-
PDF
- Split View
-
Views
-
Cite
Cite
Li Zhang, Yang Xu, Yanting Li, Saisai Zheng, Zhenmei Zhao, Meiling Chen, Haijian Yang, Hualin Yi, Juxun Wu, Transcription factor CsMYB77 negatively regulates fruit ripening and fruit size in citrus, Plant Physiology, Volume 194, Issue 2, February 2024, Pages 867–883, https://doi.org/10.1093/plphys/kiad592
- Share Icon Share
Abstract
MYB family transcription factors (TFs) play essential roles in various biological processes, yet their involvement in regulating fruit ripening and fruit size in citrus remains poorly understood. In this study, we have established that the R2R3-MYB TF, CsMYB77, exerts a negative regulatory influence on fruit ripening in both citrus and tomato (Solanum lycopersicum), while also playing a role in modulating fruit size in citrus. The overexpression of CsMYB77 in tomato and Hongkong kumquat (Fortunella hindsii) led to notably delayed fruit ripening phenotypes. Moreover, the fruit size of Hongkong kumquat transgenic lines was largely reduced. Based on DNA affinity purification sequencing and verified interaction assays, SEVEN IN ABSENTIA OF ARABIDOPSIS THALIANA4 (SINAT4) and PIN-FORMED PROTEIN5 (PIN5) were identified as downstream target genes of CsMYB77. CsMYB77 inhibited the expression of SINAT4 to modulate abscisic acid (ABA) signaling, which delayed fruit ripening in transgenic tomato and Hongkong kumquat lines. The expression of PIN5 was activated by CsMYB77, which promoted free indole-3-acetic acid decline and modulated auxin signaling in the fruits of transgenic Hongkong kumquat lines. Taken together, our findings revealed a fruit development and ripening regulation module (MYB77-SINAT4/PIN5-ABA/auxin) in citrus, which enriches the understanding of the molecular regulatory network underlying fruit ripening and size.
Introduction
Citrus is one of the most vital crops globally. Fruit size and fruit ripening are 2 important characteristics that determine the overall yield and maturity of citrus fruits, and are formed during the development and ripening of citrus fruits. The development and ripening of citrus fruits can be divided into the cell division stage, expansion stage, and ripening stage, constituting a highly ordered and complex process (Bain 1958; Feng et al. 2021). Despite research efforts, the regulatory mechanisms governing fruit development and ripening in citrus remain unclear.
It is known that ethylene (Yang et al. 2022) and abscisic acid (ABA; Leng et al. 2014) play active roles in fruit ripening. ABA has been recognized for its importance in regulating ripening processes across both climacteric fruits [e.g. tomato (Solanum lycopersicum)] and nonclimacteric fruits (e.g. citrus). In tomato, which is a climacteric fruit, an overexpression (OE) of the ABA receptor gene PYRABACTIN RESISTANCE-LIKE9 (PYL9) promotes ripening by stimulating the expression of ethylene synthesis genes ACSs [encoding 1-aminocyclopropane-1-carboxylic acid (ACC) synthase] and ACOs (encoding ACC oxidase; Kai et al. 2019). Knocking down the ABA key synthetic gene 9-CIS-EPOXYCAROTENOID DIOXYGENASE1 (NCED1) results in firmer tomato fruits with slower cell wall degradation, indicating a delayed ripening phenotype (Sun et al. 2012). Exogenous ABA can promote peach (Prunus persica; Zhang et al. 2009a, 2009b) and banana (Musa acuminate; Thakur et al. 2019) fruit ripening. In strawberry (Fragaria spp.), which is a nonclimacteric fruit, silencing the NCED1 gene results in slow fruit coloration, which can be rescued by exogenous ABA (Jia et al. 2011). In citrus, NCED2 affects fruit ripening by changing fruit sensitivity (Zhang et al. 2021). Simultaneously, NCED5 has the capacity to enhance ABA synthesis and significantly advance citrus fruit ripening (Zhu et al. 2017). These findings strongly suggest that changes in ABA synthesis and sensitivity have substantial effects on fruit ripening in both climacteric and nonclimacteric fruits.
In plants, the ubiquitin-proteasome system and multivesicle body (MVB)-mediated vacuolar sorting are essential for protein degradation (Vierstra 2009). The endosomal sorting complex required for transport (ESCRT) machinery plays an important role in MVB-mediated vacuolar sorting (Gao et al. 2017) In Arabidopsis (Arabidopsis thaliana), FYVE DOMAIN PROTEINREQUIRED FOR ENDOSOMAL SORTING1 (FREE1) and VACUOLEPROTEIN SORTING23A (VPS23A) are key components of ESCRT-I (Spitzer et al. 2006; Gao et al. 2014). Previous studies reveal that the ubiquitinated ABA receptors PYRABACTIN RESISTANCE1 (PYR1) and PYL4 can be recognized by FREE1 and VPS23A for vacuolar degradation, and the free1 or vps23a mutants accumulate more PYR1/PYL4 proteins and are hypersensitive to ABA treatment (Belda-Palazon et al. 2016; Yu et al. 2016). More recently, a study reported that FREE1 and VPS23A could be ubiquitinated by SEVEN IN ABSENTIA OF ARABIDOPSIS THALIANA (SINAT) proteins (RING-type E3 ubiquitin ligases; Xia et al. 2020). The OE of SINATs increases the protein abundance of PYL4 and the expression levels of ABSCISIC ACID-RESPONSIVE ELEMENT-BINDING FACTOR2 (ABF2) and ABSCISIC ACID-INSENSITIVE5 (ABI5) transcription factors (TFs), thereby changing the sensitivity of plants to ABA (Xia et al. 2020). However, the roles of SINATs, VPS23A, and FREE1 in fruit ripening remain unclear.
