-
PDF
- Split View
-
Views
-
Cite
Cite
Jia Guo, Qiang Yao, Jie Dong, Jinrong Hou, Pulian Jia, Xueying Chen, Guoyang Li, Qi Zhao, Jingyi Wang, Fang Liu, Ziyu Wang, Yuying Shan, Tengyue Zhang, Aigen Fu, Fei Wang, Immunophilin FKB20-2 participates in oligomerization of Photosystem I in Chlamydomonas, Plant Physiology, Volume 194, Issue 3, March 2024, Pages 1631–1645, https://doi.org/10.1093/plphys/kiad645
- Share Icon Share
Abstract
PSI is a sophisticated photosynthesis protein complex that fuels the light reaction of photosynthesis in algae and vascular plants. While the structure and function of PSI have been studied extensively, the dynamic regulation on PSI oligomerization and high light response is less understood. In this work, we characterized a high light-responsive immunophilin gene FKB20-2 (FK506-binding protein 20-2) required for PSI oligomerization and high light tolerance in Chlamydomonas (Chlamydomonas reinhardtii). Biochemical assays and 77-K fluorescence measurement showed that loss of FKB20-2 led to the reduced accumulation of PSI core subunits and abnormal oligomerization of PSI complexes and, particularly, reduced PSI intermediate complexes in fkb20-2. It is noteworthy that the abnormal PSI oligomerization was observed in fkb20-2 even under dark and dim light growth conditions. Coimmunoprecipitation, MS, and yeast 2-hybrid assay revealed that FKB20-2 directly interacted with the low molecular weight PSI subunit PsaG, which might be involved in the dynamic regulation of PSI-light-harvesting complex I supercomplexes. Moreover, abnormal PSI oligomerization caused accelerated photodamage to PSII in fkb20-2 under high light stress. Together, we demonstrated that immunophilin FKB20-2 affects PSI oligomerization probably by interacting with PsaG and plays pivotal roles during Chlamydomonas tolerance to high light.
Introduction
Oxygenic photosynthesis, which converts solar energy to organic energy, is the fundamental physiological process to nurture lives on our planet. The light reaction of photosynthesis relies on 2 pigment-bound protein complexes embedded in thylakoid membranes, PSII and PSI for light absorption (Nelson and Ben-Shem 2004; Dekker and Boekema 2005). PSII performs the primary charge separation and transfers electrons to reduce plastoquinone pools. PSI performs as the light-driven plastocyanin:ferredoxin oxidoreductase, transferring electrons from the lumen-located donor plastocyanin to ferredoxin at the stroma side, providing the reducing power for carbon fixation cartelized by the Calvin cycle. Moreover, PSI also involves in the cyclic electron transfer and state transition to adapt to varying light conditions (Finazzi et al. 2002). PSI consists of the core complex for electron transfer reaction and the peripheral antenna system, light-harvesting complex I (LHCI), for light absorption, each including multiple protein subunits. The correct oligomerization of PSI complex with LHCI and its regulation determine the efficient PSI function and, in turn, safeguard the growth ability of photosynthetic organisms under different light conditions.
The advanced development of structural biology based on cryo-electron microscopy sheds light on the structure and pigment network of PSI-LHCI in several organisms, including land plants and Chlamydomonas (Chlamydomonas reinhardtii) (Qin et al. 2015; Steinbeck et al. 2018; Suga et al. 2019; Huang et al. 2021; Pan et al. 2021). Chlamydomonas PSI core complex is highly resembled to those of land plants, composed of 14 conserved subunits (Le Quiniou et al. 2015). The spatial arrangement of LHCI in algae and vascular plants has large variation during evolution. The Chlamydomonas PSI-LHCI10 supercomplex dissected by Suga et al. (2019) revealed an inner LHCI belt composed of LhcA3-LhcA7-LhcA8-LhcA1 and an outer belt composed of LhcA5-LhcA6-LhcA4-LhcA1, besides that, LhcA2 and LhcA9 bind weakly to the PSI core through PsaH/PsaL and PsaG in the side layer. The loose attachment of LhcA2 and LhcA9 may allow the rapid regulation of antenna size in PSI-LHCI complexes under different light conditions, and that was deduced to relate to the formation of PSI-LHCI-Cytb6f supercomplexes required for cyclic electron flow (Steinbeck et al. 2018).
The de novo assembly of PSI-LHCI complexes is a highly complex procedure, demanding the concerted action of several subunits and regulation factors (Schöttler et al. 2011; Yang et al. 2015). Some auxiliary factors, vesicle-inducing protein in plastid 1 and PSBP-domain protein 1, were characterized to take part in the early steps of correct PsaA/B subunit folding (Liu et al. 2012; Zhang et al. 2014). Hypothetical chloroplast open reading frame 3 (Ycf3), Ycf3-interacting protein 1, and Ycf4 might be involved in the integration of PsaC, PsaD, and PsaE, to form the reaction center (Boudreau et al. 1997; Albus et al. 2010; Nellaepalli et al. 2018). In the later steps, several other factors such as pale yellow green 7 and Albino3.1 are required, and small PSI subunits PsaF, PsaJ, PsaH, PsaI, PsaL, PsaG, PsaK, PsaN, and LhcA are assembled subsequently (Stöckel et al. 2006; Yang et al. 2017; Rathod et al. 2021). Among them, PsaG, PsaH, PsaN, and PsaO only exist in green algae and plants. PsaG was verified to foster the stabilization of PSI core and facilitate the binding of the peripheral antenna to PSI core in Arabidopsis (Arabidopsis thaliana) (Jensen et al. 2002; Varotto et al. 2002). PsaH and PsaO, together with PsaL, were identified to associate with LHCII during state transition (Lunde et al. 2000; Knoetzel et al. 2002; Jensen et al. 2007). PsaN was demonstrated to be necessary for the interaction between plastocyanin and PSI (Haldrup et al. 1999; Amunts et al. 2007). However, there is still a long way to go for approaching a clear conclusion on how this entire intricate procedure happens stepwise and how it is regulated properly, due to the functional divergence of PSI subunits and cofactors as well as the rapid occurrence. One solution for dissecting PSI oligomerization and its regulation is to identify more regulation factors involved in this physiological process.
Chlamydomonas has 56 immunophilins, including 23 FKBs (FKBs was referring to FKBPs in Chlamydomonas; Vallon 2005), which were named after their ability to bind rapamycin and FK506 and characterized to play important physiological roles in plants. Proteome data revealed there are 11 FKBPs existing in the thylakoid lumen, most of which lost their PPIase activity in Arabidopsis (Peltier et al. 2002; Schubert et al. 2002; Friso et al. 2004). AtFKBP13 is involved in Rieske protein accumulation and regulated by redox state (Gupta et al. 2002). AtFKBP16-2 was shown to take part in NAD(H) dehydrogenase complex stabilization (Peng et al. 2009). AtFKBP16-1 has been proven to enhance photosynthetic stress tolerance probably by stabilizing the PsaL protein (Seok et al. 2014). And the work of Lima described the function of AtFKBP20-2 as a regulator for PSII supercomplex formation (Lima et al. 2006). AtFKBP13 is the only FKBP identified to harbor PPIase activity so far; however, it does not play essential roles in photosynthesis under normal conditions.
