-
PDF
- Split View
-
Views
-
Cite
Cite
Le Fang, Chao Geng, Xin-Yu Wei, Chen-Chen Dong, Ju-Ping Pang, Zhi-Yong Yan, Jun Jiang, Yan-Ping Tian, Xiang-Dong Li, Potato virus Y viral protein 6K1 inhibits the interaction between defense proteins during virus infection, Plant Physiology, Volume 194, Issue 3, March 2024, Pages 1447–1466, https://doi.org/10.1093/plphys/kiad612
- Share Icon Share
Abstract
14-3-3 proteins play vital roles in plant defense against various pathogen invasions. To date, how 14-3-3 affects virus infections in plants remains largely unclear. In this study, we found that Nicotiana benthamiana 14-3-3h interacts with TRANSLATIONALLY CONTROLLED TUMOR PROTEIN (TCTP), a susceptibility factor of potato virus Y (PVY). Silencing of Nb14-3-3h facilitates PVY accumulation, whereas overexpression of Nb14-3-3h inhibits PVY replication. The antiviral activities of 3 Nb14-3-3h dimerization defective mutants are significantly decreased, indicating that dimerization of Nb14-3-3h is indispensable for restricting PVY infection. Our results also showed that the mutant Nb14-3-3hE16A, which is capable of dimerizing but not interacting with NbTCTP, has reduced anti-PVY activity; the mutant NbTCTPI65A, which is unable to interact with Nb14-3-3h, facilitates PVY replication compared with the wild-type NbTCTP, indicating that dimeric Nb14-3-3h restricts PVY infection by interacting with NbTCTP and preventing its proviral function. As a counter-defense, PVY 6K1 interferes with the interaction between Nb14-3-3h and NbTCTP by competitively binding to Nb14-3-3h and rescues NbTCTP to promote PVY infection. Our results provide insights into the arms race between plants and potyviruses.
Introduction
To establish a successful infection, plant RNA viruses hijack host factors to replicate and move. For example, beet black scorch virus (BBSV, genus Betanecrovirus) utilizes Nicotiana benthamiana RETICULON-LIKE PROTEIN B2 (RTNLB2) to remodel the endoplasmic reticulum membrane and facilitate the assembly of viral replication complex (VRC) (Zhang et al. 2023); host receptor-like kinase BARELY ANY MERISTEM 1 (BAM1) is required for tobacco mosaic virus (TMV, genus Tobamovirus) cell-to-cell movement (Tran and Citovsky 2021). Recently, 2 reports have shown that silencing of translationally controlled tumor protein (TCTP) expression in tomato (Solanum lycopersicum) or N. benthamiana separately suppresses pepper yellow mosaic virus (PepYMV) and turnip mosaic virus (TuMV) infection. TCTP-silenced plants exhibit milder symptom and accumulate less virus compared with the control plants (Bruckner et al. 2017, 2021). In another study, almost no symptom has been observed in potato virus Y (PVY)–infected TCTP-silenced Nicotiana tabacum plants (Guo et al. 2017). These results indicate that TCTP is a host susceptibility factor for potyvirus infection. However, it remains unknown how TCTP is involved in potyvirus infection.
Drosophila melanogaster TCTP has been reported to interact with 14-3-3 and coregulates organ development (Le et al. 2016). The 14-3-3 family proteins are known to play diverse roles in plant signal transduction, metabolism, hormone signaling, and defense responses to biotic and abiotic stresses (Dougherty and Morrison 2004; Camoni et al. 2018; Kong and Ma 2018; Visconti et al. 2019; Ren et al. 2021; Zhao et al. 2021; Jiang et al. 2023; Song et al. 2023). Crystal structures show that 14-3-3 proteins can dimerize and form flattened horseshoe-like structures, and each structure has a central cavity and 2 amphipathic grooves (Lozano-Duran and Robatzek 2015; Gupta et al. 2019; Harada et al. 2022), allowing these proteins to modulate various biological processes through interaction with specific targets in plants and animals (Ottmann et al. 2007; Aitken 2011; Hartman and Hirsch 2017). Multiple 14-3-3 homologous genes have been found in different plants: 8 in rice (Oryza sativa; Yao et al. 2007), 12 in tomato (Xu and Shi 2006), 13 in Arabidopsis (Arabidopsis thaliana; Rosenquist et al. 2001), and 17 in N. tabacum (Konagaya et al. 2004). Increasing evidence has shown that 14-3-3s play essential roles in plant–pathogen interactions (Lozano-Duran and Robatzek 2015). The expressions of 14-3-3 genes in wheat (Triticum aestivum), rice, maize (Zea mays), and many other plant species are induced during responses to various pathogen infections (Konagaya et al. 2004; Manickavelu et al. 2010; Manosalva et al. 2011; Campo et al. 2012; Carrasco et al. 2014; Hartman and Hirsch 2017). 14-3-3 proteins can affect pathogen infections by interacting with host defense-related proteins or acting as targets of pathogen effectors (Konagaya et al. 2004; Chang et al. 2009; Kim et al. 2009; Yang et al. 2009; Oh et al. 2010; Taylor et al. 2012; Teper et al. 2014). For example, tomato 14-3-3 protein 7 interacts with MAPKKKα to positively regulate host programmed cell death (Oh et al. 2010). Konagaya et al. (2004) have shown that 14-3-3 can function as a scaffold or an adaptor between N. tabacum N protein and the helicase domain of TMV replicase protein; Gao et al. (2022) have shown that N. benthamiana 14-3-3a can positively regulate host defense against BBSV infection. However, how plant viruses target 14-3-3 to subvert host antiviral defense remains to be fully understood.
Potyviruses, which form the largest plant RNA virus group, cause severe diseases in many economically important crops (Yang et al. 2021). Genomes of potyviruses are positive-sense single-stranded RNAs and usually encode 2 polyproteins that are further processed into 11 mature proteins by 3 viral proteinases, the first protein (P1), the helper component-proteinase, and the nuclear inclusion protein a-proteinase (Revers and García 2015; Wang 2021). The first 6 kilodalton protein (6K1) is one of the smallest viral proteins of potyviruses (Waltermann and Maiss 2006). Cui and Wang (2016) have shown that plum pox virus (PPV) 6K1 is an essential element of the VRC and is required for virus replication. TuMV 6K1 can reduce host protease activity to promote virus accumulation (Bera et al. 2022). Our previous results have shown that tobacco vein banding mosaic virus (TVBMV) 6K1 can be recruited to VRC by the 6K2 protein (Geng et al. 2017). To date, how 6K1 regulates potyvirus infection in plants remains largely unknown.
In this study, we investigated the roles of the Nb14-3-3h and NbTCTP in PVY infection. Our results showed that overexpression of Nb14-3-3h in N. benthamiana plants suppressed PVY replication while silencing of Nb14-3-3h enhanced PVY accumulation. We also found that dimeric Nb14-3-3h inhibited PVY infection via sequestering the susceptibility factor NbTCTP. Further, 6K1 can subvert Nb14-3-3h–mediated antiviral defense via interfering with Nb14-3-3h and NbTCTP interaction to promote virus infection.
Results
Silencing of TCTP inhibits PVY replication
TCTP has been reported to participate in potyvirus infection in N. benthamiana and S. lycopersicum plants (Bruckner et al. 2017, 2021). To investigate the role of TCTP during PVY infection, we silenced NbTCTP in N. benthamiana plants through tobacco rattle virus (TRV) –based virus-induced gene silencing (VIGS) and then inoculated the NbTCTP-silenced (TRV-TCTP) and nonsilenced (TRV-GUS) control plants with PVY-GFP through agro-infiltration (Fig. 1, A to C). At 4 d post-PVY-GFP inoculation, weaker GFP fluorescence was observed in the systemic leaves of the plants infected with TRV-NbTCTP compared with the control plants (Fig. 1, D and E). Western blot results showed that the accumulation levels of PVY coat protein (CP) in the NbTCTP-silenced plants were 56% of those in the control plants at 4 d postinoculation (dpi; Fig. 1F). To further confirm the role of NbTCTP in PVY replication, we infiltrated NbTCTP-silenced and nonsilenced control plants with a cell-to-cell movement deficient of PVY-GFP replicon (referred to as PVYrep-GFP thereafter). The results of reverse transcription quantitative PCR (RT-qPCR) showed that the accumulation levels of PVYrep-GFP genomic (+) and minus-strand (−) RNAs in the NbTCTP-silenced plants were about 40% of those in the control plants (Fig. 1G), indicating that silencing of NbTCTP inhibited PVY replication. To further confirm this finding, we inoculated the TCTP knockdown (NtTCTP-KD, lines R16 and R20) and TCTP overexpression (NtTCTP-OE, lines O2 and O7) transgenic N. tabacum lines with PVY-GFP (Fig. 1, H and I). Compared with the wild-type N. tabacum and the NtTCTP-OE plants, the PVY-GFP–inoculated NtTCTP-KD plants showed weaker GFP fluorescence in their systemic leaves and accumulated less CP at 5 dpi (Fig. 1, H to J), indicating the proviral role of TCTP might apply to more pathosystems. These data suggest that TCTP of both N. benthamiana and N. tabacum is a susceptibility factor and positively regulates PVY infection.
