-
PDF
- Split View
-
Views
-
Cite
Cite
Karolina Golec-Staśkiewicz, Jakub Wojciechowski, Maciej Haman, Tomasz Wolak, Joanna Wysocka, Agnieszka Pluta, Unveiling the neural dynamics of the theory of mind: a fMRI study on belief processing phases, Social Cognitive and Affective Neuroscience, Volume 19, Issue 1, 2024, nsae095, https://doi.org/10.1093/scan/nsae095
- Share Icon Share
Abstract
Theory of mind (ToM), the ability to interpret others’ behaviors in terms of mental states, has been extensively studied through the False-Belief Task (FBT). However, limited research exists regarding the distinction between different phases of FBT, suggesting that they are subserved by separate neural mechanisms. Further inquiry into this matter seems crucial for deepening our knowledge of the neurocognitive basis of mental-state processing. Therefore, we employed functional Magnetic Resonance Imaging (fMRI) to examine neural responses and functional connectivity within the core network for ToM across phases of the FBT, which was administered to 61 healthy adults during scanning. The region-of-interest analysis revealed heightened responses of the temporoparietal junction (TPJ) during and increased activation of medial prefrontal cortex (mPFC) during the outcome phase. Negative connectivity between these regions was observed during belief-formation. Unlike the TPJ, mPFC responded similarly to conditions that require belief reasoning and to control conditions that do not entail tracking mental states. Our results indicate a functional dissociation within the core network for ToM. While the TPJ is possibly engaged in coding beliefs, the mPFC shows no such specificity. These findings advance our understanding of the unique roles of the TPJ and mPFC in mental-state processing.
Introduction
Successful social interaction relies heavily on theory of mind (ToM), our ability to interpret others’ behaviors by understanding their mental states (Premack and Woodruff 1978). ToM involves inferring others’ beliefs, goals, or desires to predict and adapt to their actions in social interactions. The behavioral manifestation of ToM has been the subject of extensive research in various age groups and clinical populations over the past decades; however, the neuronal and cognitive mechanisms of ToM are still debated (Quesque and Rossetti 2020, Golec-Staśkiewicz et al. 2022). The predominant approach in this domain involves utilizing the False-Belief Task and/or its modifications (FBT; Dennett 1978) to evaluate whether individuals comprehend that another agent holds beliefs influencing their behaviors. A standard change-of-location FBT typically involves a scenario presented through a story or cartoon, where a protagonist is either aware or unaware of a change in circumstances (e.g. an object is moved or replaced in their absence). If the protagonist witnesses the change, their belief aligns with reality and is referred to as a true belief. However, if the protagonist is unaware of the change, their belief becomes outdated, i.e. false. The key challenge for participants is to demonstrate an understanding that others can hold beliefs that are false (Wimmer and Perner 1983). Though the complexity of the attribution differs, both conditions require participants to attribute a mental state to the protagonist and explicitly answer the task’s question based on such attribution (see Mahy et al. 2014).
Hence, at the cognitive level, solving the belief conditions of FBT may involve two successive computational processes: (I) forming the representation of another person’s mental state which might be inconsistent with objective reality (e.g. “A believes that the key is in their pocket, although B put it in the drawer”), often referred to as the belief-formation phase, and (II) using this representation to perform the task, e.g. respond to a question, referred to as the outcome phase (Kovács et al. 2014).
The distinction between the belief-formation and outcome phases has already been suggested by previous studies (Leslie and Thaiss 1992, Leslie et al. 2005, Kovács et al. 2010, Qureshi et al. 2010). It has been argued that the former is an efficient computation dedicated to social information regarding others’ mental states, which can be triggered automatically by the mere presence of social agents (Kovács et al. 2010). Once computed, such a representation of a mental state seems to be maintained for further use in the outcome phase (Aichhorn et al. 2009), as it has been demonstrated to influence our social behavior (see Kovács et al. 2010). In contrast, the outcome phase typically requires deliberate actions and is therefore thought to rely more heavily on effortful, controlled, domain-general mechanisms such as response inhibition, selection, and updating (Leslie et al. 2005, Qureshi et al. 2010). An argument supporting the notion that these phases are relatively distinct is backed by research, such as that of Qureshi et al. (2010). They demonstrated that overloading cognitive resources by requiring participants to perform an additional task impaired performance during the outcome phase, but did not disrupt the representation of mental states in a perspective-taking task. This was indicated by the fact that participants still computed the avatar’s perspective, even when it was irrelevant to the task (leading to slower responses in cases of inconsistency), despite simultaneously performing an executive task. The studies conducted on the pediatric population using nonverbal measures of looking behavior during implicit FBTs (with no explicit instruction to track and report beliefs) also seem to support the notion of a dissociation between belief formation and outcome processes in FBTs. For instance, it has been shown that by the second year of life, toddlers tend to expect other social agents’ actions to be consistent with their false beliefs (Onishi and Baillargeon 2005, Southgate et al. 2007). This suggests that the mechanism for belief formation, which involves representing others’ mental states, is already present by the second year of life. However, the ability to accurately respond in the outcome phase of verbal/explicit FBTs, which typically requires acting on this belief information, emerges later during the preschool years. This delay is often attributed to the maturation of executive and linguistic functions (Baillargeon et al. 2010).
The divergent behavioral patterns in belief processing phases suggest separate neural mechanisms. This issue is crucial in studying ToM neurocognitive foundations. Existing neuroimaging studies have identified a network of brain regions consistently involved in FBTs, comprising the temporoparietal junction (TPJ), the superior temporal sulcus (STS), the precuneus (PC), the temporal poles, and the medial prefrontal cortex (mPFC) (Saxe and Kanwisher 2003, Schurz et al. 2014). Due to the complexity of social information required for belief processing, activation of the ToM network is frequently accompanied by the activation of brain regions involved in various subprocesses of social cognition, such as action perception and emotion processing (Van Overwalle 2008, Schurz et al. 2021, Golec-Staśkiewicz et al. 2022, Arioli et al. 2023). However, the bilateral TPJ and mPFC have been consistently recognized by meta-analytic data as crucial nodes specific to ToM, often referred to as the core network for ToM (Mar 2011, Schurz et al. 2014, Molenberghs et al. 2016). Mounting evidence suggests functional specialization within the ToM core network (Saxe and Powell 2006, Van Overwalle 2008, Golec-Staśkiewicz et al. 2022). The right temporoparietal junction (RTPJ) is thought to play a pivotal role in the mechanisms involved in representing others’ mental states (Santiesteban et al. 2012, Carter and Huettel 2013, Molenberghs et al. 2016). The role of the mPFC in ToM is a subject of ongoing discussion (Hartwright et al. 2014, Lieberman et al. 2019, Lieberman 2022). While it has been proposed that its function extends beyond coding others’ mental states to encompass broader social agent characteristics, such as personality traits (Ma et al. 2014, Ferrari et al. 2016), the mPFC is also consistently associated with general cognitive functions, including executive control and memory. These functions support success in various complex tasks like the FBT, but may not be specific to either ToM or the domain of social cognition (Friedman and Robbins 2022). For instance, in a study on 7-month-old infants, Hyde et al. (2018) found temporoparietal, but no prefrontal, activity during implicit FBT (see also: Richardson et al. 2018). This aligns with the above-mentioned behavioral findings that delineate the distinction between the belief-formation and the outcome stage of the FBT. Given this background, providing further data on how the core network for ToM responds during the two phases of belief reasoning could yield insights into the potentially distinct roles of the TPJ and mPFC in ToM.