Unlike the functional study on immunophilins in land plants, the study on Chlamydomonas immunophilins is still in its infancy. We constructed systematic analyses on chloroplast immunophilins in Chlamydomonas to explore their function on photosynthesis regulation (Fu et al. 2022). In this study, we identified the thylakoid lumen localized FKB20-2 protein as a regulator for PSI oligomerization in Chlamydomonas. Knocking out FKB20-2 led to the reduced accumulation of PSI core subunits and PSI intermediate complexes, which resulted in a high light (HL)-sensitive phenotype in fkb20-2. The more detailed mechanism was analyzed, and a hypothesized action model of FKB20-2 was discussed in our study.
Results
Lacking FKB20-2 causes HL-sensitive phenotype in Chlamydomonas
To identify important factors involved in HL tolerance in Chlamydomonas, we previously performed RNA-seq using Chlamydomonas wild-type (WT) cells treated with HL and obtained a group of HL-responsive immunophilins (Fu et al. 2022). The transcription profile of several immunophilins showed a particular pattern, decreasing after 15-min HL treatment (HL, 1,000 µmol m−2 s−1) and resuming gradually to the level before treatment (Fu et al. 2022). Among those, the expression of FKB20-2 decreased to about 50% after 15-min HL treatment compared with that of low light (LL) condition, and it resumed to 100% of LL level gradually after 60-min HL treatment (Fig. 1A). To understand the physiological function of FKB20-2, 1 fkb20-2 mutant (LMJ.RY0402.123087) was obtained from the Chlamydomonas mutant library (Chlamydomonas Library Project [CLiP]) (Li et al. 2019). The cassette containing the APHVIII gene was inserted into the third intron of FKB20-2 (Cre13.g577850) (Fig. 1B). The fkb20-2 mutant grew normally under LL on both TAP (mixotrophic) and HSM (autotrophic) media. However, when grown under HL, fkb20-2 displayed an obvious HL-sensitive phenotype (Fig. 1C). When the HSP70/RBCS2 double promoter-driven FKB20-2 genomic DNA was introduced into fkb20-2 by electroporation, the FKB20-2 transcript was highly accumulated (Fig. 1D), and the FKB20-2 protein accumulated to ∼50% of the WT level in the complementation strain fkb20-2C (Fig. 1E). The HL growth phenotype of fkb20-2C resembled that of WT strain (Fig. 1F), indicating that FKB20-2 is required for HL resistance in Chlamydomonas.
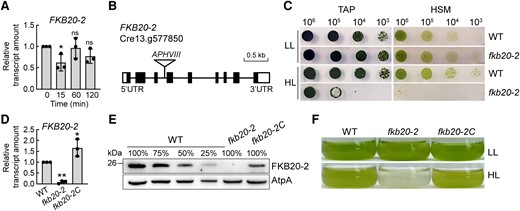
Deletion of FKB20-2 leads to HL-sensitive phenotype in Chlamydomonas. A) The transcript accumulation of FKB20-2 during 2-h HL treatment was detected by RT-qPCR in WT, with CBLP as a reference gene. The average values and Sd were calculated from biological triplicates. For the comparison of the values between LL (0) and different HL time points, the asterisks denote statistical significance according to 2-tailed Student's t-test: *P < 0.05; ns = not significant. B) The fkb20-2 mutant was generated by the insertion of a cassette with the paromomycin resistance gene (APHVIII) into the third intron of FKB20-2. C) The growth of fkb20-2 under LL (60 µmol m−2 s−1) and HL (1,000 µmol m−2 s−1) condition. Cells of WT and fkb20-2 in exponential phase were dropped on TAP (acetate-containing media) and HSM (minimum media) plates with 10 times dilution and grown under LL and HL conditions for 5 d. D) The transcript accumulation of FKB20-2 in WT, fkb20-2, and fkb20-2C was detected by RT-qPCR. The average values and Sd were calculated from biological triplicates. For the comparison of the values between WT and other strains, the asterisks denote statistical significance according to 2-tailed Student's t-test: *P < 0.05; **P < 0.01. E) The protein accumulation of FKB20-2 in WT, fkb20-2, and fkb20-2C was detected by immunoblotting with the antibody against FKB20-2, which was generated in this study. The signal of AtpA was used as the loading control. F) The growth phenotype of WT, fkb20-2, and fkb20-2C was observed in liquid TAP medium. The LL cultivated cell culture reaching exponential phase with the cell concentration ∼2 × 105/mL was grown under continuous LL or HL for 1 d.
The photosynthesis performance was affected in fkb20-2
To analyze how the loss of FKB20-2 influences the HL tolerance and photosynthesis performance, the chlorophyll fluorescence of WT, fkb20-2, and fkb20-2C grown under continuous LL conditions, was measured by dual PAM 100. The Fv/Fm value, which indicates the maximal quantum yield of PSII, was slightly lower in fkb20-2 compared with those of the WT and the complementation line fkb20-2C (Fig. 2A), indicating a slight deficiency in the steady-state level of PSII under LL. The ETR(II) value, which represents the linear electron transfer rate, decreased prominently along with the increasing irradiance in fkb20-2 (Fig. 2B). Consistent with the reduction of ETR(II), the oxygen evolution in fkb20-2 is also strongly reduced compared with those in WT and fkb20-2C (Fig. 2C). In contrast, their oxygen consumption levels are comparable. These together indicated that the photosynthesis performance was obviously diminished in fkb20-2, being consistent with the Arabidopsis Atfkbp20-2 mutants (Lima et al. 2006).
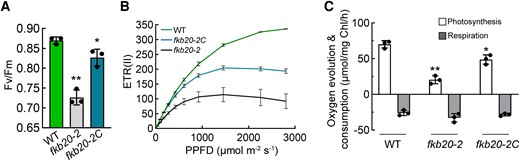
Steady-state photosynthesis was impaired in fkb20-2. A) The maximal PSII quantum yield (Fv/Fm) of WT, fkb20-2, and fkb20-2C was measured with the LL-grown (60 µmol m−2 s−1) cells. The average values and Sd were calculated from biological triplicates. For the comparison of the values between WT and other strains, the asterisks denote statistical significance according to 2-tailed Student's t-test: *P < 0.05; **P < 0.01. B) The linear electron transfer rate ETR(II) of WT, fkb20-2, and fkb20-2C was measured along with the increasing irradiance. The average values and Sd were calculated from biological triplicates. C) Oxygen evolution and consumption measurements were carried out with LL-grown cells. The average values and Sd were calculated from biological triplicates. For the comparison of the values between WT and other strains, the asterisks denote statistical significance according to 2-tailed Student's t-test: *P < 0.05; **P < 0.01.
The structure of AtFKP20-2 and FKB20-2 is highly conserved, and FKB20-2 shows no detectable PPIase activity in vitro
The phylogenetic analysis of FKBP proteins in different organisms showed close evolutionary conservation between Chlamydomonas and Arabidopsis, with almost one-to-one association between homologs (Vallon 2005). Chlamydomonas FKB20-2 was clustered together with Arabidopsis FKBP20-2 and Physcomitrium patens PHYPA 017085 (Supplemental Fig. S1). The amino acid (AA) sequence alignment between FKB20-2 and AtFKBP20-2 revealed a high similarity of the mature proteins (Fig. 3A), with 67% similarities and 46% identities. The predicted 3D structure of the mature AtFKBP20-2 by AlphaFold composes a short N-terminal region having 2 β-sheets and 1 α-helix and a C-terminal PPIase domain with a half-barrel-like structure formed by β-sheets (Supplemental Fig. S2; Jumper et al. 2021). Compared with AtFKBP20-2, the predicted 3D structure of FKB20-2 has 1 longer N-terminus possessing an additional 1 β-strand (β1) and 1 α-helix (α1) and a comparable C-terminal PPIase domain (Fig. 3B; Supplemental Fig. S2).
