = 17.5) | Iturralde-Vinent and MacPhee (2019) | Early to Middle Miocene, Burdigalian to Langhian |
Grassy Lake amber | Grassy Lake, Alberta, Canada | 76.5–79.5 Ma (mean = 78) | Poinar et al. (2000) | Late Cretaceous, Campanian |
New Jersey amber | Atlantic coast of New Jersey, USA | 89.8–93.9 Ma (mean = 91.9) | Grimaldi et al. (1997) | Late Cretaceous, Turonian |
Jiulongshan Formation, rock deposit | Daohugou Village, Inner-Mongolia, China | 162–163 Ma (mean = 162.5) | Huang (2019) | Late Jurassic, Oxfordian |
Klein Lehmhagen clay pit | Grimmen, Germany | 182.7–174.1 Ma (mean = 178.4) | Selden and Dunlop (2014) | Early Jurassic, Toarcian |
Karabastau Formation sedimentary deposit | Karatau Mountains, Kazakhstan | 163.5–157.3 Ma (mean = 160.4) | Doludenko et al. (1990) | Late Jurassic, Oxfordian to Kimmeridgian |
Oise amber, Le Quesnoy | Oise River, Paris basin, France | 55–53 Ma (mean = 54) | Nel et al. (1999) | Early Eocene, Ypresian |
List of fossil deposits containing palpimanoids, and their location, age and time period
Deposit . | Location . | Age . | Citation for age . | Geologic period . |
---|---|---|---|---|
Burmese amber | Hukawng Valley, Myanmar | 98.79 ± 0.62 Ma (mean = 98.8) | Shi et al. (2012) | Late Cretaceous, Cenomanian |
Baltic amber | Baltic sea southern coastline | 43–48.5 Ma (mean = 45.8) | Ritzkowski et al. (1997) | Middle Eocene, Lutetian |
Bitterfeld amber | Bitterfeld, Germany | likely 47.8–38 Ma (mean = 42.9) | Wolfe et al. (2016); Dunlop et al. (2018) | Middle Eocene, Bartonian to Lutetian |
Cambay amber | Gujarat state, western India | 50–52 Ma (mean = 51) | Rust et al. (2010) | Early Eocene, Ypresian |
Crato Formation | Araripe Plateau, Brazil | 115–120 Ma (mean = 117.5) | Heimhofer and Hochuli (2010); Downen and Selden (2021) | Early Cretaceous, Late Aptian to Early Albian |
Dominican amber | Hispaniola island, Dominican Republic | 15–20 Ma (mean = 17.5) | Iturralde-Vinent and MacPhee (2019) | Early to Middle Miocene, Burdigalian to Langhian |
Grassy Lake amber | Grassy Lake, Alberta, Canada | 76.5–79.5 Ma (mean = 78) | Poinar et al. (2000) | Late Cretaceous, Campanian |
New Jersey amber | Atlantic coast of New Jersey, USA | 89.8–93.9 Ma (mean = 91.9) | Grimaldi et al. (1997) | Late Cretaceous, Turonian |
Jiulongshan Formation, rock deposit | Daohugou Village, Inner-Mongolia, China | 162–163 Ma (mean = 162.5) | Huang (2019) | Late Jurassic, Oxfordian |
Klein Lehmhagen clay pit | Grimmen, Germany | 182.7–174.1 Ma (mean = 178.4) | Selden and Dunlop (2014) | Early Jurassic, Toarcian |
Karabastau Formation sedimentary deposit | Karatau Mountains, Kazakhstan | 163.5–157.3 Ma (mean = 160.4) | Doludenko et al. (1990) | Late Jurassic, Oxfordian to Kimmeridgian |
Oise amber, Le Quesnoy | Oise River, Paris basin, France | 55–53 Ma (mean = 54) | Nel et al. (1999) | Early Eocene, Ypresian |
Deposit . | Location . | Age . | Citation for age . | Geologic period . |
---|---|---|---|---|
Burmese amber | Hukawng Valley, Myanmar | 98.79 ± 0.62 Ma (mean = 98.8) | Shi et al. (2012) | Late Cretaceous, Cenomanian |
Baltic amber | Baltic sea southern coastline | 43–48.5 Ma (mean = 45.8) | Ritzkowski et al. (1997) | Middle Eocene, Lutetian |
Bitterfeld amber | Bitterfeld, Germany | likely 47.8–38 Ma (mean = 42.9) | Wolfe et al. (2016); Dunlop et al. (2018) | Middle Eocene, Bartonian to Lutetian |
Cambay amber | Gujarat state, western India | 50–52 Ma (mean = 51) | Rust et al. (2010) | Early Eocene, Ypresian |
Crato Formation | Araripe Plateau, Brazil | 115–120 Ma (mean = 117.5) | Heimhofer and Hochuli (2010); Downen and Selden (2021) | Early Cretaceous, Late Aptian to Early Albian |
Dominican amber | Hispaniola island, Dominican Republic | 15–20 Ma (mean = 17.5) | Iturralde-Vinent and MacPhee (2019) | Early to Middle Miocene, Burdigalian to Langhian |
Grassy Lake amber | Grassy Lake, Alberta, Canada | 76.5–79.5 Ma (mean = 78) | Poinar et al. (2000) | Late Cretaceous, Campanian |
New Jersey amber | Atlantic coast of New Jersey, USA | 89.8–93.9 Ma (mean = 91.9) | Grimaldi et al. (1997) | Late Cretaceous, Turonian |
Jiulongshan Formation, rock deposit | Daohugou Village, Inner-Mongolia, China | 162–163 Ma (mean = 162.5) | Huang (2019) | Late Jurassic, Oxfordian |
Klein Lehmhagen clay pit | Grimmen, Germany | 182.7–174.1 Ma (mean = 178.4) | Selden and Dunlop (2014) | Early Jurassic, Toarcian |
Karabastau Formation sedimentary deposit | Karatau Mountains, Kazakhstan | 163.5–157.3 Ma (mean = 160.4) | Doludenko et al. (1990) | Late Jurassic, Oxfordian to Kimmeridgian |
Oise amber, Le Quesnoy | Oise River, Paris basin, France | 55–53 Ma (mean = 54) | Nel et al. (1999) | Early Eocene, Ypresian |
The distributions of the extant members suggest palpimanoids are a model system for biogeography studies. In general, the extant members have narrow distributions, with some families restricted entirely to former Gondwanan areas. Genera are typically restricted to one landmass and species are usually narrow endemics, often confined to a mountaintop (Rix and Harvey 2011, 2012; Wood et al. 2015). For example, extant Huttoniidae occurs solely on New Zealand, although there are Late Cretaceous, North American fossils. Extant Archaeidae consists of an Australian group, a South African group, and 2 monophyletic groups in Madagascar (Rix and Harvey 2012; Wood et al. 2015), but the archaeid fossil record spans the Jurassic to the Eocene from deposits in the Northern Hemisphere, including Burmese amber. Extant Mecysmaucheniidae consists of a New Zealand group, a southern South American group, and 1 monotypic genus from southern South America (Wood et al. 2016). Palpimanoids have never been observed ballooning—a mechanism for dispersal in some spiders where a line of silk is released that carries them in the wind for long distances, such as to oceanic islands. Even among Palpimanidae, the most widespread family, evidence suggests there is a monophyletic South American group that is sister to a group that mostly occurs in Africa, but also extends into parts of Asia (Wood et al. 2018; Ramírez et al. 2021).