However, consensus is currently lacking regarding which phase of the FBT should be considered indicative of accurate ToM reasoning. This ability is often considered a uniform social-cognitive capacity (Schaafsma et al. 2015). Consequently, neural activity related to the belief-formation and outcome phases is often undifferentiated and analyzed jointly (e.g. Dodell-Feder et al. 2011, Jacoby et al. 2016). In several studies that considered phase differences, the authors often focused on the belief-formation phase as representative of ToM ability, omitting data related to the outcome period (e.g. Kovács et al. 2014, Nijhof et al. 2018, Boccadoro et al. 2019). Others, for instance, took another approach, focusing on analyzing the outcome phase (Schneider et al. 2014). Hence, the potential for divergent results in neural activity patterns seems noteworthy, depending on the FBT phase that is being analyzed. For example, Schneider et al. (2014), focusing exclusively on the FBT’s outcome phase, observed no typical activity patterns in the right temporoparietal regions. This contrasts with findings from Boccadoro et al. (2019), Kovács et al. (2014), and Nijhof et al. (2018), who reported such patterns during the belief-formation period in both implicit and explicit FBTs.
Meanwhile, in the aforementioned studies, results regarding the mPFC’s role in false-belief processing phases are mixed, with medial prefrontal activations being absent in the belief-formation phase in most cases (Boccadoro et al. 2019, Nijhof et al. 2018; but see: Kovács et al. 2014). Regarding the outcome phase, Schneider et al. (2014) did not observe increased mPFC activity. However, the majority of studies that analyzed data from both the belief-formation and outcome periods together consistently reported activation in the mPFC (Dodell-Feder et al. 2011, Jacoby et al. 2016).
Therefore, the available neuroimaging data seems to support the behavioral results, indicating a dissociation between the belief-formation and outcome phases, despite being relatively scarce. To our knowledge, only two studies, Aichhorn et al. (2009) and (Bardi et al. 2017a), have directly compared the two phases, producing inconsistent results. Aichhorn et al. (2009) found activity in the bilateral TPJ during both phases but did not observe mPFC activation in either phase. Conversely, Bardi et al. provided evidence suggesting functional dissociation between the TPJ and mPFC. The TPJ was observed to respond primarily during the belief-formation phase. At the same time, the mPFC showed activation during the outcome phase in both implicit and explicit ToM versions of the task. However, it is important to note that the experimental task in this study aimed to contrast mechanisms of implicit and explicit ToM, rather than FBT phases (Bardi et al. 2017a). Hence, the durations of belief-formation and outcome vary significantly, impeding direct comparison of the neural mechanisms underlying these phases of mental-state processing. Given the time resolution of fMRI, differences in brain activation between FBT phases should be interpreted with caution. Therefore, more data from direct comparisons of specific phases are needed to determine brain regions involved in each FBT stage.
Existing data indicating a potential functional dissociation between the TPJ and mPFC have been derived primarily from univariate analyses. These analyses help identify regions with significant responses during specific phases of false-belief reasoning and show the extent to which these regions are involved in particular phases of the task relative to each other. Investigating the dynamics within the ToM core network during both phases of the FBT could provide a broader understanding of functional divisions between the TPJ and mPFC (Moessnang et al. 2016). However, whether and how the TPJ and mPFC communicate during the ToM task remains unclear. Addressing this question could involve examining changes in functional connectivity between these regions, elicited by certain phases of FBT (Biswal et al. 1995, Friston 2011, Cole et al. 2021, Di et al. 2021). An increase in functional connectivity between brain areas during a particular task condition can indicate an enhanced exchange of information between them, suggesting that they work in concert to support the underlying cognitive process. Conversely, a decrease in functional connectivity during a specific phase of the task could imply a reduction in information transfer and a possible disengagement of one of these structures from the process being served (O’Reilly et al. 2012). Thus, task-related functional connectivity analysis could strengthen conclusions regarding the specificity of activity in a particular region-of-interest(ROI) during any phase. Specifically, the generalized psychophysiological interaction (gPPI; McLaren et al. 2012) is a prominent regression-based method of analysis that might reveal such task-related connectivity changes (Friston et al. 1997).
Therefore, the goal of the current fMRI study was to directly compare the activity and functional connectivity of TPJ and mPFC in two stages of false-belief-processing. We aimed to confront the issue of functional specialization within the core network for ToM by determining which brain regions were involved in each stage of FBT (and to what extent relative to each other) and how the functional connectivity between these regions changed with the phase of the task. To this end, we combined ROI and gPPI analyses. We employed a change-of-location FBT designed to differentiate between the belief-formation and outcome phases, featuring experimental conditions that required belief reasoning (true and false belief) and a control condition that did not involve mental-state tracking (no belief). Building on the literature, we hypothesized that the TPJ selectively responds to belief-formation, while the mPFC is preferentially activated during the outcome phase. Furthermore, we anticipated observing negative functional connectivity between the TPJ and mPFC ROIs during the belief-formation period. In this case, we expected these effects to be present in both true and false-belief conditions, but not in the control condition. However, we also posited that if the mPFC’s role is specific to ToM as a social-cognitive ability, it will exhibit sensitivity to mental states (beliefs) by displaying greater responsiveness to true and false-belief conditions compared to the no belief condition.
Methods
Participants
We recruited 61 healthy undergraduate volunteers without a history of neurological or psychiatric treatment. Four subjects were excluded due to incomplete data, leaving a final sample of 57 participants (White/Caucasian, 31 females; mean age: 27.07, SD = 7.52; years of education: M = 16.56, SD = 2.97). All participants were right-handed with normal or corrected-to-normal vision, provided written informed consent, and received financial compensation of 110 PLN (approximately 25 EUR). The study received approval from the local ethical committee and adhered to the principles of the Declaration of Helsinki.