Fruit size is one of the most important traits of citrus fruits. Auxin is closely involved in the growth and development of plants, especially their organ size (Vanneste and Friml 2009). Auxin synthesis or signal transduction proteins substantially regulate organ size, such as the tryptophan aminotransferase of Arabidopsis/YUCCA (YUC) proteins (Zhao et al. 2001), auxin receptor AUXIN-SIGNALING F-BOX4 (AFB4; Hu et al. 2012), AUXIN RESPONSE FACTOR6/8 (ARF6/8; Mao et al. 2020), and ARF19 (Ye et al. 2014). Fruit size is also positively controlled by auxin. In Arabidopsis, the MOB KINASE ACTIVATOR 1A protein mediates auxin signaling and is involved in embryogenesis and cell division (Cui et al. 2016). The moba1 mutant has shorter siliques (Cui et al. 2016), and upregulation of the YUC4 gene can markedly increase the size of siliques (Li et al. 2021). In tomato, the transcriptional level of AUXIN/INDOLE3-ACETIC ACID17 (IAA17) gradually decreases before fruit color change. The silencing of IAA17 results in increased fruit weight and volume (Su et al. 2014). Meanwhile, ARF9 suppresses cell differentiation in the fruit, and reduced transcriptional level of ARF9 leads to fruit enlargement (de Jong et al. 2015). The PIN gene family encodes an auxin efflux carrier protein, regulating the polar transport of auxin in cells, which functions in many aspects, including asymmetric growth (Friml et al. 2002a, 2002b), root patterning (Friml et al. 2002a, 2002b), plant organ formation (Benková et al. 2003), and plant regeneration (Xu et al. 2006). The PIN-FORMED PROTEIN5 (PIN5) protein does not function in cell-to-cell transport but plays a vital role in transporting auxin from the cytoplasm to the endoplasmic reticulum (ER); it also promotes indole-3-acetic acid (IAA) conjugation by GRETCHEN HAGEN3 (GH3) proteins and eventually leads to a decrease in free IAA content in cells and dwarf plants (Mravec et al. 2009). However, the molecular mechanism of the auxin-signaling pathway in citrus fruit size formation has not been well understood.
MYB family TFs constitute one of the largest TF families in plants (Li et al. 2016). Among them, R2R3-TFs respond positively to ABA and auxin to regulate fruit ripening and plant organ size. MYB70 delays tomato fruit ripening by directly binding to the promoters of ACS2 and ACO3 and inhibiting their expression (Cao et al. 2020). MYB68 delays citrus fruit ripening by inhibiting NCED5 (Zhu et al. 2017). The OE of MYB77 in Arabidopsis results in smaller organs (Shin et al. 2007), and MYB77 is regulated by ABA and interacts with the ARF7 protein to modulate downstream auxin signaling (Zhao et al. 2014).
In our previous study, the R2R3-MYB gene CsMYB77 was identified from a sweet orange (Citrus sinensis) late-ripening mutant (MT) by RNA-seq (Wu et al. 2016). At the ripening stage, the expression of CsMYB77 was higher in MT than in its wild type (WT; Wu et al. 2016). Consequently, we postulated that CsMYB77 might be associated with citrus fruit ripening. Subsequently, we confirmed the function of CsMYB77 through a genetic transformation of tomato and Hongkong kumquat, resulting in the inhibition of fruit ripening and a reduction in fruit size in Hongkong kumquat. Furthermore, CsMYB77 was found to directly target SINAT4/PIN5, thereby participating in the ABA/AUXIN regulation of fruit development and ripening.
Results
Characterization of CsMYB77 in citrus
Sequence analysis showed that CsMYB77 occupied 765 bp of the coding sequence that encoded 255 amino acids. Annotation analysis revealed that CsMYB77 shared ∼78% identity with AtMYB77 (At3g50060) and belonged to the R2R3-MYB 22nd subtribe with AtMYB44, AtMYB70, and AtMYB73 (Supplemental Fig. S1). We also analyzed the expression patterns of CsMYB77 in 4 tissues of sweet orange fruit across 6 developmental periods. As shown in Fig. 1A, CsMYB77 had the highest expression levels at the early fruit development stage, the expression of which was continuously downregulated with fruit development until 120 d after flowering (DAF) in the 4 fruit tissues. Notably, during citrus fruit ripening periods (155 to 220 DAF), the expression of CsMYB77 was also continuously downregulated in albedo (AL), segment membrane (SM), and juice sac (JS) tissues. Overall, the expression of CsMYB77 decreased with fruit development and ripening, which suggested that CsMYB77 might be a negative regulator of fruit development and ripening. Subcellular localization analysis revealed that CsMB77 resided in the nucleus (Fig. 1B). In addition, we analyzed the transcriptional activation activity of CsMYB77, and the results confirmed transcriptional activation activity (Fig. 1C).

Gene characterization of CsMYB77. A) The expression pattern of CsMYB77 in the epicarp (EP), AL, SM, and JS at 50, 80, 120, 155, 180, and 220 DAF. B) Subcellular localization of CsMYB77 in N. benthamiana leaves. The coding sequence of CsMYB77 was constructed upstream of the 35S promoter in PRI101-GFP vector. Scale bar, 10 μm. C) Transcriptional activation analysis of CsMYB77.