This study makes use of the extensive fossil record in palpimanoids, together with representatives of the living groups, to test the following two hypotheses. Hypothesis 1: Burmese amber fauna has affinities to Gondwana due to previous connection in the Cretaceous. Evidence supporting this would be 1) Burmese amber taxa being phylogenetically more closely related to taxa from Gondwanan areas, and 2) divergence times mimic the order and timing of Pangean breakup, with the Northern Hemisphere taxa breaking off first and the BT taxa then breaking away from Gondwana in the Cretaceous. Hypothesis 2: The Burma Terrane transported lineages from Gondwana to the Northern Hemisphere. Evidence supporting this would be 1) a phylogenetic pattern of groups from the Northern Hemisphere nesting within the BT fauna; and 2) ancestral range reconstructions showing range expansion from the BT to the Northern Hemisphere.
With palpimanoid fossil distributions differing from their living counterparts, the inclusion of fossil taxa is critical for testing our biogeographic hypotheses. In the current study, the anatomical traits of over 20 extant species and over 60 fossil species, encompassing 95% of the known fossil record, were examined and a morphological data set was constructed. These data were combined with modern molecular sequences, available only for extant taxa, and were used for phylogenetic inference. The fossilized-birth-death model was used to estimate the timing of divergence, and using the resulting chronogram, we estimated palpimanoid ancestral ranges. Our conclusions provide evidence that the Burmese amber fauna has ties to Gondwanan and suggest that the BT served as a conduit, ferrying lineages to the Northern Hemisphere, similar to what has been suggested for the Indian Plate.
Materials and Methods
Taxon Sampling
This study includes 21 extant taxa, consisting of 16 palpimanoid genera, and 5 outgroups, sampled as follows for both molecular and morphological data. For Archaeidae, the endemic Australian group, the 2 endemic Madagascan genera, and the endemic southern African group are all represented (Zephyrarchaea, Madagascarchaea, Eriauchenius, and Afrarchaea). Huttoniidae, restricted to New Zealand, is represented (Huttonia). For Mecysmaucheniidae, the New Zealand group, the southern South American group, and the monotypic genus from southern South America are represented (Aotearoa, Zearchaea, Mecysmauchenius, and Mesarchaea). Stenochilidae consists of 2 genera that mainly occur in South and Southeast Asia and both representatives were included (Stenochilus from India, Colopea from Borneo). Palpimanidae is more widespread compared to other palpimanoids and the 3 subfamilies are represented: Palpimaninae, which mostly occurs in Africa, with a few species in the Mediterranean and Central Asia (Palpimanus from South Africa); Chediminae, which mostly occurs in Africa, including Madagascar, but also Central, Southeast, and South Asia, and the Seychelles (Scelidocteus from Uganda, Diaphoracellus from Madagascar); Otiothopinae, which occurs in South America, but extends into the Caribbean (Notiothops from Chile, Otiothops from northern South America). The 5 extant outgroups are: Dysdera (major clade: Synspermiata), Hickmania (Austrochiloidea), Stegodyphus (Entelegynae), Schizocosa and Zelotes (both Retrolateral-Tibial-Apophysis clade). Resulting phylogenies were rooted with Dysdera. See Supplementary Tables S1 and S2 for extant morphological and molecular voucher information and species identification.
For fossil taxa, over 100 fossil specimens preserved in amber were examined, consisting of 55 putative species, including specimens that are likely new species based on morphology. An additional 9 species were documented from compression fossils that were not physically examined but were included by examining published figures, resulting in a total of 65 putative fossil species. Juveniles were excluded from the study except in the case where a juvenile specimen represents a novel area or geologic era (e.g., Huttoniidae unnamed genus from Grassy Lake amber, Penney and Selden 2006; unnamed Myrmecarchaea species from Cambay amber, Wood et al. 2021), critical for understanding biogeography patterns. Other than †Lagonomegopidae, which is only represented by 1 taxon, this study includes nearly 95% of known Palpimanoidea fossils. Fossil vouchers used for morphological character acquisition are listed in Supplementary Table S3.
Morphological Character Acquisition
Wood et al. (2012) constructed a morphological matrix of 126 characters that focused on relationships within Archaeidae and placement of Archaeidae amongst spiders. For the current study this matrix was expanded to 275 characters that focus on traits of living and extinct Palpimanoidea, presented in Supplementary Appendix 1 (including data files, can be found in the Dryad repository: https://doi.org/10.5061/dryad.12jm63z11). For some extant taxa some characters were scored using published data and images (Forster and Platnick 1984; Griswold et al. 2005; Azevedo et al. 2022) or by using the image database from the “Assembling the Tree of Life: Phylogeny of Spiders” project (Creative Commons license Attribution-NonCommercial-ShareAlike 3.0 United States, CC BY-NC-SA 3.0 US) (see Supplementary Table S1). Morphological data were gathered for extant and fossil taxa using a Leica M205C microscope, and included dissecting internal female genitalia. When possible, 1 voucher of each sex was selected per taxon to score the characters. For fossil taxa, there were higher rates of missing data per specimen, and so, when available, additional conspecifics were used for scoring (see Supplementary Table S3).
