-
PDF
- Split View
-
Views
-
Cite
Cite
Degao Liu, Erik A Myers, Shuya Xuan, Lynn E Prichard, Lilee I Donahue, Evan E Ellison, Colby G Starker, Daniel F Voytas, Heritable, multinucleotide deletions in plants using viral delivery of a repair exonuclease and guide RNAs, Plant Physiology, Volume 194, Issue 4, April 2024, Pages 2229–2239, https://doi.org/10.1093/plphys/kiae015
- Share Icon Share
Abstract
CRISPR/Cas9-mediated mutagenesis typically results in short insertion/deletion mutations, which are often too small to disrupt the function of cis-acting regulatory elements. Here, we describe a highly efficient in planta gene editing approach called VirTREX2-HLDel that achieves heritable multinucleotide deletions in both protein-coding genes and noncoding DNA regulatory elements. VirTREX2-HLDel uses RNA viruses to deliver both the 3 prime repair exonuclease 2 (TREX2) and single-guide RNAs. Our method enables recovery of multiplexed heritable deletions and increases the heritable gene editing frequency at poorly edited sites. We identified functional conservation and divergence of MICRORNA164 (miR164) in Nicotiana benthamiana and tomato (Solanum lycopersicum) using VirTREX2-HLDel and observed previously uncharacterized phenotypes in plants with large deletions at this locus. Our viral delivery method reduces the need for tissue culture and will accelerate the understanding of protein-coding and regulatory regions in plants.
Introduction
Noncoding DNA sequences, including cis-regulatory elements, play important roles in controlling plant growth and development at both the transcriptional and posttranscriptional levels (Michael and Jackson 2013; Rodríguez-Leal et al. 2017; Schmitz et al. 2022). Gene editing provides 1 approach to dissect the function of cis-regulatory elements; however, the use of CRISPR/Cas9 for this purpose has been technically challenging. CRISPR/Cas9 typically makes small insertion/deletion (indel) mutations, typically 1 bp insertions or ∼1 to 3 bp deletions, and such small sequence changes may not be large enough, for example, to abrogate binding of a transcription factor to a cis-regulatory element (Feng et al. 2013; Shan et al. 2013; Wang et al. 2020). Several strategies have been used to create larger deletions, including simultaneous expression of multiple single-guide RNAs (sgRNAs) (Rodríguez-Leal et al. 2017) and use of the 3 prime repair exonuclease 2 (TREX2)-Cas9 (Weiss et al. 2020), APOBEC-Cas9 (Wang et al. 2020), and T5 exonuclease (T5exo)-Cas (Zhang et al. 2020). All of these approaches have met with some success; however, all require the use of tissue culture to deliver these reagents to plant cells, and regenerating gene-edited plants through tissue culture is one of the biggest bottlenecks in the use of plant gene editing to create novel genetic variation.
RNA viruses have been employed to deliver zinc finger nucleases to tobacco (Nicotiana tabacum) and petunia (Petunia hybrida), resulting in targeted genome modifications of somatic cells. The successful recovery of mutants depended on the ability to regenerate plants from the edited somatic cells (Marton et al. 2010). To minimize the need for tissue culture, RNA viruses are increasingly used to deliver sgRNAs to plants through infection to create a variety of targeted modifications, including heritable short indel mutations, base edits, or changes in DNA demethylation (Ali et al. 2015; Ellison et al. 2020; Ghoshal et al. 2020; Li et al. 2021; Liu et al. 2022; Nagalakshmi et al. 2022; Wang et al. 2022). Because of their limited cargo capacity, viruses are typically used to deliver sgRNAs to transgenic plants that express Cas9 or a Cas9-derived gene editing reagent. Large deletions can be created with RNA virus vectors; for example, we used tobacco rattle virus (TRV) to deliver 3 sgRNAs targeting PHYTOENE DESATURASE (PDS) and 2 sites in AGAMOUS (AG) (Ellison et al. 2020). Heritable large deletions (i.e. a 4.05 kb deletion between the 2 AG sgRNA target sites) were recovered in 2/30 progeny (Ellison et al. 2020). In a separate study, 5 sgRNAs targeting the promoter of the Q gene of wheat (Triticum aestivum) were delivered using a barley stripe mosaic virus (BSMV) vector (Wang et al. 2022). Heritable mutations at 2 of the 5 target sites were recovered in 13/238 M1 plants. The low frequency in creating large deletions could be because (i) the use of paired or multiple sgRNAs requires efficient, simultaneous cleavage of the target sites and (ii) simultaneous expression of multiple sgRNAs in the same plant cell using a single viral vector may be not efficient. In addition, expression of paired sgRNAs may result in large chromosomal aberrations such as inversions or duplications (Zhang et al. 2017; Lynagh et al. 2018; Schwartz et al. 2020). Clearly, there is a need for novel, alternative approaches to efficiently create gene-edited plants with large deletions and to reduce the need for tissue culture.