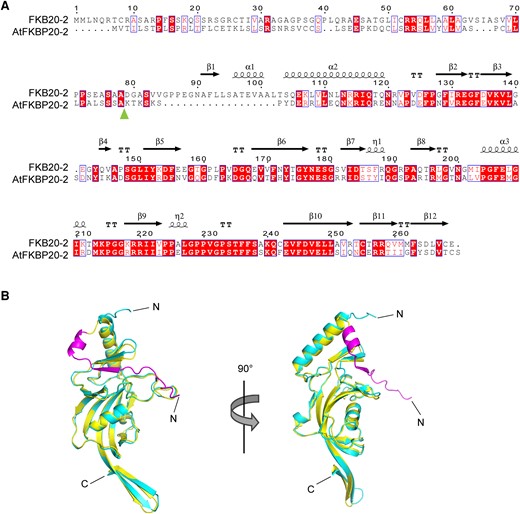
The comparison between AtFKBP20-2 and FKB20-2 proteins. A) The protein sequence alignment between FKB20-2 and AtFKBP20-2. The predicted cleavage site of chloroplast and lumen-targeting peptide was marked by the arrow. The secondary structures were indicated on the top of the sequences. B) The 3D structure of FKB20-2 and AtFKBP20-2 was predicted by the AlphaFold server and aligned in Pymol. The structure of AtFKBP20-2 was shown in canyon; that of FKB20-2 was in yellow. The extended extra peptide in FKB20-2 N-terminus was shown in purple.
The presence of the PPIase domain is a typical characteristic of immunophilins. When aligned with well-studied human FKBP12, only 29% of AA essential for PPIase activity were conserved in FKB20-2 (Vallon 2005). AtFKBP20-2 was previously identified to have a low PPIase activity (Lima et al. 2006). To verify if FKB20-2 has an in vitro PPIase activity, the small synthetic peptide N-succinyl-Ala-Ala-Pro-Phe-p-nitroanilide was applied as a substrate for the enzymatic assay. The recombinant AtFKBP13-GST displaying highly active PPIase was used as the positive control, and the GST protein was a negative control. In our measurement, the recombinant FKB20-2-GST does not have detectable PPIase activity in vitro, showing a similar OD390 changing pattern to that of GST protein (Supplemental Fig. S3A). By applying different amounts of proteins, AtFKBP13-GST showed distinct kinetics of OD390 curves, whereas no obvious difference was detected for FKB20-2-GST and GST (Supplemental Fig. S3, B to D).
AtFKP20-2 and FKB20-2 cannot complement reciprocally
The previous work showed that Atfkbp20-2 has a retarded growth phenotype and lower electron transfer rate in Arabidopsis (Lima et al. 2006). In an attempt to study the function conservation between FKB20-2 and AtFKBP20-2, we obtained a null mutant (SALK_134696) with a T-DNA insertion into the fifth intron of AtFKBP20-2 (At3g60307) and transformed the cDNA encoding mature FKB20-2 protein (79 to 269 AA) led by the chloroplast transit peptide and lumen-targeting peptide of AtFKBP20-2 (1 to 67 AA) into the Atfkbp20-2 mutant (Supplemental Fig. S4, A to C; Lima et al. 2006). The expression of exogenous FKB20-2 in Arabidopsis transgenic lines was confirmed by immunoblotting with FKB20-2 antibody, which cannot recognize the AtFKBP20-2 protein (Supplemental Fig. S4D). However, as shown in Supplemental Fig. S4E, FKB20-2 could not complement the retarded growth phenotype of Atfkbp20-2, indicating that FKB20-2 is probably not capable to replace the function of AtFKBP20-2 in Arabidopsis. In contrast, introducing the 35S promoter-driven AtFKBP20-2 cDNA into Atfkbp20-2 could restore its growth ability (Supplemental Fig. S4, C and E). We also transformed the cDNA encoding mature AtFKBP20-2 protein (68 to 242 AA) led by the chloroplast transit peptide and lumen-targeting peptide of FKB20-2 (1 to 78 AA) into Chlamydomonas fkb20-2 mutant to obtain fkb20-2-At (Supplemental Fig. S4C; Lima et al. 2006). Without AtFKBP20-2 antibody, we detected the transgene expression by reverse transcription PCR (RT-PCR), showing that the transcript of AtFKBP20-2 was accumulated in fkb20-2-At (Supplemental Fig. S4F). The photosynthetic performance of fkb20-2-At was analyzed by oxygen evolution and ETR(II) measurements. By both methods, fkb20-2-At behaved like fkb20-2 (Supplemental Fig. S4, G and H), which demonstrated that AtFKBP20-2 cannot complement the HL-sensitive phenotype of fkb20-2 in Chlamydomonas.
The oligomerization of PSI was affected in fkb20-2
Although with a high structure similarity, the uninterchangeable role of AtFKBP20-2 and FKB20-2 prompted us to investigate the function of FKB20-2 in Chlamydomonas. First, the steady-state level of chlorophyll content was measured. The total chlorophyll content had no obvious differences in all 3 strains (Fig. 4A). Compared with WT (∼2.62 ± 0.04), fkb20-2 had lower chlorophyll a/b ratio (∼2.03 ± 0.02), which was partially recovered in fkb20-2C (∼2.49 ± 0.05). The reduced chlorophyll a/b ratio indicated that the accumulation of photosystem core complexes, rather than antenna complexes, was impaired.
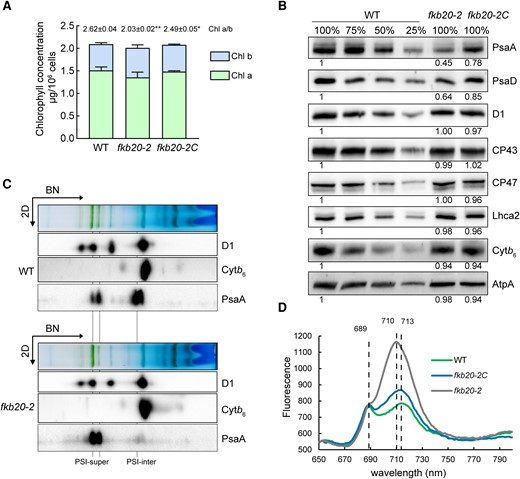
PSI oligomerization was altered in fkb20-2. A) The concentration of chlorophyll a and b was measured with the LL-grown WT, fkb20-2, and fkb20-2C. The average values and Sd were calculated from biological triplicates. The ratio between chlorophyll a and b in each strain was indicated on the top. For the comparison of the values between WT and other strains, the asterisks denote statistical significance according to 2-tailed Student's t-test: *P < 0.05; **P < 0.01. B) The accumulation of PSII (D1, CP43, and CP47), PSI (PsaA, PsaD, and LhcA2), Cytb6f (Cytb6) and ATPase (AtpA) subunits was analyzed by immunoblotting. The relative protein amount was indicated below the blots based on the quantification of the foci by ImageJ. C) The accumulation of photosynthesis complexes in WT and fkb20-2 was analyzed by immunoblotting on the 2D following 1D BN-PAGE. The position of PSI supercomplexes with LHCI (PSI-super) and that of PSI intermediate complexes (PSI-inter) were indicated below. D) 77-K fluorescence emission spectra of WT, fkb20-2, and fkb20-2C grown under LL was measured after excitation at 436 nm. The signals were normalized to the PSII emission maximum at 685 nm.