Molecular Character Acquisition
For all extant taxa (except Stenochilus, which has no available published molecular data or recently collected tissue), raw molecular sequence data were downloaded from the Sequence Read Archives (SRA) using fastq-dump (see Supplementary Table S3 for SRA accession numbers). Data were genomic or transcriptomic and were assembled into contigs using Trinity (Grabherr et al. 2011). Following the PHYLUCE pipeline (Faircloth 2016), contigs were matched to the Arachnida probeset (Faircloth 2017) with minimum coverage and identity values set to 65. Loci obtained were aligned using MAFFT (min length = 20, no-trim) (Katoh and Standley 2013) and trimmed with GBLOCKS (b1 = 0.5, b2 = 0.5, b3 = 12, and b4 = 7) (Talavera and Castresana 2007). We selected 50% and 70% taxa per locus completeness for the final alignments.
Phylogenetic Analysis
Data were concatenated into 3 different matrices: 1) molecular-only, 2) morphology-only, and 3) combined, total evidence (TE). Phylogenetic analyses were carried out using Maximum Parsimony and Likelihood methods. Parsimony searches were performed in PAUP* v.4a168 (Swofford 2003) without partitioning data, without models of evolution, and with all characters treated as unordered, with equal weighting, and gaps coded as missing data. PAUP search strategy used the random stepwise addition option of the heuristic search for 1000 replicates with tree bisection-reconnection branch swapping, collapse of zero-length branches, and with retaining a maximum of 1000 trees per replicate. Branch support values were calculated by performing bootstrapping (Felsenstein 1985), using the random stepwise addition of the heuristic search for 500 replications, and for each replicate, with 10 random-addition sequences and retaining 1000 max trees.
Maximum likelihood analyses were implemented in IQTREE (Nguyen et al. 2015). For molecular data, the best-fit substitution model and data partitions were estimated using Partition Finder v.2.1.1 (Lanfear et al. 2016), with the rclusterf algorithm, corrected Akaike Information Criteria (AICc) model selection criterion, and “all” models of evolution, after implementing the Sliding-Window Site Characteristics method (Tagliacollo and Lanfear 2018). For morphological data, the standard discrete Markov model was used (Lewis 2001). Branch support was estimated by performing 5000 replications each of Shimodaira–Hasegawa-like approximate likelihood ratio test (SH-aLRT) (Guindon et al. 2010) and of ultrafast bootstrapping (UFBoot) (Hoang et al. 2018).
Divergence Time Estimation
Timing of divergence was estimated using tip-dating and the fossilized birth-death (FBD) model (Heath et al. 2014), implemented in BEAST v2.6.6 (Bouckaert et al. 2019). Both morphological and molecular characters were used in the analysis, using the smaller 70% occupancy molecular data set as it was less computationally challenging. Constant morphological characters (total = 7) were removed and the morphological data set was input by selecting to condition on recording variable characters. Seven undetermined specimens were removed because they may be conspecifics, but undescribed morphospecies were retained. BEAST partitions the morphological data set into groups based on the number of character states, and the Mk model (Lewis 2001) was used for these partitions, keeping the default model with no gamma variation. The molecular data was partitioned in the same way as the phylogenetic analyses, except each partition was set to Hasegawa–Kishono–Yano + gamma (with molecular partition 36 set to Jukes Cantor), since using more complicated models (e.g., General-Time-Reversible + gamma) resulted in analyses that did not converge. Partitions were unlinked for site models, but the overall clock and tree models were linked. The clock model was set to relaxed clock log normal. Fossils were treated as non-contemporaneous terminal tips and were dated as millions of years before the present based on the mean age of the deposit (see Table 1), and extant taxa had time set to zero. The diversification rate prior was set to exponential and the sampling proportion and turnover priors were set to uniform. The FBD tree prior was set to condition on rho sampling (rho = 16 extant palpimanoid species out of 290 known = 0.055) and to condition on the root. To speed up analysis the majority of nodes recovered in the TE Likelihood phylogenetic analysis, except for a few nodes with poor branch support, were constrained as monophyletic. The MCMC (Markov Chain Monte Carlo) chain was run 6 times for 60 million generations, sampling every 6000. Log files were combined and visualized in Tracer v.1.7.2 to confirm that the estimated sample size (ESS) reached 200 for all parameters and to visualize burn-in (Rambaut et al. 2018). A maximum clade credibility (MCC) tree of mean heights was constructed using the combined postburn-in trees.
Biogeography
This study focuses on Pangea breakup and biogeography areas consist of the different Pangean fragments, resulting in 8 areas: 1) the Neotropics (N); 2) the BT, which contains the Cretaceous Burmese amber deposit (B); 3) Madagascar (M); 4) Australia (A); 5) India (I); 6) Africa (F); 7) New Zealand (Z); and 8) the Holarctic (H). The Palearctic and Nearctic were combined into the Holarctic, an area that includes fossil specimens from the Jurassic of inner-Mongolia, from Eocene Baltic, Bitterfeld and Cambay ambers, from Cretaceous New Jersey and Canadian ambers, and 1 extant taxon, Colopea sp. that was collected from Borneo. Borneo was treated as Holarctic because it connected to northern Pangea in the Late Triassic (from fragments that rifted from southern Pangea in the Carboniferous), before Pangea had begun to break apart (Seton et al. 2012), and has maintained connections to mainland Asia until recently depending on sea levels. Taxa were coded based on the locality of the species and all taxa occupied only 1 area.