Previous work has shown that the TREX2 can create multinucleotide deletions when codelivered with a DNA breaking reagent (Čermák et al. 2017; Weiss et al. 2020). Here, we developed an in planta gene editing method, called VirTREX2-HLDel, to efficiently create gene-edited plants with large deletions by using viral delivery of the TREX2 and esgRNAs to Cas9 transgenic plants.
Results and discussion
Heritable multinucleotide deletions in protein-coding gene using VirTREX2-HLDel
We hypothesized that heritable large deletions could be generated if RNA viral vectors were used to deliver both TREX2 and sgRNAs to Cas9 transgenic plants. To test this hypothesis, we designed 2 systems: VirTREX2-HLDel-1 and VirTREX2-HLDel-2 (Fig. 1). For VirTREX2-HLDel-1, we cloned TREX2 and an enhanced sgRNA (esgRNA) targeting 2 homologs of PDS of Nicotiana benthamiana into our TRV vector. The esgRNA included sequences from tRNA isoleucine (tRNAIleu) to promote cell-to-cell mobility (Fig. 1B). For VirTREX2-HLDel-2, 2 copies of the com RNA aptamer were cloned to the 3′ end of the esgRNA. The engineered com-esgRNA scaffold should recruit to the Cas9 target site Com fused with TREX2. After cleavage by Cas9, TREX2 should digest the 3′ ends of the DNA, such that larger deletions would be created after repair by nonhomologous end joining (NHEJ) or microhomology-mediated end joining (MMEJ).
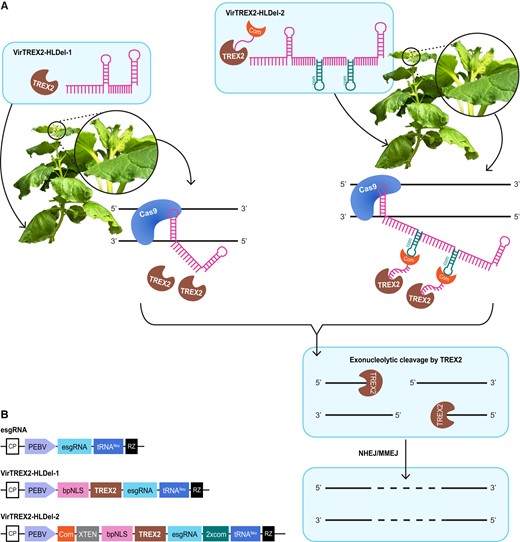
Overview of methods to create heritable multinucleotide deletions. A) In the VirTREX2-HLDel-1 approach, TRV vectors deliver through infection the TREX2, esgRNA, and RNA mobility enhancer tRNAIleu. In the VirTREX2-HLDel-2 approach, 2 copies of the RNA aptamer com are cloned to the 3′ end of the esgRNA. The engineered com-crRNA scaffold can recruit its cognate RNA-binding protein Com fused with TREX2 to Cas9 target sites. The Com-com recruiting system and tRNAIleu are cloned into TRV vectors. The VirTREX2-HLDel is delivered to Cas9 transgenic plants. As TRV moves systemically throughout the plant, Cas9/esgRNA creates a DSB to target site. The end-processing enzyme TREX2 can resect the DSB and create multinucleotide deletions. DSBs with deletions are repaired through the NHEJ or MMEJ pathways. The resulting multinucleotide deletions are transmitted to the next generation. B) Schematic of TRV vectors expressing TREX2 and an esgRNA fused to tRNAIleu. CP, coat protein; PEBV, pea early browning virus subgenomic promoter; bpNLS, bipartite NLS; XTEN, a 16-aa linker; RZ, terminating ribozyme.
To test our approach, the VirTREX2-HLDel vectors or the control vector without TREX2 and just the esgRNA were delivered to Cas9 transgenic N. benthamiana plants via the Agrobacterium tumefaciens leaf infiltration method. PDS is required for carotenoid biosynthesis, and loss-of-function of both PDS homologs results in an albino phenotype (Ellison et al. 2020). Eighteen days postinfection, bleached spots were observed in newly emerging leaves in plants infected by VirTREX2-HLDel vectors or the control vector without TREX2 (just the esgRNA) (Supplementary Fig. S1A). Total indel frequencies of leaves systemically infected by the control esgRNA vector, VirTREX2-HLDel-1, or VirTREX2-HLDel-2 were 95.9%, 96.5%, and 91.9%, respectively (Supplementary Fig. S1B). The control vector created small indels (1 bp insertion, 1 to 5 bp deletions) at a frequency of 77.2%; multinucleotide deletions (≥11 bp) were created at a frequency of 4.3%. The frequency of small indels (1 bp insertion, 1 to 5 bp deletions) created by VirTREX2-HLDel-1 and VirTREX2-HLDel-2 were 43.1% and 37.0%, respectively. VirTREX2-HLDel-1 and VirTREX2-HLDel-2 created multinucleotide deletions (≥11 bp) at frequencies of 35.7% and 39.0%, respectively, considerably higher than the 4.3% observed when the esgRNA was expressed alone.