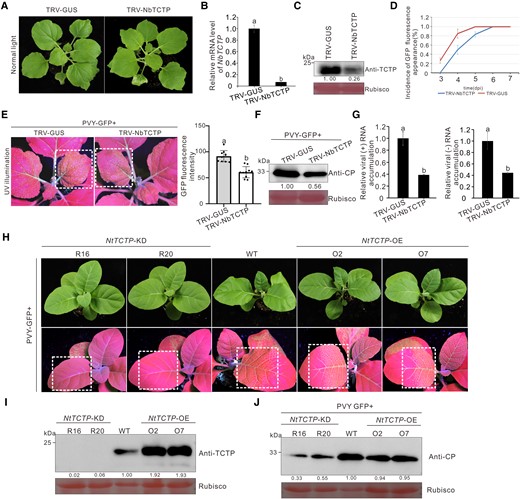
Silencing of TCTP gene inhibited PVY infection in N. benthamiana. A) The phenotype of N. benthamiana plants infected with TRV-NbTCTP or TRV-GUS was photographed at 12 dpi. B) The relative expression levels of NbTCTP in TRV-NbTCTP–inoculated and mock-inoculated N. benthamiana plants were determined through RT-qPCR. The data represented the means ± Sd from 3 biological replicates and 3 technical replicates. Statistical significance between the 2 treatments was determined using Duncan's multiple-range test. Different letters represented significant differences (P < 0.05). C) Western blot analysis of the TCTP accumulation levels in N. benthamiana plants systematically infected with TRV-NbTCTP or TRV-GUS at 12 dpi using a TCTP-specific antibody. The Rubisco large subunit protein was used as a loading control. Protein band intensities were measured using the ImageJ software. Numbers indicated the accumulation levels of TCTP normalized to Rubisco staining. D) Incidence of plants with GFP fluorescence at various days post PVY-GFP inoculation. The error bars represented the means ± Sd from 3 independent experiments and each treatment had 10 plants. E) The phenotype of PVY-GFP in TCTP-silenced and mock-inoculated N. benthamiana plants. The plants were photographed under UV illumination at 4 d post PVY-GFP inoculation. The GFP fluorescence intensity derived from PVY-GFP as shown in dotted rectangle regions was measured using the ImageJ software. The bar graphs represented the means ± Sd (n = 10). Statistical significance between the 2 treatments was determined using Duncan's multiple-range test. Different letters represented significant differences (P < 0.05). F) The accumulation levels of PVY-GFP in the TCTP-silenced and mock-inoculated N. benthamiana plants at 4 dpi. The Rubisco large subunit protein was used as a loading control. Band intensities were measured using the ImageJ software. Numbers indicated the accumulation levels of PVY CP normalized to Rubisco staining. G) Accumulation levels of PVY-GFP replicon (referred to as PVYrep-GFP thereafter) genomic (+) and minus-strand (−) RNAs in the TRV-NbTCTP–inoculated or the TRV-GUS–inoculated plants were determined through RT-qPCR. The error bars represented the means ± Sd from 3 biological replicates and 3 technical replicates. Statistical significance between the 2 treatments was determined using Duncan's multiple-range test. Different letters represented significant differences (P < 0.05). H) The phenotype of PVY-GFP in N. tabacum TCTP-knockdown (NtTCTP-KD) and overexpression (NtTCTP-OE) transgenic lines. The plants were photographed under UV illumination at 5 d post PVY-GFP inoculation. I and J) The protein levels of TCTP in the transgenic N. tabacum plants, and the accumulation levels of CP in the leaves systematically infected with PVY-GFP were determined through Western blot assay using a TCTP-specific or CP-specific antibody. The Rubisco large subunit protein was used as a loading control. Protein band intensities were measured using the ImageJ software. Numbers indicated the accumulation levels of TCTP and PVY CP normalized to Rubisco staining, and the WT band in each was set to 1. The experiments were repeated 3 times independently.
Nb14-3-3h interacts with NbTCTP
In Drosophila, 14-3-3 interacts with TCTP to modulate organ growth through the TOR pathway (Le et al. 2016). To investigate whether Nb14-3-3h could interact with NbTCTP in plants, we transiently coexpressed NbTCTP-Myc (3×Myc fused to the C-terminus of NbTCTP) and eGFP N-terminally or C-terminally tagged Nb14-3-3h in N. benthamiana leaves through agro-infiltration. In the co-immunoprecipitation (Co-IP) assay, NbTCTP-Myc was immunoprecipitated by eGFP-Nb14-3-3h or Nb14-3-3h-eGFP, but not by eGFP (Fig. 2A). We then analyzed the interaction between Nb14-3-3h-Nluc (Nluc fused to the C-terminus of Nb14-3-3h) and Cluc-NbTCTP (Cluc fused to the N-terminus of NbTCTP) through luciferase complementation imaging (LCI) assay in N. benthamiana leaves. The results showed that the luciferase activity in the leaf patches coexpressing Nb14-3-3h-Nluc and Cluc-NbTCTP was strong, while the controls showed no luciferase signal (Fig. 2B). In the bimolecular fluorescence complementation (BiFC) assay, YFP fluorescence was only observed in N. benthamiana cells that coexpressed NbTCTP-nYFP (nYFP fused to the C-terminus of NbTCTP) and Nb14-3-3h-cYFP (cYFP fused to the C-terminus of Nb14-3-3h) in the context of PVY infection (Fig. 2C). Similarly, we found that NbTCTP-eGFP (eGFP fused to the C-terminus of NbTCTP) colocalized with Nb14-3-3h-DsRed (DsRed fused to the C-terminus of Nb14-3-3h) in the cytoplasm of N. benthamiana leaf cells (Fig. 2D). In addition, pull-down assay results showed that eGFP-NbTCTP (eGFP fused to the N-terminus of NbTCTP) was captured by GST-Nb14-3-3h (GST fused to the N-terminus of Nb14-3-3h) in vitro, but not by GST (Fig. 2E). These results indicate that Nb14-3-3h can interact with NbTCTP in vivo and in vitro.
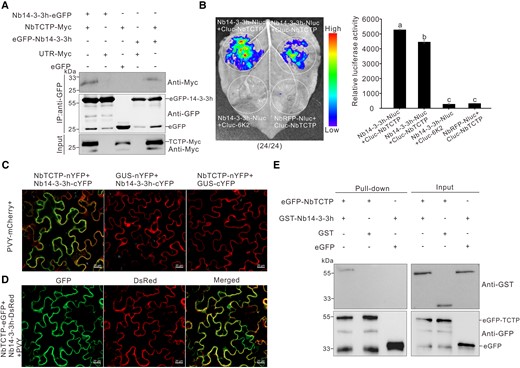
Nb14-3-3h can interact with NbTCTP in vivo and in vitro. A) Co-IP analysis of the interaction between Nb14-3-3h and NbTCTP. The N. benthamiana leaf tissues expressing proteins were harvested at 3 dpi. Total proteins were extracted and immunoprecipitated with GFP-Trap beads. The immunoprecipitated protein (IP) and input samples were analyzed through Western blot assay using a GFP-specific or Myc-specific antibody. B) LCI analysis of the interaction between Nb14-3-3h and NbTCTP at 2 dpi. The N. benthamiana leaves were infiltrated with various Agrobacterium cultures, as indicated in the figure. The combinations of Nb14-3-3h-Nluc and Cluc-6K2 or Ring finger protein-Nluc and Cluc-NbTCTP were controls. The dotted circles indicated the infiltrated regions. The numbers represented the ratio of leaves with similar phenotypes as shown in the picture to the total number of leaves in 3 experiments. Quantification of luciferase activity from various infiltrated N. benthamiana leaf patches as shown in dotted circle regions using ImageJ software. The results were presented as the means ± Sd from 3 biological replicates. Statistical significance was determined using Duncan's multiple-range test. Different letters represented significant differences (P < 0.05). C) BiFC assay showed that Nb14-3-3h interacted with NbTCTP in N. benthamiana leaves systematically infected with PVY. The mCherry fluorescence was used to monitor the virus infection. The GFP fluorescence indicated the interaction signal. NbTCTP-nYFP and Nb14-3-3h-cYFP were coexpressed in the symptomatic leaves 5 d after PVY-mCherry inoculation, and the infiltrated leaves were examined under a confocal microscope at 2 dpi. Scale bars = 20 μm. D) Confocal images of N. benthamiana leaf cells coexpressing NbTCTP-eGFP, Nb14-3-3h-DsRed, and PVY at 3 dpi. Scale bars = 20 μm. E) Pull-down assay showed the interaction between Nb14-3-3h and NbTCTP. GST-Nb14-3-3h, GST, eGFP-NbTCTP, and eGFP were purified from Rosetta (DE3), mixed in various combinations, and immunoprecipitated with GFP-Trap beads. The IP and input samples were then analyzed through Western blot assay using a GFP-specific or GST-specific antibody.
Nb14-3-3h restricts PVY replication
We found Nb14-3-3h was significantly upregulated at the early stage of PVY infection (Supplemental Fig. S1A). To investigate the role of Nb14-3-3h, we mechanically inoculated the leaves overexpressing Nb14-3-3h-Myc (3×Myc fused to the C-terminus of Nb14-3-3h) and Rluc-Myc (3×Myc fused to the C-terminus of Rluc) with PVY-GFP. At 4 dpi, the area of infection sites derived from PVY-GFP in leaf patches overexpressing Nb14-3-3h-Myc was 64% of those in leaf patches overexpressing Rluc-Myc (Fig. 3A). And, the PVY CP and RNA accumulation levels were about half of those in leaf patches overexpressing Rluc-Myc (Fig. 3, B and C). To further confirm the role of Nb14-3-3h in PVY replication, we infiltrated N. benthamiana leaves overexpressing Nb14-3-3h-Myc or empty vector (EV) with PVYrep-GFP. The results showed that compared with the control leaf patches expressing EV, the leaves overexpressing Nb14-3-3h-Myc showed significantly reduced GFP fluorescence and accumulated less GFP protein at 4 dpi (Supplemental Fig. S1, B and C), suggesting that overexpression of Nb14-3-3h inhibits PVY replication. We also obtained Nb14-3-3h-HA overexpressing transgenic N. benthamiana plants, which showed no obvious growth defect. Then, we inoculated the wild-type and Nb14-3-3h overexpressing N. benthamiana plants with PVY-GFP. At 5 dpi, PVY induced less GFP fluorescence in Nb14-3-3h-OE plants than in the control plants (Fig. 3E). Consistently, the accumulation levels of PVY CP and RNAs in Nb14-3-3h-OE plants were 38% to 46% of those in the wild-type plants (Fig. 3, D and F).
