-
PDF
- Split View
-
Views
-
Cite
Cite
Liu Cong, Yi-ke Shi, Xin-yi Gao, Xiao-fei Zhao, Hai-qi Zhang, Feng-li Zhou, Hong-juan Zhang, Bai-quan Ma, Rui Zhai, Cheng-quan Yang, Zhi-gang Wang, Feng-wang Ma, Ling-fei Xu, Transcription factor PbNAC71 regulates xylem and vessel development to control plant height, Plant Physiology, Volume 195, Issue 1, May 2024, Pages 395–409, https://doi.org/10.1093/plphys/kiae011
- Share Icon Share
Abstract
Dwarfism is an important agronomic trait in fruit breeding programs. However, the germplasm resources required to generate dwarf pear (Pyrus spp.) varieties are limited. Moreover, the mechanisms underlying dwarfism remain unclear. In this study, “Yunnan” quince (Cydonia oblonga Mill.) had a dwarfing effect on “Zaosu” pear. Additionally, the dwarfism-related NAC transcription factor gene PbNAC71 was isolated from pear trees comprising “Zaosu” (scion) grafted onto “Yunnan” quince (rootstock). Transgenic Nicotiana benthamiana and pear OHF-333 (Pyrus communis) plants overexpressing PbNAC71 exhibited dwarfism, with a substantially smaller xylem and vessel area relative to the wild-type controls. Yeast one-hybrid, dual-luciferase, chromatin immunoprecipitation-qPCR, and electrophoretic mobility shift assays indicated that PbNAC71 downregulates PbWalls are thin 1 expression by binding to NAC-binding elements in its promoter. Yeast two-hybrid assays showed that PbNAC71 interacts with the E3 ubiquitin ligase PbRING finger protein 217 (PbRNF217). Furthermore, PbRNF217 promotes the ubiquitin-mediated degradation of PbNAC71 by the 26S proteasome, thereby regulating plant height as well as xylem and vessel development. Our findings reveal a mechanism underlying pear dwarfism and expand our understanding of the molecular basis of dwarfism in woody plants.
Introduction
Plant height is related to plant architecture, lodging resistance, and yield, which makes dwarfism an important agronomic trait (Wang et al. 2017; Yang et al. 2021). Because of its advantages (e.g. early flowering, relatively simple mechanized management, and high yield), the high-density planting of dwarf pear (Pyrus spp.) trees has gradually replaced sparse planting practices to satisfy the demands of modern fruit production systems (Smolka et al. 2010; Zheng et al. 2018). Thus, there is a need for good dwarfing rootstocks and dwarf cultivars. Many dwarfing rootstocks and dwarf varieties are currently used for apple (Malus × domestica Borkh.) production, but there are relatively few resources for the development of dwarf pear cultivars (Elkins et al. 2012). Additionally, the molecular mechanisms regulating the height of woody plants remain unclear. Accordingly, further characterizing the mechanism underlying dwarfism may enhance the breeding of dwarf pear varieties.
The height of woody plants is considerably influenced by the development of the xylem, which is involved in water transport and nutrient storage, while also providing mechanical support (Pratt and Jacobsen 2017; Plavcová et al. 2019). In apple and peach, the rootstock xylem vessel size varies depending on tree vigor, with a substantial decrease in the number and size of xylem vessels in dwarfing rootstock (Tombesi et al. 2010; Tworkoski and Fazio 2015). Xylem formation requires the synthesis and deposition of secondary cell wall (SCW) components, including cellulose, hemicellulose, and lignin (Taylor-Teeples et al. 2015), which is mediated by the regulated expression of multiple genes. The NAM/ATAF/CUC (NAC) domain transcription factors (TFs), which belong to one of the largest plant TF families, are the master regulators of xylem formation. Earlier research showed VASCULAR-RELATED NAC-DOMAIN7 (VND7) is expressed specifically in vessel cells; the repressed expression of VND7 results in a xylem-deficient dwarf phenotype (Yamaguchi et al. 2011). Phylogenetically, NAC SECONDARY WALL THICKENING PROMOTING FACTOR1 (NST1) is the gene most closely related to the VND gene family, with Arabidopsis (Arabidopsis thaliana) AtNST1/3 encoding a regulator of secondary wall formation in woody tissues (Mitsuda et al. 2007; Mitsuda and Ohme-Takag 2008). The SECONDARY WALL-ASSOCIATED NAC DOMAIN1 (SND1) gene regulates the deposition of SCWs in A. thaliana fibers and affects wood formation in Populus species (Zhong et al. 2006; Li et al. 2012; Lin et al. 2017). In pear, the overexpression of Pb Xylem NAC Domain 1 (PbXND1), which encodes a negative regulator of secondary wall formation, leads to xylem deficiency and dwarfism (Xiao et al. 2022b). In addition to NAC family members, other genes are involved in SCW formation. The overexpression of PtrbHLH186 in Populus trichocarpa increases the proportion of small stem vessels and decreases plant height (Liu et al. 2022). The Walls are thin 1 (WAT1) gene, which is a homolog of the Medicago truncatula NODULIN21 (MtN21) gene, is essential for secondary wall formation in A. thaliana. Previous studies showed that WAT1 is a tonoplast membrane-localized auxin efflux carrier that controls SCW deposition in wood fiber tissues (Ranocha et al. 2010, 2013). Compared with the wild-type control, Atwat1 mutants are substantially shorter (i.e. dwarfism) and have substantially thinner secondary walls (Ranocha et al. 2010). In tomato, SlBZR1/2 facilitate xylem differentiation and the subsequent wood formation by activating SlWAT1 expression (Lee et al. 2021).
The post-translational modification of proteins leads to functional diversification. Ubiquitination-mediated degradation is an important post-translational regulatory process that modulates various physiological and biochemical processes (Li et al. 2011; Sun et al. 2019; Su et al. 2023; Zhang et al. 2023). In the ubiquitin/26S proteasome pathway, E3 ubiquitin ligase is a key determinant of substrate specificity. This enzyme specifically recognizes its substrate and catalyzes the formation of an isopeptic bond between the substrate and ubiquitin, thereby regulating a wide range of physiological and biochemical processes, including plant growth and development (Fleury et al. 2007; Zeba et al. 2009; Sun et al. 2019). The overexpression of the pepper (Capsicum annuum) gene RING ZINC FINGER PROTEIN 1 (CaRZFP1) in Nicotiana benthamiana enhances the growth of transgenic lines, leading to accelerated growth and increases in root size and fresh weight (Zeba et al. 2009). In A. thaliana, the endoplasmic reticulum-associated degradation (ERAD)-mediating RING finger protein regulates plant height by influencing the synthesis of the BR signaling-related protein on the endoplasmic reticulum (Park et al. 2018). Transgenic N. benthamiana plants overexpressing ZINC FINGER 1 (NbZFP1) reportedly have relatively short internodes and a sturdy stem phenotype (Wu et al. 2014).