Next, the amount of representative photosynthesis proteins was detected by immunoblotting. The PSI core subunits, such as PsaA and PsaD, were largely decreased in LL-grown (60 µE) fkb20-2, being around 50% of the WT level, which coincides with the lower chlorophyll a/b ratio in the mutant (Fig. 4B). The peripheral subunit LhcA2, which is tightly attached to PSI core, had no obvious difference in fkb20-2 compared with those in WT (Fig. 4B). Moreover, the accumulation of PSII core subunits, including D1, CP43, and CP47, was almost unaffected in fkb20-2 (Fig. 4B). In addition, we did not observe the substantial alterations on accumulations of subunits Cytb6f and ATPase, represented by Cytb6 and AtpA, respectively (Fig. 4B).
We then analyzed the accumulation of photosynthetic complexes by 1D blue-native PAGE (BN-PAGE) and immunoblotting on the 2D SDS-PAGE. Thylakoid membranes were isolated from LL-grown WT and fkb20-2, solubilized by β-DM, and migrated on BN-PAGE gel. In WT, both PSI-LHCI supercomplexes and PSI core intermediates (indicated by PsaA) could be detected by immunoblotting. In stark contrast, the PSI intermediates were prominently reduced in fkb20-2 (Fig. 4C). The oligomerization of PSII (indicated by D1) and Cytb6f (indicated by Cytb6) are not affected in fkb20-2 compared with those in WT (Fig. 4C). To further confirm the impaired PSI oligomerization in fkb20-2, the 77-K fluorescence emission spectra were measured with LL-grown cells (Fig. 4D). The maximum fluorescence emission signal peaking at 713 nm representing the steady-state level of PSI-LHC was much higher in fkb20-2 compared with that of WT. Moreover, the maximum fluorescence emission was shifted from 713 to 710 nm in fkb20-2, indicating the disturbed association between PSI core and LHC. The fluorescence emission peak at 689 nm, corresponding to PSII supercomplexes, was comparable in all strains, consistent with the unaffected PSII complexes detected by BN-PAGE. Taken together, the results above demonstrated that the oligomerization of PSI complexes is affected in fkb20-2.
FKB20-2 proteins localize in thylakoid lumen and form complexes on thylakoid membrane
Chlamydomonas FKB20-2 possesses the typical thylakoid lumen-targeting signal as its homologous protein in Arabidopsis (Vallon 2005). To confirm its lumenal localization, immunoblotting was performed on different fractions isolated from WT chloroplasts. D1, plastocyanin, and rbcL were considered as marker proteins for thylakoid membrane, lumen, and stroma, respectively. FKB20-2 was detected in both the thylakoid membrane and lumen samples, suggesting that it is a lumen protein attaching to the lumen side of thylakoid membranes (Fig. 5A). In our BN-PAGE system, FKB20-2 proteins were mainly present in 2 parts, one as free proteins and the other forming complexes (Fig. 5B).
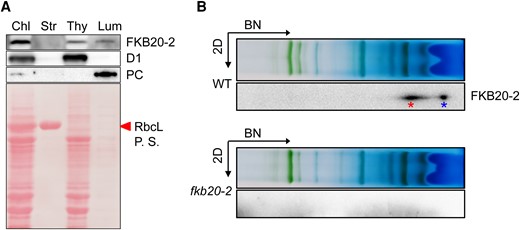
FKB20-2 proteins localize in thylakoid lumen and form protein complexes. A) FKB20-2 proteins were detected by immunoblotting in different chloroplast (Chl) fractions, using the thylakoid membrane (Thy) protein D1, the lumen (Lum) protein plastocyanin (PC), and the stroma (Str) protein rbcL as controls. P.S., Ponceau stain. B) The migration of FKB20-2 proteins in WT thylakoid membrane was analyzed by immunoblotting on the 2D following 1D BN-PAGE. The blot with fkb20-2 sample was used as a negative control. The FKB20-2 complex was marked by the left asterisk, and the free FKB20-2 protein was marked by the right asterisk.
FKB20-2 interacts with PsaG to influence the oligomerization of PSI
We then tried to identify the interacted proteins of FKB20-2 by coimmunoprecipitation/tandem MS (Co-IP/MS-MS). To this end, we generated fkb20-2C-HA strains by transforming the gene encoding FKB20-2 with a C-terminal HA tag into fkb20-2. The exogenous recombinant FKB20-2-HA protein could be detected in fkb20-2C-HA trans-genetic strains with immunoblotting using FKB20-2 and HA antibodies, respectively (Fig. 6A). Meanwhile, the FKB20-2-HA fusion protein could successfully restore the phenotype of fkb20-2, shown by the recovery of Fv/Fm values (Fig. 6B). The strain fkb20-2C-HA-31 expressing higher amount of FKB20-2-HA protein was used for the following experiments. Crude membranes were isolated from fkb20-2C-HA and WT (the negative control) and then solubilized by β-DM. The interacted proteins were copurified with FKB20-2-HA and analyzed by MS-MS. Obtained thylakoid membrane proteins with enrich ratio (iBAQFKB20-2C-HA/iBAQWT) > 2 and protein Q score > 10 were considered to be the potential interacted proteins of FKB20-2 (Supplemental Table S1). As fkb20-2 showed the PSI-deficient phenotype, the PSI subunits were further investigated. Among them, PsaG, the nucleus-encoded low molecular weight PSI peripheral protein, was listed on the top of the target proteins according to the enrich ratio, which was not detected in the WT negative control sample. The interaction between FKB20-2 and PsaG was further confirmed by independent Co-IP and immunoblotting using the elution samples. Clearly, the FKB20-2-HA protein was enriched and detected with immunoblotting using both anti-HA and anti-FKB20-2 antibodies (Supplemental Fig. S5). PsaG was enriched in the elution sample of fkb20-2C-HA but not in that of WT. However, the peripheral LhcA2 cannot be detected (Fig. 6C). The structure of PSI supercomplexes dissected in Suga et al. (2019) indicated that PsaG possesses 2 transmembrane helixes (36 to 56and 97 to 117 AA), expanding across the thylakoid membranes. The N-terminus and C-terminus are both exposed to the lumen. The association between PsaG and FKB20-2 was further confirmed by the DUAL membrane yeast 2-hybrid system based on the split-ubiquitin mechanism (Fig. 6D).