As the infected plants matured, the pds knockout phenotype became prominent in the upper portion of the plants, sometimes resulting in a completely albino phenotype (Supplementary Fig. S1C). We next determined whether the multinucleotide deletions observed in the systemically infected leaves were heritable. We previously found that high-frequency heritable mutagenesis was particularly pronounced in seed pods that developed latest on the inflorescence (8th to 10th seed pod, counting from the base of the inflorescence) (Ellison et al. 2020). In progeny derived from the 8th to 10th seed pods, fully bleached seedlings due to inactivating biallelic mutations were recovered from plants infected with the esgRNA control vector, VirTREX2-HLDel-1, or VirTREX2-HLDel-2 (Fig. 2A). Biallelic mutants were recovered from the plants infected with the esgRNA, VirTREX2-HLDel-1, and VirTREX2-HLDel-2 at frequencies of 98.3%, 100%, and 62.2%, respectively (Fig. 2B, Supplementary Table S1). Monoallelic mutants were recovered at frequencies of 1.7%, 0, and 35.6%, respectively. The esgRNA, VirTREX2-HLDel-1, and VirTREX2-HLDel-2 created progeny with insertions (both alleles are insertion or 1 allele is an insertion and the other allele is wild-type) at frequencies of 58.3%, 1.3%, and 0%, respectively (Fig. 2C, Supplementary Table S1). The size of all the deletions created by esgRNA were 1 to 10 base pair (bp), whereas the VirTREX2-HLDel-1 and VirTREX2-HLDel-2 produced significantly larger deletions (the size of deletions were between 11 and 20 bp in at least 1 allele) at frequencies of 57.3% and 31.1%, respectively. We were often able to recover progeny with 21 to 40 bp deletions from parental plants infected with VirTREX2-HLDel-1 and VirTREX2-HLDel-2 (Fig. 2C, Supplementary Table S1). These data demonstrate that heritable multinucleotide deletions could be generated at high frequencies using viral delivery of TREX2 and esgRNAs. Expression of only TREX2 and the esgRNA is sufficient for deleting multiple nucleotides in the genome; tethering TREX2 to the target site does not provide further benefit.
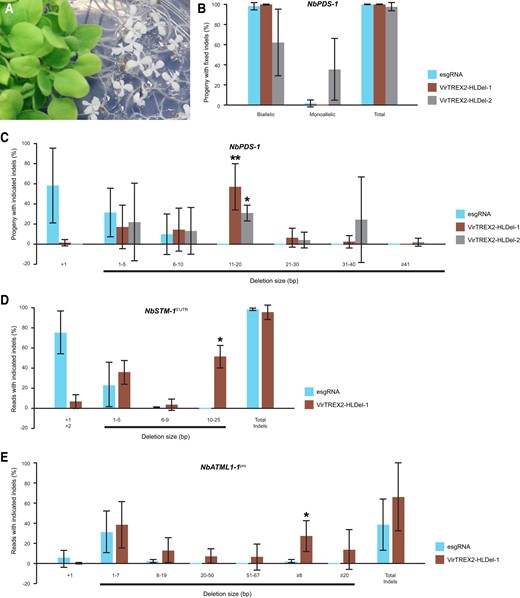
Heritable multinucleotide deletions in protein-coding genes and cis-regulatory elements in N.benthamiana. A) Albino phenotypes of the NbPDS knockout progeny from the infected parental plant. B) The frequency of heritable gene editing at NbPDS-1 in M1 seedlings. C) The frequency of heritable multinucleotide deletions at NbPDS-1 in M1 seedlings. NbPDS-1, Niben101Scf14708g00023. For B) and C), the 8th to 10th seed pods were harvested. Fifteen progenies (i.e. 5 progeny per seed pod) per parental line were genotyped. The mutation type “Deletion” describes progeny with the indicated deletion type in at least 1 allele. The mutation type “Insertion” describes progeny with insertions in both alleles or an insertion in 1 allele, and the other allele is wild-type. Data are presented as the mean ± Sd (n = 4, 5, and 3 parental lines for esgRNA, VirTREX2-HLDel-1, and VirTREX2-HLDel-2, respectively). D) The frequency of heritable gene editing at the core K-box of SHOOT MERISTEMLESS (NbSTM-15′UTR) target site in M1 seedlings. NbSTM-1, Niben101Scf02145g02001.1. Data are presented as the mean ± Sd (n = 3 parental plants). The next-generation sequencing read filtering threshold of NbSTM-15′UTR is ≥0.5%. After filtering, the frequencies of remaining reads were normalized to sum to 100%. E) The frequency of heritable gene editing at the L1-box of the putative ortholog of ATML1 (called NbATML1-1pro) target site in M1 seedlings. Data are presented as the mean ± Sd (n = 3 parental plants for the control vector esgRNA; n = 4 parental plants for VirTREX2-HLDel-1). The next-generation sequencing read filtering threshold of NbATML1-1pro is ≥0.50%. After filtering, the frequencies of the remaining reads were normalized to sum to 100%. For each replicate, a total of 60 seedlings from the 8th to 10th seed pods (counting from the base of the parent plant) of the parental plant were pooled and sequenced by next-generation sequencing. Statistical significance in this figure was determined using the 2-tailed t test in Microsoft Excel. *, **, and *** indicate significant differences from that of esgRNA at P < 0.05, P < 0.01, and P < 0.001, respectively.