In this study, we observed that the pear dwarfing rootstock “Yunnan” quince (Cydonia oblonga) substantially inhibits the growth of “Zaosu” shoots, and isolated a dwarfism-related NAC TF gene (PbNAC71). The transgenic pear OHF-333 (Pyrus communis) and N. benthamiana plants overexpressing PbNAC71 with abnormal xylem and vessel development exhibited obvious dwarfism. Moreover, PbNAC71 regulated PbWAT1 expression by binding to secondary wall NAC-binding elements (SNBEs). Furthermore, the interaction between PbRNF217 and PbNAC71 promoted the ubiquitination-mediated degradation of PbNAC71 through the 26S proteasome pathway, ultimately regulating plant height. Our findings provide insights relevant to breeding dwarf pear varieties, which is crucial for increasing pear fruit production in modern orchards.
Results
“Yunnan” quince inhibits scion shoot growth
Compared with the “Zaosu” (scion)/“Duli” (rootstock) (Z/D) plants, “Zaosu” (scion)/“Yunnan” quince (rootstock; with “Hardy” serving as the interstock) (Z/Q) plants were significantly shorter and had significantly more short shoots (Fig. 1, A to C). According to the analysis of annual shoot development at 10, 20, and 30 days after full bloom (DAFB), there was a significant decrease in the annual shoot length of the Z/Q plants (Fig. 1, D and E). Xylem development and hormone contents are important factors associated with dwarfism. The cross-sectional examination showed the xylem of the Z/Q shoots was significantly smaller than that of the Z/D shoots (Fig. 1, F and G). In terms of the hormone contents, the indole-3-acetic acid (IAA) and abscisic acid (ABA) contents decreased and increased significantly, respectively, in the Z/Q annual shoots at 10, 20, and 30 DAFB (Supplementary Fig. S1, A and D). In addition, the trans-zeatin (TZ) content decreased significantly at 20 DAFB (Supplementary Fig. S1C). There was no difference in the gibberellic acid (GA3) content between the Z/D and Z/Q plants (Supplementary Fig. S1B).
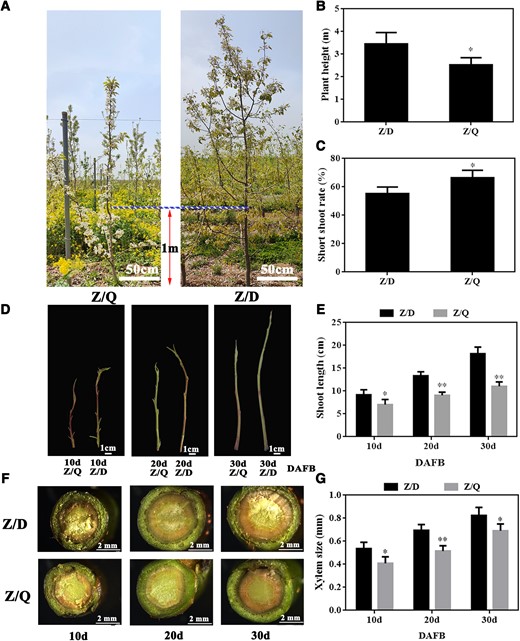
“Yunnan” quince induced the dwarfing of “Zaosu” pear. A) “Zaosu” pear grafted on “Duli” and “Yunnan” quince rootstocks. B) Plant height and C) short shoot rate of Z/D (“Zaosu”/“Duli”) and Z/Q (“Zaosu”/“Yunnan” quince). D) Annual shoots of Z/D and Z/Q at 10, 20, and 30 DAFB. DAFB, days after full bloom. E) Annual shoot length of Z/D and Z/Q at 10, 20, and 30 DAFB (images were digitally extracted for comparison). Bar, 1 cm. F) Cross section and G) xylem size of annual shoots at 10, 20, and 30 DAFB. Data are presented as the mean ± Sd (n = 9). Data were analyzed using Student’s t-test: *P < 0.05, **P < 0.01.
PbNAC71 negatively regulates Z/Q shoot growth
On the basis of the physiological data, the annual shoots at 20 DAFB were selected for the RNA-sequencing analysis, which revealed 224 genes that were differentially expressed (138 upregulated genes and 86 downregulated genes), including genes related to hormone pathways and growth (Fig. 2A; Supplementary Table S1). Among the differentially expressed genes (DEGs), the expression levels of four NAC TF-encoding genes (LOC103932913, LOC103932902, LOC103964786, and LOC103964789) were upregulated in the Z/Q shoots, with the largest increase in expression observed for LOC103932913 (Fig. 2B). To more precisely identify key dwarfism-related genes, we examined the expression of four NAC family genes in dwarf germplasm (e.g. “Aiganlu” and “Qingai 2/3”). Notably, the expression of only LOC103932913 was significantly upregulated in all dwarf germplasm (Fig. 2, C to F). Thus, LOC103932913 was selected for the further study. The phylogenetic analysis and amino acid sequence alignment results indicated LOC103932913 is closely related to MdNAC71L (Supplementary Fig. S2, A and B). Therefore, we named LOC103932913 as PbNAC71. The subcellular localization analysis suggested PbNAC71 is a nuclear and cytoplasmic protein (Supplementary Fig. S2C). Moreover, PbNAC71 was expressed in the flowers, ovaries, shoots, and leaves, but there was a significant decrease in PbNAC71 expression as the shoots elongated (Supplementary Fig. S2, D and E). These results imply that PbNAC71 might negatively regulate shoot growth.
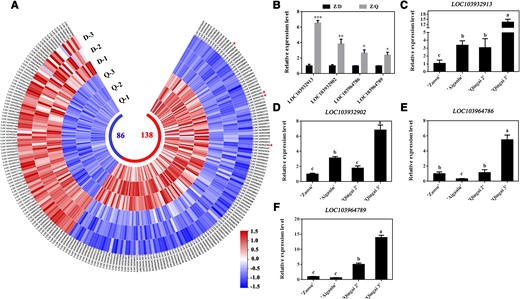
PbNAC71 was isolated as a dwarfism-related gene. A) Heat map of DEGs. D, the annual shoots of “Zaosu”/“Duli”; Q, the annual shoots of “Zaosu”/“Yunnan” quince. B) Relative expression levels of LOC103932913, LOC103932902, LOC103964786, and LOC103964789. Data are presented as the mean ± Sd (n = 3). Data were analyzed using Student’s t-test: *P < 0.05, **P < 0.01. Expression levels of (C) LOC103932913, (D) LOC103932902, (E) LOC103964786, and (F) LOC103964789 in “Aiganlu”, “Qingai 2”, “Qingai 3”, and “Zaosu” pear. Data are presented as the mean ± Sd (n = 3). Different letters indicate significant differences (P < 0.05, one-way ANOVA with Tukey’s test).