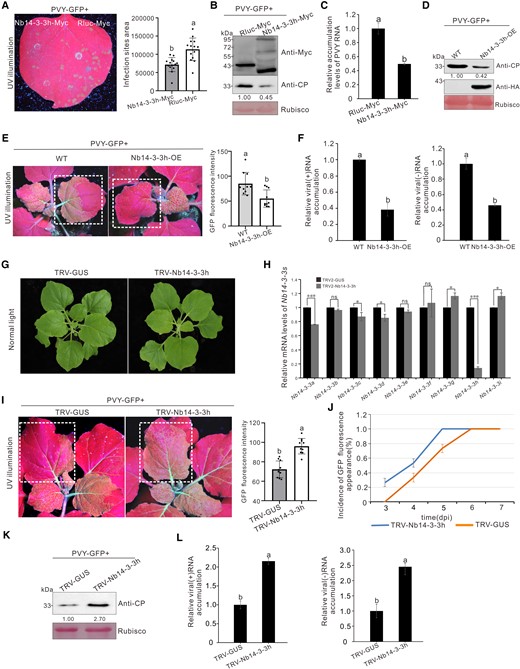
Overexpression of Nb14-3-3h–suppressed PVY infection. A) PVY-GFP infection sites at 4 dpi in the presence of Nb14-3-3h-Myc in one half of the leaf and of Rluc-Myc in the other half. The area of PVY-GFP infection sites was analyzed using the ImageJ software. The bar graph represented the means ± Sd, and n represented the number of leaves in each experiment (n = 14). Statistical significance between the 2 treatments was determined using Duncan's multiple-range test. Different letters represented significant differences (P < 0.05). B) Analysis of the Nb14-3-3h and virus accumulation levels through Western blot assay using a Myc-specific or CP-specific antibody. The Rubisco large subunit protein was used as a loading control. Protein band intensities were measured using the ImageJ software. Numbers indicated the accumulation levels of PVY CP normalized to Rubisco staining, and the left band was set to 1. C) Determination of the PVY RNA accumulation levels through RT-qPCR. The results were presented as the means ± Sd from 3 biological replicates and 3 technical replicates. Statistical significance between the 2 treatments was determined using Duncan's multiple-range test. Different letters represented significant differences (P < 0.05). D) Western blot analysis of PVY and Nb14-3-3h accumulation levels in wild-type and Nb14-3-3h overexpression plants as shown in E). The Rubisco large subunit protein was used to show sample loading. Protein band intensities were measured using the ImageJ software. Numbers indicated the accumulation levels of PVY CP normalized to Rubisco staining, and the left band was set to 1. E) GFP fluorescence in PVY-GFP infected wild-type and Nb14-3-3h-OE transgenic N. benthamiana plants. The plants were photographed at 5 d post PVY-GFP inoculation. The GFP fluorescence intensity derived from PVY-GFP as shown in dotted rectangle regions was measured using the ImageJ software. The bar graphs represented the means ± Sd (n = 10). Statistical significance between the 2 treatments was determined using Duncan's multiple-range test. Different letters represented significant differences (P < 0.05). F) Analysis of PVYrep-GFP genomic (+) and minus-strand (−) RNA accumulation levels in wild-type and Nb14-3-3h-OE plants. The bar graphs represented the means ± Sd from 3 biological replicates and 3 technical replicates. Statistical significance between the 2 treatments was determined using Duncan's multiple-range test. Different letters represented significant differences (P < 0.05). G) The phenotype of Nb14-3-3h–silenced (TRV-Nb14-3-3h) and nonsilenced (TRV-GUS) plants at 12 dpi. H) Analysis of different Nb14-3-3 isoform expression levels in the Nb14-3-3h–silenced (TRV-Nb14-3-3h) and nonsilenced (TRV-GUS) plants through RT-qPCR. Total RNA was isolated from systematically infected leaves at 12 dpi. The RT-qPCR analysis was done using gene-specific primers. The error bars indicated the means ± Sd of 3 biological replicates and 3 technical replicates, and statistical significance between the 2 treatments was determined using paired Student's t-test: *P < 0.05, **P < 0.01, ***P < 0.001. I) GFP fluorescence in the PVY-GFP infected Nb14-3-3h–silenced or nonsilenced N. benthamiana plants. The plants were photographed at 5 d post PVY-GFP inoculation. The GFP fluorescence intensity derived from PVY-GFP as shown in dotted rectangle regions was measured using the ImageJ software. The bar graphs represented the means ± Sd (n = 10). Statistical significance between the 2 treatments was determined using Duncan's multiple-range test. Different letters represented significant differences (P < 0.05). J) Incidence of plants showing GFP fluorescence at 3, 4, 5, and 6 d post PVY-GFP inoculation. Error bars indicated mean ± Sd from 3 independent experiments, and each treatment had 10 plants. K) Western blot analysis of PVY CP accumulation levels in the TRV-Nb14-3-3h–inoculated or TRV-GUS–inoculated plants at 5 dpi. The Rubisco large subunit protein was used as a loading control. Band intensities were measured using the ImageJ software. Numbers indicated the accumulation levels of PVY CP normalized to Rubisco staining. L) Analysis of PVYrep-GFP (+) and (−) RNA accumulation levels in the TRV-Nb14-3-3h–inoculated and the TRV-GUS–inoculated plants. The results were presented as the means ± Sd from 3 biological replicates and 3 technical replicates. Statistical significance between the 2 treatments was determined using Duncan's multiple-range test. Different letters represented significant differences (P < 0.05).
To further investigate the role of Nb14-3-3h in virus infection, we silenced its expression in N. benthamiana plants by using a TRV-based vector carrying an insert representing the 3′-UTR of Nb14-3-3h. At 12 dpi, neither of the plants showed an abnormal phenotype (Fig. 3G). To verify the silencing efficiency of Nb14-3-3h, we analyzed the Nb14-3-3h mRNA accumulation levels in systemic leaves infected with TRV-Nb14-3-3h or TRV-GUS at 12 dpi. The RT-qPCR results showed that the levels of Nb14-3-3h mRNA in N. benthamiana plants infected with TRV-Nb14-3-3h were 15% of the control plants (Fig. 3H). Then, all the N. benthamiana plants were inoculated with PVY-GFP. Under the UV illumination, PVY spread more rapidly, and much more GFP fluorescence was observed in the systemic leaves of Nb14-3-3h–silenced plants than those of the control plants at 5 dpi (Fig. 3, I and J). The results of Western blot showed that the accumulation levels of PVY CP in Nb14-3-3h–silenced plants were 2.7 times higher than those in the control plants (Fig. 3K). The RT-qPCR results indicated that the accumulation levels of PVYrep-GFP genomic (+) and minus-strand (−) RNAs in Nb14-3-3h–silenced plants were about twice higher than those in the control plants (Fig. 3L). These results indicate that 14-3-3h restricts PVY infection in N. benthamiana plants.
Amino acid residues LAE, S63, and Y89 are essential for Nb14-3-3h dimerization and restricting PVY replication
The 14-3-3 proteins are known to form homodimers and heterodimers (Brandwein and Wang 2017), and their dimerization is important for 14-3-3s in regulating plant growth and development and responses to pathogen invasions (Lozano-Duran and Robatzek 2015). To investigate whether Nb14-3-3h can dimerize, we transiently overexpressed Nb14-3-3h-Myc in N. benthamiana leaves. At 3 dpi, total proteins were extracted from leaf samples and analyzed through a Western blot assay. The results showed that Nb14-3-3h-Myc did form dimers and tetramers in vivo (Fig. 4B). Several conserved amino acids that determine the dimerization of human 14-3-3ζ have been identified (Liu et al. 1995; Tzivion et al. 1998; Gu et al. 2006). We then constructed a series of dimerization-deficient mutants: Nb14-3-3hE7K, Nb14-3-3hQQR (the amino acids at 14-LAE-16 were mutated to QQR), Nb14-3-3hS63E, Nb14-3-3hY89Q, Nb14-3-3hK92N, and Nb14-3-3hE94Q (Supplemental Fig. S2A), among which mutants Nb14-3-3hQQR, Nb14-3-3hS63E, and Nb14-3-3hY89Q almost completely lost the ability of dimerization (Fig. 4, B, E, and H; Supplemental Fig. S2B). To determine whether disruption of Nb14-3-3h dimerization affects the antiviral role of Nb14-3-3h, we inoculated these N. benthamiana leaf patches overexpressing wild-type Nb14-3-3h-Myc and Nb14-3-3hQQR, Nb14-3-3hS63E, or Nb14-3-3hY89Q with PVYrep-GFP. By 5 d after PVYrep-GFP inoculation, the leaf patches coexpressing Nb14-3-3h and PVYrep-GFP showed very weak GFP fluorescence, while the leaves coexpressing the Nb14-3-3h mutants and PVYrep-GFP showed strong GFP fluorescence (Fig. 4, A, D, and G). The results of Western blot and RT-qPCR indicated that the accumulation levels of PVY CP and RNAs in the leaf patches overexpressing Nb14-3-3hQQR, Nb14-3-3hS63E-Myc, or Nb14-3-3hY89Q were 1.8 to 4.3 times higher than those in the leaf patches overexpressing Nb14-3-3h-Myc (Fig. 4, B, C, E, F, H, and I), indicating that dimerization is critical for Nb14-3-3h antiviral activity. We observed that the representative image was not reproduced in all of the leaves (Fig. 4, A, D, and G), which might be due to the differences in the physiological state of N. benthamiana leaves. To further confirm the role of Nb14-3-3h dimerization in PVY infection, we mechanically inoculated the leaves overexpressing wild-type Nb14-3-3h-Myc and the mutants Nb14-3-3hQQR-Myc or Nb14-3-3hS63E-Myc with PVY-GFP. At 4 dpi, more infection sites derived from PVY-GFP were observed in leaf patches overexpressing Nb14-3-3hQQR-Myc or Nb14-3-3hS63E-Myc compared with Nb14-3-3h-Myc, respectively (Supplemental Fig. S3, A and D). The Western blot and RT-qPCR results indicated that the accumulation levels of PVY CP and RNAs in leaf patches over-expressing Nb14-3-3hQQR-Myc or Nb14-3-3hS63E-Myc were 1.5 to 1.7 times higher than those in the leaf patches overexpressing Nb14-3-3h-Myc (Supplemental Fig. S3, B, C, E, and F). These results further demonstrate that dimeric Nb14-3-3h functions in restricting PVY infection.