Procedure
The study is part of a broader project investigating the neurocognitive basis of ToM. The Magnetic Resonance Imaging (MRI) procedure comprised two 60 min sessions, detailed in our previous work by Golec-Staśkiewicz et al. (2022). The FBT (19:14 min) was administered at the start of the second session, along with the T1-weighted (6:52 min) imaging sequence. During the FBT, we assessed BOLD response, accuracy, and response times.
False-Belief Task
Participants performed the FBT in the MRI scanner. The task comprised 35 s animations depicting a female protagonist, two boxes, and a toy. Each animation began with the toy (moving autonomously) placed in the first box. The protagonist is absent throughout the scene in the no-belief (NB) condition. In the true-belief (TB) and false-belief (FB) conditions, the protagonist observes the toy entering the first box before leaving the scene. Then, the two subsequent phases can be indicated in the videos: the belief-formation phase (18–24 s) and the “outcome phase” (29–35 s; Fig. 1).
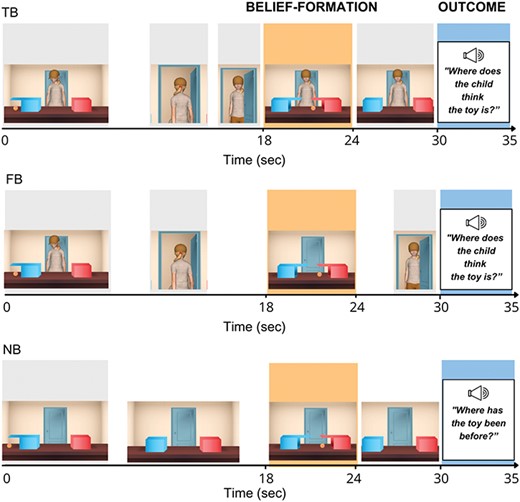
The structure of trials in three conditions—false-belief (FB), true-belief (TB), and no-belief (NB)—is shown, with the belief-formation phase marked in orange and the outcome phase marked in blue.
Belief-formation phase
In the belief-formation phase, the toy autonomously moves to the second box. This stage varies between conditions. In the TB condition, the protagonist witnesses the relocation, maintaining an up-to-date (true) belief about the toy’s location. In the FB condition, the protagonist is unaware of the relocation, resulting in an outdated (false) belief about the toy’s location. In the NB condition, no mental state can be attributed, due to the protagonist’s absence, leading the subject to solely represent the object’s location.
Outcome phase
In the outcome phase, the screen freezes and displays the recorded question. Participants are instructed to press the button corresponding to the proper box (left or right). In the belief conditions (TB, FB), participants report the protagonist’s mental state (“Where does the child think the toy is?”), while in the NB condition, they indicate the toy’s previous physical location (“Where has the toy been before?”).
There were 8 animations per condition, presented in 2 runs of 12 trials each, resulting in a total of 24 trials. The order of TB, FB, and NB trials, as well as the toy’s initial location (pink or blue box), were pseudo-randomized, such that concurrent trials were always of different types. The jitter was introduced at the beginning of the run and between trials, with the display of a static picture of the sun serving as the fixation cross, lasting 9–12.5 s. We adhered to the standard of keeping regressors (conditions and phases) of interest orthogonal from each other (<0.2) to ensure an unbiased estimation of the effects of each phase of the task in our experimental design (Poldrack et al. 2011).
fMRI data acquisition
Functional scans were acquired during the Explicit False-Belief Task (TA = 9:34 min, 704 volumes) using a 3 T Siemens Prisma MRI scanner equipped with a 64-channel phased-array RF head coil. We employed a Multi-band Echo Planar Imaging (EPI) sequence (TR = 800 ms, TE = 38 ms, flip angle = 52°, FOV = 216 × 216 mm, 108 × 108 mm image matrix, 72 transversal slices of 2 mm slice thickness, voxel size of 2 × 2 × 2 mm, Slice Accel. Factor = 8, In-Plane Accel. Factor = 1, IPAT = 8). Functional images were collected in two runs with opposite phase-encoding directions (anterior–posterior and posterior–anterior) to compensate for phase-encoding direction distortions. Structural images were obtained using a T1-weighted 3D MP-Rage sequence (TR = 2400 ms, TI = 1000 ms, TE = 2.74 ms, 8°flip angle, FOV = 256 × 256 mm, image matrix 320 × 320 mm, voxel size of 0.8 × 0.8 × 0.8 mm, 240 slices of 0.8 mm slice thickness, TA = 6:52 min). Experimental protocol was displayed using a VisualSystem HD (NordicNeuroLab).
fMRI data preprocessing
For ROI analysis, fMRI data were preprocessed using SPM12 (Ashburner and Friston 2005) and custom scripts. Spatial distortions were corrected using FSL’s topup (Andersson et al. 2003), aligning images from both encoding directions. Functional data were then realigned to the mean image and co-registered with individual structural images. After segmentation, structural data were normalized to Montreal Neurological Institute space, and the transformation was applied to functional volumes. Spatial smoothing (full-width at half maximum = 6 mm) and high-pass filtering of 0.008 Hz were applied to functional images.
For task-based functional connectivity analysis, we used the CONN toolbox (version 21a, Whitfield-Gabrieli and Nieto-Castanon 2012). The data underwent similar preprocessing to the ROI analysis, but with additional denoising steps, in accordance with standard CONN preprocessing pipeline (Nieto-Castanon 2020). This included regressing out task-related effects (boxcar function of all task-related conditions convolved with canonical hemodynamic response function) and five main PCA components for each white matter and cerebrospinal fluid noise signals using aCompCor (Behzadi et al. 2007). Additionally, six head-movement parameters and their first-order derivatives were modeled as nuisance regressors.
fMRI data analysis
ROI analysis
First-level analysis was performed subject-wise in SPM12 using a general linear model. Phases of the FBT were modeled separately for each task condition, resulting in six regressors: 2 (belief-formation, outcome) × 3 (FB, TB, NB), each lasting 6 s. All regressors were convolved with the canonical hemodynamic response function. The model also included six subject-specific movement regressors per run to correct for head motion.