Phenotype of transgenic OHF-333 and N. benthamiana plants overexpressing PbNAC71
To functionally characterize PbNAC71, we overexpressed it in N. benthamiana (Fig. 3A; Supplementary Fig. S3). The resulting transgenic plants exhibited a distinct dwarf phenotype (Fig. 3A). Compared with the wild-type plants, the PbNAC71-overexpressing (OE) plants were significantly shorter and had a smaller diameter (Fig. 3, B and C). The microscopic examination of paraffin sections indicated that the xylem size and vessel area decreased significantly in the PbNAC71-OE transgenic N. benthamiana plants (Fig. 3, D and E). We also overexpressed PbNAC71 in OHF-333 plants (Fig. 3F; Supplementary Fig. S4). The significant decrease in the height of the transgenic OHF-333 plants was apparent 2 mo after transplanting (Fig. 3, G and H). According to the analysis of paraffin sections, there were significant decreases in the xylem size and vessel area in the transgenic OHF-333 plants (Fig, 3, I to M). These results were consistent with the findings for the transgenic N. benthamiana plants. Therefore, PbNAC71 expression induced pear dwarfism via the regulation of xylem and vessel development.
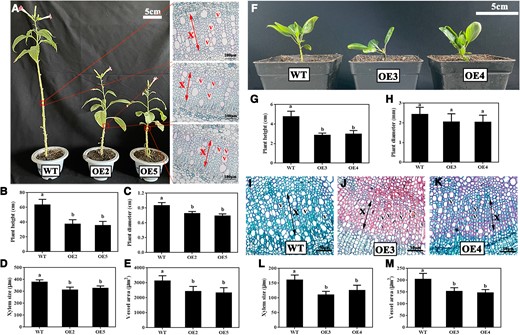
Overexpression of PbNAC71-induced dwarfism in OHF-333 and N. benthamiana. A) Four-month-old wild-type and transgenic tobacco plants (Bars, 5 cm) and their stem cross-sections (Bar, 100 μm). WT, wild type. OE2/5, transgenic N. benthamiana overexpressing PbNAC71. The red arrow refers to the xylem of transgenic N. benthamiana. X, xylem; V, vessel. B) Height and C) diameter of WT and transgenic N. benthamiana plants. D) Xylem size and E) vessel area of WT and transgenic N. benthamiana plants. Data are presented as the mean ± Sd (n = 15). Different letters indicate significant differences (P < 0.05, one-way ANOVA with Tukey’s test). F) WT and transgenic OHF-333 at 2 mo after transplanting. Bar, 5 cm. OE3/4, transgenic OHF-333 overexpressing PbNAC71. G) Height and H) diameter of WT and transgenic OHF-333 plants. Data are presented as the mean ± Sd (n = 5). Different letters indicate significant differences (P < 0.05, one-way ANOVA with Tukey’s test). I to K) Stem cross-sections of WT and transgenic OHF-333 plants. The black arrow refers to the xylem of transgenic OHF-333. Bar, 50 μm. L) Xylem size and (M) vessel area of WT and transgenic OHF-333 plants. Data are presented as the mean ± Sd (n = 15). Different letters indicate significant differences (P < 0.05, one-way ANOVA with Tukey’s test).
PbNAC71 binds to the PbWAT1 promoter and represses transcription
On the basis of the defective xylem of the transgenic plants, we analyzed the RNA-sequencing data and identified two secondary wall synthesis-related genes, namely PbWAT1 (LOC103928336) and PbWAT1-related (LOC103965756), as candidate downstream genes targeted by PbNAC71 (Supplementary Figs. S5A and S6A). The expression of both genes was significantly downregulated in the PbNAC71-OE lines, which was in accordance with our speculation that PbNAC71 controls their expression (Supplementary Figs. S5B and S6B). The PbWAT1 and PbWAT1-related amino acid sequences were similar to the sequences of their homologs AtWAT1 and AtWAT1-related, respectively (Supplementary Figs. S5D and S6C). Additionally, the atwat1 mutants exhibited a substantially dwarf phenotype, but the atwat1-related mutants did not (Supplementary Figs. S5E and S6D). Thus, PbWAT1 was isolated as a candidate downstream target of PbNAC71. The PbWAT1 expression level was significantly higher in the shoots and leaves than in the flowers and ovaries (Supplementary Fig. S5C). To functionally characterize PbWAT1, we overexpressed the gene in atwat1 mutants (Supplementary Fig. S5, E to H). This overexpression partially reversed the atwat1 mutant phenotype. Specifically, the PbWAT1-OE/atwat1 plants were significantly taller and had a larger vessel area than the atwat1 plants (Supplementary Fig. S5, I to M). Hence, PbWAT1 may modulate plant height via its regulatory effects on vessel development.
To determine whether PbWAT1 expression is directly regulated by PbNAC71, a dual-luciferase assay was performed. The 1,500-bp PbWAT1 promoter was cloned into the pGreenII 0800-LUC vector, whereas the full-length PbNAC71 coding sequence (CDS) was inserted into the MCS of the pGreenII 0029 62-SK binary vector. The dual-luciferase assay results showed that PbNAC71 can decrease the PbWAT1 promoter activity (Fig. 4A). Several secondary wall NAC TFs can bind to SNBEs. The analysis of the PbWAT1 promoter detected three SNBEs (P1, P2, P3; P4 fragment as a control) (Fig. 4B). By performing a yeast one-hybrid (Y1H) analysis, we determined that PbNAC71 can bind directly to the P1, P2, and P3 fragments (Fig. 4, C to F). We subsequently transiently overexpressed PbNAC71 in pear tissue culture seedlings and conducted a chromatin immunoprecipitation (ChIP)-qPCR assay. The ChIP-qPCR results revealed a significant increase in the P1, P2, and P3 fragments in the PbNAC71-OE lines (Fig. 4G). To further verify the binding between PbNAC71 and SNBEs, the PbWAT1 promoter SNBEs were analyzed in an electrophoretic mobility shift assay (EMSA). We observed PbNAC71 can bind to the P1, P2, and P3 fragments in vitro, with the unlabeled competitor substantially decreasing the binding of PbNAC71 (Fig. 4, H to K). According to these findings, PbNAC71 can bind specifically to the SNBEs in the PbWAT1 promoter and decrease promoter activity.