A time-stratified analysis was performed based on the following events, following Seton et al. (2012, and references therein), except when noted otherwise. Time slice 250–175 Ma: Pangea is intact. Time slice 175–156 Ma: Gondwana starts to break away from Laurasia around 180 Ma, getting more rapid around 170 Ma. Time slice 156–145 Ma: the BT breaks away from Australia around 156 Ma. Time slice 145–135 Ma: Eastern and Western Gondwana start to separate around 160 Ma, but is complete by 145 Ma, although still connected via Antarctica. Time slice 135–110 Ma: India and Madagascar together separate from East Gondwana (Australia) starting at 135 Ma. Time slice 110–87 Ma: By 110 Ma South America and Africa are separated. Time slice 87–80 Ma: India breaks away from Madagascar around 87 Ma, and Zealandia (New Zealand) breaks away from Australia around 85 Ma (Li and Powell 2001). Time slice 80–55 Ma: The BT attaches to Asia around 80 Ma. Time slice 55–35 Ma: India attaches to Asia around 55 Ma. Time slice 35–15 Ma: Australia breaks away from Antarctica (and therefore connection with South America) at 35 Ma (Li and Powell 2001). Time slice 15–0 Ma: Africa attaches to the Holarctic around 15 Ma. For each time slice dispersal multipliers were set as follows. Adjacent landmasses were set to 1.0, allowing dispersal between connected areas. Areas connected via another landmass (e.g., 30 Ma Australia and South America were connected through Antarctica) were set to 0.5, making dispersal to these areas relatively easy but less likely than for adjacent areas. Areas separated by large distances of water were set to 0.000001, which still allows for dispersal events but makes them unlikely. This low value, which biases our study against dispersal, was selected based on our understanding that Palpimanoidea has very limited dispersal capabilities. An additional simplified analysis was run consisting of only 3 areas (Southern Hemisphere [S]; Northern Hemisphere [N]; and the BT [B]) and the time slices were reduced based on events between these areas.
Ancestral areas were estimated using the R package BioGeoBEARS (Matzke 2018) implementing the Dispersal Extinction Cladogenesis (DEC) continuous-time model (Ree and Smith 2008). The DEC model performs maximum likelihood estimation of ancestral ranges at speciation events, with dispersal (range expansion) and local extinction (contraction) as estimated parameters. The jump dispersal parameter was not included in these analyses because the assumption of instantaneous speciation during dispersal is considered unrealistic and not central to our hypothesis of ancient vicariance (Ree and Sanmartín 2018). The analysis was performed using the fully resolved MCC tree with outgroups pruned. The maximum number of areas was set to 5, which allowed the analysis to run reasonably quickly while still able to model widespread ancestors.
Results
Phylogenetic Analysis
Seven of the 275 morphological characters were constant, 22 were variable but parsimony-uninformative, and 246 were parsimony-informative. For the molecular data, the number of loci recovered per extant taxa ranged from 82 to 682 loci, with an average of 309. The aligned, concatenated 50% and 70% occupancy molecular data sets were 41,490 base pairs (bp) and 13,061 bp, respectively.
We report only results from the TE Likelihood analysis that combined morphology with the 70% occupancy molecular data, but see Supplementary Appendix 2 and Supplementary Figs. S1–S10 for all additional results. Our preference is for the Likelihood TE 70% results for the following reasons: 1) the Parsimony TE results overall had less resolution than the Likelihood, probably because the Likelihood analyses used models of molecular and morphological evolution; 2) the 70% molecular data is reduced in bp size compared to the 50% data set and so, is less likely to overwhelm the signal from the morphological data; and 3) the TE results with the 70% sequence occupancy data set, compared to the 50%, are more in agreement with results from other independent, yet more comprehensive studies that more thoroughly sample across spiders and that support a monophyletic Palpimanoidea (e.g., Kallal et al. 2021).
Major findings are that Palpimanoidea was recovered as monophyletic (84% SH-alrt and 97% UFBoot), although with the exclusion of †Lagonomegopidae Lineaburmops, which grouped with Schizocosa and Zelotes (97% SH-alrt and 100% UFBoot). For the remainder of the manuscript, when we refer to Palpimanoidea this is done with the exclusion of †Lagonomegopidae. Within Palpimanoidea, the earliest diverging branch is a group consisting of the oldest fossils from the Jurassic: †Seppo, †Onychopalpus, and †Sinaranea (73% SH-alrt and 88% UFBoot), the first from Germany (Selden and Dunlop, 2014), and the latter 2 from Inner Mongolia (Selden et al. 2008, 2020). Palpimanidae plus †Cretapalpus, all southern, was monophyletic (100% SH-alrt and UFBoot) and diverged next. Then, a monophyletic group was recovered (100% SH-alrt and UFBoot) that consisted of Huttoniidae (diverging first), followed by †Spatiatoridae (paraphyletic), †Micropalpimanidae, and then †Vetiatoridae, known only from Burmese amber, with Stenochilidae, known from the Indomalayan region, nested within †Vetiatoridae. Archaeidae and Mecysmaucheniidae and their numerous fossil relatives, including †Planarchaeidae, formed another monophyletic group (99% SH-alrt and 96% UFBoot), termed here the “high-carapace clade.” Archaeidae was polyphyletic with a number of fossil species falling as close relatives to Mecysmaucheniidae, and †Planarchaeidae and †Pretervetiator also fall as close relatives to Mecysmaucheniidae. Within the true Archaeidae (i.e., excluding those fossils that are closer to Mecysmaucheniidae), the extant genera, all from the Southern Hemisphere, form a clade with the Burmese amber archaeids (with 1 line from Baltic amber nested within), and †Saxonarchaea, from Baltic amber (97% SH-alrt and UFBoot). This mainly southern group is sister to a monophyletic group of Northern Hemisphere fossils (87% SH-alrt and 98% UFBoot). The extant Mecysmaucheniidae from New Zealand and South America are monophyletic (99% SH-alrt and 100% UFBoot), and their closest relative was †Pretervetiator from Burmese amber (72% SH-alrt and 94% UFBoot), and together they were sister to a large group from Burmese amber (91% SH-alrt and 95% UFBoot).