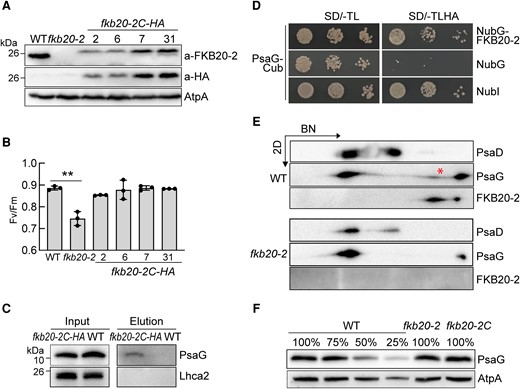
FKB20-2 associates with PSI peripheral subunit PsaG. A) The accumulation of FKB20-2-HA proteins was detected in fkb20-2C-HA by immunoblotting with FKB20-2 antibody and HA antibody, respectively. The signal of ATPase (AtpA) was used as the loading control. B) The Fv/Fm values of FKB20-2-HA complemented strains were measured with the LL-grown cells. The average values and Sd were calculated from biological triplicates. The asterisks denote statistical significance according to 2-tailed Student's t-test: **P < 0.01. C) The FKB20-2-PsaG association was confirmed by immunoblotting with the samples from independent Co-IP experiment. WT and fkb20-2C-HA cells were grown under continuous LL before membrane extraction. D) The interaction between PsaG and FKB20-2 was confirmed by the split-ubiquitin yeast 2-hybrid experiment. SD, synthetic drop-out medium. E) The comigration of FKB20-2 and PsaG proteins in WT (marked by the asterisk) was analyzed by immunoblotting on the 2D following 1D BN-PAGE. F) The accumulation of PsaG protein in WT, fkb20-2, and fkb20-2C was analyzed by immunoblotting. The signal of AtpA was used as the loading control.
The comigration of PsaG and FKB20-2 was investigated by BN-PAGE. Consistently, both PSI-LHCI supercomplexes and PSI core intermediates (indicated by PsaD) were observed in WT, whereas only a trace amount of PSI core intermediates were detected in fkb20-2 (Fig. 6E). In WT, PsaG mainly exists in 3 partitions, one comigrating with PSI-LHCI supercomplexes, one as small protein complex (marked by asterisk), and the rest as free protein present in the lowest molecular weight position (Fig. 6E), whereas, in fkb20-2, PsaG proteins were mainly associated with PSI-LHCI supercomplexes, leaving less free proteins and no small complexes. In addition, we observed that the total amount of PsaG in fkb20-2 and WT was comparable (Fig. 6F).
FKB20-2 is required for the oligomerization of PSI under various light conditions
Unlike land plants that require light for chlorophyll biosynthesis and biogenesis of photosynthesis protein complexes, Chlamydomonas can achieve those in the darkness (dark). To avoid the photodamages to PSI under light, we evaluated the oligomerization of PSI complexes in the cells grown in complete dark and dim light (DL, 5 µmol m−2 s−1) using BN-PAGE and 2D SDS-PAGE; meanwhile, the cells grown under LL and treated by 1-h HL were also supplemented to the analyses. To be noticed, the PSI intermediate complexes were always strongly reduced in fkb20-2 compared with those in WT, regardless of the light regimes (Fig. 7A). Consistently, the accumulations of PSI core subunit PsaD in fkb20-2 cells grown under different conditions were reduced to a similar extent (Fig. 7B). These suggested that the reduced PSI core intermediates in fkb20-2 are not likely caused by photodamages from HL.
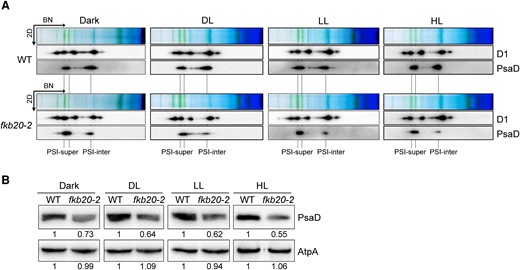
PSI oligomerization was altered under different growth light conditions in fkb20-2. A) The accumulation of PSI (PsaD) and PSII (D1) complexes under complete dark, DL (5 µmol m−2 s−1), LL (60 µmol m−2 s−1), and 1-h HL treatment (1,000 µmol m−2 s−1) in WT and fkb20-2 was analyzed by immunoblotting on the 2D following 1D BN-PAGE. The position of PSI supercomplexes (PSI-super) and that of PSI intermediate complexes (PSI-inter) were indicated below. B) The accumulation of PsaD in WT and fkb20-2 under dark, DL, LL, and 1-h HL treatment was analyzed by immunoblotting, using ATPase (AtpA) as loading control. The relative protein amount was indicated below the blots based on the quantification of the foci by ImageJ.
HL causes photodamage to PSII in fkb20-2
PSII was foremost injured by excess light among photosynthetic complexes, the core subunit of which D1 protein has a rapid turnover rate (Nixon et al. 2010). In attempt to dissect the HL-sensitive phenotype of fkb20-2 more precisely, we detected the accumulation of PSII core subunit D1, PSI core subunits PsaA and PsaD in WT and fkb20-2 under both LL and HL treatments (Fig. 8A). To discriminate between the turnover and stability of D1 and PsaA that were translated by chloroplast ribosomes, lincomycin and chloramphenicol were applied in parallel to inhibit the chloroplast translation (+LC). Being consistent with previous observations, the accumulations of PsaA and PsaD were obviously reduced in fkb20-2 compared with those in WT, regardless of light intensities (Fig. 8A, −LC). After 1-h HL treatment without LC, D1 protein amount was comparable between WT and fkb20-2, with only a slight decrease (Fig. 8A, −LC). To be noticed, when the chloroplast translation was blocked by LC, in contrast to more stable PSI subunits PsaA, a drastic decrease was observed for D1 protein in fkb20-2 after HL treatment, indicating the instability of PSII under HL (Fig. 8A, +LC). This coincided with the HL-sensitive phenotype of fkb20-2. The instability of PSII was mainly caused by highly accumulated reactive oxygen species (ROS) in the chlorophyll-bound photosystems under HL stress (Apel and Hirt 2004). Therefore, the ROS levels in WT and fkb20-2 were measured under continuous DL and LL or after being treated by HL for 15 min. Under both LL and HL conditions, more ROS was produced that might cause more damage to PSII core complexes in fkb20-2 (Fig. 8B). We also analyzed the accumulation of FKB20-2 proteins during HL treatment. Consistent with the gradual increase of FKB20-2 transcript, after 2-h HL treatment, its protein accumulation increased to ∼1.5-fold compared with that under LL (Fig. 8C, −CHX). Moreover, FKB20-2 was relatively stable under HL stress, shown by immunoblotting with the samples in the presence of cytosol translation inhibitor cycloheximide to block de novo translation of FKB20-2 (Fig. 8C, +CHX).