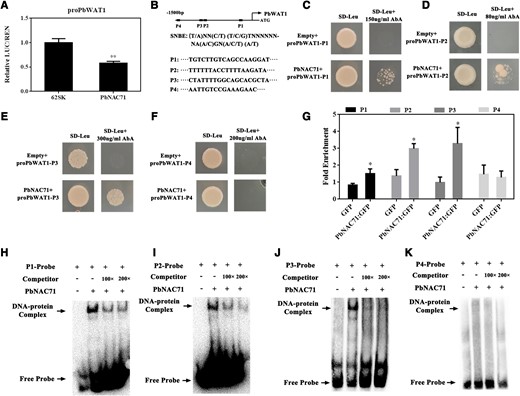
PbNAC71 was able to bind to the SNBEs of the PbWAT1 promoter and affect expression. A) The dual-luciferase assay indicated PbNAC71 represses the PbWAT1 promoter activity. LUC, firefly luciferase; REN, Renilla luciferase. 62SK, empty pGreenII 0029 62-SK binary vector as control. B) Three SNBEs in the PbWAT1 promoter (P4 fragment as a control). C to F) Y1H, G) ChIP-qPCR, and H to K) EMSA results verified the binding of PbNAC71 to the SNBEs in the PbWAT1 promoter. SD−, synthetic dropout nutrient medium. Data are presented as the mean ± Sd (n = 3). Data were analyzed using Student’s t-test: *P < 0.05, **P < 0.01.
PbRNF217 is an E3 ubiquitin ligase that interacts with PbNAC71
The yeast self-activation experiment detected the self-activation of the C-terminal of PbNAC71 (Fig. 5A). To further clarify the molecular network involving PbNAC71, the N-terminal of PbNAC71 was used for a yeast two-hybrid (Y2H) screening, which indicated the RING1-BRcat-Rcat (RBR)-type E3 ubiquitin ligase PbRNF217 (LOC103950223) can interact with PbNAC71 (Fig. 5B). The full-length PbRNF217 CDS (987 bp) encoded a protein comprising 328 amino acids (aa). The predicted protein contained a RING finger/U-box domain (123 to 176 aa), a BRcat domain (208 to 260 aa), and an Rcat domain (278 to 314) (Supplementary Fig. S7). Similar to PbNAC71, PbRNF217 was also localized to the nucleus and cytoplasm (Supplementary Fig. S2C). We produced constructs for the fusion of PbNAC71 to the YFP C-terminal fragment (YC) and the fusion of PbRNF217 to the YFP N-terminal fragment (YN). After the co-transfection involving PbNAC71-YC and PbRNF217-YN, the fluorescence of the reconstituted YFP was observed (Fig. 5C). The bimolecular fluorescence complementation (BiFC) assay results provided additional evidence of the interaction between PbNAC71 and PbRNF217 in plant cells. To assess whether PbNAC71 can interact with PbRNF217 in vitro, the PbNAC71-glutathione S-transferase (GST) and PbRNF217-His fusion proteins produced in a prokaryotic expression system were purified. The immunoblot analysis completed using an anti-His antibody detected PbRNF217-His in the experimental and control groups. Moreover, PbRNF217-His was able to pull down PbNAC71-GST (Fig. 5D). These results suggested that PbRNF217 can interact with PbNAC71 in vivo and in vitro.
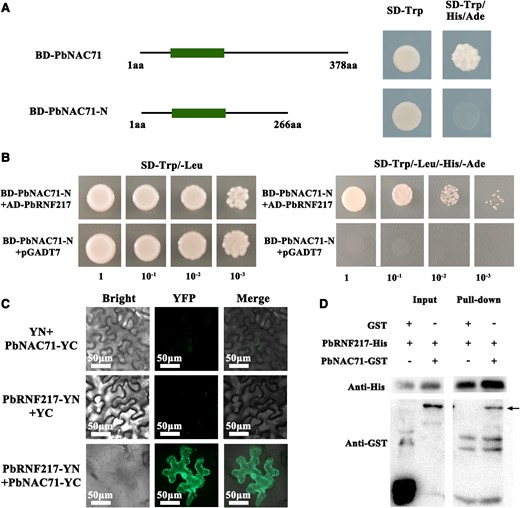
PbRNF217 interacted with PbNAC71. A) Analysis of the self-activation of PbNAC71. aa, amino acid; SD−, synthetic dropout nutrient medium; BD, pGBKT7 vector. B) The Y2H assay verified the interaction between PbRNF217 and PbNAC71. C) Interaction between PbRNF217 and PbNAC71 as determined by the BiFC assay. The PbNAC71-YC + YN (YFPN) and YC (YFPC) + PbRNF217-YN pairs were used as negative controls. Bar, 50 μm. D) The pull-down assay confirmed the interaction between PbRNF217 and PbNAC71. The PbRNF217-His protein was first incubated with anti-His magnetic beads and then the mixture was incubated with GST or PbNAC71-GST. The bound proteins were eluted and detected using anti-GST and anti-His antibodies. The black arrow refers to immunoblot of PbNAC71-GST.
PbRNF217 ubiquitinates PbNAC71
Because RBR-type E3 ubiquitin ligases reportedly promote the ubiquitination and degradation of target proteins (Spratt et al. 2014; Fernandez et al. 2020), we speculated that PbNAC71 may be targeted by PbRNF217 for ubiquitination and degradation. We analyzed the ubiquitination of PbNAC71 by co-expressing PbNAC71 and PbRNF217 in N. benthamiana leaves. The results of the immunoprecipitation involving anti-ubiquitin and anti-MYC antibodies indicated that the extent of the ubiquitination of PbNAC71 increased substantially in the leaves in which PbNAC71 and PbRNF217 were co-expressed (Fig. 6A), suggesting PbRNF217 can ubiquitinate PbNAC71 in vivo. To verify this result, we generated transgenic “Duli” roots overexpressing both PbNAC71 and PbRNF217 (Supplementary Fig. S8). Proteins extracted from transgenic plants were immunoblotted with anti-MYC antibody. The double-transgenic plants (PbNAC71-OE + PbRNF217-OE) accumulated less PbNAC71 than the single-transgenic plants (PbNAC71-OE) (Fig. 6B). In addition, an in vitro ubiquitination assay was performed to produce further evidence of the PbRNF217-mediated ubiquitination of PbNAC71. We extracted protein from the wild-type “Duli” roots and the PbRNF217-OE transgenic “Duli” roots and incubated them with PbNAC71-GST in the presence of MG132 or DMSO. The degradation of PbNAC71 was accelerated by the proteins extracted from the PbRNF217-OE roots (Fig. 6C), suggesting that PbRNF217 promotes the degradation of PbNAC71. However, the proteasome inhibitor MG132 substantially inhibited the degradation of PbNAC71, implying PbNAC71 degradation was mediated by the 26S proteasome (Fig. 6C).