To evaluate how the core network for ToM responds during specific phases of FBT, we conducted a ROI analysis. ROIs were defined a priori as 8 mm spheres centered around peak coordinates identified in a meta-analysis by Schurz et al. (2014), denoting regions consistently engaged in various ToM tasks and thus recognized as the core ToM network. These regions encompass the right posterior TPJ (RTPJp), left posterior TPJ (LTPJp), and three mPFC clusters—ventromedial PFC (vmPFC), dorsomedial PFC (dmPFC), and pre-supplementary motor area (preSMA)—defined based on connectivity parcellation of mPFC by Sallet et al. (2013). The localization of ROIs is depicted in Fig. 2. Mean beta values for the regressors of interest were obtained from the individual first-level models and then subjected to repeated measures analysis of variance (ANOVA), with phase (belief-formation, outcome) and FBT condition (FB, TB, NB) factors, performed in R Studio. Bonferroni correction was applied for multiple comparisons.
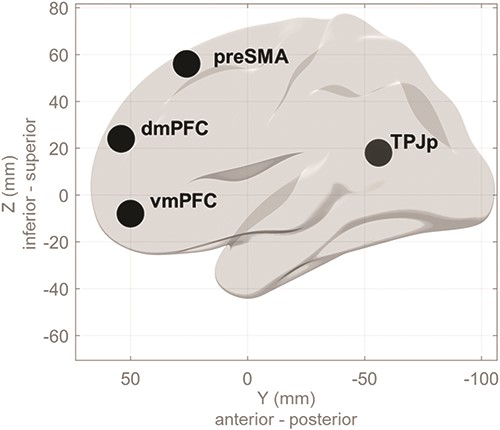
Task-related connectivity analysis
To assess changes in functional connectivity within the core network for ToM across stages of false-belief processing, we utilized ROI-to-ROI gPPI analysis implemented in CONN, using a bivariate correlation approach. The connectivity analysis on subject-level was conducted for each of the five designated ROIs (seed regions) with the remaining ones (target regions). For each seed region, a gPPI term was defined as the product of the seed ROI’s BOLD signal (physiological factor) and the boxcar signals characterizing each task phase (belief formation, outcome) and condition (FB, TB, NB), convolved with an SPM canonical hemodynamic response function (psychological factors). Alterations in connectivity between each seed ROI and all other target ROIs for each of the conditions were assessed by the estimated beta coefficients of the gPPI terms in each target ROI. On group-level analysis, we selected ROIs in which changes in connectivity could be explained by the task phase. To this end, F-tests were performed separately for each condition. Next, a simple effects analysis was conducted on these selected ROIs to explore patterns of connectivity at each level of the phase factor, condition-wise. Post-hoc t-tests were applied to evaluate changes in coupling for each significant ROI-to-ROI connection. Results were reported using a false discovery rate (FDR) threshold with cluster-based correction employing a multivariate pattern analysis omnibus test. This involved grouping the significant seed-to-target connections (P < .05, uncorrected) into clusters using hierarchical clustering and a singular value decomposition (SVD)-based procedure (Nieto-Castanon 2022). Only clusters that survived p-FDR correction (P < .05) were considered statistically significant.
Results
Behavioral results
The summary of the behavioral performance in the FBT is presented in Table 1. Participants demonstrated good performance and maintained focus on the task during the fMRI assessment.
Summary of behavioral results from the FBT across three conditions [false-belief (FB), true-belief (TB), no belief (NB)]
Task . | Accuracy: mean (standard deviation) . | Mean response times: mean (standard deviation) . |
---|---|---|
FBT | FB: 95.9% (11.8%) | FB: 2858.0 ms (880.2 ms) |
TB: 98.8% (5.4%) | TB = 2908.3 ms (823.6 ms) | |
NB: 98.6% (7.3%) | NB = 2917.9 ms (802.2 ms) |
Task . | Accuracy: mean (standard deviation) . | Mean response times: mean (standard deviation) . |
---|---|---|
FBT | FB: 95.9% (11.8%) | FB: 2858.0 ms (880.2 ms) |
TB: 98.8% (5.4%) | TB = 2908.3 ms (823.6 ms) | |
NB: 98.6% (7.3%) | NB = 2917.9 ms (802.2 ms) |
Summary of behavioral results from the FBT across three conditions [false-belief (FB), true-belief (TB), no belief (NB)]
Task . | Accuracy: mean (standard deviation) . | Mean response times: mean (standard deviation) . |
---|---|---|
FBT | FB: 95.9% (11.8%) | FB: 2858.0 ms (880.2 ms) |
TB: 98.8% (5.4%) | TB = 2908.3 ms (823.6 ms) | |
NB: 98.6% (7.3%) | NB = 2917.9 ms (802.2 ms) |
Task . | Accuracy: mean (standard deviation) . | Mean response times: mean (standard deviation) . |
---|---|---|
FBT | FB: 95.9% (11.8%) | FB: 2858.0 ms (880.2 ms) |
TB: 98.8% (5.4%) | TB = 2908.3 ms (823.6 ms) | |
NB: 98.6% (7.3%) | NB = 2917.9 ms (802.2 ms) |
ROI results
The ANOVA analysis revealed differential activation patterns for temporoparietal and medial prefrontal regions during the FBT. Significant main effects of phase were observed in LTPJp (F [1,56] = 8.914; P = .004, η2 = 0.025), preSMA (F[1,56] = 34.491; P < .001, η2 = 0.097), and dmPFC (F[1,56] = 4.201; P = .045, η2 = 0.015). LTPJp exhibited stronger activation during the belief-formation than the outcome phase (t[170] = 3.54; P= .001, d = 0.271), with a similar trend observed in RTPJp (F[1,56] = 3.157; P = .081, η2 = 0.012), showing a stronger response to the belief-formation phase compared to the outcome phase (t[170] = 2.24, P = .026, d = 0.171). Conversely, preSMA (t[170] = 7.79; P < .001, d = 0.595) and dmPFC (t[170] = 2.58; P = .011, d = 0.197) exhibited a more robust BOLD response during the outcome phase.
The main effect of condition was evident in bilateral TPJ (RTPJp: F[2.0,112.00] = 16.025, P < .001, η2 = 0.038; LTPJp: F[2112] = 12.710, P < .001, η2 = 0.041) and vmPFC (F[2112] = 4.981; P = .008, η2 = 0.023). Both true (RTPJp: t[113] = -4.44, P < .001, d = 0.416; LTPJp: t[113] = −3.25, P = .005, d = 0.304) and false (RTPJp: t(113) = −5.05, P < .001, d = 0.473; LTPJp: t(113) = −5.03, P < .001, d = 0.471) belief conditions elicited stronger responses in bilateral TPJ compared to the NB control condition. In contrast, the vmPFC exhibited a higher BOLD response to the NB condition than both the TB (t[113] = 3.28; P = .004, d = 0.307) and FB conditions (t[113] = 2.63; P = .029, d = 0.246).