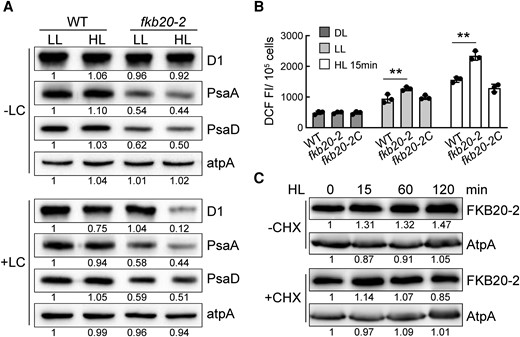
PSII was photodamaged after HL treatment in fkb20-2. A) The accumulation of D1 and PsaD proteins under LL and 1-h HL treatment was detected in WT and fkb20-2 by immunoblotting. +LC for the presence of lincomycin and chloramphenicol. The relative protein amount was indicated below the blots based on the quantification of the foci by ImageJ. B) The intracellular ROS content in WT, fkb20-2, and fkb20-2C either grown under continuous DL and LL or treated by HL for 15 min. The average values and Sd were calculated from biological triplicates. The asterisks denote statistical significance according to 2-tailed Student's t-test: **P < 0.01. DCF FI, the fluorescence intensity of DCF. C) The FKB20-2 protein accumulation during 2-h HL treatment with (+)/without (−) cycloheximide (CHX) was detected by immunoblotting, with ATPase (AtpA) signal as the loading control. The relative protein amount was indicated below the blots based on the quantification of the foci by ImageJ.
Discussion
Immunophilin FKB20-2 affects the oligomerization of PSI complexes and HL tolerance in Chlamydomonas
Oxygenic photosynthetic organisms evolved various mechanisms to overcome photodamages caused by HL stress. In thylakoid membranes, PSI and PSII are the 2 main large complexes bound by light-harvesting proteins for solar energy absorption; therefore, they are the foremost targets of the harmful ROS produced by excited chlorophylls (Tanaka and Tanaka 2011). In an effort to understand the physiological function of immunophilin proteins in HL response, we systematically characterized the HL growth phenotype of Chlamydomonas immunophilin mutants. Here, we found that immunophilin FKB20-2 is involved in HL resistance, using a null mutant fkb20-2 (Fig. 1). In contrast to immunophilin CYN28, which is required for the turnover of FtsH and the PSII repair cycle identified in our previous work (Fu et al. 2022), the loss of FKB20-2 results in a weak PSII-deficient phenotype but a strong alteration in the oligomerization of PSI under LL conditions (Figs. 4, 5, and 7). The impeded oligomerization of PSI may lead to ROS production due to the restricted electron flow and cause a severe photodamage to PSII, which in turn results in a HL-sensitive phenotype, shown by the instability of D1 protein in the presence of chloroplast translation inhibitors (Figs. 1, 4, and 8). Similar phenomena showing exaggerated PSII photoinhibition and HL sensitivity were observed in other PSI mutants. Hippler et al. verified that a PsaF-deficient mutant is capable of photoautotrophic growth, however, sensitive to HL stress, due to the direct photodamage to PSII reaction center (Farah et al. 1995; Hippler et al. 2000).
In WT, our BN-PAGE mainly detected 2 PSI supercomplexes (Figs. 4C and 7A), which might represent PSI-LHCI8 and PSI-LHCI10, respectively, according to previous research (Suga et al. 2019; Huang et al. 2021; Pan et al. 2021). Moreover, 1 stable PSI complex without PsaG was present in a lower molecular weight position, which was usually considered as the assembly intermediate of PSI core without peripheral antenna complexes, especially in young leaf tissues of Arabidopsis and fast-growing Chlamydomonas cell (Ozawa et al. 2010; Wittenberg et al. 2017). In our BN-PAGE analyses, the similar PSI intermediate complex without PsaG was observed, which was remarkably reduced in fkb20-2 compared with WT (Figs. 4C and 6E). Since the decrease of PSI intermediates in fkb20-2 does not rely on light intensity, it would be plausible to assume that FKB20-2 is involved in the formation of the PSI intermediates, and the PSI intermediate complexes are not stably established in fkb20-2 without FKB20-2. Some other possible scenarios should also be considered. We also could not rule out the possibility that FKB20-2 is involved in the disassociation of LHC antenna from PSI supercomplexes to balance the proportion between PSI supercomplexes and PSI intermediates.
FKB20-2 may affect PSI oligomerization by direct association with PsaG, the small subunit of PSI
PSI complexes are multisubunit assemblies composed of nucleus genome-encoded proteins and chloroplast genome-encoded small proteins. The deletion of essential chloroplast-encoded subunits, such as PsaA, PsaB, and PsaC abolished the photoautotrophic growth of Chlamydomonas (Takahashi et al. 1991). To understand how FKB20-2 affects PSI oligomerization, we performed Co-IP/MS-MS (Fig. 6C). A list of PSI small subunits, including PsaG, instead of the core protein PsaA/B, was identified in the fkb20-2C-HA samples (Supplemental Table S1). Further biochemical assays, including Co-IP, yeast 2-hybrid, and comigration in BN-PAGE, confirmed the association between FKB20-2 and PsaG (Fig. 6, C to E).
The function of the nucleus-encoded PSI small subunits remains to be relatively vague. Although the mechanisms are still not known yet, phenotypic characterization of the knockout and knockdown mutants illustrated that they are mainly involved in the assembly of mature PSI complexes, as well as the association of cofactors. Although there has been no PsaG mutant characterized in Chlamydomonas, the elaborate dissection of Chlamydomonas PSI-LHCI supercomplexes deduced that PsaG might affect the association between PSI core and loosely attached LHCI (Suga et al. 2019). In Arabidopsis, PsaG was characterized to stabilize the PSI core and to facilitate the binding of the peripheral LHC antenna (Varotto et al. 2002). Jensen et al. (2002) revealed that the chlorophyll a/b ratio is slightly decreased in the Arabidopsis PsaG mutant, and there was a 1-nm blueshift in the far-red emission spectra from 734 to 733 nm in 77-K measurement, indicating that the interaction between LhcA and PSI core was perturbed. Similar to the Arabidopsis PsaG mutant, we found that the fkb20-2 mutant also displayed lower chlorophyll a/b value (Fig. 4A), reduced accumulation of PSI core subunits (Fig. 4B), and blueshift in the long-wavelength fluorescence emission (Fig. 4D). Moreover, the dramatic decrease of PSI intermediates supported the PSI-deficient phenotype of fkb20-2 (Figs. 4C and 7A). To be noticed, in fkb20-2, PsaG proteins mainly comigrated with PSI-LHCI supercomplexes with less free proteins compared with WT. This altered partitioning of PsaG in PSI-LHCI and low molecular weight position proposed that FKB20-2 might be involved in the regulation of PSI oligomerization by directly interacting with PsaG. The proper and dynamic oligomerization of PSI complexes determines the vitality of plants and algae under different light intensities. Lacking FKB20-2 probably leads to a disturbed function of PsaG, and the impaired regulation of PSI oligomerization results in the imbalance between PSI-LHCI and PSI intermediates, leaving less accumulated PSI intermediates, which in turn leads to more severe photodamage under excess light conditions, and causes hampered growth of Chlamydomonas cells.
