-
PDF
- Split View
-
Views
-
Cite
Cite
Yaoyu Chen, Zhe Yang, Huanhuan Wang, Chuan Xia, Lichao Zhang, Jiaqiang Sun, Xiuying Kong, Xu Liu, Two open reading frames of Rht-B1b acting as brake and throttle contributed to wheat Green Revolution, Plant Physiology, Volume 194, Issue 3, March 2024, Pages 1290–1293, https://doi.org/10.1093/plphys/kiad636
- Share Icon Share
Dear Editor,
Since the 1960s, the introduction of the Reduced height (Rht)-B1b gene has contributed to the Green Revolution in wheat (Triticum aestivum L.) by reducing plant height, increasing resistance to lodging, suitable for intensive cultivation, and increasing yields dramatically (Peng et al. 1999). The Rht-B1b semi-dwarfing allele contains 1 base substitution mutation, causing a stop codon following the DELLA region and leading to the production of 2 open reading frames (ORFs) different from 1 ORF of Rht-B1a, a small 5′-ORF and a main 3′-ORF, respectively (Peng et al. 1999; Liu et al. 2021; Van De Velde et al. 2021). The 3′-ORF could produce a N-terminally truncated DELLA protein, which is stabilized by the lack of an intact DELLA motif, leading to reduced plant height (Van De Velde et al. 2021). However, whether the small 5′-ORF of Rht-B1b played a role in the Green Revolution of wheat remains unclear.
In this study, we identified a severe dwarfing wheat mutant, EF6085, in the Fielder variety background containing the Rht-B1b allele from 1 ethyl methanesulfonate (EMS) wheat mutant library (Fig. 1A). Compared with Fielder, EF6085 showed a significant reduction in length of each internode, resulting in dramatically dwarfism in plant height, and exhibited a greater tiller angle (Fig. 1, B and C).
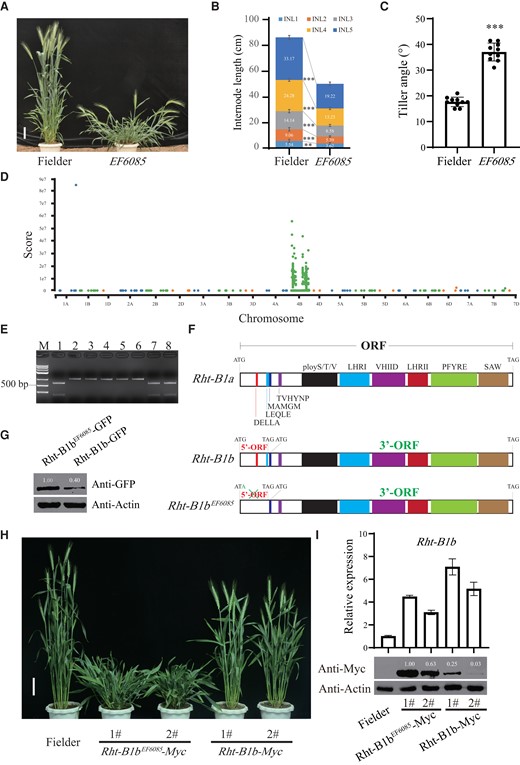
BSA-based cloning of a new allele of Rht-B1b revealing that 5′-ORF of Rht-B1b functions as an uORF to prevent overaccumulation of stabilized Rht-B1b protein, contributing to the Green Revolution in wheat. A) Phenotypes of Fielder and the EF6085 mutant at the grain filling stage. Bar, 10 cm. B) Comparison of the internode lengths (INLs) between Fielder and the EF6085 mutant at the grain ripening stage. C) Comparison of the tiller angles between Fielder and the EF6085 mutant at the grain filling stage. In B) and C), error bars indicate Sem, n = 10. Student's t-test was used to generate P values (**P < 0.01; ***P < 0.001). D) Manhattan plot of the varBScore along each chromosome. E) Genotyping of Rht-B1b in Fielder, EF6085, and their F2 progenies using a CAPS marker. Fielder-type Rht-B1b can be digested into 2 gel bands (232 and 585 bp) by restriction endonuclease BspHI, EF6085-type contains only 1 gel band (817 bp) that cannot be digested. M, marker; lane 1, Fielder; lane 2, EF6085; lanes 3 to 6, F2 individuals showing EF6085-type phenotype; lanes 7 to 8, F2 individuals showing Fielder-type phenotype. F) Gene structures of Rht-B1a, Rht-B1b, and Rht-B1bEF6085. Rht-B1a contains an intact ORF; Rht-B1b harbors 2 ORFs named as 5′-ORF and 3′-ORF; Rht-B1bEF6085 only has a 3′-ORF, in which the 5′-ORF was disrupted. G) Transient expression assays in wheat protoplasts showing that the 3′-ORF encoding protein of Rht-B1bEF6085 was highly accumulated compared with that of the Rht-B1b. H) Phenotypes of the pRht-B1b::Rht-B1b-Myc and pRht-B1b::Rht-B1bEF6085-Myc transgenic plants at the heading stage. Bar, 10 cm. I) Comparisons of transcriptional (above) and protein (below) expression levels of Rht-B1b between pRht-B1b::Rht-B1b-Myc and pRht-B1b::Rht-B1bEF6085-Myc transgenic plants. Error bars indicate Sem, n = 3. ImageJ software was used to measure the intensity values of the protein level in Fig. 1, G and I.