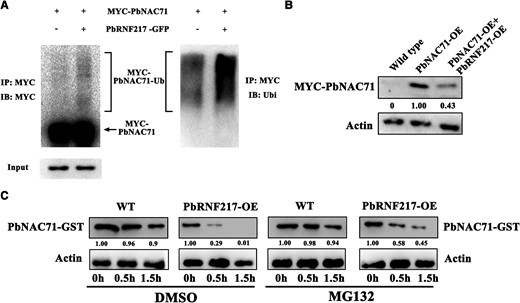
PbNAC71 was ubiquitinated by PbRNF217 and then degraded. A) PbRNF217 promotes the ubiquitination of PbNAC71 in N. benthamiana leaves. MYC-PbNAC71 was expressed alone or co-expressed with PbRNF217-GFP in N. benthamiana leaves. The proteins immunoprecipitated using the anti-MYC antibody were analyzed in a western blot involving anti-MYC and antiubiquitin antibodies. IP, immunoprecipitate; IB, immunoblot; Ubi, ubiquitin. B) The abundance of MYC-PbNAC71 in “Duli” roots was analyzed by immunoblotting using the anti-MYC antibody. OE, overexpressing. C) Degradation of PbNAC71 mediated by PbRNF217 in a cell-free system. Proteins were extracted from the PbRNF217-OE “Duli” roots or the wild-type “Duli” roots and then incubated with PbNAC71-GST and MG132 or DMSO for 0, 0.5, and 1.5 h at 22 °C. The abundance of PbNAC71 was determined via a western blot analysis involving the anti-GST antibody. β-Actin was used as the reference protein. WT, wild type.
PbRNF217 contributes to PbNAC71-induced dwarfism
To further elucidate the regulatory effects of PbRNF217–PbNAC71 on plant growth, we overexpressed PbRNF217 in transgenic N. benthamiana overexpressing PbNAC71 (Fig. 7A; Supplementary Fig. S3, B and C). The double-transgenic plants were significantly taller than the single-transgenic plants, but they were shorter than the wild-type controls (Fig. 7, A and E). The histological examination indicated that the xylem and vessel area were significantly larger in the double-transgenic plants than in the single-transgenic plants (Fig. 7, B to G). The expression level of NtWAT1-Like (NtWAT1L), which is a homolog of PbWAT1, was consistent with the histological observation. Specifically, NtWAT1L expression was significantly downregulated in the transgenic lines, but the downregulated expression was greater in the single-transgenic plants than in the double-transgenic plants (Supplementary Fig. S3D). The double-transgenic N. benthamiana plants were treated with MG132, which resulted in a substantial increase in the abundance of PbNAC71 (Fig. 7, H to K and N). The plant height, xylem and vessel area were significantly decreased at 8 days after treatment (DAT) (Fig. 7, L to M and O to Q). Similarly, the NtWAT1L expression level decreased significantly (Fig. 7R). Considered together, these results indicated that PbRNF217 likely affects plant height and xylem vessel development by promoting the ubiquitination-mediated degradation of PbNAC71.
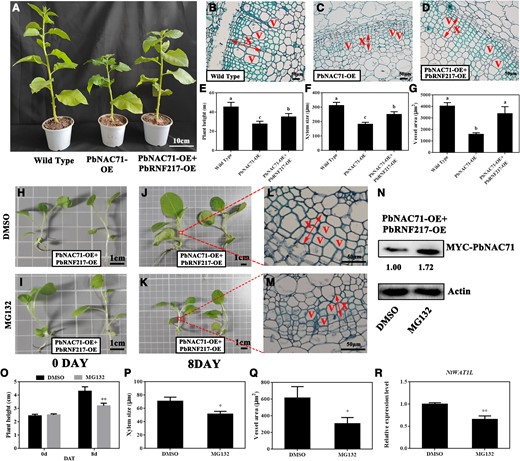
The PbRNF217-promoted degradation of PbNAC71 affected plant dwarfism. A) Two-month-old wild-type (WT), single-transgenic, and double-transgenic N. benthamiana plants. Bar, 10 cm. OE, overexpressing. Stem cross-sections of (B) WT, (C) single-transgenic, and (D) double-transgenic N. benthamiana plants. Bar, 50 μm. E) Height, F) xylem size, and G) vessel area of WT, single-transgenic, and double-transgenic N. benthamiana plants. Data are presented as the mean ± Sd (n = 15). Different letters indicate significant differences (P < 0.05, one-way ANOVA with Tukey’s test). H to K) Double-transgenic N. benthamiana plants treated with DMSO or MG132 for 0 and 8 d. L to M) Stem cross-sections of double-transgenic N. benthamiana plants treated with DMSO or MG132 at 8 DAT. Bars, 50 μm. The arrow refers to the xylem of transgenic N. benthamiana. N) Abundance of MYC-PbNAC71 in double-transgenic N. benthamiana plants at 8 DAT. The protein was detected using the anti-MYC antibody. O) Height of double-transgenic N. benthamiana plants before and after the treatment. P) Xylem size and Q) vessel area of double-transgenic N. benthamiana plants treated with DMSO or MG132 at 8 DAT. Data are presented as the mean ± Sd (n = 15). R)NtWAT1L expression level in double-transgenic N. benthamiana plants treated with DMSO or MG132 at 8 DAT. X, xylem; V, vessel. Data are presented as the mean ± Sd (n = 3). The asterisks in (O) to (R) indicate significant differences as determined by independent t-tests (*P < 0.05, **P < 0.01).
Discussion
Pear is a fruit that is consumed worldwide, but the development of dwarf pear varieties is limited by the lack of germplasm resources. Combining quince, which is commonly used as a dwarfing rootstock for pear cultivation, with the interstock “Hardy” results in an ideal dwarf phenotype (du Plooy and van Huyssteen 2000). Consistent with the findings of earlier research, we observed that “Yunnan” quince has an obvious dwarfing effect on “Zaosu” pear. “Yunnan” quince (with the interstock “Hardy”) significantly decreased the plant height and inhibited the development of annual shoots. Therefore, the mechanism underlying pear dwarfism may be investigated using the Z/Q scion/rootstock combination. Because “Yunnan” quince is a heterogeneous rootstock, we focused on the mechanism mediating the dwarfism of “Zaosu” pear. To elucidate the dwarfing mechanism of Z/Q, the RNA-sequencing was performed. The NAC TFs are involved in regulating the whole physiological process of plants and have been reported to induce dwarfing (Jia et al. 2018; Singh et al. 2021; Srivastava et al. 2022; Xiao et al. 2022b). There were a total of 185 NAC genes were found in pear (Ahmad et al. 2018). In this study, the expression levels of four NAC TF genes were significantly upregulated in Z/Q shoots, among which PbNAC71 was identified as the most significant DEG. We subsequently analyzed the expression of these four NAC genes in dwarf germplasm resources, including “Aiganlu”, “Qingai 2”, and “Qingai 3”. The expression of only PbNAC71 was significantly upregulated in all dwarf germplasm resources. Therefore, PbNAC71 was selected for further analyses.