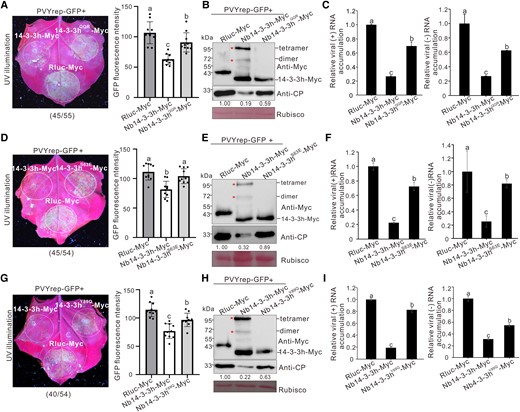
The mutations of QQR, S63E, and Y89Q influenced the antiviral function of Nb14-3-3h. A, D, and G) PVYrep-GFP fluorescence in the N. benthamiana leaf patches transiently overexpressing Nb14-3-3h-Myc, 3 dimerization mutants (Nb14-3-3hQQR-Myc, Nb14-3-3hS63E-Myc, and Nb14-3-3hY89Q-Myc, respectively), and Rluc-Myc. The leaves were photographed 5 d post PVYrep-GFP inoculation, and dotted circles indicated the infiltrated regions. The numbers below images represented the ratio of leaves with similar phenotypes as shown in the figure to the total number of leaves in 3 experiments. The GFP fluorescence intensity derived from PVYrep-GFP as shown in dotted circle regions was measured using the ImageJ software. The bar graphs represented the means ± Sd (n = 10). Statistical significance was determined using Duncan's multiple-range test. Different letters represented significant differences (P < 0.05). B, E, and H) Total proteins were extracted from leaf patches and analyzed for Nb14-3-3h and virus accumulation levels through Western blot assay using a Myc-specific or CP-specific antibody. The Rubisco large subunit protein was used as a loading control. Protein band intensities were measured using the ImageJ software. Numbers indicated the accumulation levels of PVY CP normalized to Rubisco staining, and the left band was set to 1. C, F, and I) Total RNAs were extracted from the leaf patches shown in A), D), and G) and analyzed for PVY (+) and (−) RNA accumulation levels through RT-qPCR. The results were presented as the means ± Sd from 3 biological replicates and 3 technical replicates. Statistical significance was determined using Duncan's multiple-range test. Different letters represented significant differences (P < 0.05).
Dimeric Nb14-3-3h inhibits PVY infection via sequestering NbTCTP
To explore the biological relevance of the interaction between Nb14-3-3h and NbTCTP in PVY infection, by using LCI assay, we screened a series of Nb14-3-3h mutants and found that residue Glu at position 16 (E16) of Nb14-3-3h was required for its interaction with NbTCTP (Supplemental Fig. S4A). Results of Co-IP and pull-down assays showed that Nb14-3-3hE16A did not interact with NbTCTP (Supplemental Fig. S4, B and C). However, like wild-type Nb14-3-3h-Myc, mutant Nb14-3-3hE16A-Myc can self-interact (Supplemental Fig. S5, A and B). To explore whether this mutation influences the antiviral ability of Nb14-3-3h, we inoculated PVYrep-GFP to N. benthamiana leaves transiently overexpressing wild-type Nb14-3-3h or mutant Nb14-3-3hE16A through agro-infiltration. Four days after agro-infiltration, stronger GFP fluorescence from PVYrep-GFP was observed in the leaf regions overexpressing Nb14-3-3hE16A-Myc than that in the leaf regions overexpressing Nb14-3-3h-Myc (Fig. 5A). The results of RT-qPCR showed that the accumulation levels of PVY (+) RNAs and (−) RNAs in the leaf patches overexpressing Nb14-3-3hE16A-Myc were 2.0 to 3.7 times higher than those in the patches overexpressing Nb14-3-3h-Myc (Fig. 5B). The Western blot results showed that the accumulation levels of PVY CP in the leaf patches overexpressing Nb14-3-3hE16A-Myc were about 3.6 times higher than those in the patches overexpressing Nb14-3-3h-Myc (Fig. 5C). In addition, we mechanically inoculated the leaves overexpressing wild-type Nb14-3-3h-Myc and Nb14-3-3hE16A-Myc with PVY-GFP. The area of infection sites derived from PVY-GFP in the leaf patches overexpressing Nb14-3-3hE16A-Myc was 1.4 times higher than those in the leaf patches overexpressing Nb14-3-3h-Myc (Supplemental Fig. S4D). And, the Western blot and RT-qPCR results indicated that the accumulation levels of PVY CP and RNA were 1.5 to 1.8 times higher than those in leaf patches overexpressing Nb14-3-3h-Myc (Supplemental Fig. S4, E and F). Therefore, the mutation of glutamic acid at position 16 to alanine in Nb14-3-3h compromises its interaction with NbTCTP and its activity in mediating antiviral defense.
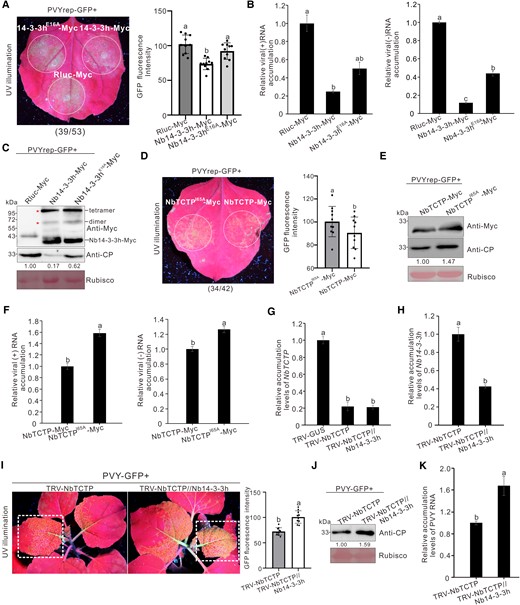
Nb14-3-3h restricted PVY infection via sequestering NbTCTP. A) PVYrep-GFP fluorescence in the N. benthamiana leaf patches transiently overexpressing Nb14-3-3h-Myc and Nb14-3-3hE16A-Myc. Nb14-3-3h-Myc, Nb14-3-3hE16A-Myc, and Rluc-Myc were individually transiently overexpressed in N. benthamiana leaf patches through agro-infiltration. Three days later, these infiltrated leaves were inoculated again with PVYrep-GFP and photographed 4 d post–PVYrep-GFP inoculation. The dotted circles indicated the PVYrep-GFP infiltrated regions. The numbers below the image represented the ratio of leaves with similar phenotypes as shown in the figure to the total number of leaves in 3 experiments. The GFP fluorescence intensity derived from PVYrep-GFP as shown in the dotted circle regions was measured using the ImageJ software. The bar graphs represented the means ± Sd (n = 10). Statistical significance between the 3 treatments was determined using Duncan's multiple-range test. Different letters represented significant differences (P < 0.05). B) Accumulation levels of PVY (+) and (−) RNAs in infiltrated N. benthamiana leaf patches as shown in A) were analyzed through RT-qPCR. The error bars represented the means ± Sd from 3 biological replicates and 3 technical replicates. Statistical significance between the 3 treatments was determined using Duncan's multiple-range test. Different letters represented significant differences (P < 0.05). C) Analysis of Nb14-3-3hE16A-Myc and PVY CP accumulation levels through Western blot assay with a Myc-specific or CP-specific antibody. The Rubisco large subunit protein was used as a loading control. Protein band intensities were measured using the ImageJ software. Numbers indicated the accumulation levels of PVY CP normalized to Rubisco staining, and the left band was set to 1. D) GFP fluorescence derived from PVYrep-GFP in N. benthamiana leaves expressing NbTCTP-Myc or NbTCTPI65A-Myc. The numbers below the image represented the ratio of leaves with similar phenotypes as shown in the figure to the total number of leaves in 3 experiments. The GFP fluorescence intensity as shown in the dotted circle regions was measured using ImageJ software. The bar graphs represented the means ± Sd (n = 10). Statistical significance between the 2 treatments was determined using Duncan's multiple-range test. Different letters represented significant differences (P < 0.05). E) Analysis of the NbTCTP and PVY CP accumulation levels through Western blot assay with a Myc-specific or CP-specific antibody. The Rubisco large subunit protein was used as a loading control. Protein band intensities were measured using the ImageJ software. Numbers indicated the accumulation levels of PVY CP normalized to Rubisco staining. F) RT-qPCR analysis of the accumulation levels of PVY (+) and (−) RNAs in infiltrated N. benthamiana leaf patches as shown in D). The error bars represented the means ± Sd from 3 biological replicates and 3 technical replicates. Statistical significance between the 2 treatments was determined using Duncan's multiple-range test. Different letters represented significant differences (P < 0.05). G and H) RT-qPCR analysis of the relative accumulation levels of NbTCTPG) or Nb14-3-3hH) in the systematic leaves infected with TRV-NbTCTP and TRV-NbTCTP//Nb14-3-3h at 12 dpi. The error bars represented the means ± Sd from 3 biological replicates and 3 technical replicates. Statistical significance was determined using Duncan's multiple-range test. Different letters represented significant differences (P < 0.05). I) GFP fluorescence of NbTCTP-silenced and NbTCTP//Nb14-3-3h–silenced plants was photographed at 5 d post challenge inoculation with PVY-GFP under UV illumination. The GFP fluorescence intensity as shown in dotted rectangle regions was measured using ImageJ software. The bar graphs represented the means ± Sd (n = 10). Statistical significance between the 2 treatments was determined using Duncan's multiple-range test. Different letters represented significant differences (P < 0.05). J) Western blot analysis of the PVY CP accumulation levels in NbTCTP-silenced or NbTCTP//Nb14-3-3h–silenced plants. The Rubisco large subunit protein was used as a loading control. Protein band intensities were measured using the ImageJ software. Numbers indicated the accumulation levels of PVY CP normalized to Rubisco staining, and the left band was set to 1. K) RT-qPCR analysis of the accumulation levels of PVY RNA in NbTCTP-silenced or NbTCTP//Nb14-3-3h–silenced plants. The error bars represented the means ± Sd from 3 biological replicates and 3 technical replicates. Statistical significance between the 2 treatments was determined using Duncan's multiple-range test. Different letters represented significant differences (P < 0.05).