A significant interaction between phase and condition was observed in RTPJp (F[1.8, 100.64] = 5.447, P = .007, η2 =0.017), vmPFC (F(2112) = 7.674, P = .001, η2 =0.019), and dmPFC (F(2112) = 4.307, P = .016, η2 =0.013). Pairwise comparisons revealed that the difference between belief and no-belief conditions in RTPJ was evident only during the belief-formation stage (FB vs. NB: t[56] = 4.92, P < .001, d = 0.652; TB vs. NB: t[56] = 4.95, P < .001, d = 0.655). Additionally, RTPJp exhibited a significantly stronger response during the belief-formation than the outcome phase in the TB condition (t[56] = 2.94, P = .005, d = 0.389). In vmPFC and dmPFC, stronger activation was observed for the FB than TB condition during the belief-formation phase (vmPFC: t[56] = 2.75, P = .024, d = 0.364; dmPFC: t[56]= 2.57, P = .039, d = 0.340). However, there were no significant differences between the belief and control conditions in the medial prefrontal ROIs during the belief-formation period, except for a more robust reaction to the NB than TB condition in vmPFC (t[56] = 3.65, P = .002, d = 0.483). In the outcome phase, the NB condition evoked a significantly greater BOLD response than the FB in vmPFC (t[56] = 2.81, P = .02, d = 0.372). Generally, higher activation was observed during the outcome than the belief-formation period in vmPFC for the TB condition (t[56] = 2.27, P = .027, d = 0.301) and in dmPFC for both TB (t[56] = 2.58, P < .05, d = 0.341) and NB (t[56] = 2.39, P = .02, d = 0.316) conditions (see Fig. 3).
![ROI analysis revealed an interaction between the phase (belief-formation, outcome) and condition [false-belief (FB), true-belief (TB), no-belief (NB)] of the FBT, with significant effects shown in the upper row and indicated by a black frame, where the belief-formation phase is marked in orange and the outcome phase in blue.](https://oup.silverchair-cdn.com/oup/backfile/Content_public/Journal/scan/19/1/10.1093_scan_nsae095/1/m_nsae095f3.jpeg?Expires=1747852865&Signature=Tgbx4KYP9vaMviE70eCaWs~0ZjoKyAcms2t6xWQY5F1PQxTt7we4r7MOkq0LtgH0Frz78YW4zWVpnqC9Xt~efoH-vlWJJ9kIt8V6OafunN83rViVjxBloMOvMabAENgd~TVibYkpUXlCbkGgfcT6sZBBPUEB8FLvmHN8jFhnRVcg~p49-c-kjcE7K8in2B3Q8mTQK6UMfIe3KsL0OL3JWdbf-KUsWqrkHuGbwW5Zja9eX74~2cHAXvG4TpchmZjoLL-nMYNSJ48FISwoFJwieNpF9wraSnfSviinDd9jb36VtNFgv5Beaqam0YRHLyCLX6R1R~OO2m~h1I1nyfe4NQ__&Key-Pair-Id=APKAIE5G5CRDK6RD3PGA)
ROI analysis revealed an interaction between the phase (belief-formation, outcome) and condition [false-belief (FB), true-belief (TB), no-belief (NB)] of the FBT, with significant effects shown in the upper row and indicated by a black frame, where the belief-formation phase is marked in orange and the outcome phase in blue.
Connectivity results
The ROI-to-ROI gPPI F-test analysis demonstrated a main effect of phase in both belief conditions (FB, TB) but not in the control condition (NB). For FB, functional connectivity in two clusters changed significantly depending on the task phase. The first cluster involved connections between bilateral TPJp and medial prefrontal regions (F[4,53] = 4.50, P = .008), while the second cluster comprised connectivity between right and left TPJp (F[4,53] = 6.15, P = .002). Subsequent simple effects analysis (see Table 2, Fig. 4a) indicated that in the FB condition, changes in connectivity were driven by the belief-formation phase rather than the outcome phase. Specifically, bidirectional negative connectivity was observed between bilateral TPJ and ventromedial and dorsomedial PFC ROIs during belief-formation. Additionally, a significant decrease in connectivity was detected between RTPJp and LTPJp during belief-formation in the FB condition. In the TB condition, connectivity significantly changed with the phase of FBT in a cluster formed by bilateral TPJp, dmPFC, and vmPFC (F[4,53] = 5.41, P = .001). Simple effects analysis (see Table 2, Fig. 4b) revealed a bidirectional decrease in connectivity between bilateral TPJp, vmPFC, and dmPFC during belief-formation in the TB condition, similar to FB. Furthermore, decreased connectivity between LTPJp and RTPJp was observed during the outcome phase in the TB condition (see Table 2, Fig. 4c).
![Simple effects of FBT phases on task-modulated functional connectivity between ROIs across FBT conditions [false-belief (FB), true-belief (TB), no-belief (NB)] are shown, with negative changes in connectivity depicted in blue and positive changes in red (though no positive changes were observed).](https://oup.silverchair-cdn.com/oup/backfile/Content_public/Journal/scan/19/1/10.1093_scan_nsae095/1/m_nsae095f4.jpeg?Expires=1747852865&Signature=Eute6B4jUgeEmFGsKMcgSX-Z-kvPSQKsX8fNOAwjeYcN1OWZI2PUbO2jU2gV-vi0Y09QFy2ObYuolOUVSdWt9RBFBVZo0SYv3~bha296NXigf85aTOu2Mjs1Zp2vwPy5qQHKJbqeGEj1PnNzMv9-S1Rh3Pv4tAffGrCoksDke8OjgJZIVLfO66j0MGjVq2ZMmpprawPzjrzR1u~Z-3Vrb-eHqspuwESugiTCHi0pXopf2IQ122vQNuKhlS4laomRDlfU8EFy1c5azwWO3OclYHIxiNHeeqTo8lh9BAfl~IcGUDIYUkIsgH34tI-mrUIN3FJq~E7xWUyQPTROyj3n0A__&Key-Pair-Id=APKAIE5G5CRDK6RD3PGA)
Simple effects of FBT phases on task-modulated functional connectivity between ROIs across FBT conditions [false-belief (FB), true-belief (TB), no-belief (NB)] are shown, with negative changes in connectivity depicted in blue and positive changes in red (though no positive changes were observed).