Heritable multinucleotide deletions in cis-regulatory elements
After demonstrating our mutagenesis approach in a protein-coding gene, we next tested whether the VirTREX2-HLDel-1 could create heritable large deletions in 5′ cis-regulatory elements. We targeted 2 conserved cis-elements in N. benthamiana, the core K-box of SHOOT MERISTEMLESS (NbSTM5′UTR) (Uchida et al. 2007), and the L1-box of the putative ortholog of Arabidopsis (Arabidopsis thaliana) MERISTEM LAYER 1 (ATML1) (NbATML1-1pro) (Burgess and Freeling 2014) (Supplementary Fig. S2). The VirTREX2-HLDel-1 vector and the control vector expressing the esgRNA targeted to NbSTM5′UTR or NbATML1-1pro were delivered to Cas9-expressing N. benthamiana plants. No statistically significant difference in the frequencies of heritable indels at NbSTM-15′UTR was observed between the VirTREX2-HLDel-1 (95.9% ± 7.0%) and the control vector expressing just the esgRNA (98.9% ± 1.0%) (Fig. 2D, Supplementary Table S2). All the deletions created by the esgRNA at NbSTM-15′UTR were 1 to 9 bp, whereas the VirTREX2-HLDel-1 produced deletions between 10 and 25 bp at a frequency of 51.2% (Fig. 2D, Supplementary Table S2). Also, we obtained M1 progeny with multinucleotide deletions at both NbSTM-15′UTR (−16 bp/−16 bp) and NbSTM-25′UTR (−16 bp/−16 bp); these deletions were stable and transmitted to the M2 generation (Supplementary Fig. S3).
VirTREX2-HLDel-1 and the control vector produced heritable indels at NbATML1-1pro at frequencies of 66.4% and 38.9%, respectively (Fig. 2E, Supplementary Table S3). Deletions ≥8 bp were created by VirTREX2-HLDel-1 at a frequency of 27.4% compared with 2.5% for the control vector with just the esgRNA. The size of all the deletions created by the esgRNA at NbATML1-1pro was 1 to 19 bp, whereas VirTREX2-HLDel-1 produced deletions between 20 and 67 bp at a frequency of 14.1% (Fig. 2E, Supplementary Table S3). These results demonstrate that VirTREX2 can produce larger deletions in cis-regulatory elements compared with vectors expressing just the esgRNA.
Functional conservation and divergence of MIR164 in N. benthamiana and tomato (Solanum lycopersicum) as revealed by VirTREX2-HLDel
Noncoding RNAs (e.g. microRNAs and miRNAs) are crucial regulators of plant growth and development (Liu et al. 2017). To test whether VirTREX2-HLDel can be used for in situ analyses of noncoding RNAs, we designed an esgRNA targeting both NbMIR164e and NbMIR164h in N. benthamiana (Fig. 3A). In A. thaliana, miR164 regulates organ number and the formation of organ boundaries (Laufs et al. 2004; Sieber et al. 2007). The A. thaliana mir164abc triple mutant, which carries loss-of-function mutations in all the miR164 family members, exhibited slightly more serrated rosette leaves compared with wild-type plants and an increased number of petals, disrupted flower phyllotaxis, and a severe reduction in fertility (Sieber et al. 2007). A strawberry (Fragaria vesca) fvemir164a mutant showed wrinkled leaves with deep serrations and serrated petals (Zheng et al. 2019). A tomato slmir164b mutant, which harbors an intact sly-miR164 sequence but a mutated miRNA precursor (pre-miRNA), exhibited smaller leaves with fewer lobes and misshapen, infertile flowers with fewer petals (Gupta et al. 2021). To the best of our knowledge, loss-of-function analysis of MIR164 has not been performed in other plant species.