The OE of CsMYB77 significantly delayed fruit ripening in tomato
To determine the role of CsMYB77 in fruit ripening, the CDS of CsMYB77 was overexpressed in tomato plants driven by the 35S promoter. Two independent transgenic lines were generated (#1 and #3) by assessing the expression level and protein abundance in plants (Fig. 2, A and B). Consistent with our speculation, late-ripening phenotypes were observed in the 2 positive lines (Fig. 2C). We analyzed the days from anthesis to the breaker (BR) stage. CsMYB77-OE fruits delayed BR by 3 to 5 d compared with WT fruits (Fig. 2D). Furthermore, the content of ethylene, ABA, and carotenoids was lower in OE fruits than in WT fruit at the BR stage. The content of chlorophyll was higher in OE fruit than in WT fruits at the BR stage (Fig. 2E). These results indicate that CsMYB77 could negatively regulate fruit ripening in climacteric fruit.
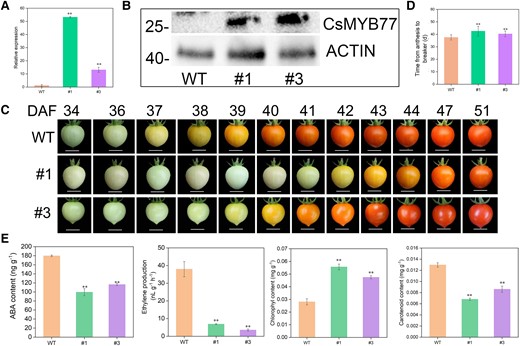
OE of CsMYB77 delayed fruit ripening in transgenic tomato lines. A) Relative expression of CsMYB77 in 2 independent OE lines and WT lines by RT-qPCR. B) Western blot analysis of fruit samples was conducted to detect the presence of the CsMYB77 protein (28.05 kDa), using a gene-specific antibody, comparing it between the WT and stable transgenic lines (#1 and #3). C) Representative phenotypes of fruit ripening in OE and WT plants at different days. Images were digitally extracted for comparison. Scale bar, 1 cm; WT (n = 16), #1 (n = 24), and #3 (n = 19) were marked at 0 DAF. D) Days from anthesis to the BR stage in WT, and OE fruits. WT (n = 16), #1 (n = 24), and #3 (n = 19) were analyzed. Values are the means ± Se. E) Ethylene production, the content of carotenoids, chlorophyll and ABA in WT and OE fruits at BR stage. Values are the means ± Se of 3 biological replicates in A) and E), and asterisks indicate statistical significance in A), D), and E) determined by Student's t-test: **P < 0.01 compared with WT plants.
CsMYB77 negatively regulates citrus fruit ripening
To elucidate whether CsMYB77 has the same delayed ripening function in citrus as in tomato, the CDS of CsMYB77 was genetically transformed into Fortunella hindsii. After 3 yr, 4 positive lines past their juvenile phase were identified by using polymerase chain reaction (PCR) assay (Supplemental Fig. S2). Two robust plants (#1 and #10) were selected and reverse transcription-quantitative PCR (RT-qPCR) was used to detect the expression levels of CsMYB77, which were significantly upregulated in the ripening fruits of the transgenic lines (#1 and #10; Fig. 3A). We used western blotting to detect the protein abundance of CsMYB77 and found that the transgenic lines accumulated more MYB77 protein than the WT lines in ripening fruits (Fig. 3B). Consistent with the expected results, we observed late-ripening phenotypes in transgenic lines (Fig. 3C). We analyzed the proportion of fruit color in different developmental stages (Fig. 3D). Compared with WT fruits, color breaking and full ripening occurred 20 to 40 d later in transgenic fruits. In view of the important regulation of ABA in fruit ripening, fruits sampled at 170 DAF (color breaking stage) were used to measure ABA content. The results showed that the ABA concentration of the fruits of the transgenic lines was lower than that of the WT lines (Fig. 3E). We also detected higher chlorophyll content and lower carotenoid content in the transgenic line fruits than in the WT line fruits (Fig. 3E). These results revealed that the OE of CsMYB77 resulted in late ripening of the citrus fruit.
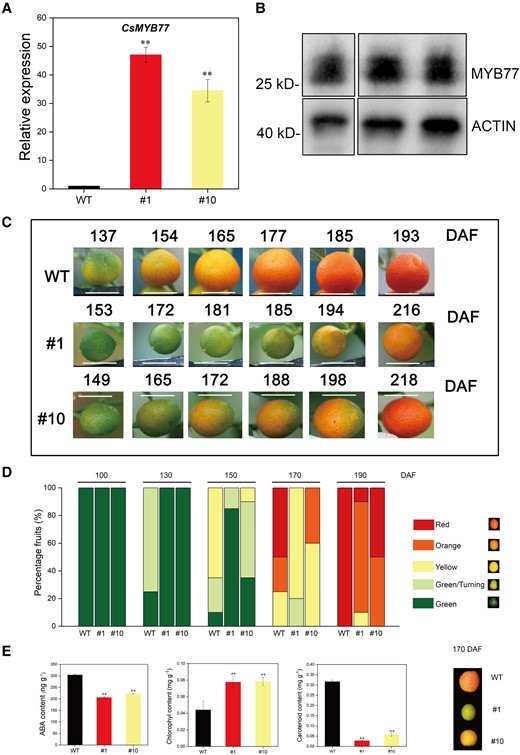
OE of CsMYB77 significantly delayed fruit ripening in transgenic Hongkong kumquat lines. A) Relative expression of CsMYB77 in WT and stable transgenic lines (#1 and #10) determined by RT-qPCR in ripening fruits at 170 DAF. CsActin was used as an internal reference. B) Western blotting of ripening fruits was used to detect CsMYB77 protein (28.05 kDa) by the use of a gene-specific antibody between WT and stable transgenic lines (#1 and #10) at 170 DAF. Actin is a universal plant-specific antibody (42 kDa). C) Dynamic color images of fruits of each line at different developmental stages. Representative pictures of a single fruit were obtained, a total of 20 fruits were marked at 0 DAF. Scale bar, 1 cm. D) Percentage of fruit of each color for each line in the same day, n = 20. E) Measurement of ABA, chlorophyll, and carotenoids. Values are the means ± Se of 3 biological replicates in A) and E), and asterisks indicate statistical significance determined by Student's t-test: **P < 0.01 compared with WT plants.