In addition, we identified that the residue Ile at position 65 (I65) of NbTCTP was necessary for its interaction with Nb14-3-3h. The results of LCI, Co-IP, and pull-down assays showed that NbTCTPI65A was unable to interact with Nb14-3-3h (Supplemental Fig. S6). To further investigate the role of Nb14-3-3h-NbTCTP interaction during PVY infection, we inoculated PVYrep-GFP to N. benthamiana leaves transiently overexpressing NbTCTP-Myc and NbTCTPI65A-Myc. Stronger GFP fluorescence was observed in NbTCTPI65A-Myc overexpressing leaf patches at 4 dpi (Fig. 5D). The results of Western blot and RT-qPCR showed that the PVY CP and RNA accumulation levels in the leaf patches overexpressing NbTCTPI65A-Myc were 1.3 to 1.6 times higher than those in the leaf patches overexpressing NbTCTP-Myc (Fig. 5, E and F). Silencing NbTCTP suppresses PVY replication, while Nb14-3-3h interacts with NbTCTP and prevents its proviral function. To test whether silencing Nb14-3-3h can alleviate the inhibition of NbTCTP, we simultaneously silence NbTCTP and Nb14-3-3h using pTRV1 and pTRV2-NbTCTP//Nb14-3-3h. At 12 dpi, the RT-qPCR results showed that the accumulation levels of NbTCTP in N. benthamiana plants infected with TRV-NbTCTP was about 22% of the TRV-GUS control, and the accumulation levels of NbTCTP and Nb14-3-3h mRNAs in N. benthamiana plants infected with TRV-NbTCTP//Nb14-3-3h were about 21% and 43% of the control plants, respectively (Fig. 5, G and H). Compared with the plants in which NbTCTP was silenced alone, GFP fluorescence derived from PVY-GFP in the systematical leaves of both NbTCTP- silenced and Nb14-3-3h–silenced (NbTCTP//Nb14-3-3h) plants was significantly stronger at 5 dpi (Fig. 5I). The results of Western blot and RT-qPCR showed that PVY CP and RNA accumulation levels in the NbTCTP//Nb14-3-3h–silenced plants were 1.6 times higher than those in the control plants (Fig. 5, J and K), suggesting that silencing of Nb14-3-3h can alleviate its inhibition of NbTCTP and facilitate PVY infection on NbTCTP-silenced plants. These results indicate that Nb14-3-3h can sequester NbTCTP and prevent its proviral function to inhibit PVY infection.
PVY 6K1 can interact with Nb14-3-3h in vivo and in vitro
Nb14-3-3h was identified in the 6K1 interacting protein complex through liquid chromatography-tandem MS (LC-MS/MS) assay. We then cloned Nb14-3-3h gene into a transient expression vector p35SUTR-Myc and tested whether Nb14-3-3h interacts with 6K1 through a Co-IP assay. The results showed that 6K1-eGFP (eGFP fused to the C-terminus of 6K1) interacted with Nb14-3-3h-Myc in vivo (Fig. 6A). To further verify whether Nb14-3-3 interacts with 6K1 in vitro, we expressed and purified eGFP-Nb14-3-3h (eGFP fused to the N-terminus of Nb14-3-3h) and 6K1-GST (GST fused to the C-terminus of 6K1) proteins in Escherichia coli (Rosetta, DE3) cells and analyzed their interaction through GST pull-down assay. Results showed that eGFP-Nb14-3-3h was captured by 6K1-GST (Fig. 6B). To confirm whether Nb14-3-3h interacts with PVY 6K1 in the context of virus infection, we performed BiFC assay. Nb14-3-3h-nYFP (nYFP fused to the C-terminus of Nb14-3-3h) and 6K1-cYFP (cYFP fused to the C-terminus of 6K1) were coexpressed in N. benthamiana leaves systemically infected with PVY-mCherry, and the red fluorescence from PVY-mCherry was used to monitor the virus infection. The YFP fluorescence was observed in PVY-infected N. benthamiana cells coexpressing the Nb14-3-3h-nYFP and 6K1-cYFP at 2 dpi (Fig. 6C). To determine the subcellular localization of Nb14-3-3h and 6K1 during PVY infection, we inoculated the PVY-6K1-GFP systemically infected N. benthamiana leaves using Agrobacterium strain GV3101 harboring Nb14-3-3h-DsRed, and the results showed that Nb14-3-3h-DsRed colocalized with 6K1-GFP (GFP fused to the C-terminus of 6K1) in the cytoplasm of virus-infected cells (Fig. 6D). These results indicate that PVY 6K1 interacts with Nb14-3-3h. Interestingly, Co-IP and GST pull-down assays showed that 3 dimerization defective mutants, Nb14-3-3hS63E, Nb14-3-3hQQR, and Nb14-3-3hY89Q, failed to coimmunoprecipitate with 6K1, indicating that the amino acid residues that determine Nb14-3-3h dimerization and Nb14-3-3h-6K1 interaction are the same (Fig. 6, E and F).
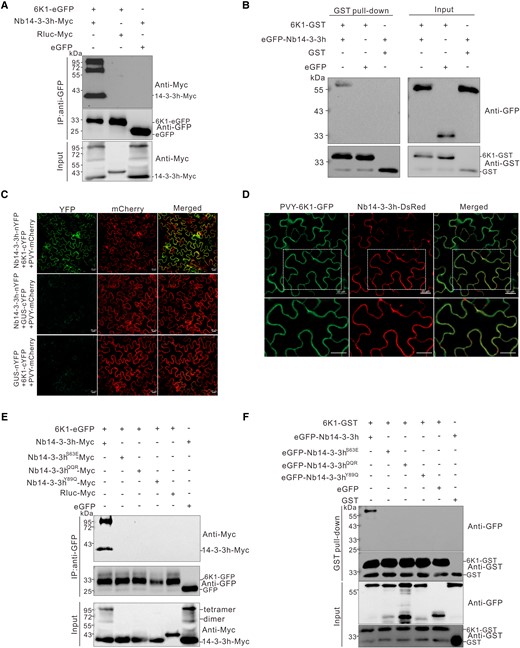
PVY 6K1 interacted with Nb14-3-3h. A) Co-IP analysis of the interaction between 6K1 and Nb14-3-3h. The 6K1-eGFP was coexpressed with Nb14-3-3h-Myc in N. benthamiana leaves, and the infiltrated leaves were harvested at 3 dpi. Total proteins were extracted and immunoprecipitated with GFP-Trap beads from individual samples followed by protein gel analysis using a GFP-specific or Myc-specific antibody. B) Analysis of the interaction between 6K1 and Nb14-3-3h in vitro. Purified eGFP-Nb14-3-3h and eGFP were coincubated with 6K1-GST or GST in different combinations. After being immunoprecipitated with GST-Trap beads, the precipitate was analyzed through Western blot using a GFP-specific or GST-specific antibody. C) BiFC analysis of the interaction between Nb14-3-3h and 6K1 in N. benthamiana leaves systematically infected with PVY-mCherry. Nb14-3-3h-nYFP and CUS-cYFP or GUS-nYFP and 6K1-cYFP were coexpressed as negative controls. The mCherry fluorescence was used to monitor the virus infection. The infiltrated leaves were examined under a confocal microscope at 2 dpi. The experiments were repeated 3 times independently with similar results. Scale bars = 20 μm. D) Confocal images showed that Nb14-3-3h-DsRed colocalized with 6K1-GFP. The Agrobacterium culture harboring Nb14-3-3h-DsRed was inoculated to the PVY-6K1-GFP systematically infected leaves, and the pictures were taken at 2 dpi. Scale bars = 20 μm. The second row showed a magnification of dotted rectangle regions in the first row. E) Co-IP analysis of the interaction between 6K1-eGFP and Nb14-3-3hS63E-Myc, Nb14-3-3hQQR-Myc, or Nb14-3-3hY89Q-Myc. The N. benthamiana leaf tissues coexpressing various combinations of proteins were harvested at 3 dpi. Total proteins were extracted and immunoprecipitated with GFP-Trap beads. The input and IP samples were then analyzed through Western blot assay using a Myc-specific or GFP-specific antibody. F) GST pull-down analysis of the interaction between 6K1 and 3 dimerization defective mutants. Purified 6K1-GST or GST was incubated with eGFP-Nb14-3-3h, eGFP-Nb14-3-3hS63E, eGFP-Nb14-3-3hQQR, eGFP-Nb14-3-3hY89Q, or eGFP. After immunoprecipitation with GST-Trap beads, the IP and input samples were analyzed through Western blot assay using a GST or GFP antibody.
The N. tabacum plants are known to have 17 different 14-3-3 isoforms (Konagaya et al. 2004). We searched the N. benthamiana genome database using these sequences, but only found 9 isoforms (Supplemental Fig. S7). To determine whether other Nb14-3-3 isoforms can interact with PVY 6K1, we individually cloned the other 8 representative Nb14-3-3 isoforms (Nb14-3-3a, b, c, d, e, f, g, and i) and tested their potential interactions with 6K1 through Co-IP, GST pull-down, and BiFC assays. The results showed that 6K1 interacted with all 9 14-3-3 isoforms (Supplemental Fig. S8).
PVY 6K1 interferes with the interaction between Nb14-3-3h and NbTCTP to promote virus infection
Considering both 6K1 and NbTCTP interact with Nb14-3-3h, we investigated whether 6K1 affects Nb14-3-3h and NbTCTP interaction. Nb14-3-3h-Nluc, Cluc-NbTCTP, and 6K1-eGFP were coexpressed in N. benthamiana leaves followed by LCI assay. The results showed that the leaf patches coexpressing Nb14-3-3-Nluc, Cluc-NbTCTP, and 6K1-eGFP showed a much weaker reaction signal than the control (Fig. 7A), though the expression levels of the assayed proteins were similar (Fig. 7B). To test whether 6K1 directly affects their interaction, we expressed and purified GST-Nb14-3-3h and eGFP-NbTCTP and analyzed them through a competitive GST pull-down assay. After immunoprecipitation with GST agarose beads, the precipitated proteins were individually incubated with 0, 10, 20, or 40 μg 6K1-MBP, followed by a Western blot assay. The results showed that, with the increasing concentration of 6K1-MBP (MBP fused to the C-terminus of 6K1), less eGFP-NbTCTP was pulled down by GST-Nb14-3-3h (Fig. 7C). These results indicated that 6K1 directly interfered with the interaction between Nb14-3-3h and NbTCTP. We then confirmed these results using BiFC assay. Weaker signals were detected in leaf tissues expressing NbTCTP-nYFP and Nb14-3-3h-cYFP in the presence of 6K1 than that in the absence of 6K1 (Fig. 7D). To evaluate the effect of 6K1 on Nb14-3-3h-NbTCTP interaction during PVY infection, PVY-GFP was coexpressed with Nb14-3-3h-Nluc and Cluc-NbTCTP, and coexpression of PVY-GFP significantly inhibited their interaction signal (Fig. 7, E and F). BiFC assay confirmed that a weaker signal was observed in leaf tissues coexpressing PVY-mCherry with NbTCTP-nYFP and Nb14-3-3h-cYFP than that with mCherry (Fig. 7G). The fusion 6K1-eGFP specifically interacted with Nb14-3-3h-Myc, but not with NbTCTP-Myc (Supplemental Fig. S9, A and B). These findings indicate that 6K1 interferes with the interaction between Nb14-3-3h and NbTCTP by competitively binding to Nb14-3-3h but not NbTCTP. The substitution of Phe (F) at position 23 with Ala (A) in 6K1 attenuated its interaction with Nb14-3-3h through Co-IP assay (Supplemental Fig. S10A). Then, we introduced the F23A mutation into PVY infectious clone. The results showed that PVY-GFP-6K1F23A–inoculated plants displayed milder symptoms. The viral protein and RNA accumulation levels of PVY-GFP-6K1F23A were significantly lower than those of the wild-type PVY-GFP (Supplemental Fig. S10, B and D). In addition, the replication level of PVYrep-GFP-6K1F23A significantly decreased (Supplemental Fig. S10E), indicating that 6K1-Nb14-3-3h interaction is required for 6K1 to subvert Nb14-3-3h-mediated defense.