Divergence Time Estimation
The resulting chronogram and associated 95% highest posterior density (HPD) are shown in Fig. 1. Palpimanoidea (excluding †Lagonomegopidae) diverged from its sister clade in the Triassic (95% HPD = 256–204 Ma, mean = 229 Ma), and divergence within Palpimanoidea into the main groups (labeled 1–4 on Fig. 1) occurred in the Late Triassic to Early Jurassic, prior to continental breakup. Diversification within each of these 4 groups was contemporaneous with Pangean breakup, some examples follow. For both Archaeidae and Mecysmaucheniidae and their fossil relatives (group 3 and 4, Fig. 1), divergence between the most basal lineages from the Northern Hemisphere with the taxa from the Southern Hemisphere and Burmese amber spans the Jurassic, when Pangea split into Laurasia and Gondwana (Seton et al. 2012). For both groups, the Burmese amber taxa diverged from its closest Southern Hemisphere relative in the Late Jurassic/Early Cretaceous, congruent with when the BT broke away from northwestern Australia following Seton et al. (2012). There are 3 instances of Northern Hemisphere taxa nested within a monophyletic group from Burmese amber, with divergence of the northern taxa occurring around 110 Ma, but with the 95% HPDs spanning the Cretaceous (see asterisks in Figs. 1 and 2).
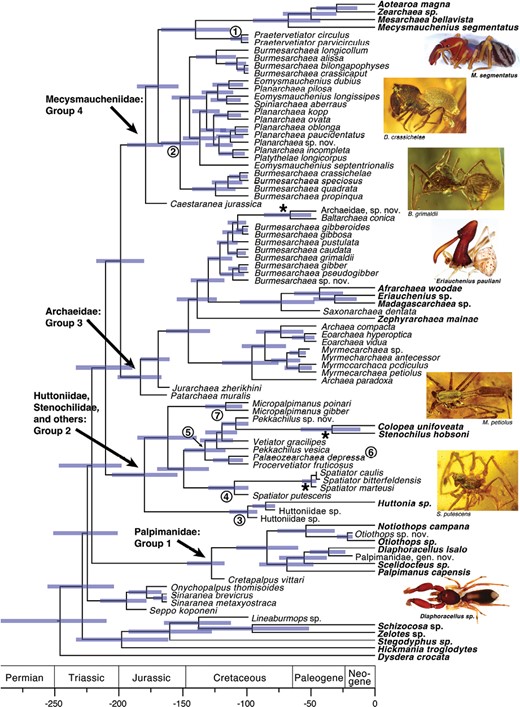
Dated phylogeny with branch lengths drawn to reflect divergence time estimations. Timing of divergence was estimated using Bayesian methods on the TE data set (morphology + 70% occupancy sequences), with topology constrained based on the TE Likelihood phylogeny. Error bars reflect the 95% HPD. Extant taxa are shown in bold. Asterisks (*) show Northern Hemisphere taxa that nest within a group mainly from the BT. Circled numbers show lineages that are currently considered: 1) Vetiatoridae; 2) Archaeidae and Planarchaeidae; 3) Huttoniidae; 4) Spatiatoridae; 5) Vetiatoridae with Stenochilidae nested within; 6) P. depressa is currently considered Mecysmaucheniidae; and 7) Micropalpimanidae. Scale = millions of years before present.
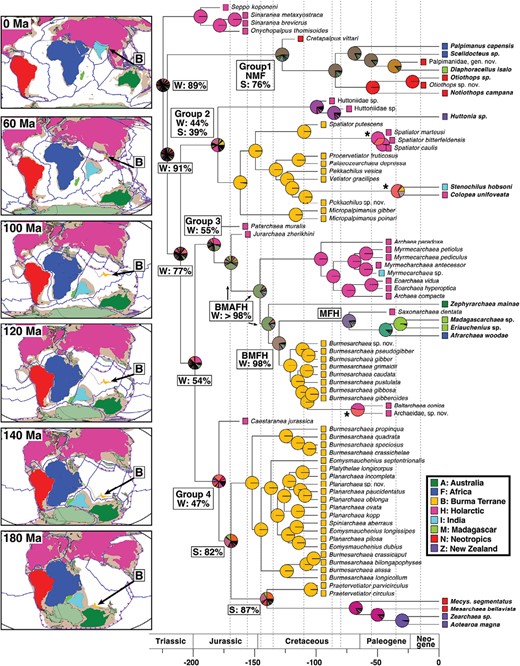
Ancestral area estimations from the DEC-model biogeography analysis. Extant taxa are shown in bold. Colored squares at terminals represent the distribution of terminal taxa. Pie charts at nodes represent the relative likelihood (RL) of the estimated ancestral range. Deeper nodes also show the summed RL for being widespread (W, occurring in the Holarctic and at least 2 other Gondwanan areas), or southern (S, occurring in at least 2 Gondwanan areas). Dashed lines mark the different time slices. Asterisks (*) show range expansion or dispersal from the BT into the Holarctic. Panels (modified from Matthews et al. 2016) show the area position through time and the present day coastlines; areas are colored according to the legend, Antarctica is light green, and the approximate extent of the continents in brown; arrow showing the BT. Scale = millions of years before present.
Biogeography
The DEC model 8-area analysis is shown in Fig. 2, Supplementary Figs. S11–S12, and the 3-area analysis produced similar results (Supplementary Figs. S13–S14). The deepest nodes of Palpimanoidea have many estimated ranges that are nearly as likely, and the relative likelihoods (RL) were summed to examine general patterns. The estimated ranges for the first 4 nodes, when summed, are consistent with a widespread distribution across Pangea (summed RL of estimated ranges that contain the Holarctic and at least 2 other Gondwanan areas = 89%, 91%, 77%, and 54%; for the 3-area analysis 71%, 54%, 40%, and 27% for ranges that contain both the northern and southern areas).
Archaeidae and its fossil relatives (group 3 in Fig. 2) are estimated to have the most likely ancestral range in northern Pangea in the Early Jurassic (RL = 29%), but the summed RL shows a widespread ancestor, occurring in the Holarctic and at least 2 other Gondwanan areas (summed RL = 55%). In the Late Jurassic, the most likely ancestral range is widespread across Pangea, with daughter lineages then spliting off (summed RL for the 2nd–5th placed nodes = 98–100% widespread, containing the Holarctic and at least 2 other Gondwanan areas). The Mecysmaucheniidae and its fossil relatives (group 4 in Fig. 2) have an estimated ancestral range in the Holarctic and the BT (RL = 24%) in the Early Jurassic, but when summed, have an RL of 47% for a widespread ancestor. The next 2 nodes have a summed RL of 82% and 87% that the ancestor occupies southern Pangea (occurring in 2 or more Gondwanan areas), with daughter lineages then splitting off.