FBT condition, phase . | Connection . | Statistic . | P-value . |
---|---|---|---|
FB, belief-formation | Cluster 1 (F(2,55) = 7,60, P = .004 | ||
vmPFC—LTPJp | T (56) = −3.18 | .005 | |
LTPJp—vmPFC | T (56) = −2.53 | .021 | |
vmPFC—RTPJp | T (56) = −3.08 | .005 | |
RTPJp—vmPFC | T (56) = −2.47 | .025 | |
dmPFC—LTPJp | T (56) = −3.03 | .011 | |
dmPFC—RTPJp | T (56) = −2.67 | .015 | |
RTPJp—dmPFC | T (56) = −2.09 | .041 | |
Cluster 2 (F(2,55) = 6,34, P = .005 | |||
RTPJp—LTPJp | T (56) = −3.51 | .003 | |
FB, outcome | – | – | – |
TB, belief-formation | Cluster 1 (F(2,55) = 4,78, P = .037 | ||
vmPFC—RTPJp | T (56) = −3.08 | .010 | |
RTPJp—vmPFC | T (56) = −2.62 | .034 | |
vmPFC—LTPJp | T (56) = −2.43 | .027 | |
LTPJp—vmPFC | T (56) = −2.48 | .043 | |
LTPJp—dmPFC | T (56) = −2.25 | .043 | |
dmPFC—RTPJp | T (56) = −2.24 | .090 | |
TB, outcome | Cluster 1 (F(2,55) = 6,33, P = .010 | ||
LTPJp—RTPJp | T (56) = −3,57 | .002 | |
NB, belief-formation | – | – | – |
NB, outcome | – | – | – |
FBT condition, phase . | Connection . | Statistic . | P-value . |
---|---|---|---|
FB, belief-formation | Cluster 1 (F(2,55) = 7,60, P = .004 | ||
vmPFC—LTPJp | T (56) = −3.18 | .005 | |
LTPJp—vmPFC | T (56) = −2.53 | .021 | |
vmPFC—RTPJp | T (56) = −3.08 | .005 | |
RTPJp—vmPFC | T (56) = −2.47 | .025 | |
dmPFC—LTPJp | T (56) = −3.03 | .011 | |
dmPFC—RTPJp | T (56) = −2.67 | .015 | |
RTPJp—dmPFC | T (56) = −2.09 | .041 | |
Cluster 2 (F(2,55) = 6,34, P = .005 | |||
RTPJp—LTPJp | T (56) = −3.51 | .003 | |
FB, outcome | – | – | – |
TB, belief-formation | Cluster 1 (F(2,55) = 4,78, P = .037 | ||
vmPFC—RTPJp | T (56) = −3.08 | .010 | |
RTPJp—vmPFC | T (56) = −2.62 | .034 | |
vmPFC—LTPJp | T (56) = −2.43 | .027 | |
LTPJp—vmPFC | T (56) = −2.48 | .043 | |
LTPJp—dmPFC | T (56) = −2.25 | .043 | |
dmPFC—RTPJp | T (56) = −2.24 | .090 | |
TB, outcome | Cluster 1 (F(2,55) = 6,33, P = .010 | ||
LTPJp—RTPJp | T (56) = −3,57 | .002 | |
NB, belief-formation | – | – | – |
NB, outcome | – | – | – |
The significant simple effects during the FBT are shown.
FBT condition, phase . | Connection . | Statistic . | P-value . |
---|---|---|---|
FB, belief-formation | Cluster 1 (F(2,55) = 7,60, P = .004 | ||
vmPFC—LTPJp | T (56) = −3.18 | .005 | |
LTPJp—vmPFC | T (56) = −2.53 | .021 | |
vmPFC—RTPJp | T (56) = −3.08 | .005 | |
RTPJp—vmPFC | T (56) = −2.47 | .025 | |
dmPFC—LTPJp | T (56) = −3.03 | .011 | |
dmPFC—RTPJp | T (56) = −2.67 | .015 | |
RTPJp—dmPFC | T (56) = −2.09 | .041 | |
Cluster 2 (F(2,55) = 6,34, P = .005 | |||
RTPJp—LTPJp | T (56) = −3.51 | .003 | |
FB, outcome | – | – | – |
TB, belief-formation | Cluster 1 (F(2,55) = 4,78, P = .037 | ||
vmPFC—RTPJp | T (56) = −3.08 | .010 | |
RTPJp—vmPFC | T (56) = −2.62 | .034 | |
vmPFC—LTPJp | T (56) = −2.43 | .027 | |
LTPJp—vmPFC | T (56) = −2.48 | .043 | |
LTPJp—dmPFC | T (56) = −2.25 | .043 | |
dmPFC—RTPJp | T (56) = −2.24 | .090 | |
TB, outcome | Cluster 1 (F(2,55) = 6,33, P = .010 | ||
LTPJp—RTPJp | T (56) = −3,57 | .002 | |
NB, belief-formation | – | – | – |
NB, outcome | – | – | – |
FBT condition, phase . | Connection . | Statistic . | P-value . |
---|---|---|---|
FB, belief-formation | Cluster 1 (F(2,55) = 7,60, P = .004 | ||
vmPFC—LTPJp | T (56) = −3.18 | .005 | |
LTPJp—vmPFC | T (56) = −2.53 | .021 | |
vmPFC—RTPJp | T (56) = −3.08 | .005 | |
RTPJp—vmPFC | T (56) = −2.47 | .025 | |
dmPFC—LTPJp | T (56) = −3.03 | .011 | |
dmPFC—RTPJp | T (56) = −2.67 | .015 | |
RTPJp—dmPFC | T (56) = −2.09 | .041 | |
Cluster 2 (F(2,55) = 6,34, P = .005 | |||
RTPJp—LTPJp | T (56) = −3.51 | .003 | |
FB, outcome | – | – | – |
TB, belief-formation | Cluster 1 (F(2,55) = 4,78, P = .037 | ||
vmPFC—RTPJp | T (56) = −3.08 | .010 | |
RTPJp—vmPFC | T (56) = −2.62 | .034 | |
vmPFC—LTPJp | T (56) = −2.43 | .027 | |
LTPJp—vmPFC | T (56) = −2.48 | .043 | |
LTPJp—dmPFC | T (56) = −2.25 | .043 | |
dmPFC—RTPJp | T (56) = −2.24 | .090 | |
TB, outcome | Cluster 1 (F(2,55) = 6,33, P = .010 | ||
LTPJp—RTPJp | T (56) = −3,57 | .002 | |
NB, belief-formation | – | – | – |
NB, outcome | – | – | – |
The significant simple effects during the FBT are shown.