The OE of CsMYB77 reduced fruit size in citrus
Through observation for 2 consecutive years, we quantified the single fruit weights of the WT, #1, and #10 lines. We were surprised to find that the fruit size of the CsMYB77-OE lines was smaller than that of the WT lines (Fig. 4A), and the single fruit weight of the transgenic lines decreased by 40.43% to 50.18% compared with that of the WT lines (Fig. 4B). In addition, the fruit shape indices of the 2 transgenic lines also significantly changed (Fig. 4B). Moreover, the seed size of Lines #1 and #10 was significantly smaller than that of the WT (Fig. 4C), and the seed weight was reduced by 53.22% to 73.22%, but there was no significant difference in the average seed number per fruit between the WT and the transgenic lines (Fig. 4D).
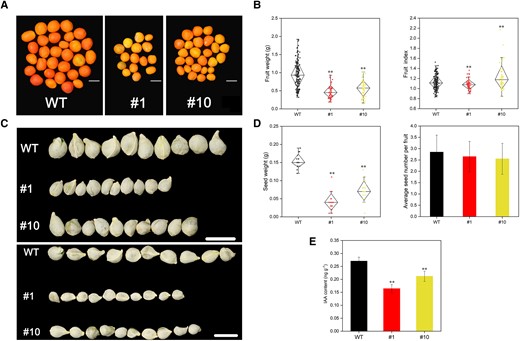
OE of CsMYB77 significantly reduced fruit size in transgenic Hongkong kumquat lines. A) Fruit size phenotype of WT, #1, and #10 lines at 220 DAF. Scale bar, 1 cm. Images were digitally extracted for comparison. B) Fruit weight and fruit index. WT (n = 192), #1 (n = 62), and #3 (n = 59); scale bar, 1 cm. Values are the means ± Sd. Images were digitally extracted for comparison. The number of plots (independent samples) was indicated above the boxes. Center line, median; box limits, upper and lower quartiles; whiskers, 1.5× interquartile range; points, outliers. C) Seed phenotypes in WT, #1, and #10 lines. Scale bar, 1 cm. Images were digitally extracted for comparison. Representative pictures of a single seed for each line from 20 fruits, where 2 rows from the same groups showed the seed size differences compared with WT. D) Seed weight and average seed number per fruit, n = 20. Values are the means ± Se. The number of plots (independent samples) was indicated above the boxes. Center line, median; box limits, upper and lower quartiles; whiskers, 1.5× interquartile range; points, outliers. E) Measurement of IAA in WT, #1, and #10 lines. Values are the means ± Se of 3 biological replicates in E), and asterisks indicate statistical significance in B), D), and E) determined by Student's t-test: **P < 0.01 compared with WT plants.
Plant organ size is often associated with auxin content (Li et al. 2021). With the development and ripening of the fruit in sweet orange, the auxin concentration first increased and then decreased, reaching the highest value around the fruit color-breaking stage (Feng et al. 2021). Therefore, the fruits sampled at 170 DAF were used to measure IAA concentration. The results showed that the IAA content in the transgenic lines decreased by 21.72% to 39.28% compared with that in the WT lines (Fig. 4E). These results demonstrated that CsMYB77 played a role in regulating citrus fruit size.
Identification of the target genes of CsMYB77 by DNA affinity purification sequencing
DNA affinity purification sequencing (DAP-seq) is able to search for DNA sequences binding to target proteins throughout the genome (O’Malley Ronan et al. 2016). The peak accounted for 29.12% in the promoter region (Supplemental Fig. S3A), where 3802 peaks were enriched within 2,000 bp from the transcription start site (Supplemental Data Set 1). Gene ontology (GO) enrichment analysis showed that the main functions of peak genes were membrane processes such as endomembrane system, membrane-bounded organelle, and anchored component of membrane, and 3 genes were involved in the ubiquitination process (Fig. 5A). Kyoto encyclopedia of genes and genomes (KEGG) enrichment analysis revealed that these genes were not only involved in metabolic pathways but also in processes related to the control of protein quality, such as autophagy and ubiquitin biosynthesis (Fig. 5B). GO and KEGG enrichment analyses showed that CsMYB77 could not directly regulate ABA-related processes, so we hypothesized that CsMYB77 might be indirectly involved in the regulation of ABA-related biological processes, such as through ubiquitination, the autophagy-vacuole pathway and vacuolar sorting. We screened a candidate gene, orange1.1t02726, affecting plant sensitivity to ABA. It was located on the unassembled chromosome (Supplemental Fig. S3B), and the promoter contained the binding motif (ATTTACAAGTCTTAT) of CsMYB77 (Fig. 5C). It was named CsSINAT4 (Supplemental Fig. S3C) and contained a RING domain at the N-terminus, ZINC in the middle, and a TUMOR NECROSIS FACTOR RECEPTOR-ASSOCIATED FACTOR (TRAF) domain at the C-terminus for the protein‒protein interaction (Supplemental Fig. S3D). Subcellular localization analysis showed that CsSINAT4 resided at punctate structures in the cell membrane (Fig. 5D). Furthermore, we hypothesized that CsMYB77 might regulate the expression of CsSINAT4.