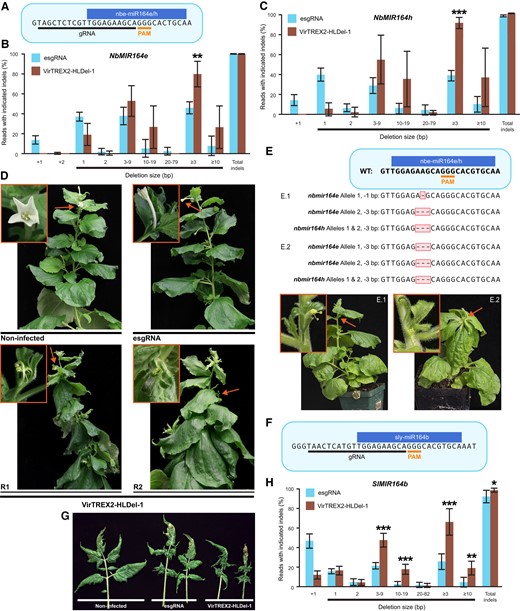
Functional conservation and divergence of MIR164 in N.benthamiana and tomato as revealed by VirTREX2-HLDel. A) The CRISPR/Cas9 target site in NbMIR164e and NbMIR164h of N. benthamiana. PAM, protospacer adjacent motif. B) to C) The NbmiR164e/h editing frequency in systemic leaf 16th after 78 d postinfiltration. Data are presented as the mean ± Sd (n = 4). D)NbMIR164e and NbMIR164h knockout phenotypes in the infected plants. Photos were taken ∼2 mo postinfiltration. esgRNA, control vector without TREX2; VirTREX2-HLDel-1, vector with TREX2. R1 and R2 are 2 replicates infected by VirTREX2-HLDel-1. E) Phenotypes and genotypes of the progeny from the parental plant infected by VirTREX2-HLDel-1. F) The CRISPR/Cas9 target site in SlMIR164b of tomato. G)SlMIR164b knockout phenotypes in the infected plants. H) Multinucleotide deletions in the systemically infected leaves in tomato. Data are presented as the mean ± Sd (n = 6). The next-generation sequencing read filtering threshold of all the target genes in this figure is 1%. After filtering, the frequencies of remaining reads were normalized to sum to 100%. Statistical significance in this figure was determined using the 2-tailed t test in Microsoft Excel. *, **, and *** indicate significant difference from that of esgRNA at P < 0.05, P < 0.01, and P < 0.001, respectively.
The VirTREX2-HLDel-NbmiR164e/h esgRNA vectors or the control vector NbmiR164e/h esgRNA was delivered to Cas9 transgenic N. benthamiana plants via A. tumefaciens leaf infiltration. Different from the phenotypes observed in the A. thaliana mir164abc mutant, the strawberry fvemir164a mutant, and the tomato slmir164b mutant, we observed sessile leaves, an abnormal leaf arrangement (e.g. opposite or whorled), and misshapen infertile flowers without petals in the upper systemic tissues of N. benthamiana plants infected by VirTREX2-HLDel-NbmiR164e/h; such phenotypes were not observed in the plants infected by NbmiR164e/h esgRNA or VirTREX2-HLDel-NbPDS esgRNA (Fig. 3D and Supplementary Fig. S1C). The sessile leaves, abnormal leaf arrangement, and leaf angle resulted in a very bushy architecture. None of the plants exhibited serration of the leaf margin and wrinkled leaves. We analyzed the NbmiR164e/h editing frequency using NGS in systemic leaf 16 at 78 d postinfiltration. Total indel frequency in plants infected by VirTREX2-HLDel and the NbmiR164e/h esgRNA was ∼100% at both NbMIR164e and NbMIR164h (Figs. 3, B and C, Supplementary Tables S4 and S5). For the NbMIR164e site, the vector delivering the esgRNA alone produced 1 to 2 bp insertions and 1 to 2 bp deletions at a frequency of 53.8%. In contrast, the VirTREX2-HLDel created 1 to 2 bp deletions at a frequency of 19.9% and ≥3 bp deletions at a frequency of 80.1% which is significantly higher than the 46.2% observed for the esgRNA alone. VirTREX2-HLDel frequently created ≥10 bp deletions as well.
For the NbMIR164h site, the NbmiR164e/h esgRNA vector produced 1 bp insertion and 1 to 2 bp deletions at a frequency of 60.5%, whereas VirTREX2-HLDel created 1 to 2 bp deletions at a frequency of 7.7%. The frequency of deletions ≥3 bp created by VirTREX2-HLDel (92.3%) is significantly higher than that created by NbmiR164e/h esgRNA (39.1%) (Fig. 3C, Supplementary Table S5). VirTREX2-HLDel also frequently created ≥10 bp deletions. Since the plants infected by VirTREX2-HLDel developed misshapen infertile flowers, we were only able to collect the upper seed pod from 1 parental line. We found that the progeny carrying larger deletions at NbmiR164e (−3 bp/−3 bp) and NbmiR164h (−3 bp/−3 bp) exhibited sessile leaves, abnormal leaf arrangement (e.g. opposite or whorled), and misshapen infertile flowers without petals, whereas the phenotype was not observed in the progeny carrying −1 bp/−3 bp mutations at NbmiR164e and −3 bp/−3 bp mutations at NbmiR164h (Fig. 3E). Our data indicated that the abnormal phenotypes observed in both the parental plants infected by VirTREX2-HLDel and their progeny were due to multinucleotide deletions (≥3 bp) in both NbmiR164e/h. Further, the large deletions created by VirTREX2-HLDel enable functional analysis of miRNAs in as early as the M0 generation.