To understand the nature of the EF6085 mutant, we analyzed the phenotypes of F1 and F2 progenies obtained from the genetic cross between EF6085 and Fielder variety. We found that the plant height of all the F1 plants was intermediate between their 2 parents and the segregation ratio of their F2 progenies (422 individuals, whose phenotypes were verified by the F2:3 populations) fits a Mendelian model of 1 (severe dwarfing): 2 (intermediate): 1 (normal) (Supplemental Fig. S1, Supplemental Table S1), indicating that the severe dwarfing phenotype of the mutant is controlled by a semi-dominant mutation. To clone the gene causing the severe dwarfing phenotype of EF6085, 2 bulked DNA pools of the F2 population were used to conduct whole-exome sequencing (Dong et al. 2020; Zhang et al. 2021) and 2 bulked DNA pools of another F2 population (447 individuals) deriving from a genetic cross between EF6085 and ‘Jimai22’ cultivar were genotyped by 55K SNP array. Results showed that SNPs were mainly enriched on chromosome 4B (Fig. 1D, Supplemental Fig. S2). Then, we developed 7 KASP markers through these SNPs and mapped the gene to the Rht-B1b interval region (Supplemental Fig. S3, Supplemental Table S2). A G to A mutation in the Rht-B1b gene of EF6085 mutant was identified and then confirmed by a CAPS marker. Furthermore, we verified that all the Fielder-type lines are the “G” genotype and all the EF6085-type lines are “A” genotype in the F2 population (Fig. 1E), indicating that the G to A mutation in Rht-B1b causes a severe dwarfing phenotype.
Sequence analysis revealed that the G to A mutation in the EF6085 mutant exactly disrupted the translational start codon from ATG to ATA of the small 5′-ORF of Rht-B1b. Compared to Rht-B1b, Rht-B1bEF6085 retains only the 3′-ORF (Fig. 1F). Upstream ORFs (uORFs) are regulatory elements that are prevalent in eukaryotic mRNAs (Zhang et al. 2019), which generally decrease the translation of the downstream main ORFs through ribosome stalling (Kurihara 2020; Zhang et al. 2020; Dong and Ryabova 2023). Based on previous studies on the effects of uORFs (Xu et al. 2017a; Xu et al. 2017b; Xue et al. 2023), we predicted that the small 5′-ORF of Rht-B1b might act as an uORF to competitively repress the translation of the main 3′-ORF of Rht-B1b, restricting overaccumulation of 3′-ORF encoding protein. The transcription of this uORF was verified by RT-PCR and sequencing (Supplemental Fig. S4A), which could generate a small protein (Van De Velde et al. 2021). To investigate the effect of this uORF on the translation of 3′-ORF, 2 constructs with the 35S promoter driving the expression of either the Rht-B1b or Rht-B1bEF6085 were generated and transiently expressed in wheat protoplasts. As expected, the 3′-ORF of Rht-B1b allele produces less proteins than that of the Rht-B1bEF6085 allele (Fig. 1G, Supplemental Fig. S4B), demonstrating that the 5′-ORF competitively represses the translation of the 3′-ORF of Rht-B1b.