As a NAC TF in pear, there are no reports regarding the potential contribution of PbNAC71 to dwarfism. Thus, we overexpressed PbNAC71 in OHF-333 and N. benthamiana plants. The transgenic pear and N. benthamiana plants overexpressing PbNAC71 were significantly shorter than the wild-type plants, suggesting PbNAC71 negatively regulates plant development. Xylem, which is an important factor influencing woody plant height, affects water transport, mechanical strength, and nutrient storage (Pratt and Jacobsen 2017; Plavcová et al. 2019). Compared with standard pear varieties, dwarf pear varieties have a smaller vessel diameter and a lower vessel density (Chen et al. 2015). In the current study, using “Yunnan” quince as the rootstock significantly decreased the size of the xylem in the annual shoots. Recent studies showed that NAC TF-induced abnormal xylem development can lead to dwarfism (Liu et al. 2022; Xiao et al. 2022b). To clarify the relationship between PbNAC71 expression and xylem development, the stems of transgenic pear and N. benthamiana plants were analyzed. The microscopic examination of paraffin sections indicated that the overexpression of PbNAC71 in transgenic lines significantly decreased the xylem size and vessel area. Therefore, PbNAC71 may induce dwarfism by regulating xylem and vessel development.
To explore how PbNAC71 modulates xylem and vessel development, we analyzed the RNA-sequencing data and determined that the expression levels of PbWAT1 and PbWAT1-related were significantly downregulated in Z/Q shoots and PbNAC71-OE OHF-333 plants. In A. thaliana and tomato, WAT1 encodes a critical regulator that precisely controls local auxin homeostasis, while also contributing to xylem differentiation (Ranocha et al. 2010, 2013; Lee et al. 2021). Protein structures and amino acid sequences are closely associated with protein functions. We determined that the amino acid sequences of PbWAT1 and PbWAT1-related are highly similar to those of their A. thaliana homologs, suggestive of the similarities in their functions. Additionally, the atwat1 mutant exhibited substantial dwarfism, but the atwat1-related mutant did not. Hence, we speculated that PbWAT1 is targeted by PbNAC71 during the regulation of xylem development. To further elucidate the PbWAT1 function, we overexpressed PbWAT1 in the atwat1 mutant. This overexpression reversed the dwarfism of the atwat1 mutant, with significant increases in the height and vessel area in the PbWAT1-OE/atwat1 plants. These results reflected the similarity in the PbWAT1 and AtWAT1 functions associated with xylem and vessel development. We performed a dual-luciferase assay to determine whether PbWAT1 is a downstream target of PbNAC71. The assay results indicated that PbNAC71 can inhibit PbWAT1 promoter activity. The promoter elements of PbNAC71, which is a NAC TF-encoding gene, were unclear. The SNBE is a palindromic 19 bp consensus sequence. Many NAC TFs, such as SNDs, regulate the expression of secondary wall synthesis-related genes by binding to SNBEs (Zhong et al. 2021). Thus, PbNAC71 may bind to SNBEs. Our sequence analysis identified four SNBEs in the 1,500 bp PbWAT1 promoter. The Y1H, ChIP-qPCR, and EMSA results suggested that PbNAC71 can bind directly to the P1, P2, and P3 fragments in the PbWAT1 promoter. Accordingly, PbNAC71 controls xylem and vessel development by binding to the SNBEs in the PbWAT1 promoter, thereby decreasing transcription.
Ubiquitination is a post-translational modification of proteins that influences multiple plant physiological and biochemical processes, including growth, development, and stress responses, by modulating protein stability and abundance (Sun et al. 2019; Su et al. 2023; Zhang et al. 2023). Our Y2H screening analysis identified an RBR-type E3 ubiquitin ligase (PbRNF217) as a protein that interacts with PbNAC71. RBR-type E3 ubiquitin ligase is an important group of E3 ligases (Spratt et al. 2014). In A. thaliana, AtRING finger ABA-related 1 (AtRFA1) and AtRFA4 mediate the degradation of PYRABACTIN RESISTANCE1 (PYR1) through the ubiquitin/proteasome pathway (Fernandez et al. 2020). Therefore, we hypothesized the regulatory effects of PbRNF217 involve the ubiquitination-mediated degradation of PbNAC71. This hypothesis was supported by the results of several experiments conducted in this study. First, the Y2H, BiFC, and pull-down assays demonstrated that PbRNF217 can interact with PbNAC71. Second, the co-expression of PbRNF217 and PbNAC71 substantially increased the accumulation of ubiquitinated PbNAC71 in N. benthamiana. Third, the presence of PbRNF217 accelerated the degradation of PbNAC71 in the cell-free degradation assay. Moreover, PbNAC71 was less abundant in the double-transgenic (PbNAC71-OE + PbRNF217-OE) plants than in the single-transgenic (PbNAC71-OE) plants, which implied that PbRNF217 promoted the degradation of PbNAC71. Ubiquitinated proteins enter the 26S proteasome and are degraded, which alters protein levels and affects several regulatory processes (Smalle and Vierstra 2004). We observed that the addition of the proteasome inhibitor MG132 adversely affected the degradation of PbNAC71 in vivo and in vitro, indicating that ubiquitinated PbNAC71 was degraded by the proteasome. Hence, PbRNF217 interacts with and ubiquitinates PbNAC71 for the subsequent degradation via the ubiquitin/proteasome pathway.
The regulatory effects of ubiquitination on plant development have been reported (Fleury et al. 2007; Zeba et al. 2009; Sun et al. 2019). Thus, we investigated whether PbRNF217 helps regulate PbNAC71-mediated plant development through ubiquitination and protein degradation. To prove this hypothesis, we further overexpressed PbRNF217 in PbNAC71-OE N. benthamiana. The abundance of PbNAC71 was relatively low in the double-transgenic plants, indicative of the PbRNF217-promoted degradation of PbNAC71. Compared with the single-transgenic N. benthamiana plants, the double-transgenic N. benthamiana plants were significantly taller and had a larger xylem and vessel area. Moreover, the expression of NtWAT1L (i.e. PbWAT1 homolog) was downregulated significantly more in the double-transgenic plants than in the single-transgenic plants. Notably, the MG132 treatment significantly inhibited the growth of double-transgenic N. benthamiana plants and decreased the xylem size and vessel area. Furthermore, the NtWAT1L expression level decreased significantly following the MG132 treatment. These results provide further evidence that PbRNF217 likely regulates plant height and xylem development via the ubiquitination of PbNAC71.