To determine whether VirTREX2-HLDel can be used for functional analysis of miRNAs in planta in the M0 generation in another species, we targeted SlMIR164b in tomato (Fig. 3F). The mature sequences of sly-miR164 and nbe-miR164e/h are identical. The VirTREX2-HLDel-SlMIR164b esgRNA vector or the control vector SlMIR164b esgRNA was delivered to Cas9 transgenic tomato plants via A. tumefaciens cotyledon and leaf infiltration. In the upper systemic tissues of tomato plants, VirTREX2-HLDel generated more wrinkled leaves with deep serrations than the SlMIR164b esgRNA (Fig. 3G). The abnormal leaf phenotype is different from the phenotype observed in slmir164b mutant, namely leaflets with fewer lobes (Gupta et al. 2021). The predominant mutations created by SlMIR164b esgRNA were 1 bp insertions and 1 to 2 bp deletions at a frequency of 66.7%, whereas VirTREX2-HLDel-SlMIR164b esgRNA created ≥3 bp deletions at a frequency of 66.3% (Fig. 3H, Supplementary Table S6). The frequency of multinucleotide deletions (≥10 bp) created by VirTREX2-HLDel-SlMIR164b esgRNA is also significantly higher than that created by the SlMIR164b esgRNA. Our data indicate that the abnormal leaf phenotypes in VirTREX2-HLDel were due to large deletions in SlMIR164b. Based on these collective data, VirTREX2-HLDel revealed the functional conservation and divergence of miR164 in different plant species.
Multiplexed heritable multinucleotide deletions using VirTREX2-HLDel
To test whether VirTREX2-HLDel could increase the frequency of multiplexed heritable edits at poorly edited sites and could create multiplexed heritable large deletions, we targeted the poorly edited site NbATML1-1pro (Fig. 2) and the promoter of the other ATML1 homolog NbATML1-2 (NbATML1-2pro) (Supplementary Fig. S2). The 2 esgRNAs were arranged in tandem (Fig. 4A). The VirTREX2-HLDel esgRNA vector or the control vector esgRNA was delivered into Cas9 transgenic plants. The 8th to 10th seed pods were harvested. The frequencies of total heritable editing and biallelic mutants in NbATML1-1pro created by VirTREX2-HLDel-1 (at frequencies of 95.5% and 74.7%, respectively) are significantly higher than those created by the esgRNA (at frequencies of 17.5% and 1.8%, respectively) (Fig. 4B, Supplementary Table S7). The frequency of heritable multinucleotide deletions (the size of deletion ≥3 bp in at least 1 allele) from the plants infected by VirTREX2-HLDel-1 (at a frequency of 76.7%) was significantly higher than that by esgRNA (at a frequency of 10.4%) (Fig. 4C, Supplementary Table S7). We were able to recover the progeny with ≥10 bp deletions from the parental plants infected with VirTREX2-HLDel-1 at a frequency of 57.1% and from the plants infected by esgRNA at a frequency of 3.3% (Fig. 4C, Supplementary Table S7). Also, VirTREX2-HLDel-1 produced a significantly higher frequency of heritable editing (including both monoallelic mutants and biallelic mutants) at NbATML1-2pro at frequencies of 79.5% compared with esgRNA at frequencies of 29.9% (Fig. 4D, Supplementary Table S8). We were able to recover the progeny with ≥15 bp deletions at NbATML1-2pro from the parental plants infected with VirTREX2-HLDel-1 at a frequency of 40.1% and from the plants infected by esgRNA at a frequency of 1.5% (Fig. 4E, Supplementary Table S8). There is no progeny carrying ≥31 bp deletions at NbATML1-2pro recovered from the parental plants infected with esgRNA, whereas we frequently recover progeny with ≥31 bp deletions from the plants infected by VirTREX2-HLDel-1 (Fig. 4E, Supplementary Table S8). Furthermore, we found that VirTREX2-HLDel-1 produced a significantly higher frequency of heritable duplexed editing of NbATML1-1pro and NbATML1-2pro (including both monoallelic mutants and biallelic mutants) at a frequency of 75.0% compared with esgRNA at a frequency of 6.8% (Fig. 4F, Supplementary Table S9). We were able to recover the progeny with ≥10 bp deletions at both NbATML1-1pro and NbATML1-2pro from the parental plants infected with VirTREX2-HLDel-1 at a frequency of 24.7% and from the plants infected by esgRNA at a frequency of 1.5% (Fig. 4G, Supplementary Table S9). Thus, VirTREX2-HLDel increased multiplexed heritable editing frequency and frequently produced multiplexed, heritable multinucleotide deletions.