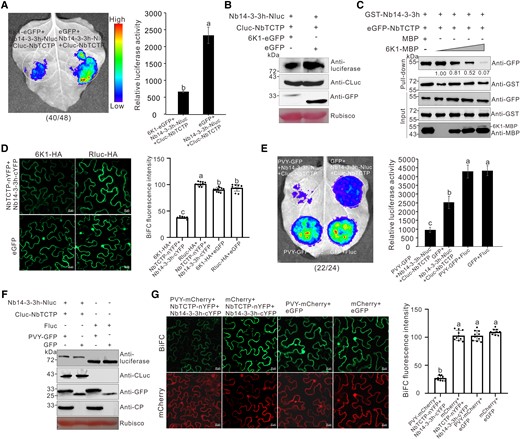
PVY 6K1 can interfere with the interaction between Nb14-3-3h and NbTCTP. A) LCI assay showed the effect of 6K1 on Nb14-3-3h-NbTCTP interaction. The N. benthamiana leaves were infiltrated with Agrobacterium cultures harboring different combinations and then photographed at 2 dpi. The dotted circles indicated the infiltrated regions. The numbers below the image represented the ratio of leaves with similar phenotypes as shown in the figure to the total number of leaves in 3 experiments. Quantification of luciferase activity from various infiltrated N. benthamiana leaf patches as shown in dotted circle regions at 2 dpi. The error bars represented the means ± Sd from 3 biological replicates. The experiments were repeated 3 times with similar results, and the statistical significance was determined using Duncan's multiple-range test. Different letters represented significant differences (P < 0.05). B) Western blot analysis of the Nb14-3-3h-Nluc, Cluc-NbTCTP, and 6K1-eGFP expression levels using a luciferase-specific, CLuc-specific, or GFP-specific antibody. C) Pull-down analysis of the effect of 6K1 on Nb14-3-3h-NbTCTP interaction. Purified GST-Nb14-3-3h, eGFP-NbTCTP, MBP, and 6K1-MBP (diluted to 0, 10, 20, or 40 μg) were incubated in various combinations followed by immunoprecipitation using GST-Trap beads. The resulting IP and input samples were analyzed through Western blot assay using a GFP-specific, GST-specific, or MBP-specific antibody. Protein band intensities were measured using the ImageJ software. The second band was set to 1, and numbers indicated the GFP protein levels normalized to the second band. This experiment was repeated 3 times with similar results. D) BiFC assay showed the effect of 6K1 on Nb14-3-3h-NbTCTP interaction. NbTCTP-nYFP, Nb14-3-3h-cYFP, and 6K1-HA were coexpressed in N. benthamiana plants. Confocal images were taken at 2 dpi. Scale bars = 20 μm. The BiFC intensity according to YFP fluorescence was measured using the ImageJ software. The bar graphs represented the means ± Sd (n = 10). The statistical significance was determined using Duncan's multiple-range test. Different letters represented significant differences (P < 0.05). E) LCI analysis of the effect of 6K1 on the interaction between Nb14-3-3h and NbTCTP during PVY infection. The N. benthamiana leaves were photographed at 3 dpi. The numbers below the image represented the ratio of leaves with similar phenotype as shown in the figure to the total number of leaves in 3 experiments. Quantification of luciferase activity from various infiltrated N. benthamiana leaf patches as shown in dotted circle regions at 3 dpi. The error bars represented the means ± Sd from 3 biological replicates. The experiments were repeated 3 times with similar results, and the statistical significance was determined using Duncan's multiple-range test. Different letters represented significant differences (P < 0.05). F) Analysis of the protein expression levels through Western blot assay using a luciferase-specific, CLuc-specific, GFP-specific, or CP-specific antibody. G) BiFC assay showed the effect of PVY infection on Nb14-3-3h-NbTCTP interaction. NbTCTP-nYFP, Nb14-3-3h-cYFP, and PVY-mCherry were coexpressed in N. benthamiana plants. Confocal images were taken at 3 dpi. Scale bars = 20 μm. The intensity of BiFC according to YFP fluorescence (top row) was measured using the ImageJ software. The bar graphs represented means ± Sd (n = 10). The statistical significance was determined using Duncan's multiple-range test. Different letters represented significant differences (P < 0.05).
Discussion
In this study, we found that Nb14-3-3h can mediate antiviral defense against PVY infection in a manner of sequestering NbTCTP. As a counter-defense strategy, PVY deploys 6K1 to interfere with Nb14-3-3h-TCTP interaction to promote virus infection. These results increase our understanding of the mechanisms of how virus counters 14-3-3–mediated antiviral response.
In plants, TCTP functions as a growth regulator and has been reported to participate in potyvirus infection (Brioudes et al. 2010; Bruckner et al. 2017, 2021). Silencing of TCTP significantly reduces TuMV accumulation in N. benthamiana protoplasts, and TCTP could colocalize with 6K2-induced vesicles, suggesting it may play a role in TuMV replication (Bruckner et al. 2021). Our results showed that PVY replication levels drastically decreased in TCTP-silencing N. benthamiana plants (Fig. 1). Previous studies have shown that silencing of TCTP gene negatively affects the accumulation of PepYMV and TuMV (Bruckner et al. 2017, 2021). These results suggest a general role of plant TCTP in facilitating potyvirus infection.
The 14-3-3 proteins are widely involved in multiple biological processes including plant growth and development (Huang et al. 2021). Accumulating studies have indicated that 14-3-3 proteins participate in plant–pathogen interactions (Denison et al. 2011; Lozano-Duran and Robatzek 2015). Rice 14-3-3 protein GF14c positively regulates rice immunity to Magnaporthe oryzae (Lu et al. 2022). Arabidopsis 14-3-3 proteins GRF6 and GRF8 can facilitate the activation of basal immunity against bacteria and fungi (Dong et al. 2023). Recent studies showed that 14-3-3 proteins play an important role in plant antiviral defense against necroviruses (Gao et al. 2022). However, the role of 14-3-3 proteins during plant response to diverse virus infections remains to be fully understood. Here, we found that dimeric Nb14-3-3h can positively regulate N. benthamiana defense against PVY infection (Figs. 3 and 4). In this study, silencing Nb14-3-3h using its 3′-UTR–specific sequence greatly reduced the expression levels of Nb14-3-3h and Nb14-3-3a, slightly affected those of Nb14-3-3c, Nb14-3-3d, Nb14-3-3g, and Nb14-3-3i, but had no significant effect on those of Nb14-3-3b, Nb14-3-3e, or Nb14-3-3f (Fig. 3H). Although overexpression of Nb14-3-3h showed the most significant inhibition effect on PVY infection, overexpression of Nb14-3-3c, d, and g restricted PVY accumulation (Supplemental Fig. S1, B and C). Interestingly, our results showed that transient overexpression of Nb14-3-3a in N. benthamiana leaf patches had no obvious effect on PVY infection (Supplemental Fig. S1B), which was different from the antiviral role of Nb14-3-3a in regulating the necrovirus BBSV infection (Gao et al. 2022), indicating that plants may deploy distinct 14-3-3 isoform to defend against a specific virus.
Drosophila 14-3-3 has been shown to physically interact with TCTP to modulate organ development (Le et al. 2016). These results promote us to test whether Nb14-3-3h interacts with NbTCTP in plants. Our data confirmed their interaction (Fig. 2) and demonstrated the mutant Nb14-3-3hE16A, which still dimerized and interacted with 6K1 but was unable to interact with NbTCTP (Supplemental Figs. S4 and S5), had reduced anti-PVY ability (Fig. 5, A to C). And NbTCTPI65A, which was unable to interact with Nb14-3-3h, promoted PVY infection compared with the wild-type NbTCTP (Fig. 5, D to F; Supplemental Fig. S6). These findings revealed that Nb14-3-3h negatively regulated PVY infection by sequestering NbTCTP. TCTP has been shown to stabilize or destabilize their interacting proteins (Bonhoure et al. 2017). In our study, we found that NbTCTP did not influence Nb14-3-3h dimerization (Supplemental Fig. S9, C and D). A previous study implied that Arabidopsis TCTP may be a regulator of the TOR pathway (Berkowitz et al. 2008), which integrates various signals, such as nutrients, hormones, and growth factors to control multicellular proliferation and growth (Shi et al. 2018). In Drosophila, 14-3-3 was reported to regulate the TOR signaling pathway by interacting with TCTP and Rheb GTPase (Le et al. 2016). Plants do not have a Rheb homolog, but 4 other Rab GTPases were identified to interact with TCTP (Brioudes et al. 2010). We also found Rab7-like, RABB1c, and RABA2a-like genes in our IP-MS results. Because Arabidopsis TCTP can fully functionally rescue the cell proliferation defects in TCTP knockout Drosophila (Brioudes et al. 2010), we hypothesized that some unknown GTPases participate in the plant 14-3-3-TCTP interaction and regulate PVY infection through the TOR pathway. We noticed that the viral protein and RNA accumulation levels in leaf patches overexpressing Nb14-3-3hE16A-Myc were 44% to 62% of those in leaf patches overexpressing Rluc-Myc (Fig. 5, B and C), indicating that disrupting the Nb14-3-3h-NbTCTP interaction could not fully abolish the antiviral function of Nb14-3-3h. These results suggested that Nb14-3-3h participating in plant antiviral defense may be not only via sequestering NbTCTP but also dependent on other host factors. A recent study has shown that Arabidopsis GRF6 and GRF8 directly interact with MAPKKK5 to positively regulate plant immunity signals (Dong et al. 2023). Plants have likely evolved diverse 14-3-3-MAPKKKs or 14-3-3–mediated MAPKKK-independent antiviral strategies.