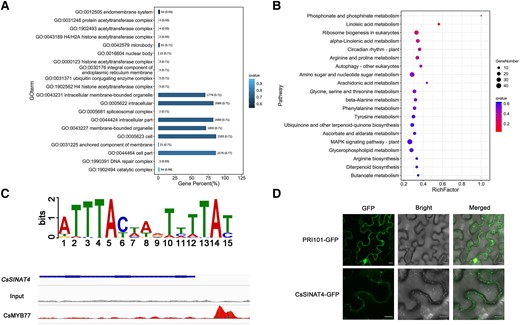
DAP-seq analysis of CsMYB77 and subcellular localization analysis of CsSINAT4. A, B) Gene ontology A) and Kyoto Encyclopedia of Genes and Genomes results B) for CsMYB77-binding genes. C) The binding motif of CsMYB77 and INTEGRATIVE GENOMICS VIEWER (IGV) image of CsMYB77 binding to CsSINAT4. D) Subcellular localization of CsSINAT4. Scale bars, 10 μm.
CsMYB77 negatively regulates SINAT4 to modulate ABA signaling in citrus and tomato
To verify whether CsMYB77 directly targeted the promoter sequence of CsSINAT4, we introduced the promoter fragment of CsSINAT4 into yeast 1-hybrid (Y1H) and dual-luciferase systems. The results showed that CsMYB77 inhibited the transcription level of CsSINAT4 in vivo (Fig. 6, A and B). Meanwhile, electrophoretic mobility shift assay (EMSA) was also conducted to prove that CsMYB77 can directly bind to CsSINAT4 to produce the shift band in vitro (Fig. 6C). Then, RT-qPCR was used to detect the transcriptional level of SINAT4 in Hongkong kumquat lines. The results showed that the expression level of SINAT4 in the transgenic lines was significantly lower than that in WT lines (Fig. 6D). Therefore, we assumed that CsMYB77 might negatively regulate CsSINAT4 and affect the abundance of downstream interacting proteins. We prepared specific antibodies against CsFREE1, CsVPS23A, CsPYR1, and CsPYL4. Amino acid sequence alignment analysis revealed similarity between 4 proteins in sweet orange and Arabidopsis (A. thaliana) functional domains (Supplemental Fig. S4). Subsequent western blot experiments revealed that the protein abundances of FREE1 and VPS23A were increased considerably and the protein abundances of PYR1 and PYL4 were decreased in transgenic lines compared with in WT lines (Fig. 6E). However, at the transcription level, the expression levels of FREE1, VPS23A, and PYR1 were downregulated in the transgenic lines, except for PYL4 (Supplemental Fig. S5), which indicated that the expression patterns at the transcriptional and protein levels were different. This result suggested that posttranslational regulation might play an important role in this process. Additionally, the transcriptional levels of the ABA biosynthesis gene NCED2 and ABA response genes, including ABF2 and ABI5, were downregulated (Fig. 6F).
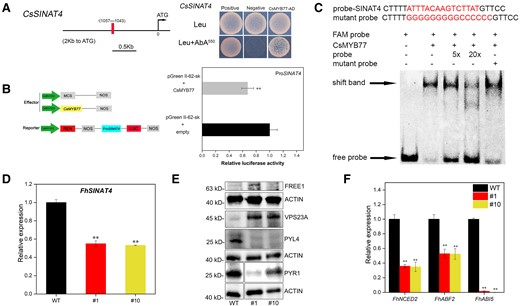
CsMYB77 negatively regulated CsSINAT4 to modulate the protein and transcript levels of related genes in both Hongkong kumquat and tomato fruits. A) Y1H analysis of CsMYB77 directly targeting the CsSINAT4 promoter fragment. The prey vector, CsMYB77-pGADT7; the bait vector, SINAT4-pAbAi. Positive control, pGADT-Rec2-53; negative control, pGADT7 + SINAT4-pAbAi. B) Dual-luciferase system for the detection of CsMYB77 targeting CsSINAT4. LUC, firefly luciferase activity; REN, Renilla luciferase. C) EMSA showing the binding of CsMYB77 to CsSINAT4. “+,” presence; “−,” absence; 5- and 20-fold excesses of nonlabeled probes and mutant probes were used for competitor. D) Relative expression of SINAT4 in Hongkong kumquat lines. E) Western blot of FREE1 (63 kDa), VPS23A (45 kDa), PYL4 (28 kDa), PYR1 (28 kDa), and Actin (42 kDa) in Hongkong kumquat. F) Relative expression of NCED2, ABF2, ABI5, in Hongkong kumquat lines. Values are the means ± Se of 3 biological replicates in B), D), and F), and asterisks indicate statistical significance determined by Student's t-test: **P < 0.01 compared with WT plants.
In addition, the promoters of SlSINAT4 and FhSINAT4 also owned CsMYB77-binding motif, indicating that CsMYB77 was able to regulate SINAT4 in tomato and Hongkong kumquat lines (Supplemental Text S1). In tomato, dual-luciferase systems and EMSA proved that CsMYB77 bound to SlSINAT4 and negatively regulated its transcription levels (Fig. 7, A and B). Meanwhile, the expression levels of SlPYR1, SlPYL4, SlABF2, SlABI5, SlNCED5, SlPSY1, SlACO1, SlACS4, SlNOR, and SlRIN were significantly downregulated in tomato OE lines (Fig. 7C). These results indicated that CsMYB77 inhibited the expression of SINAT4 to modulate ABA signaling in both citrus and tomato.