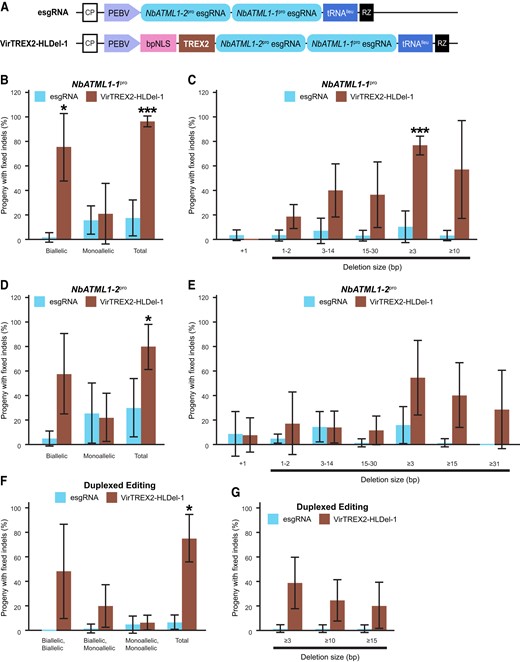
Multiplexed heritable multinucleotide deletions in N.benthamiana using VirTREX2-HLDel. A) Schematic representation of the TRV vector expressing TREX2 and esgRNAs fused to a tRNAIleu to promote RNA mobility. CP, coat protein; PEBV, pea early browning virus subgenomic promoter; bpNLS, bipartite NLS; RZ, terminating ribozyme; NbATML1-1pro, promoter of the putative ortholog of ATML1 in N. benthamiana. The gene ID is Niben101Scf00703g00003. The esgRNA targets the L1 cis-element of the promoter. NbATML1-2pro, promoter of the putative ortholog of ATML1 in N. benthamiana. The gene ID is Niben101Scf01158g03010. The esgRNA targets the L1 cis-element of the promoter. B), D), and F) The frequency of heritable gene editing in M1 seedlings. C), E), and G) The frequency of heritable multinucleotide deletions in M1 seedlings. Data are presented as the mean ± Sd (n = 4 parental plants for the control vector esgRNA; n = 3 parental plants for VirTREX2-HLDel-1). Statistical significance in this figure was determined using a 2-tailed t test in Microsoft Excel. *, **, and *** indicate significant difference from that of esgRNA at P < 0.05, P < 0.01 and P < 0.001, respectively.
In this study, we developed an in planta gene editing method to achieve highly efficient and heritable multinucleotide deletions in both a protein-coding region and noncoding DNA using viral delivery of TREX2 and esgRNAs. Accurate repair of Cas9-induced blunt-ended double-stranded breaks (DSBs) is a frequent outcome (Cisneros-Aguirre et al. 2022). In the case of 2 adjacent Cas9-induced DSBs, accurate NHEJ was observed from 0.00% to 94.05% (with a mean of 47.33%) across 70 target sites (Guo et al. 2018). The expression of TREX2 can stimulate mutagenic end processing prior to the rejoining of DSBs, thereby leading to an increased frequency of mutagenesis (Certo et al. 2012). In this study, we found that codelivery of TREX2 and an esgRNA using TRV increased heritable gene editing frequencies at poorly edited sites and allowed recovery of multiplexed heritable large deletions. As proof-of-concept, we revealed the functional conservation and divergence of the miR164 in different plant species. Our viral delivery method reduces the need for tissue culture—a current bottleneck in plant gene editing. Virus-based gene editing has been reported in N. benthamiana, A. thaliana, and wheat (Li et al. 2021; Wang et al. 2022). We anticipate that viral-induced heritable gene editing will be implemented in other plant species in the near future, thereby accelerating functional genomics research and making it possible to perform gene editing of genomic regulatory elements at scale.
Materials and methods
TRV vector construction
Golden Gate assembly was used to construct all TRV2 vectors using the TRV2 cloning backbone pEE083 (vector sequences and vector are available at Addgene) (Ellison et al. 2020). For the VirTREX2-HLDel-1-NbPDS esgRNA vector and VirTREX2-HLDel-2-NbPDS esgRNA vector, the sequences for bpNLS-TREX2-NbPDS esgRNA-tRNAIleu and Com-XTEN-bpNLS-TREX2-NbPDS esgRNA-2Xcom-tRNAIleu were chemically synthesized by Integrated DNA Technologies (Coralville, IA). For the TREX2-related vectors targeting other genes, PCR amplification was used to clone the sequences for the bpNLS-TREX2 and esgRNA-tRNAIleu.
TRV inoculation
TRV inoculation of N. benthamiana was performed as previously described (Ellison et al. 2020). For TRV inoculation of tomato (S. lycopersicum), the protocols for growth and preparation of A. tumefaciens strain GV3101 were similar to those used for N. benthamiana with some modifications. The A. tumefaciens cells were resuspended in infiltration buffer (10 mM MgCl2, 10 mM MES, pH 5.6) with 0.2 mM acetosyringone and placed at room temperature for 3 h. For the SlMIR164b experiment, the concentrations of TRV1 and TRV2 were adjusted to OD600 = 1.5. TRV1 and TRV2 were mixed in a 1:1 ratio and infiltrated into the cotyledons and 1st true leaf of tomato seedlings using a needleless syringe. The infected tomato plants were grown under a constant temperature ∼17 °C with a 16/8 h day/night cycle, a light intensity of 100 μmol m−2 s−1, and 50% humidity.