Although 14-3-3 proteins positively regulate plant immunity, as a counter-defense, pathogens have evolved strategies to interfere with 14-3-3 to ensure their infections in plants. Bacterial effectors can target host 14-3-3 proteins to suppress the effector-triggered host immunity to establish their infections in plants (Kim et al. 2009; Taylor et al. 2012; Li et al. 2013; Teper et al. 2014). BBSV CP can target N. benthamiana 14-3-3a and disturb its interaction with MAPKKKα to impair MAPKKKα-mediated antiviral defense (Gao et al. 2022). In our study, we found that 6K1 can suppress Nb14-3-3h–mediated antiviral response to PVY by interfering with Nb14-3-3h-NbTCTP interaction (Fig. 7). 14-3-3 proteins form homo-dimer or hetero-dimer, which are required in many biological processes (Aitken 2011). Human dimeric 14-3-3 protein can activate and maintain Raf kinase activity in vivo, but the monomeric type could not (Tzivion et al. 1998). A mammalian 14-3-3ζ dimerization mutant loses its ability to interact with the p53 protein and cannot enhance the transcription activity of p53 (Gu et al. 2006). Likewise, in our study, we found dimerization of Nb14-3-3h is essential for restricting PVY infection (Fig. 4; Supplemental Fig. S3), similar to the role of Nb14-3-3aQQR in BBSV infection (Gao et al. 2022). Interestingly, the amino acids LAE, S63, and Y89 that determined Nb14-3-3h dimerization and 6K1-Nb14-3-3h interaction are the same (Figs. 4 and 6). Sequence alignment showed that the corresponding amino acids of LAE, S63, and Y89 were relatively conserved in some crops 14-3-3h genes and in most of the N. benthamiana 14-3-3 isoforms (Supplemental Fig. S2A). In addition, Nb14-3-3h mediated antiviral defense to TVBMV (Supplemental Fig. S11), suggesting a general role of 14-3-3h in different pathosystems. PPV 6K1 and TVBMV 6K1 can form punctate structures associated with chloroplast and colocalize with 6K2-induced VRC (Cui and Wang 2016; Geng et al. 2017). Our results showed that 6K1 interacted with Nb14-3-3h in the cell cytoplasm during PVY infection (Fig. 6, C and D). Likewise, Hong et al. (2007) found that soybean mosaic virus 6K1 localizes to the cell periphery in virus-infected leaves. The differences in 6K1 subcellular localization might be virus-dependent, and whether Nb14-3-3h influences PVY 6K1 localization remains to be studied. One of the function mechanisms mediated by 14-3-3 proteins is that it can function as a scaffold to bridge 2 partner proteins together (Lozano-Duran and Robatzek 2015). For example, a N. tabacum 14-3-3h molecular scaffold can support the interaction between the N protein and the helicase domain of TMV (Konagaya et al. 2004). The 14-3-3 protein can also act as a scaffold between RIG-I and TRIM25 ligase and stabilize their interaction to facilitate type I interferon responses in mammals (Gupta et al. 2019). Interestingly, we found the key amino acid E16 of Nb14-3-3h responsible for 14-3-3h–TCTP interaction is located in the LAE motif (Supplemental Fig. S2A), suggesting that this motif is not only essential for Nb14-3-3h dimerization and interaction with 6K1 but also participates in 14-3-3h–TCTP interaction. These 3 proteins and other potential host factors may cooperatively form a complex to regulate PVY infection.
According to the results of this study, we proposed a model for how 6K1 interferes with 14-3-3h–mediated antiviral defense (Fig. 8). TCTP is a susceptibility factor and necessary for potyvirus infection. 14-3-3h has antiviral activity and exists in the form of dimer or tetramer in plants. The dimerization is essential for 14-3-3h in inhibiting PVY replication. In normal conditions, 14-3-3 and TCTP coregulate plant growth. Upon PVY infection, TCTP is recruited to facilitate PVY infection. 14-3-3h is rapidly induced and mediates a series of antiviral defenses by sequestering NbTCTP or other host factors. As a counter-defense, PVY 6K1 competitively binds to Nb14-3-3h to rescue TCTP, resulting in the successful infection of PVY. The results obtained in this study provide insights into the arms race between plants and viruses.
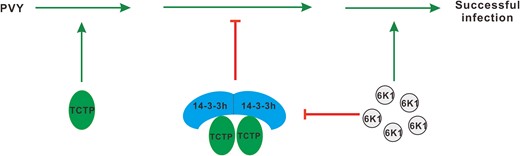
A proposed model for the role of 6K1 in regulating the 14-3-3h-TCTP complex to counteract antiviral defense. TCTP is a susceptibility factor for potyvirus infection. Upon virus infection, 14-3-3h is rapidly induced and mediates host antiviral defenses via sequestering TCTP. But, PVY 6K1 interferes with the interaction between 14-3-3h and TCTP to achieve successful virus infection. The green arrows represented promotion effect, and the red symbols indicated the inhibition effect.
Materials and methods
Plant materials and growth conditions
N. benthamiana and transgenic tobacco (N. tabacum) plants were grown in a greenhouse maintained at 24/22 °C (day/night) and a 16 h light/8 h dark cycle. The TCTP-KD and TCTP-OE N. tabacum materials have been previously described (Guo et al. 2017) and were provided by Yu-Shuang Guo from Guizhou Institute of Tobacco Science.
Plasmid construction
The full-length sequence of PVY N605 (X97895.1) was RT-PCR amplified and cloned into the pCB301 vector to generate pPVY N605. Then, the sequences coding GFP, mCherry, and 6K1-GFP were individually inserted between the coding sequences (CDSs) of nuclear inclusion protein b and CP, producing pPVY N605-GFP, pPVY N605-mCherry, and pPVY N605-6K1-GFP (referred to as pPVY-GFP, pPVY-mCherry, and pPVY-6K1-GFP). To access the virus replication level, the expression cassette of mCherry-HDEL was incorporated into pPVY-GFP after the Nos terminator as previously described (Agbeci et al. 2013). And then, codons for 2 amino acids of P3N-PIPO at positions of 177 (K) and 200 (Q) were simultaneously mutated to stop codons, thus generating the cell-to-cell movement deficient of PVY replicon (referred to as pPVYrep-GFP). Similarly, we incorporated the expression cassette of mCherry-HDEL after the Nos terminator of pTVBMV-PIPOSTOP, a GFP-tagged cell-to-cell movement deficient of TVBMV mutant as previously described (Geng et al. 2015, 2017), thus generating the TVBMV replicon referred to as pTVBMVrep-GFP. The PVY 6K1 gene was cloned into the p35SUTR-eGFP (an expression vector containing a 35S promoter, 5ʹ-UTR of TVBMV, and eGFP CDS), p35ScYFP (an expression vector containing a 35S promoter and C-terminal fragment of eYFP), pETEVGSTHIS, and pETEVMBPHIS vectors to produce p6K1-eGFP, p6K1-cYFP, pE6K1-GST, and pE6K1-MBP, respectively. The full-length CDS of Nb14-3-3h was RT-PCR amplified from N. benthamiana cDNA and cloned into the p35SUTR-Myc (an expression vector containing a 35S promoter, 5ʹ-UTR of TVBMV, and 3×Myc CDS), p35SMyc (an expression vector containing a 35S promoter and 3×Myc CDS), p35SnYFP (an expression vector containing a 35S promoter and N-terminal fragment of eYFP), p35ScYFP, pNluc, pCluc, pEHISeGFPTEV, and pEHISGSTTEV vectors to produce pNb14-3-3h-Myc, p35S-Nb14-3-3h-Myc, pNb14-3-3h-nYFP, pNb14-3-3h-cYFP, pNb14-3-3h-Nluc, pCluc-Nb14-3-3h, pEeGFP-Nb14-3-3h, and pEGST-Nb14-3-3h, respectively. The obtained plasmids were used for site-directed mutagenesis following the method previously reported (Liu and Naismith 2008). The full-length CDS of NbTCTP was RT-PCR amplified from N. benthamiana cDNA and cloned into the p35SUTR-eGFP, pEHISeGFPTEV, p35SUTR-Myc, p35SnYFP, and pCluc vectors to produce pNbTCTP-eGFP, pEeGFP-NbTCTP, pNbTCTP-Myc, pNbTCTP-nYFP, and pCluc-NbTCTP, respectively. The full-length CDS of Nb14-3-3 isoforms, Nb14-3-3a, Nb14-3-3b, Nb14-3-3c, Nb14-3-3d, Nb14-3-3e, Nb14-3-3f, Nb14-3-3g, and Nb14-3-3i, were retrieved from the SOL Genomics Network (https://solgenomics.net/) and cloned into the Xba I-digested and Xma I-digested p35SUTR-Myc, p35SnYFP, and pEeGFP vectors. For TRV-mediated gene silencing assay, the 3ʹ-UTR fragments corresponding to Nb14-3-3h and NbTCTP genes were cloned into the pTRV2 vector, respectively. To construct pTRV2-NbTCTP//Nb14-3-3h, the fragments containing 167 nucleotides from NbTCTP 3′-UTR and 239 nucleotides from Nb14-3-3h 3′-UTR were separately amplified from N. benthamiana cDNA through overlap PCR with no spacer and then were cloned into the Xba I-digested and Xma I-digested TRV2 vector using T4 DNA ligase (Takara, Dalian, China). The sequences from NbTCTP and Nb14-3-3h 3′-UTR used in pTRV2-NbTCTP//Nb14-3-3h were supplied in Supplemental Fig. S12. The primers used in this study were listed in Supplemental Table S1.