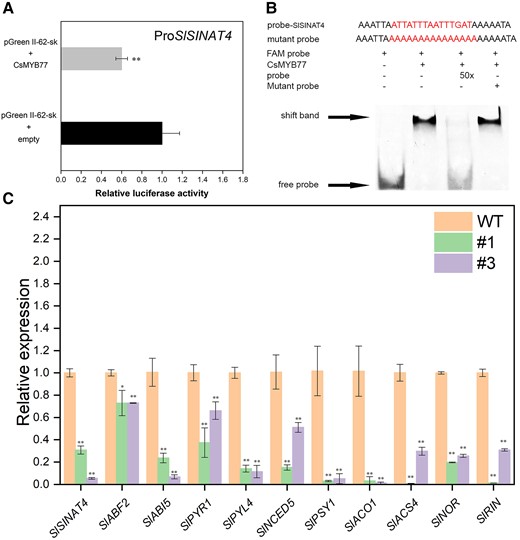
CsMYB77 negatively regulated SlSINAT4 to inhibit genes related to ripening. A) Dual-luciferase system for the detection of CsMYB77 targeting SlSINAT4. B) The binding of CsMYB77 to SlSINAT4 using EMSA. C) Relative expression of genes at BR stage in tomato. Values are the means ± Se of 3 biological replicates in A) and C), and asterisks indicate statistical significance determined by Student's t-test: **P < 0.01, *P < 0.05 compared with WT plants.
CsMYB77 positively regulates PIN5 to modulate IAA homeostasis
To uncover the molecular mechanism by which CsMYB77 regulates fruit size in citrus, we analyzed DAP-seq data to identify the related target genes. We screened out an auxin transporter gene Cs6g02450 (Fig. 8A). After amino acid sequence alignment with the PIN family of A. thaliana, we named it CsPIN5 (Supplemental Fig. S6A). Then, we carried out Y1H, dual luciferase, and EMSA interaction experiments. The results showed that CsMYB77 could directly target the promoter of CsPIN5 and activate its expression (Fig. 8, B to D). RT-qPCR was used to analyze the transcription level of FhPIN5, which contained the same motif as CsPIN5 (Supplemental Text S1) in the promoter region. Compared with that in the WT lines, the expression of PIN5 in the fruits of the transgenic lines was significantly upregulated (Fig. 8E). For other PINs, the expression of PIN2 and PIN6 was significantly downregulated in the transgenic lines and other PIN genes showed inconsistent expression patterns in the 2 transgenic lines (Supplemental Fig. S6B). This result indicated that auxin transport genes were directly or indirectly regulated by CsMYB77. Moreover, we also detected the expression levels of GRETCHEN HAGEN3.6 (GH3.6), ARF6, ARF8, and ARF19. As shown in Fig. 8F, the expression of GH3.6 was significantly upregulated in the transgenic lines. GH3 proteins catalyze the conjugation of IAA to amino acids, thereby reducing free IAA (Staswick et al. 2005). Notably, the expression levels of ARFs were downregulated in the transgenic lines (Fig. 8F). Overall, these results revealed that CsMYB77 could positively regulate the expression of PIN5 to modulate IAA homeostasis and auxin signal transduction.
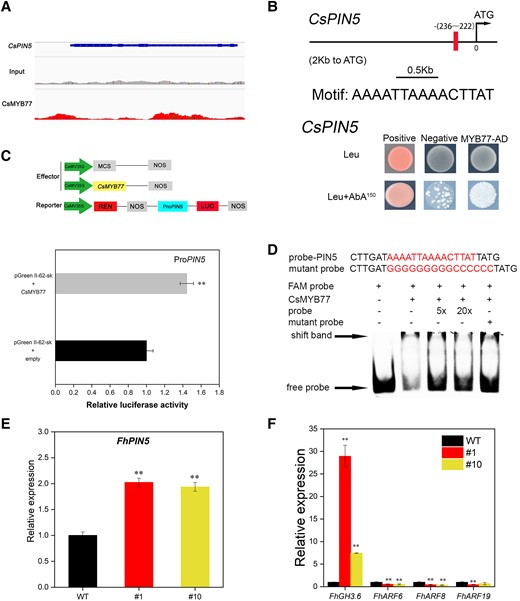
CsMYB77 positively regulated CsPIN5 to modulate the expression levels of auxin-signaling-related genes in Hongkong kumquat fruits. A) IGV image of CsMYB77 binding to CsPIN5. B) Y1H analysis of CsMYB77 directly targeting the CsPIN5 promoter fragment. C) Dual-luciferase system for the detection of CsMYB77 targeting CsPIN5. D) EMSA showing CsMYB77 the binding of CsMB77 to CsPIN5. E, F) Relative expression levels of PIN5, GH3.6, ARF6, ARF8, and ARF19 in Hongkong kumquat line fruits. Values are the means ± Se of 3 biological replicates in C), E), and F), and asterisks indicate statistical significance determined by Student's t-test: **P < 0.01 compared with WT plants.
Transiently overexpressing CsSINAT4 leads to the early ripening of citrus and tomato fruit
To further validate the function of the target gene of CsMYB77, we performed transient OE of CsSINAT4 in both tomato and Hongkong kumquat fruits. As shown in Fig. 9A, OE of CsSINAT4 significantly promoted the ripening of tomato and citrus fruits.