Analysis of somatic editing efficiency
Genomic DNA was extracted from systemically infected leaves and noninfected plants using either the DNeasy Plant Pro Kit (QIAGEN, Cat. No.: 69206) or the DNeasy Plant Mini Kit (QIAGEN, Cat. No. 69106). esgRNA target sites were amplified by PCR using gene-specific primers (Supplementary Table S10). The PCR products were submitted for Sanger sequencing (Eurofins Genomics LLC) or Amplicon-EZ (Azenta Life Sciences). For Sanger sequencing data analysis, the indel frequency was quantified using the ICE CRISPR Analysis Tool (Conant et al. 2022) or Deconvolution of Complex DNA Repair (DECODR) (Bloh et al. 2021). The Amplicon-EZ data was analyzed using CRISPResso2 (Clement et al. 2019).
Analysis of heritable editing efficiency
Progenies from infected lines were germinated on ½ MS medium or soil. DNA was extracted from individual seedlings, and esgRNA target sites were PCR-amplified and submitted for sequencing. Indel frequencies were quantified using the ICE CRISPR Analysis Tool (Conant et al. 2022) or DECODR (Bloh et al. 2021). Genotypes were defined as follows: indel frequencies between 0% and 15.0% wild-type; a single unique sequence modification between 35.1% and 65.0% (with a nonmodified sequence between 35.1% and 65.0%), monoallelic mutant; and a single unique sequence modification between 85.1% and 100.0% or 2 sequence modifications between 35.1% and 65.0% (with sum of the 2 sequence modifications between 85.1% and 100.0%), biallelic mutant. Amplicon-EZ data were analyzed using CRISPResso2 (Clement et al. 2019), and unspecific reads were filtered using gene-specific sequences. The reads with insertions or deletions were extracted using the threshold established with Amplicon-EZ reads of noninfected control plants.
Accession numbers
Sequence data from this article can be found under the following accession numbers: THREE PRIME REPAIR EXONUCLEASE 2 (TREX2) from Mus musculus: NCBI accession number, NP_036037.1; PHYTOENE DESATURASE-1 from N. benthamiana (NbPDS-1): Solanaceae Genomics Network database accession number, Niben101Scf14708g00023; SHOOT MERISTEMLESS-1 from N. benthamiana (NbSTM-1): Solanaceae Genomics Network database accession number, Niben101Scf02145g02001; putative ortholog of ATML1 in N. benthamiana (NbATML1-1): Solanaceae Genomics Network database accession number, Niben101Scf00703g00003; putative ortholog of ATML1 in N. benthamiana (NbATML1-2): Solanaceae Genomics Network database accession number, Niben101Scf01158g03010; NbMIR164e from N. benthamiana: N. benthamiana microRNA family database (https://sefapps02.qut.edu.au/benWeb/subpages/miRs6.php) accession number, nbe-miR164e; and NbMIR164h from N. benthamiana: N. benthamiana microRNA family database (https://sefapps02.qut.edu.au/benWeb/subpages/miRs6.php) accession number, nbe-miR164h.
Acknowledgments
We thank M. Leffler for help with the figures.
Author contributions
D.F.V. conceived, designed, and supervised the project and reviewed and edited the manuscript. D.L. conceived, designed, and performed experiments, analyzed the data, and wrote the manuscript. E.A.M., S.X., L.E.P., L.I.D., and C.G.S. performed experiments. E.E.E. provided pEE083 vector.
Supplementary data
The following materials are available in the online version of this article.
Supplementary Figure S1.PHYTOENE DESATURASE (NbPDS) knockout phenotypes and somatic editing in infected N. benthamiana plants.
Supplementary Figure S2. Target sites of cis-regulatory elements.
Supplementary Figure S3. Multinucleotide deletions of NbSTM5′UTR in N. benthamiana were transmitted to M2 generation.
Supplementary Table S1. Frequency of progeny with fixed indels at NbPDS-1.
Supplementary Table S2. Frequency of reads with indicated indels at NbSTM-15′UTR.
Supplementary Table S3. Frequency of reads with indicated indels at NbATML1-1pro.
Supplementary Table S4. Frequency of reads with indicated indels at NbMIR164e.
Supplementary Table S5. Frequency of reads with indicated indels at NbMIR164h.
Supplementary Table S6. Frequency of reads with indicated indels at SlMIR164b.
Supplementary Table S7. Frequency of progeny with fixed indels at NbATML1-1pro.
Supplementary Table S8. Frequency of progeny with fixed indels at NbATML1-2pro.
Supplementary Table S9. Frequency of progeny with fixed duplexed editing.
Supplementary Table S10. Primers used in this study.
Funding
This material is based upon work supported by the U.S. Department of Energy, Office of Science, Office of Basic Energy Sciences Energy Frontier Research Centers program under award numbers DE-SC-0018277 and DE-SC-0023160.
Data availability
The data supporting the research are available in the article and its supplementary materials.
Dive Curated Terms
The following phenotypic, genotypic, and functional terms are of significance to the work described in this paper:
References
Author notes
Daniel F. Voytas Senior author.
The author responsible for distribution of materials integral to the findings presented in this article in accordance with the policy described in the Instructions for Authors (https://dbpia.nl.go.kr/plphys/pages/General-Instructions) is Daniel F. Voytas ([email protected]).
Conflict of interest statement. None declared.