In conclusion, we isolated a dwarfism-related gene (PbNAC71) from Z/Q plants. The encoded protein downregulated PbWAT1 expression by binding directly to SNBEs, thereby regulating xylem and vessel development and inducing dwarfism (Fig. 8A). This process was regulated by the E3 ubiquitin ligase PbRNF217. More specifically, PbRNF217 modulated the plant height and xylem development through the ubiquitination of PbNAC71 (Fig. 8B). This study considerably advances our understanding of the dwarfism of woody plants, which may enhance the breeding of ideal dwarf pear varieties.
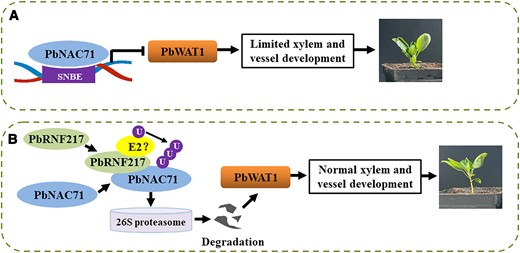
Proposed model for the regulatory effects of PbRNF217–PbNAC71–PbWAT1 on pear dwarfism. A) PbNAC71 inhibits xylem and vessel development by downregulating PbWAT1 expression. SNBE, secondary wall NAC-binding elements. B) PbRNF217 regulates plant height and xylem development via the ubiquitination of PbNAC71. U, ubiquitin. Pointed arrows indicate positive regulation. Flat head arrow indicates negative regulation.
Materials and methods
Plant materials and growth conditions
Five-year-old pear (Pyrus spp.) trees derived from the grafting of “Zaosu” pear scions on “Hardy”/“Yunnan” quince (C. oblonga Mill.) (with “Hardy” serving as the interstock) and Pyrus betulaefolia (“Duli”) were cultivated in an orchard in Pucheng county, Shaanxi Province, China. Conventional orchard management practices were applied. The annual shoots were collected at 10, 20, and 30 DAFB, immediately frozen in liquid nitrogen, and stored at −80 °C. N. benthamiana plants were grown in an incubator set at 22 °C, with a 16-h light/8-h dark cycle. The OHF-333 tissue culture seedlings and “Duli” seedlings were used for the gene transformation experiments. The OHF-333 tissue culture seedlings were grown at 25 °C in MS medium supplemented with 0.8 mg/L 6-BA and 0.3 mg/L IBA.
Total RNA extraction and RT-qPCR
Total RNA was extracted from the annual shoots, N. benthamiana leaves, and transgenic OHF-333 and N. benthamiana plants using the RNAprep Pure Plant Kit (Tiangen, Beijing, China). The RNA was reverse transcribed to cDNA as described by Cong et al. (2021). The RT-qPCR analysis was performed using the Life Technologies system (ABI, MA, USA) and 2× SYBR Green Pro Taq HS Premix II (Accurate Biotechnology, Hunan, China), with three biological replicates and three technical replicates. The RT-qPCR primers are listed in Supplementary Table S2. Relative transcription levels were calculated according to the 2−ΔΔCt method (Schmittgen and Livak 2008).
Phytohormone analysis
Approximately 0.2 g samples were ground in a precooled mortar containing 4 mL extraction buffer (ethyl acetate). After shaking the extract at 4 °C for 30 min, the sample was centrifuged at 18,000×g for 5 min. The supernatant was collected and dried using nitrogen gas. The dried material was dissolved in 150 µL methanol and filtered through a 0.22-µm membrane. The GA3, TZ, IAA, and ABA contents were determined using the TripleTOF 5600 + HPLC-MS/MS system (AB SCIEX, MA, USA).
RNA-sequencing analysis
For each sample, 1 μg RNA was used for the RNA-sequencing analysis, which was completed using the Illumina HiSeq instrument. The clustering of index-coded samples was performed using the cBot Cluster Generation System and the TruSeq PE Cluster Kit v3-cBot-HS (Illumina, CA, USA) according to the manufacturer’s instructions. After generating clusters, libraries were constructed and sequenced on the Illumina HiSeq platform to generate 125-bp/150-bp paired-end reads. A false discovery rate <0.001 and an absolute |log2 ratio| > 1 were used as the thresholds for identifying significant DEGs. Three independent biological replicates were sequenced and analyzed.
Phylogenetic analysis
The MEGA X software was used to analyze protein sequences and construct a phylogenetic tree according to the maximum likelihood method, with 1,000 bootstrap replicates.
Transformation of pear and N. benthamiana plants
The PbNAC71 CDS was cloned into the PK7-203 vector. The PbRNF217 CDS was incorporated into PK7-203-35S-PbNAC71 to generate the co-overexpression construct. The recombinant plasmids were inserted into Agrobacterium tumefaciens strain EHA105 for the transformation of OHF-333 and N. benthamiana plants or into Agrobacterium rhizogenes strain K599 for the transformation of roots. Details regarding the transformation were previously described by Yang et al. (2017) and Xiao et al. (2022a).
Histological analysis
The OHF-333 and N. benthamiana stem bases were fixed in a formaldehyde–acetic acid–alcohol solution. The samples were embedded in paraffin, dried, and stained with safranin and fast green. The BX63 fluorescence microscope (Olympus, Tokyo, Japan) was used for the histological examination. The xylem size and vessel area were measured for at least three sections using the Image J software.
Y1H assay
The 60-bp PbWAT1 promoter fragments were cloned into the p53-AbAi vector. The PbNAC71 CDS was cloned into the pGADT7 vector. The p p53-AbAi-bait vectors were linearized prior to the transformation of Y1H Gold cells. The integration of the sequences into the genome of Y1HGold cells was confirmed by performing a colony PCR analysis (Matchmaker Insert Check PCR Mix 1; Clontech, CA, USA). After determining the minimal inhibitory concentration of aureobasidin A (AbA) for the bait–reporter yeast strains, the AD-prey vectors were inserted into the bait yeast (Saccharomyces cerevisiae) strains, which were then selected on SD−Leu/AbA medium in plates. The empty vector pGADT7 was used as a negative control. Each experiment was repeated three times.
Dual-luciferase assay
The 1,500-bp PbWAT1 promoter sequence was cloned into the pGreenII 0800-LUC vector, whereas the PbNAC71 CDS was cloned into the pGreenII 0029 62-SK binary vector. The recombinant plasmids were inserted into A. tumefaciens strain GV3101 cells carrying the pSoup vector. The dual-luciferase assay was performed as described by Cong et al. (2021). The firefly luciferase (Luc) to Renilla luciferase (Ren) activity ratios were analyzed using the Dual-Luciferase Reporter Assay System (Promega, WI, USA) and the Infinite M200pro Full-Wavelength Multifunctional Enzyme Labeling Instrument (Tecan, ZH, CH). The analysis was completed using three biological replicates. Each experiment was repeated three times.