Discussion
In this study, we investigated the functional divisions within the core network for ToM across stages of belief processing. We examined the responses of temporoparietal (RTPJp, LTPJp) and medial prefrontal (preSMA, dmPFC, vmPFC) ROIs during the belief-formation and outcome phases of the explicit FBT. In addition to the results obtained from a traditional ROI analysis, we explored task-modulated functional connectivity. Our findings revealed differential patterns of BOLD response and connectivity for temporoparietal and medial prefrontal ROIs during the FBT, suggesting a potential functional dissociation between the bilateral TPJ and mPFC.
The temporoparietal ROIs exhibited more pronounced responses to belief (false and true) conditions compared to the no-belief condition. Such differentiation did not occur in the medial prefrontal regions. This suggests that the bilateral TPJ, but not the mPFC, is particularly sensitive to mental states, consistent with a wealth of previous neuroimaging research pointing to the central role of the TPJ in tracking belief-like states (Schurz et al. 2014, 2021). The TPJ’s neuroanatomical position may facilitate its role as a hub integrating inputs from various brain regions to construct a comprehensive representation of the social context, including others’ mental states (Carter and Huettel 2013, Patel et al. 2019). Perceptual data on social actions that are relayed to the TPJ through connections with various brain regions, such as the fusiform gyrus and lateral occipital complex (related to person and object identity), lateral occipitotemporal cortex (movement), anterior intraparietal sulcus (action understanding), and posterior superior temporal sulcus (biological motion), are thought to be utilized in forming this representation (see Lieberman 2022, for a detailed discussion).
Secondly, our study revealed that the bilateral TPJ did not differentiate between false and true beliefs, suggesting its role in general belief processing, consistent with previous studies (Saxe and Kanwisher 2003, Young et al. 2007, Aichhorn et al. 2009, Jenkins and Mitchell 2010, Bio et al. 2022). This finding, however, contrasts with Sommer et al. (2007), who demonstrated differential responses of the right TPJ to true and false beliefs. Based on such evidence, it has been proposed that the TB task may not necessarily require reasoning about beliefs but can instead be solved by reasoning about the actual state of reality (Saxe 2010). However, more recent behavioral and neuroimaging research offers a different perspective, aligning with our findings (Back and Apperly 2010, Döhnel et al. 2012). For example, participants are slower to respond to questions about true and false beliefs than to questions about reality (Back and Apperly 2010). Additionally, in direct comparisons with reality-processing control conditions—absent in Sommer et al. (2007)—both true and false-belief conditions evoke activity in the right TPJ (Döhnel et al. 2012). Therefore, we propose that both false and true belief conditions elicit a similar neural activation pattern, as both engage the cognitive process of belief representation (Saxe and Kanwisher 2003). However, they differ in complexity, as false beliefs—unlike true beliefs—do not align with reality or the observer’s knowledge (Qureshi et al. 2010, Deschrijver and Palmer 2020, Arioli et al. 2023). In fact, it has been suggested that beyond representation, social cues related to beliefs may induce relational processing—specifically, detecting and monitoring the extent to which one’s own beliefs mismatch those of others (Deschrijver and Palmer 2020, Arioli et al. 2023). Such a mismatch, which is greater in the FB than in the TB condition, seems to require greater executive control (e.g. inhibiting the spontaneous tendency to respond according to one’s own beliefs) and might be detected by the TPJ, especially in the left hemisphere. Hartwright et al. (2012) also observed that brain activity in both the bilateral TPJ and executive control regions, such as the dorsal anterior cingulate cortex, varied depending on whether the belief being processed was true or false. All this evidence suggests that TPJ activity, though specific to mental-state processing, may be modulated by processing demands. However, this modulation was not evident in the current study, which could stem from the task design. The task was tailored to study neurodevelopmental mechanisms of ToM, with executive demands reduced to a minimum for child participants (Wysocka et al. 2020, Golec-Staśkiewicz et al. 2022). Although the FB condition was the most challenging behaviorally, as indicated by accuracy (see the “Results” section), it is possible that solving it in adults did not require significantly greater TPJ activation compared to the TB condition.
In contrast, the medial prefrontal regions might be rather attuned to the cognitive load of the stimuli. Our findings indicate that while the dmPFC and vmPFC responded similarly to both belief (TB, FB) and control (NB) conditions, suggesting a lack of specificity to mental states, they showed greater activation in the FB condition compared to the TB condition. This is consistent with the findings of Dohnel and colleagues, who proposed that the activity of the mPFC reflects general reasoning and conflict monitoring required by FBT to a greater extent than true-belief tasks (2012). The results of Arioli et al. (2023) also implicated the role of the dmPFC in monitoring the discrepancy between self- and other perspectives, inhibition and switching between them. While the function of the vmPFC and dmPFC has been extensively discussed within the realm of social cognition (Bzdok et al. 2013), recent neuroimaging research employing both univariate and multivariate approaches has emphasized the role of these mPFC subsections in cognitive control and decision-making across diverse cognitive domains, in a task-independent manner (Kuzmanovic et al. 2018, Piva et al. 2019, Yao et al. 2023, Wojciechowski et al. 2024). The precise functional parcellation of mPFC is, however, beyond the scope of the current study.
In accordance with our hypotheses, the bilateral TPJ showed a stronger reaction during the belief-formation phase than during the outcome phase. In two of three prefrontal ROIs (preSMA and dmPFC), we observed an inverse relationship, with higher reactivity during the outcome phase than during the belief-formation stage. Accordingly, we demonstrated that the phases of FBT significantly modulated changes in functional connectivity between the TPJ and mPFC, which resulted in a negative connectivity between temporoparietal and prefrontal ROIs during the belief-formation phase. Together, these results support the idea of a distinction between the belief-formation and the outcome phases of the FBT (Leslie and Thaiss 1992, Leslie et al. 2005, Kovács et al. 2010, Qureshi et al. 2010). By showing that the two phases of the FBT seem to be subserved by nonoverlapping mechanisms within the core network for ToM, our outcomes imply a functional division between TPJ and mPFC. Specifically, the former appears to be preferentially engaged during belief-formation, while the latter supports the outcome period. This is consistent with the results by Boccadoro et al. (2019), Nijhof et al. (2018), and Schneider et al. (2014). Furthermore, it may elucidate why patterns of activity involving both TPJ and mPFC are observed when the phases of the FBT are not distinguished in neuroimaging analyses and the whole task is treated as an undivided whole (Dodell-Feder et al. 2011, Jacoby et al. 2016).