The function of FKBP protein diverged along with the evolution of photosynthetic organisms
The macrolide antibiotic rapamycin was identified as a potent antifungal agent and then proved to have immunosuppressive activity (Heitman et al. 1991; Schreiber 1992). FKBP proteins were named according to their ability to bind rapamycin and FK506. There are 23 FKBP proteins present in both Arabidopsis and Chlamydomonas, whereas only 2 FKBPs exist in Synechocystis (Supplemental Fig. S1), which implies the function of FKBPs diverged before the evolving of eukaryotic photosynthetic organisms. The most well-studied FKBP12 is conserved in various organisms, including land plants, yeasts, and mammalian cells (Supplemental Fig. S1). The previous research on FKBP12 proteins during the last 3 decades revealed their divergent functions (Dolinski et al. 1997; Shou et al. 1998; Crespo et al. 2005; Cheung et al. 2020; Serrano-Bueno et al. 2020). It was proposed that the functions of FKBPs vary largely during long-term evolution via targeting to different substrate proteins. Our study on Chlamydomonas FKB20-2 revealed its essential function during PSI oligomerization, unlike its Arabidopsis homolog AtFKBP20-2, which was involved in the accumulation of PSII supercomplexes. Knocking out AtFKBP20-2 caused a retarded growth in Arabidopsis (Lima et al. 2006). In this study, efforts were spent on analyzing the functional conservation between FKB20-2 and AtFKBP20-2. When we ectopically expressed Chlamydomonas FKB20-2 in the Atfkbp20-2 mutant, the retarded growth phenotype cannot be complemented (Supplemental Fig. S4E). Meanwhile, AtFKBP20-2 cannot restore the phenotype of fkb20-2 to WT either (Supplemental Fig. S4, G and H). A comparison between the structures of FKB20-2 and AtFKBP20-2 unveiled the different organizations of their N-terminal regions (Fig. 3; Supplemental Fig. S2). The varied N-termini might determine the interaction of FKBPs to specific target proteins.
The multiplicity of PPIase in plants was noticed a long time ago. For some of the immunophilins, the PPIase is inactive. Among the 5 AA that were considered to be essential for PPIase activity in human FKBP12, only 2 in both FKB20-2 and AtFKBP20-2 are conserved (Vallon 2005). The activity assay indicated the depleted PPIase activity of AtFKBP20-2, which proved the necessity of key residues for activity preservation (Lima et al. 2006). In our study, Chlamydomonas FKB20-2 showed no substantial PPIase activity compared with the highly active AtFKBP13 (Supplemental Fig. S3). The loss of PPIase activity for most of the lumenal FKBPs brings speculation that the proper function of FKBPs may be independent of their PPIase activity.
Given that PsaG is one highly conserved PSI subunit in algae and land plants, the PsaG-mediated PSI oligomerization might also exist in other photosynthetic organisms. However, the regulator protein involved in this process could be varied between algae and land plants, due to the functional divergence of immunophilin proteins. Future studies on chloroplast immunophilins will shed light on the intricate regulation of photosynthetic machinery under stress conditions, which will hopefully strengthen the foundation of using microalgae as a platform for bioenergy production.
Materials and methods
Chlamydomonas (C. reinhardtii) strains and Arabidopsis (A. thaliana) plants
Chlamydomonas WT cc5325 and fkb20-2 mutants (LMJ.RY0402.123087) were obtained from CLiP. The cells were grown in TAP medium at 25 °C under DL (5 µmol photons m−2 s−1), LL (60 µmol photons m−2 s−1), HL (1,000 µmol photons m−2 s−1), or complete dark.
For Arabidopsis plants, all genotypes used were of the ecotype Col-0. AtFKBP20-2 (At3g60307) insertional mutant (SALK_134696) was kindly provided by Dr. Sheng Luan (Department of Plant and Microbial Biology, University of California, Berkeley, CA 94720, USA). Sterilized seeds were vernalized at 4 °C for 3 d and sown on commercial soil mix (Pindstrup, Denmark). The plants were grown for 4 to 5 wk at 23 °C in a 16-h light/8-h dark cycle with a photon flux density of 100 µmol photons m−2 s−1.
Transgenic strain/plant generation
For the generation of complementation strain fkb20-2C, FKB20-2 genomic DNA was amplified and cloned into pOpt-Clover digested by NdeI and EcoRI with pEASY-Basic Seamless Cloning and Assembly Kit (Transgen) and transformed into fkb20-2 mutant by electroporation. The positive strains were screened first by hygromycin resistance and then by FKB20-2 protein accumulation. One representative strain was used for further analyses. To obtain the mutant strain complemented by HA-tagged FKB20-2 at its C-terminus, the HA sequence was added to FKB20-2 3′ end by PCR. The transformation and screening of positive colonies were performed as above.
For the generation of complementation plant Atfkbp20-2-At, the AtFKBP20-2 coding sequence (CDS) was cloned into pLM-B001 by XhoI and XbaI and transformed into Atfkbp20-2 using the floral dip method (Clough and Bent 1998). To express Chlamydomonas FKB20-2 in Atfkbp20-2, the 35S promoter-driven CDS encoding mature FKB20-2 (79 to 269 AA) led by the chloroplast and lumen-targeting peptide of AtFKBP20-2 (1 to 67 AA) was cloned into pLM-B001 by XbaI and BamHI. The positive trans-genetic lines were screened first by basta resistance and then by FKB20-2 protein accumulation detected by immunoblotting with FKB20-2 antibody. For the generation of complementation line fkb20-2-At, the cDNA sequence encoding FKB20-2 chloroplast and lumen-targeting peptide (1 to 78 AA) and AtFKBP20-2 mature peptide (68 to 242 AA) were cloned into pOpt-Clover linearized by NdeI and EcoRI with pEASY-Basic Seamless Cloning and Assembly Kit (Transgen). The construct was transformed into fkb20-2 by electroporation. The positive trans-genetic lines were screened first by hygromycin resistance and then by AtFKBP20-2 transcript accumulation detected by RT-PCR. The primers related to this study were listed in Supplemental Table S2.
RT-qPCR
Total RNA was isolated from Chlamydomonas cells and Arabidopsis leaves following the instruction of Rneasy Plant Mini Kit (Tiangen). First-strand cDNA was synthesized following the instruction of TransScript Uni All-in-one First-Strand cDNA Synthesis SuperMix for qualitative PCR (qPCR) (Transgen). RT-qPCR was performed by LightCycle480 System (Roche), using SYBR Green Premix Pro Taq HS qPCR Kit (Accurate Biology), with CBLP (Cre06.g278222) as a reference control. The average relative gene expression value was calculated based on biological triplicates. One biological replicate represents an independent HL treatment, followed by RNA extraction and cDNA synthesis. The statistical analyses were performed according to 2-tailed Student's t-test.
Blue-native gel and Co-IP
The Chlamydomonas crude thylakoid membranes were isolated according to the method from Wang et al. (2017). For blue-native gel analysis, membranes (chlorophyll concentration equal to 0.5 µg/µL) were solubilized by 1% (w/v) β-DM and separated on 3.5% to 12% blue-native gel. To detect the accumulation of individual photosynthesis subunit, a 2D SDS-PAGE with 6 m urea was applied. The antibodies used for immunoblotting were listed below. For Co-IP by HA tag, the crude membranes from fkb20-2C-HA were solubilized by 1% (w/v) β-DM, with the WT membranes as a negative control. The Co-IP experiment was conducted according to the instruction of Monoclonal Anti-HA-Agarose, clone HA-7 (A2095).
For detecting the interaction proteins of FKB20-2-HA by MS, the eluted sample mixtures were proteolyzed and separated by a Shimadzu LC-20AD model nanoliter liquid chromatograph and ionized by a nanoESI source and then passed to a tandem mass spectrometer LTQ Orbitrap Velos (Thermo Fisher Scientific) for data-dependent acquisition detection. The detected peptide sequences were aligned to Creinhardtii_281_v5.0 (JGI) and UniProt protein database (uniprot_taxonomy_3055_and_Creinhardtii_281_v5.6_all_filtered.fa). The output was then filtered by false discovery rate (FDR) 1% at spectral level (PSM-level FDR ≤ 0.01) to obtain a significant identified spectrum and peptide list.