ChIP-qPCR analysis
The PbNAC71 CDS was cloned into the pMDC83 vector. “Zaosu” pear tissue culture plantlets were transformed with the recombinant plasmid. The ChIP-qPCR assay was completed as described by Ma et al. (2022), with a slight modification. The amount of immunoprecipitated chromatin was quantified by qPCR. The primers used are listed in Supplementary Table S2. Each experiment was repeated three times.
EMSA assay
PbNAC71-GST fusion proteins were obtained using GST Resin and Ni-NTA His Bind Resin, respectively (7sea biotech, Shanghai, China). The EMSA was conducted using the LightShift Chemiluminescent EMSA Kit (Thermo Scientific, USA), with EMSA reactions completed as described by Xiao et al. (2022a, 2022b). The recombinant proteins were incubated with the probes labeled with biotin at the 5′-end or with the unlabeled competitors. The probes are listed in Supplementary Table S2. Each experiment was repeated three times.
Y2H assay
The full-length and truncated PbNAC71 CDSs were cloned into the pGBKT7 vector to screen for self-activation. Because the truncated PbNAC71 CDS (encoding 266 aa) lacked self-activation, it was used to screen the pear cDNA library. The truncated PbNAC71 CDS and the full-length PbRNF217 CDS were cloned into the pGBKT7 and pGADT7 vectors, respectively. The recombinant plasmids were used for the co-transformation of Y2HGold cells, which were transferred to medium (−Trp/−Leu/−His/−Ade) to screen for protein–protein interactions. The primers used are listed in Supplementary Table S2. Each experiment was repeated three times.
BiFC assay
The PbNAC71 and PbRNF217 CDSs were inserted into the pSPYCE-35S and pSPYNE-35S vectors, respectively. The recombinant plasmids were inserted into A. tumefaciens GV3101 cells, which were then injected into N. benthamiana leaves. Fluorescence was detected using the TCS-SP8 SR laser scanning confocal microscope (Leica, Wetzlar, Germany). The YFP fluorescence was excited at 488 nm and detected between 520 and 540 nm. The primers are listed in Supplementary Table S2. Each experiment was repeated three times.
Pull-down assay
The PbNAC71 and PbRNF217 CDSs were cloned into the pGEX-4T-1 and pET-32a(+) vectors, respectively. The recombinant plasmids were inserted into Escherichia coli BL21 (DE3) cells for the expression of proteins in a prokaryotic system. The purified PbRNF217-His protein was immobilized to anti-His magnetic beads and then the PbNAC71-GST protein was added. The samples were rotated for 2 h at 4 °C. After the washing and elution steps, the samples were analyzed in a western blot involving anti-His or anti-GST antibodies.
MG132-treated N. benthamiana
Healthy and uniformly growing 2-wk-old transgenic N. benthamiana plants were used as experimental materials. The N. benthamiana plants were cultured hydroponically in Hoagland nutrient solution. The experimental group was treated with 5 μM MG132 (Abmole Bioscience, Houston, TX, USA), whereas the control group was treated with DMSO (Abmole Bioscience).
Protein stability analysis
The PbNAC71 CDS was cloned into the pGEX-4T-1 vector and then expressed in E. coli BL21 (DE3) cells. The recombinant PbNAC71-GST protein was purified using GST Resin (7Sea Biotech, Shanghai, China). Proteins were extracted from wild-type or PbRNF217-OE P. betulaefolia roots and incubated with PbNAC71-GST as well as 50 μM MG132 (Abmole Bioscience) or DMSO (Abmole Bioscience) for 0, 0.5, and 1.5 h at 22 °C. The samples were analyzed in western blots involving anti-GST or antiactin antibodies.
Ubiquitination assay
The PbNAC71 and PbRNF217 CDSs were cloned into the PK7-203 or pMDC83 vectors. The resulting recombinant plasmids for the production of MYC-PbNAC71 and PbRNF217-GFP were inserted into A. tumefaciens GV3101 cells, which were then injected into N. benthamiana leaves. The Pierce Crosslink Magnetic IP/Co-IP Kit (Thermo Scientific, MA, USA) was used for the immunoprecipitation of proteins, which were detected using anti-MYC and antiubiquitin antibodies.
Statistical analysis
All data were analyzed using the SPSS software (SPSS, Inc., Chicago, IL, USA). A one-way ANOVA or Student’s t-test were used to evaluate the significance of the differences (P < 0.05).
Accession numbers
Sequence data from this article can be found in the National Center for Biotechnology Information (NCBI) database (https://www.ncbi.nlm.nih.gov/): PbNAC71 (LOC103932913); PbWAT1 (LOC103928336); PbWAT1-related (LOC103965756) and _PbRNF217 (LOC103950223).
Acknowledgments
We thank the Horticulture Science Research Center at College of Horticulture, NWAFU for their technical support in this work. We thank Liwen Bianji (Edanz) (www.liwenbianji.cn) for editing the English text of a draft of this manuscript.
Author contributions
L.-F. X., L.C., and F.-W. M. conceived and designed the experiments. L.C., Y.-K. S., X.-Y. G., X.-F. Z., and H.-Q. Z. performed the research. L.C., F.-L. Z., H.-J. Z., C.-Q. Y., and B.-Q. M. analyzed the data, and L.C., R.Z., and Z.-G. W. wrote the paper. L.C., L.-F. X., and Z.-G. W. revised the paper.
Supplementary data
The following materials are available in the online version of this article.
Supplementary Figure S1. The hormone analysis results of annual shoots.
Supplementary Figure S2. Partial bioinformatics analysis of PbNAC71 and PbRNF217.
Supplementary Figure S3. The expression analyses in transgenic N. benthamiana.
Supplementary Figure S4. The relative expression level of PbNAC71 in transgenic OHF-333.
Supplementary Figure S5. PbWAT1 regulated the vessel development.
Supplementary Figure S6. The bioinformatics analysis and mutant phenotype of PbWAT1-related.
Supplementary Figure S7. The multiple amino acid sequence alignment of PbRNF217.
Supplementary Figure S8. Identification of single- and double-transgenic “Duli roots.
Supplementary Table S1. The list of DEGs.
Supplementary Table S2. List of primers used in this study.
Funding
This research was funded by the National Key Research and Development Program of China (No. 2019YFD1000100).
Data availability
The data that support the findings of this study are available from the corresponding author upon reasonable request.
Dive Curated Terms
The following phenotypic, genotypic, and functional terms are of significance to the work described in this paper:
References
Author notes
The author responsible for distribution of materials integral to the findings presented in this article in accordance with the policy described in the Instructions for Authors (https://dbpia.nl.go.kr/plphys/pages/General-Instructions) is: Ling-fei Xu ([email protected]).
Conflict of interest statement. The authors declare no conflict of interest.