Moreover, we demonstrated that during the belief formation phase, compared to the outcome phase, the TPJ is preferentially sensitive to mental states. Hence, this finding supports the notion that the belief-formation period of the FBT, rather than the outcome phase, is crucial for selectively assessing the neural basis of ToM (Bardi et al. 2017a, Nijhof et al. 2018, Boccadoro et al. 2019). Kovács et al. (2010) propose that in both adults and infants as young as seven months, mental-state computations during the belief-formation phase of the FBT are automatically triggered by the mere presence of other social agents. Our results, including the negative coupling between bilateral TPJ and mPFC ROIs observed specifically during the belief-formation period of belief (TB, FB) but not control (NB) conditions, suggest that this process might rely on the TPJ particularly. This observation could explain why temporoparietal activity, rather than prefrontal activity, is usually detected during the implicit FBT, where no explicit instructions regarding mental-state tracking were given. This pattern was observed in preverbal infants, as reported by Hyde et al. (2018), and in adults, as noted by Boccadoro et al. (2019). It may seem contradictory to the well-established notion that the mPFC consistently activates during explicit FBTs, where participants are directly instructed to reason about others’ mental states and answer related questions (see Schurz et al. 2014, or Van Overwalle 2008, for meta-analyses). However, our findings show that such prefrontal activity during ToM tasks might stem from the enhanced cognitive control required to provide accurate responses during the outcome phase. In fact, Powell and Carey (2017) showed that depletion of executive function impairs FBT performance in 5-year-old children who are proficient in this type of task. Taken together, this suggests that executive functions enable the use and expression of previously acquired knowledge about others’ beliefs, a process that unfolds in the outcome phase.
By showing the anticorrelated connectivity patterns between the LTPJ and RTPJ during various phases of the FBT, we demonstrate that these regions do not necessarily operate in concert when processing mental states. Instead, they likely support distinct cognitive processes activated during the belief formation and outcome phases of the FBT. Although our task does not allow for fine-grained functional parcellation of the bilateral TPJ, the current results contribute to the ongoing debate on its lateralization (Arioli et al. 2023). Previous studies have associated the LTPJ with perceiving differences between the mental states of self and others—i.e. relational processing (Aichhorn et al. 2009, Perner et al. 2006, Schurz et al. 2013, Arora et al. 2015), but see Arioli et al. 2023, Bardi et al. 2017b for similar findings regarding the RTPJ). Interestingly, due to its proximity to left-hemispheric language processing areas, the LTPJ may also play a role in integrating domain-general semantic systems (e.g. language comprehension) with social information processing (Lin et al. 2018). While data from patients with left temporo-parietal lesions suggest that the LTPJ is necessary for successful perspective-taking (Samson et al. 2004, Biervoye et al. 2016), existing evidence points to RTPJ dominance in both implicit and explicit FBT tasks (Döhnel et al. 2012, Bardi et al. 2017a, Nijhof et al. 2018, Boccadoro et al. 2019). As such, the RTPJ is hypothesized to compute a generalized model of the social context (Carter and Huettel 2013), including representations of others’ mental states that are formed automatically during the belief formation phase (Hartwright et al. 2012, Bio et al. 2022, Lieberman 2022).
Overall, our results seem to align with the model of pre-reflective subjective construal proposed by Lieberman (2022). According to the model, to evaluate the significance of the observed social scene, the TPJ first automatically and efficiently codes the mental states of other social agents. Our results suggest that this coding possibly occurs during the belief formation phase. Only then does the mPFC perform cognitive evaluation or reasoning about the represented information.
Limitations
One limitation of our study stems from the perceptual difference between the FB and TB conditions, namely the presence of the protagonist during belief-formation in TB but not in FB. While this design choice allowed us to examine responses to both false and true-beliefs, it may have influenced ROI responses, particularly in the TB condition due to the presence of a social agent. This limitation warrants consideration, in the context of discussing the functional divisions in the ToM network, as we argue that the lack of differences between TB and FB in the temporoparietal ROIs might point to the insensitivity of the TPJ to executive demands. However, our conclusions remain robust, as similar results have been reported in studies with visually matched conditions and pattern-sensitive multivariate analyses (e.g. Bio et al. 2022).
Conclusions
The study contributes to the ongoing debate regarding the functional divisions within the core network for ToM. We provided evidence that the TPJ, rather than the mPFC, exhibits functional specificity for ToM. We demonstrated that tracking of the belief-like states, with the TPJ playing a key role in this process, occurred primarily during the belief-formation period. We argue that the mPFC, on the other hand, subserves cognitive and executive control which enables the utilization of preexisting knowledge about others’ beliefs in order to anticipate their behavior during the outcome phase. Overall, our findings suggest a functional dissociation between the TPJ and mPFC in the domain of social-cognition and thus seem vital for future studies aiming to selectively assess the ToM ability and its neural correlates.
Acknowledgements
We would like to thank all the participants who consented to participate in this study. We would also like to thank Patrycja Dzianok for designing the 3D graphics used as stimuli in the experiment.
Author contributions
Karolina Golec-Staśkiewicz (Conceptualization [lead], Formal Analysis [equal], Investigation [lead], Methodology [equal], Project Administration [lead], Visualisation [equal], Writing—Original Draft Preparation [lead], Writing—Review & Editing [equal]), Jakub Wojciechowski (Data Curation [lead], Methodology [equal], Formal Analysis [equal], Visualization [equal], Writing—Original Draft Preparation [supporting], Writing—Review & Editing [supporting]) Maciej Haman (Conceptualization [supporting], Methodology [equal], Supervision [equal], Writing—Review & Editing [supporting]) Tomasz Wolak (Formal Analysis [supporting], Methodology [equal], Resources [lead], Supervision [supporting], Writing—Review & Editing [supporting]) Joanna Wysocka (Methodology [equal], Visualisation [equal]), Agnieszka Pluta (Conceptualization [equal], Funding Acquisition [lead], Investigation [supporting], Methodology [lead], Project Administration [supporting], Supervision [lead], Writing—Original Draft Preparation [supporting], Writing—Review & Editing [equal]).
Conflict of interest
None declared.
Funding
This work was funded by The Polish National Science Center under OPUS Grant [2017/25/B/HS6/01624] to A.P.
Data availability
The datasets generated for this study are available upon request from the corresponding authors. The unthresholded and unmasked group-level whole-brain results maps are available at the Neurovault repository: https://neurovault.org/collections/QFRETHYJ/