To validate the biological role of the 5′-ORF of Rht-B1b, we transformed the pRht-B1b::Rht-B1b-Myc and pRht-B1b::Rht-B1bEF6085-Myc constructs driven by the native promoter of Rht-B1b into Fielder, respectively. Phenotypic analysis showed that the pRht-B1b::Rht-B1b-Myc transgenic plants displayed a moderate reduction in plant height, while the pRht-B1b::Rht-B1bEF6085-Myc transgenic lines displayed severe dwarfing and large tiller angle phenotypes (Fig. 1H, Supplemental Fig. S5), which might be attributed to protein over-production of the 3′-ORF in the pRht-B1b::Rht-B1bEF6085-Myc transgenic lines. Transcriptional and protein expression analyses showed that the protein expression levels of 3′-ORF in the pRht-B1b::Rht-B1bEF6085-Myc lines were higher than those in the pRht-B1b::Rht-B1b-Myc lines (Fig. 1I), confirming that the 5′-ORF plays an inhibitory effect on the translation of 3′-ORF. Furthermore, the coleoptile elongation assays showed that gibberellin (GA) treatment could significantly increase the coleoptile length of Chinese Spring wheat, but failed to promote coleoptile elongation in Fielder (Rht-B1b), EF6085 mutant, pRht-B1b::Rht-B1b-Myc, and pRht-B1b::Rht-B1bEF6085-Myc transgenic plants (Supplemental Fig. S6, A and B). Immunoblotting analyses showed that the protein levels of Rht-B1b-Myc and Rht-B1bEF6085-Myc were not substantially affected after GA3 treatment (Supplemental Fig. S6C). Taken together, the above results suggest that the EF6085 mutation increases the translation efficiency of Rht-B1b but not affect the GA-induced degradation.
Based on the findings in this study, we conclude that the 3′-ORF of Rht-B1b functions to reduce plant height, while the 5′-ORF (like an uORF) of Rht-B1b serves as a brake to restrict overaccumulation of the 3′-ORF-encoded protein, achieving an appropriate reduction in plant height for wheat Green Revolution. If the 5′-ORF of Rht-B1b is disrupted as in the EF6085 mutant, the 3′-ORF encoding protein will be over-accumulated, leading to a severe dwarfing phenotype, which is not suitable for wheat production. Therefore, we propose that 2 ORFs of Rht-B1b act as a brake-throttle system to appropriately reduce plant height, contributing to the Green Revolution in wheat.
Acknowledgments
The authors thank Ms. Lingli Zheng for wheat transformation.
Author contributions
X.K., J.S., and X.L. conceived and designed the project; Y.C., Z.Y., and H.W. performed the experiments and analyzed the data; C.X. and L.Z. provided suggestions on experiments; Y.C., J.S., X.K., and X.L. wrote and revised the manuscript. All authors accepted the final version of the manuscript.
Supplemental data
The following materials are available in the online version of this article.
Supplemental Figure S1. Genetic analysis of the EF6085 mutant.
Supplemental Figure S2. Number of single-nucleotide polymorphisms (SNPs) with biased frequency on each of the 21 wheat chromosomes based on the wheat 55K SNP array.
Supplemental Figure S3. Gene mapping by KASP markers.
Supplemental Figure S4. Transcription expression analyses in Fielder plants and wheat protoplasts.
Supplemental Figure S5. Morphological characteristics of pRht-B1b::Rht-B1b-Myc and pRht-B1b::Rht-B1bEF6085-Myc transgenic plants.
Supplemental Figure S6. Coleoptile elongation assays in response to gibberellin treatment for the Chinese Spring, Fielder, EF6085, pRht-B1b::Rht-B1b-Myc, and pRht-B1b::Rht-B1bEF6085-Myc transgenic plants.
Supplemental Table S1. Segregation analysis of the F2 population between EF6085 and Fielder.
Supplemental Table S2. Primers and vectors used in this study.
Supplemental Materials and Methods.
Funding
This work was supported by the National Natural Science Foundation of China (31991213), Innovation Program of Chinese Academy of Agricultural Sciences, Grant from Ministry of Agriculture and Rural Affairs of China.
Data availability
The data underlying this article are available in the article and in its online supplementary material.
References
Author notes
The authors responsible for distribution of materials integral to the findings presented in this article in accordance with the policy described in the Instructions for Authors (https://dbpia.nl.go.kr/plphys/pages/General-Instructions) are: Jiaqiang Sun ([email protected]), Xiuying Kong ([email protected]), and Xu Liu ([email protected]).
Conflict of interest statement. None declared.