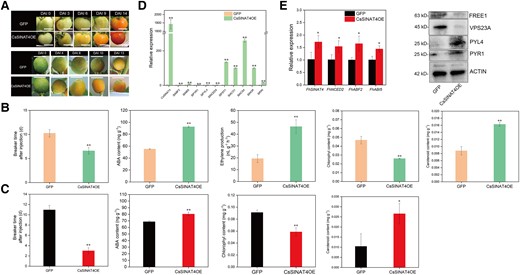
Transient OE of CsSINAT4 in tomato and Hongkong kumquat fruits. A) Transiently overexpressing CsSINAT4 in tomato and Hongkong kumquat fruits, n = 20. Day after injection (DAI). Scale bar, 1 cm. B) The BR time (values are the means ± Se of 20 biological independent samples), ethylene production, the content of carotenoids, chlorophyll, and ABA in tomato fruits at BR stage. C) The BR time (values are the means ± Se of 20 biological independent samples), the content of ABA, carotenoids, and chlorophyll in Hongkong kumquat fruits at BR stage. D) Relative expression of the previous genes in tomato, including CsSINAT4, ABF2, ABI5, PYR1, PYL4, NCED5, PSY1, ACO1, ACS4, NOR, and RIN. E) Relative expression of genes (SINAT4, NCED2, ABF2, and ABI5) and western blot of PYR1, PYL4, FREE1, and VPS23A in Hongkong kumquat. Values are the means ± Se of 3 biological replicates in B) except the BR time, C) except the BR time, D, E), and asterisks indicate statistical significance in B to E) determined by Student's t-test: **P < 0.01, *P < 0.05 compared with GFP fruits.
In tomato, the color-break stage of CsSINATOE lines was advanced by ∼5 d compared with the control group of GFP lines (Fig. 9B). Additionally, the contents of ethylene, ABA and carotenoids were higher in CsSINAT4OE lines, while the content of chlorophyll was lower (Fig. 9B). Moreover, the expression levels of the genes that positively regulate fruit ripening were upregulated in CsSINAT4OE lines, including ABA-signaling-related genes (ABF2, ABI5, PYR1, PYL4, and NCED5), ethylene-related genes (ACO1, ACS4, NOR, and RIN), and color-formation-related genes (PSY1; Fig. 9D).
In citrus, the same results were obtained as in tomato (Fig. 9, A and C). Compared with GFP lines, the ripening period of CsSINAT4OE lines was advanced about 8 d (Fig. 9, A and C). As shown in Fig. 9, C and E, the content of ABA was higher in CsSINAT4OE lines, and ABA-signal-pathway-related genes (FhABF2, FhABI5, and FhNCED2) were also upregulated in CsSINAT4OE lines. In addition, the protein abundances of PYR1 and PYL4 were upregulated in CsSINAT4OE lines, and the protein abundances of FREE1 and VPS23A were downregulated (Fig. 9E).
These results are contrary to the results of CsMYB77-OE lines (Figs. 2 and 3), indicating that CsSINAT4 is a positive fruit ripening regulator. Hence, inhibiting the expression of CsSINAT4 can negatively regulate fruit ripening. This result is consistent with our above conclusion.
Fruit size was reduced by transiently overexpressing CsPIN5 in citrus
The young fruits (before the fruit expansion period) of Hongkong kumquat were selected to perform transient OE of CsPIN5. Compared with GFP lines, the weight of fruit or seed was largely reduced 46.62% or 72.01% in CsPIN5OE lines, and the content of IAA was also reduced significantly (Fig. 10, A to C). Moreover, the expression of FhGH3.6 was upregulated, and the expressions of FhARF6, FhARF8, and FhARF19 were downregulated in CsPIN5OE lines (Fig. 10D). This result demonstrated that CsPIN5 could significantly negatively regulate citrus fruit size, which is consistent with the result of OE of CsMYB77.
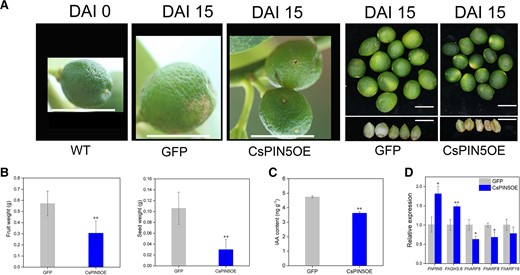
Transient OE of CsPIN5 in Hongkong kumquat fruits. A) Transiently overexpressing CsPIN5 in young Hongkong kumquat fruits. Smaller fruits and poorly developed seeds in CsPINOE fruits compared with GFP fruits. Scale bar, 1 cm. B) The fruit and seed weight in CsPIN5OE and GFP fruits. n = 17. Values are the means ± Se. C) The content of IAA in the GFP and CsPIN5OE lines. D) Relative expression of genes. Values are the means ± Se of 3 biological replicates in C, D), and asterisks indicate statistical significance in (B to D) determined by Student's t-test: **P < 0.01, *P < 0.05 compared with GFP fruits.
Discussion
In this study, we characterized a negative regulator of fruit ripening and development, CsMYB77. OE of CsMYB77 in tomato and citrus resulted in delayed ripening phenotypes, and smaller fruit size in transgenic lines of citrus. Two candidate genes were screened by DAP-seq, and it was verified that CsMYB77 could directly target the promoters of SINAT4 and PIN5 to regulate the ripening and size of citrus fruit. Subsequent transient transformation of CsSINAT4 in tomato and citrus resulted in early fruit ripening and transient transformation of CsPIN5 in citrus obtained smaller fruits.