The ancestral node of group 2 (Fig. 2), consisting of Huttoniidae, †Micropalpimanidae, †Spatiatoridae, Stenochilidae, and †Vetiatoridae, has the most likely estimation (RL = 19%) of occurring in the BT, Holarctic and New Zealand, but when summed, 44% of estimations have widespread ranges in the Holarctic and at least 2 other southern areas, and 39% of estimations show an ancestral range of at least 2 southern areas. The simplified 3-area analysis has 56% of estimates with a widespread ancestor across Pangea.
The most ancestral node of Palpimanidae (group 1 in Fig. 2) has a distribution in southern Pangea (RL = 55%), and when summed, 76% of all estimations contain 2 or more southern areas. There are 3 events of dispersal or range expansion out of the BT and into the Holarctic (see asterisks in Fig. 2).
Discussion
Spiders are ancient, with an estimated age of around 400 Ma (Magalhães et al. 2020), creating challenges in recovering deep phylogenetic relationships. Palpimanoidea is also an ancient group considering the oldest fossils are from the Jurassic (Selden et al. 2008, 2020; Selden and Dunlop 2014). The value of incorporating fossils in this situation, which necessitates the use of morphological data, is that fossils often have intermediate or transitional traits that can inform phylogenetic reconstruction. Our study shows the clear need for taxonomic transfer (see circled numbers in Fig. 1): e.g., Archaeidae is polyphyletic and †Vetiatoridae is paraphyletic.
Incorporating fossils into phylogenetic studies is also valuable because fossils can serve as non-contemporary terminal tips for estimating timing of divergence. It is critical to include fossil taxa in biogeography studies when fossil distributions are different from those of the contemporary taxa, as is the case for Palpimanoidea. This provides a more accurate picture of how distributions have changed over large time scales. Doing so in the current study provides evidence that is consistent with Hypothesis 1, that the BT fauna shows Cretaceous connections to Gondwana. Further, our results are consistent with Hypothesis 2, that the BT acted as a “biotic ferry” that transported lineages from Gondwana to the Northern Hemisphere. This evidence, discussed in detail below, is preserved in the fossil record even as Cenozoic extinction must have occurred in many parts of the world.
Origin and Early Divergence of Palpimanoidea Prior to Pangean Rifting
Our study dates Palpimanoidea as diverging in the Triassic. This result is corroborated by other studies that independently examined the fossil record and provided evidence that Palpimanoidea was dominant during the Mesozoic (Magalhães et al. 2020), or that used molecular clock dating to show divergence from other spiders by the end of the Triassic (Wood et al. 2013; Dimitrov et al. 2017; Fernández et al. 2018; Magalhães et al. 2020). The estimated ancestral range of Palpimanoidea was likely widespread across Pangea.
Regarding the limits of Palpimanoidea, in this study, †Lagonomegopidae falls within the Entelegynae (spiders with flow-through female genitalia), sister to members of the RTA-clade (bs = 95%, Supplementary Fig. S10). The RTA-clade is a diverse, specious group, named forthe retrolateral tibial apophysis that occurs on the male pedipalp, and is noticeably absent from the Mesozoic fossil record even though divergence dating analyses suggest this clade should be present by 100 Ma (Magalhães et al. 2020). †Lagonomegopidae is distinctive because of their unusual eye pattern, and they have a diverse fossil record from the Cretaceous, with over 30 species in 20 genera, mainly from Burmese amber but also from other Northern Hemisphere deposits (Guo et al. 2021; Dunlop et al. 2023). The placement of †Lagonomegopidae within Palpimanoidea has been controversial, and phylogenetic results of Guo et al (2021) recovered this family as sister to the extant members of Palpimanoidea. These spiders have been described as having haplogyne female genitalia (simple genitalia), which externally looks very similar to the juvenile condition. It is questionable whether lagonomegopids are truly haplogyne, or whether juveniles are being mistaken for females, and only examination of internal genitalia, or clear copulatory duct openings on the external genitalia, can confirm the genitalic condition in these spiders. It should also be noted that the male copulatory organs do not look stereotypically haplogyne. Complicating this is that the entelegyne condition (flow-through female genitalia) has evolved convergently (Michalik et al. 2019), there are documented instances of reversal back to the haplogyne condition (Cabra‐García et al. 2014), and homology of female genitalic structures is more complicated than previously thought (Zhan et al. 2019). In †Lagonomegopidae we scored this trait as unknown. The trichobothria on the leg tarsi is suggestive †Lagonomegopidae may be an early lineage of the RTA-clade, and further, some genera possess a retrolateral-tibial-apophysis. The results of Guo et al (2021) support convergent evolution for these traits, but based on our results, these traits support membership in the RTA-clade. However, caution should be used because our study included only 1 †Lagonomegopidae representative from this diverse group and did not include an adequate sample of RTA-clade or Araneomorphae members. To properly address phylogenetic placement future work should include greater taxonomic sampling and an expanded character matrix.
Within Palpimanoidea, divergence into the major groups (groups 1–4 on Fig. 1) preceded Pangean breakup. The earliest diverging lineages are some of the oldest palpimanoid fossils known, †Seppo, †Onychopalpus, and †Sinaranea, all of Jurassic age from the Northern Hemisphere. Our results are similar to Selden et al (2020), with †Sinaranea and †Onychopalpus falling as early-diverging palpimanoids (†Seppo was not included in their study). Caution should be used regarding the phylogenetic placement of these 3 genera, particularly for †Seppo, due to high amounts of missing data and lower branch support values (Supplementary Fig. S10). In general, fossils have more missing data compared to extant taxa, but these earliest fossils are not preserved in amber (e.g., †Seppo: mold of calcium carbonate) and have even less data compared to amber-preserved fossils.