The split-ubiquitin membrane yeast 2-hybrid
The split-ubiquitin membrane yeast 2-hybrid was applied according to the instruction of DUALmembrane Kit 2 (Dualsystems Biotech). The mature FKB20-2 peptide (79 to 269 AA) was constructed into pDSL-Nx by SfiI restriction enzyme site, which expressed NubG-FKB20-2 as prey protein. The mature PsaG peptide (36 to 126 AA) was cloned into pCCW-STE by SfiI, which produced PsaG-Cub-LexA-VP16 as bait protein.
The bait plasmid pCCW-STE-PsaG and the prey plasmid pDSL-FKB20-2 were cotransformed into the yeast strain NMY32. The interaction between PsaG and FKB20-2 was tested on SD/-TLHA medium. Meanwhile, the bait plasmid was cotransformed with plasmid pDL-Alg5 (NubG) for negative control and pAI-Alg5 (NubI) for positive control, respectively.
PPIase activity assay
The enzymatic activity assay of PPIase was performed at 10 °C by UV-VIS Double Beam Spectrophotometer (Dynamica) as described in Vasudevan et al. (2012). The substrate peptide N-succinyl-Ala-Ala-Pro-Phe-p-nitroanilide (Sigma-Aldrich) was dissolved in 2,2,2-trifluoroethanol containing 400 mm LiCl. The same amount of recombinant protein FKB20-2-GST, AtFKBP13 (positive control), and GST (negative control) was applied for the assay. The A390 value was recorded each second for 2 min.
Chlorophyll fluorescence measurement
Chlorophyll fluorescence was measured by dual PAM 100 (Walz) according to Schreiber et al. (1986). The Chlamydomonas culture at the exponential phase was dark adapted for 5 min before measurement. PSII maximal quantum yield was evaluated by Fv/Fm = (Fm − Fo)/Fm. The electron transfer rate of PSII was calculated as ETR(II) = [(Fm′ − F′)/Fm′] PAR·absorptance·dII. Other PSII energy dissipation parameters were estimated as indicated by Kramer et al. (2004). The oxygen evolution/consumption rate was measured using a Clark-type oxygen electrode. Chlamydomonas cells were grown under LL in TAP medium to exponential phase before measurement. The measurement was applied at 25 °C, 1,000 µmol m−2 s−1. The average value was calculated based on biological triplicates. One biological replicate represents an independent cell culture. The statistical analyses were performed according to 2-tailed Student's t-test.
Analysis of chlorophyll fluorescence emission at 77 K was carried out on frozen Chlamydomonas cells by using Hitachi (Tokyo) F-7100 fluorescence spectra. Samples of WT, fkb20-2, and fkb20-2C were resuspended to a chlorophyll concentration of ∼10 µg/mL, frozen in NMR tubes with liquid nitrogen in the presence of 10% (v/v) glycerol, and excited at 436 nm. Corrected emission spectra were collected between 650 and 800 nm.
ROS detection
For ROS measurement, cells grown under LL or treated by 15-min HL were harvested and washed with MOPS·P medium (pH 7.0). The cell pellets were resuspended in MOPS·P medium containing 10 µm chloromethyl-2′,7′-dichlorodihydrofluorescein diacetate (H2DCFDA) and incubated in dark for 30 min. The fluorescence intensity of DCF was measured by the microplate reader (BioTek Synergy HTX) with excitation/emission at 485/530 nm. The average value was calculated based on biological triplicates. One biological replicate represents an independent cell culture treated under different light intensities. The statistical analyses were performed according to 2-tailed Student's t-test.
Protein alignment and phylogenetic analysis
Full-length sequences of FKBP proteins in selected organisms Escherichia coli, Synechocystis (Synechocystis sp. PCC6803), Cyanidioschyzon merolae, Chlamydomonas, moss (P. patens), Arabidopsis, and human (Homo sapiens) were downloaded from National Center for Biotechnology Information (http://www.ncbi.nlm.nih.gov/) (Supplemental Table S3). The protein alignment and phylogenetic tree were built using MEGA 7.0 by neighbor-joining method and 1,000 bootstrap replicates.
Antibody used in this study
The FKB20-2 polyclonal antibody was generated as follows: the CDS corresponding to 79 to 269 AA of FKB20-2 protein was amplified and constructed into pGEX-4T-3 by restriction enzyme sites EcoRI and XhoI for the production of recombinant FKB20-2-GST protein in E. coli. The FKB20-2 protein was cleaved off from the GST tag by thrombin and injected into Rabbits. The FKB20-2 antibody was affinity purified before usage.
Anti-D1, anti-PC, anti-AtpA, and anti-Cytb6 are the polyclonal antibodies generated by our lab. Anti-LhcA2 (AS01006) and anti-PsaG (AS06144) were purchased from Agrisera. Anti-PsaA (PHY0342), anti-PsaD (PHY0343), anti-CP43 (PHY0365A), anti-CP47 (PHY0058A), and anti-HA (PHY5011) were purchased from PhytoAB.
Accession numbers
Sequence data from this article can be found in the GenBank/EMBL data libraries under accession numbers FKB20-2, Cre13.g577850; CBLP, Cre06.g278222, AtFKBP13, AT5G45680; and AtFKBP20-2, At3g60307.
Acknowledgments
We thank Dr. Sheng Luan (Department of Plant and Microbial Biology, University of California, Berkeley) for kindly providing the Atfkbp20-2 mutant line.
Author contributions
F.W and A.F. conceived and designed the experiments; J.G., Q.Y., J.D., J.H., P.J., X.C., G.L., Q.Z., J.W., F.L., Z.W., Y.S., and T.Z. performed the experiments; F.W., A.F., J.G., Q.Y., and J.D. analyzed the data; and F.W. and A.F. wrote the paper.
Supplemental data
The following materials are available in the online version of this article.
Supplemental Figure S1. Phylogenetic analysis of FKBP proteins.
Supplemental Figure S2. The predicated 3D structure of FKB20-2.
Supplemental Figure S3. FKB20-2 does not have PPIase activity.
Supplemental Figure S4.AtFKBP20-2 and FKB20-2 cannot complement reciprocally.
Supplemental Figure S5. The FKB20-2-HA protein could be immuno-precipitated from fkb20-2C-HA strain.
Supplemental Table S1. Peptide identification by MS.
Supplemental Table S2. Primers used in this study.
Supplemental Table S3. Protein sequences in phylogenetic tree.
Funding
This work was supported by the National Natural Science Foundation of China (31800065 and 32070269) and the Shaanxi Fundamental Science Research Project for Chemistry & Biology (22JHZ007).
Data availability
The data underlying this article are available in the article and in its online supplementary material.
Dive Curated Terms
The following phenotypic, genotypic, and functional terms are of significance to the work described in this paper:
References
Author notes
These authors contributed equally.
The author responsible for distribution of materials integral to the findings presented in this article in accordance with the policy described in the Instructions for Authors (https://dbpia.nl.go.kr/plphys/pages/General-Instructions) is: Fei Wang ([email protected]).
Conflict of interest statement. None declared.