Virus inoculation
Expression vectors were individually transformed into Agrobacterium tumefaciens strain GV3101 using a freeze-thaw method (Fang et al. 2021). Agrobacterium cultures harboring specific vectors were individually grown overnight at 28 °C in a LB liquid medium supplemented with appropriate antibiotics. Agrobacterium cells were pelleted, resuspended in an induction buffer (10 mm MES, pH 5.6, 10 mm MgCl2, and 150 μm acetosyringone), and incubated at room temperature for 3 h. For PVY inoculation, the Agrobacterium culture harboring pPVY-GFP, pPVY-mCherry, pPVYrep-GFP, or pPVY-6K1-GFP was diluted to OD600 = 0.5 and then infiltrated into leaves of N. benthamiana plants using needless syringes. The N. benthamiana leaves systemically infected with pPVY-GFP were harvested at 7 dpi for mechanical inoculation. For transient gene expressions, the Agrobacterium cultures were prepared as described above, individually diluted to OD600 = 1.0, mixed with an equal volume of Agrobacterium culture carrying pBinP19, and then infiltrated into the leaves of N. benthamiana plants.
Co-IP and Western blot assays
Total proteins were extracted from infiltrated or systemically infected leaves of assayed N. benthamiana plants followed by Western blot assay as described previously (Fang et al. 2021) using specific antibodies. For the Co-IP assay, total proteins were extracted from the sampled N. benthamiana leaves (3 g per sample) in an ice-cold immunoprecipitation buffer as described (Geng et al. 2015). The supernatant was collected from individual samples and incubated with GFP-Trap or HA-Trap beads (Shenzhen KT Life Technology, China) for 1 h at 4 °C followed by centrifugation. The pellets were rinsed 3 times with ice-cold immunoprecipitation buffer at 4 °C, resuspended individually in a 2× SDS sample buffer, and then analyzed through Western blot assay using a GFP-specific (Abways Technology, Shanghai, China), Myc-specific (Abways Technology), or HA-specific (Roche Diagnostics GmbH) antibody as instructed by the manufacturer.
Pull-down assay
For GST pull-down assay, plasmids pEeGFP-Nb14-3-3h, pEGST-Nb14-3-3h, pEeGFP-NbTCTP, pE6K1-MBP, pE6K1-GST, pEeGFP, pEGST, and pEMBP were individually transformed into E. coli cells (Rossetta, DE3). The proteins were expressed and purified as previously described (Cheng et al. 2021). For in vitro interaction assay, approximately 20 μg purified GST-tagged protein was mixed with 20 μg purified GFP-tagged protein followed by the addition of 15 μL GST-Trap beads (GE Healthcare, USA) in an ice-cold PBS buffer (pH 7.4). The mixtures were incubated for 1 h at 4 °C followed by 2 min centrifugation at 500 × g. The pellets were rinsed 6 times with PBS buffer, resuspended individually in 2× SDS sample buffer, boiled for 10 min, and then analyzed through Western blot assay using a GFP-specific or GST-specific antibody. For GST competitive pull-down assay, 20 μg purified GST-Nb14-3-3h and eGFP-NbTCTP were mixed with GST-Trap beads. After incubation and centrifugation, the pellet was rinsed 3 times and incubated for 1 h with 10, 20, or 40 μg 6K1-MBP or 40 μg MBP, where the number indicated the amount of the purified total protein, respectively. The pull-down products were then analyzed through Western blot assay using antibodies against GST, GFP, or MBP. The experiments were repeated 3 times independently.
LCI assay
LCI assay was performed in N. benthamiana leaves as described (Chen et al. 2008). Briefly, N. benthamiana leaves were infiltrated with Agrobacterium cultures harboring various combinations of plasmids as described above. The infiltrated leaves were sprayed with 1 mm luciferin solution—the substrate for firefly luciferase at 2 or 3 dpi—and then examined under a low-light cooled CCD imaging apparatus (Xenogen Corporation, Alameda, CA, USA). The leaf images were captured, and the luciferase activity was measured after 5 min postdark treatment. The infiltrated leaf patches were harvested, and total proteins were extracted. The supernatant was collected from individual samples and analyzed through Western blot assay using a luciferase-specific or Cluc-specific antibody. The experiments were repeated 3 times independently.
BiFC and subcellular localization assays
BiFC and subcellular localization assays were conducted as previously reported (Geng et al. 2015). Agrobacterium cultures containing various BiFC constructs were equally mixed with a final OD600 = 0.3 and coinoculated with Agrobacterium cultures harboring pPVY-mCherry (OD600 = 0.5) on 4-wk-old N. benthamiana plants. The fluorescence of YFP and mCherry was detected under a Zeiss LSM800 confocal microscope at 2 dpi and visualized using the ZEN blue version 3.5. The YFP was excited at 514 nm and captured at 565 to 585 nm. The GFP was excited at 488 nm and captured at 505 to 540 nm. DsRed or mCherry was excited at 543 nm and captured at 590 to 630 nm. The confocal images were captured using a pinhole size of 1 AU with gains of 600 to 680. BiFC fluorescence intensity was measured using ImageJ software. Three independent experiments were performed with similar results.
RNA extraction and RT-qPCR
Total RNA was extracted from the infiltrated or systemically infected N. benthamiana leaf patches using Trizol reagent (TransGen Biotech, Beijing, China). The RNA samples were then treated with a gDNA wiper enzyme (Vazyme, Nanjing, China) to remove genomic DNA. And, 1 μg total RNA was reverse transcribed using a HiScriptII qRT SuperMix kit supplemented with random primers (Vazyme) to synthesize the first strand cDNA. RT-qPCR was conducted using ChamQ SYBR qPCR Master Mix (Vazyme) on a PCR machine (LightCycler 96, Roche, Basal, Switzerland). The gene-specific primers were listed in Supplemental Table S1. The N. benthamiana EF1α was used as an internal control for the analysis of the gene mRNA levels in this study. All experiments were repeated 3 times independently.
VIGS and green fluorescence imaging assays
Plasmids, pTRV1 and pTRV2, and its derivatives were individually transformed into Agrobacterium strain GV3101. VIGS assay was conducted as previously reported (Geng et al. 2015). Briefly, Agrobacterium culture harboring pTRV1 was mixed with an equal volume of Agrobacterium culture harboring pTRV2 or one of its derivatives and then the mixtures were infiltrated individually into N. benthamiana leaves using needless syringes. At 12 dpi, systematic leaves of each assayed plant were inoculated again with an Agrobacterium culture harboring pPVY-GFP (OD600 = 0.5) or pPVYrep-GFP (OD600 = 0.2). The expression levels of individual Nb14-3-3 members and PVY-GFP in the assayed plants were determined through RT-qPCR using gene-specific primers. The crude extracts of PVY-GFP infected N. benthamiana leaves were rub-inoculated to the wild-type and TCTP transgenic N. tabacum plants. All VIGS experiments were repeated 3 times with 10 plants per treatment. GFP fluorescence in the assayed plant leaves was visualized using a long-wave UV light, and the leaves were photographed using a Canon EOS 800D digital camera. The intensities of GFP fluorescence and the area of PVY GFP infection sites were measured using ImageJ software.
Sequence alignment and phylogenetic analysis
Amino acid sequences of nine 14-3-3 isoforms from N. benthamiana plants were aligned using Clustal W and then subjected to phylogenetic analysis based on Neighbor-Joining method assembled in the software package MEGA11.
Statistical analysis
Statistical significance was determined by Duncan's multiple-range test using IBM SPSS software. Different letters represented significant differences (P < 0.05). Student's t-test analysis using GraphPad Prism 7 software was also utilized in this study. Significant differences were indicated by *P < 0.05, **P < 0.01, or ***P < 0.001 and “ns,” with no significant difference. The intensity of the bands in Western blotting was quantified using ImageJ software.
Accession numbers
The accession numbers for the genes studied in this article were NbTCTP (AF107842.1), Nb14-3-3a (AB119466.1), Nb14-3-3b (AB119467.1), Nb14-3-3c (AB119469.1), Nb14-3-3d (AB119471.1), Nb14-3-3e (AB119474.1), Nb14-3-3f (AB119476.1), Nb14-3-3g (AB119477.1), Nb14-3-3h (AB119478.1), and Nb14-3-3i (AB119480.1).
Author contributions
X.-D.L. and C.G. conceived the research plan and designed the experiments. L.F., X.-Y.W., C.-C.D., and J.-P.P. performed the experiments. L.F. prepared the Figures and Tables. X.-D.L., C.G., and L.F. cowrote the article. All authors analyzed the results.
Supplemental data
The following materials are available in the online version of this article.
Supplemental Figure S1. Overexpression of N. benthamiana 14-3-3h inhibits PVY replication.
Supplemental Figure S2. Analysis of Nb14-3-3h dimerization mutants.
Supplemental Figure S3. Effects of Nb14-3-3hQQR and Nb14-3-3hS63E on PVY-GFP infection.
Supplemental Figure S4. Nb14-3-3hE16A cannot interact with NbTCTP, and the E16A mutation affects the antiviral activity of Nb14-3-3h.
Supplemental Figure S5. Nb14-3-3hE16A interacts with 6K1 and itself.
Supplemental Figure S6. The isoleucine at position 65 of NbTCTP determines its interaction with Nb14-3-3h.
Supplemental Figure S7. Phylogenetic tree of 14-3-3 proteins from N. benthamiana.
Supplemental Figure S8. Analysis of in vivo and in vitro interactions between PVY 6K1 and N. benthamiana 14-3-3 isoforms.
Supplemental Figure S9. 6K1 does not interact with NbTCTP, and NbTCTP does not affect Nb14-3-3h dimerization.
Supplemental Figure S10. F23A mutation in 6K1 attenuates its interaction with Nb14-3-3h and inhibits PVY replication.
Supplemental Figure S11. Nb14-3-3h mediates host antiviral response to TVBMV.
Supplemental Figure S12. The sequences from NbTCTP and Nb14-3-3h 3′-UTR used in pTRV2-NbTCTP//Nb14-3-3h.
Supplemental Table S1. The name and nucleotide sequences of the primers used in this study.
Funding
This study was supported by the National Natural Science Foundation of China (NSFC, 31720103912) and the “Taishan Scholar” Construction Project (TS201712023).
Dive Curated Terms
The following phenotypic, genotypic, and functional terms are of significance to the work described in this paper:
References
Author notes
These authors contributed equally.
The author responsible for distribution of materials integral to the findings presented in this article in accordance with the policy described in the Instructions for Authors (https://dbpia.nl.go.kr/plphys/pages/General-Instructions) is: Xiang-Dong Li.
Conflict of interest statement. The authors declare no conflict of interest.