It is challenging to determine ancient distributions based on fossil distributions alone because the fossil record is uneven and incomplete. This is the case for spiders because there are not equivalent fossil deposits known from the Jurassic in the Southern Hemisphere compared to the Northern Hemisphere (see Penney and Selden 2011, for a list of amber deposits containing spider specimens). This is also true for Palpimanoidea, which has a more extensive fossil record from the Northern Hemisphere (see Table 1), going back to the Jurassic, compared to the Southern Hemisphere, where the only known fossil is a single specimen from the Crato formation of Brazil, dated 110 Ma. But with a dated phylogeny that includes fossils we can estimate ancestral ranges, which show a widespread distribution across Pangea. Thus, results here suggest it is unlikely that palpimanoids had a distribution limited to just northern Pangea in the Triassic/Early Jurassic based on the fossil record, but rather, it may be that we simply do not have a record of their existence in southern Pangea.
Regarding relationships among the major Palpimanoidea groups, our TE phylogenetic results consistently recover a “high-carapace group” that includes Archaeidae and Mecysmaucheniidae and their fossil relatives (bs = 99%, Supplementary Fig. S10). There currently is not consensus on extant family relationships within Palpimanoidea, and topology can change depending on the type of data (Wood et al. 2012). Using molecular data alone some, but not all, studies have recovered the “high-carapace group” (Kulkarni et al. 2020; Kallal et al. 2021; Ramírez et al. 2021). There are also some molecular studies that corroborate that Palpimanidae was the first to diverge among the extant palpimanoids (Fernández et al. 2018; Wood et al. 2018; Kulkarni et al. 2021).
Vicariance and BT Rifting in the Late Jurassic to Early Cretaceous
Prior to Pangea breaking apart, the 4 main Palpimanoidea groups were widespread, either across Pangea or southern Pangea, and were poised on the cusp of major geologic change. Spanning the Jurassic through the Cretaceous, the main groups are well supported (Supplementary Fig. S10) and have widespread ancestral ranges that divide into lineages that inherit different Pangean fragments, consistent with vicariance. Ideally, to address whether the Burmese amber fauna has affinities to Gondwana one would study a taxonomic group that was widespread prior to Pangea breakup, with members in Burmese amber and in both the Northern and Southern Hemispheres, criteria met by Archaeidae and Mecysmaucheniidae and their fossil relatives (group 3 and 4).
Archaeidae shows phylogenetic patterns that are consistent with the order and timing of continental breakup, for example, showing a “hierarchical pattern reflecting the geological area cladogram” (Sanmartin and Ronquist 2004). Archaeidae (group 3, bs = 87%) in the Early Jurassic occupies northern Pangea, but by the Middle Jurassic is widespread across Pangea. Then, a distinct Northern Hemisphere group (bs = 87%) is the first to diverge, similar to the events of continental breakup following Seton et al. (2012) where Pangea first breaks into Laurasia and Gondwana. This distinct Northern Hemisphere group consists of †Archaea, †Eoarchaea, and †Myrmecarchaea (bs = 87%), and shares similarities in the male copulatory organs (Wood et al. 2021) as well as other traits. The specimen from Indian Cambay amber (†Myrmecarchaea sp., BSIP-41985) nests within this group, suggesting dispersal from the Holarctic onto the Indian continent after India collided with Asia (Fig. 2).
Then, the remaining Archaeidae consists of the Burmese amber taxa and the extant taxa that occur in former Gondwanan areas (bs = 97%). Following Seton et al. (2012), after Laurasia and Gondwana are separate, then the BT breaks off from Australia just prior to separation of eastern and western Gondwana. In Archaeidae, the Australian clade breaks off first and the Burmese taxa form a group with the African/Madagascan group (bs = 88%). Our results also suggest that there was a dispersal to the Northern Hemisphere from the African/Madagascan group (†Saxonarchaea). The Northern Hemisphere group was present in the Eocene and is closer in geologic time to the extant archaeids that only occur in Australia, Madagascar, and South Africa, but nevertheless the Burmese amber group of Cretaceous age is phylogenetically more closely related to these southern extant lineages. This supports the view that archaeids are highly dispersal limited, as 1 would expect that since the Eocene there would be opportunity for dispersal to/from the Southern Hemisphere, so that phylogenetic relationships might be based more on proximity in geologic time rather than place. Instead, we observe patterns congruent with the timing and order of continental breakup.
Mecysmaucheniidae and its fossil relatives (group 4, bs = 99%) occurs in the Northern and Southern Hemispheres and in Burmese amber, and the most likely estimated ancestral range around 170 Ma is the BT and the Holarctic (BH = 47%, Fig. 2), suggestive of a widespread distribution. The Burmese taxa have closer evolutionary relationships with the Gondwanan taxa (bs = 91%), with the 1 Northern Hemsiphere taxon, †Castaranea, falling outside, mimicking patterns of continental breakup as in Archaeidae. †Caestaranea is a compression fossil dated at 163 Ma, and due to it having larger amounts of missing data, a conservative view would consider Mecysmaucheniidae as providing weaker evidence than Archaeidae. Even so, these 2 examples provide evidence that the composition of the Burmese amber fauna reflects previous connections to Gondwana. The timing of when the BT broke away from Australia is controversial based on geological evidence, but based on biological evidence, Poinar (2018) suggests that the BT could not have broken away before the Early Cretaceous, 145 Ma. Our results for the Archaeidae (group 3) and the Mecysmaucheniidae (group 4) support this timing: the Burmese amber group breaks away from its closest southern relatives around 130 Ma (95% HPD: 144–118 Ma), and around 140 Ma (95% HPD: 167–113 Ma), respectively.
The Palpimanidae (group 1 in Fig. 2, bs = 100%) are estimated to have a distribution in southern Pangea. Pangea was not uniform across its entirety, having distinct climates and biomes in different regions (Parrish 1993; Rees et al. 2002) and the distribution of Palpimanidae may reflect this regionalization. In group 2, containing Huttoniidae, Stenochilidae, and their fossil relatives, the results are harder to interpret. Huttoniidae diverges first and the remaining group has an estimated ancestral range of the BT. The timing of this first divergence occurred around 180 Ma, when Pangea first begins rifting. But alternatively, the range of the 95% HPD (205–154 Ma) also suggests divergence could have preceded Pangean breakup. The estimated ancestral range of group 2 is ambiguous, with a similar summed relative likelihood of being widespread across Pangea (47%) as with occupying only southern Pangea (39%). Future discovery of additional fossils may help to clarify biogeography patterns within this group.
“Out of Burma” Dispersal in the Cretaceous
There are 3 cases where taxa from the Northern Hemisphere are phylogenetically nested within a clade known from the BT, and the ancestral range reconstructions also show range expansion/dispersal from the BT into the Holarctic (see asterisks in Figs. 1 and 2). The 95% HPD for when these 3 Holarctic groups diverged from their Burmese amber relatives spans the Early Cretaceous (average mean: 109 Ma; range:130–101 Ma). The 3 groups are 1) Stenochilidae, which has a current distribution from Southwest to Southeast Asia, and down to New Guinea, for 2) the †Spatiator species from Eocene Baltic amber, and for 3) the archaeids †Baltarchaea and its undescribec sister species, both from Eocene Baltic amber. In fact, this study suggests that the numerous archaeid fossils from northern Europe in the Eocene are an assemblage of 3 different lineages: 1) a Northern Hemisphere group (†Archaea, †Eoarchaea, and †Myrmecarchaea) that likely diverged from its southern relatives when Pangea split into Laurasia and Gondwana; 2) a group (†Baltarchaea + an undescribed species) that dispersed into northern Europe from the BT in the Cretaceous; and 3) †Saxonarchaea dentata, an enigmatic species that is a close relative with the extant Madagascan and African archaeids.
Our study suggests range expansion from the BT resulted in cladogenesis of the 3 groups above (asterisks in Fig. 2), but that the timing of dispersal from the BT into Asia occurred earlier than when the BT is thought to have collided with Asia, in the Late Cretaceous according to Seton et al (2012), and the Eocene according to Westerweel et al (2019). Our results in turn offer biological evidence that the BT may have collided with Asia earlier than thought, or that maybe over-water dispersal occurred (e.g., via rafting on debris) prior to collision, when the BT was in close proximity to Asia. Either way, our study suggests that some of the lineages on the BT persisted beyond the time when the Burmese amber deposit was formed, and dispersed into the Northern Hemisphere. Surveying the Eocene flora of Myanmar, Huang et al (2021) also concluded that the BT facilitated biotic exchange from Gondwana to Asia. Performing biogeography analysis on a dated phylogeny, Turk et al. (2020) came to similar conclusion to explain present-day distribution of Nephilidae spiders, as did Bolotov et al (2022) in their biogeography study of freshwater mussels, but neither study included Burmese amber fossils as terminal tips. The results from Bolotov et al. suggest BT collision with Asia occurred in the Eocene.
Conclusions
The only phylogenetic study thus far to examine fossil and extant palpimanoid relationships focused primarily on Archaeidae and included only 4 fossil specimens (Wood et al. 2012, 2013). At this time most of the Palpimanoidea Burmese amber fossils had yet to be documented. The current study increases the fossil taxonomic sampling and here, includes nearly 95% of all known fossil palpimanoid taxa (excluding †Lagonomegopidae) (Dunlop et al. 2023). A few fossil species were not included because they are dubious (e.g., Saxonarchaea diabolica may present juvenile characteristics, precluding identification even to the family level). Extant palpimanoids are well sampled in our study except for Palpimanidae, which has some genera from areas that were not included in this study (e.g., South and Southeast Asia, the Indian Ocean islands), and thus, palpimanid results should be treated cautiously. For the remaining groups, because the taxonomic sampling is nearly complete for both fossil and extant groups, we consider biogeographic conclusions robust.
Burmese amber offers a glimpse of the flora and fauna that rifted from Gondwana in the Early Cretaceous and traveled northward in isolation for millions of years, and then acting as a “biotic ferry” transporting specimens to Asia. The strongest evidence is in Archaeidae, where a distinct fossil Northern Hemisphere group falls outside of the group containing BT fossils and members from the Southern Hemisphere. Within Palpimanoidea, “out of Burma” transfer occurred 3 times, and these lineages showed up later in Baltic or Bitterfeld amber of Eocene age, or persisted in Asia to the present day. Using the fossil record we are able to examine Palpimanoidea evolution through time and the future discovery of more fossil species will refute or corroborate our findings. Lastly, the real significance of studies in biogeography comes about when similar results are replicated across the tree of life and general patterns emerge. Our hope is that this study will spur additional research examining vicariance due to continental drift and the potential role the BT played as a biological ferry.
Supplementary Material
Supplementary Information and data are available from the Dryad Digital Repository: https://doi.org/10.5061/dryad.12jm63z11.
Acknowledgments
We thank the following for their images from the “Assembling the Tree of Life: Phylogeny of Spiders” project (NSF EAR-0228699): Paola Favre, Cristian Grismado, Charles Griswold, Matías Izquierdo, Facundo Labarque, Joel Ledford, Martín Ramírez, and Alma Saucedo. We acknowledge Guilherme Azevedo, Petra Sierwald, and Adrian Harris for their images of Zelotes duplex and Schizocosa ocreata. Phylogenetic analyses were performed using the Smithsonian Institution High Performance Computing Cluster (doi.org/10.25572/SIHPC) and the Cipres Science Gateway. For access to specimens we thank: Mónica Solórzano Kraemer, Axel Christian, Peter Jäeger, Christian Neumann, Jason Dunlop, Ulrich Kotthoff, Wolfgang Weitschat, Günter Bechly, Hukum Singh, David Grimaldi, Petra Sierwald, Lauren Esposito and Chris Grinter. We thank Matjaž Kuntner, Ivan Magalhães, and 1 anonymous reviewer for providing valuable comments that improved the manuscript.
Author Contributions
HMW conceived the study, gathered data, performed analysis, and wrote the manuscript. JW acquired and curated the majority of amber specimens. HMW and JW discussed the results.
Conflict of Interest
The authors declare no competing interests.
Funding
This work was supported by research support funds to HMW from the National Museum of Natural History, Smithsonian Institution, for travel to Germany to examine fossil specimens.