CsMYB77 negatively regulates fruit ripening in both climacteric and nonclimacteric fruit by modulating ABA signaling
Fruit ripening is an extremely complex process involving a series of physiological and biochemical reactions, where ABA plays a critical role in both climacteric and nonclimacteric fruit. For climacteric fruit, for instance, exogenous ABA treatment could promote tomato fruit ripening (Zhang et al. 2009a, 2009b). Silencing the ABA receptor gene SlRCAR inhibited tomato fruit ripening by downregulating ACS and ACO gene transcription (Zou et al. 2022). Suppressing SlNCED1 acutely decreased the content of ABA, which substantially delayed tomato fruit ripening (Sun et al. 2012). Moreover, the application of ABA can also lead to accelerated ripening in banana (Jiang et al. 2000) and mango (Mangifera indica L.; Zaharah et al. 2013). For nonclimacteric fruit, numerous studies have proved that ABA and ABA response genes play important roles in fruit ripening in strawberry, grape (Vitis vinifera L.), citrus, and so on. For example, strawberry fruit ripening was significantly delayed by silencing ABA receptor gene FaPYR1 using virus-induced gene silencing (Chai et al. 2011). In citrus, the HOMEODOMAIN LEUCINE ZIPPER I TF CsHB5 enhanced ABA sensitivity, thereby promoting fruit ripening (Zhang et al. 2021). Moderate concentrations of ABA could promote grape peel coloring, leading to early fruit ripening (Cecilia Peppi et al. 2008).
Our previous study showed that CsABF2 acted as the magnifier of ABA signaling and regulated various metabolic pathways related to citrus fruit ripening. In addition, CsABF2 could directly interact with CsNCED2/5 and activate its expression (Feng et al. 2021). In this study, overexpressing CsMYB77 in both tomato and citrus resulted in significantly delayed fruit ripening phenotypes (Figs. 2C and 3C). Moreover, the ABA content, expression of ABA-signaling genes (NCED2/5, PYL4, PYR1, ABF2, and ABI5) and ABA-signaling protein abundance were largely changed (Figs. 2E, 3E, 6F, and 7C). This result suggested that CsMYB77 might negatively regulate fruit ripening in both climacteric and nonclimacteric fruit by modulating ABA signaling.
There, the question arises: how does CsMYB77 regulate ABA signaling? In this study, the RING-type E3 ubiquitin ligase gene SINAT4 was identified to be targeted by CsMYB77 (Figs. 5 and 6, A to C). More recently, a study showed that SINATs (SINAT1/2/3/4) mediated the ubiquitination and degradation of FREE1 and VPS23A to modulate ABA signaling in Arabidopsis. SINAT OE lines showed increased ABA sensitivity, ABA-responsive gene expression, and PYL4 protein levels (Xia et al. 2020). In plants, FREE1 and VPS23A are key components of the ESCRT and function in regulating MVB biogenesis and the endosomal sorting of membrane proteins (Spitzer et al. 2006; Gao et al. 2014). FREE1 and VPS23A are involved in the negative regulation of ABA signaling by recognizing the ABA receptors PYR1/PYL4 for vacuole-mediated degradation (Belda-Palazon et al. 2016; Yu et al. 2016). In this study, SINAT4 was repressed by CsMYB77, that increased the protein abundances of FREE1 and VPS23A, and then promoted the degradation of PYR1/PYL4 to modulate ABA signaling (Fig. 6, D and E). However, overexpressing CsSINAT4 resulted in the opposite process (Fig. 9). Combined with previous study results, as shown in Fig. 11, a hypothetical regulatory model of CsMYB77-SINAT4-FREE1/VPS23A-PYR1/PYL4-ABA signaling was depicted.
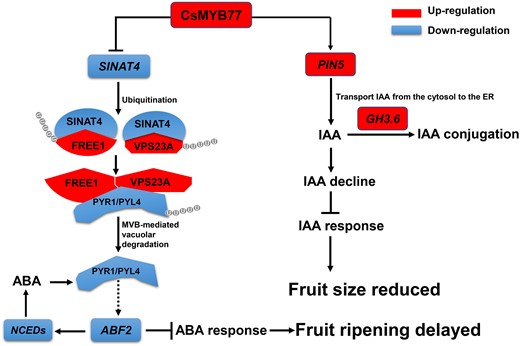
A proposed mode of CsMYB77 regulating fruit ripening and fruit size in citrus. The transcription level of SINAT4 is significantly inhibited after stable genetic transformation of CsMYB77 in vivo. As a result, a series of protein interaction reactions are affected. Specifically, the protein contents of FREE1 and VPS23A increase and the abundance of PYR1 and PYR1-LIKE4 (PYL4) decreases, which further weakens the expression levels of ABF2 and NCEDs mediating ABA synthesis, and eventually leads to late maturity of the fruits. Meanwhile, the expression level of PIN5 is significantly upregulated enabling more polar transport of IAA to the ER and upregulation of GH3.6 transcription, which reduces the content of free IAA and auxin signal transduction in fruit cells. OE of CsMYB77 ultimately leads to smaller fruits.
CsMYB77 negatively regulates the fruit size of citrus by modulating auxin homeostasis
Fruit size is determined by fertilization, pistil development, and formation, and the continuous process of fruit enlargement during cell differentiation, proliferation, and expansion (Wang et al. 2016). Auxin is positively involved in these processes and regulates fruit size. Arabidopsis exhibits a short silique phenotype when the soybean gene GmYUCCA5 is genetically transformed (Wang et al. 2017). PROTEASOME REGULATOR1 (PTRE1) is involved in auxin degradation, and the mutant, pter1, also causes shorter siliques (Yang et al. 2016). In tomato, ARF9 inhibits cell differentiation in fruit and its low transcriptional level causes fruit enlargement (de Jong et al. 2015). However, downregulation of SlARF5 expression resulted in smaller seedless fruits (Liu et al. 2018). Moreover, downregulation of SlIAA17 transcription results in larger fruit sizes (Su et al. 2014). In this study, OE of CsMYB77 in Hongkong kumquat resulted in decreased fruit size, auxin content, and auxin response gene expression (Figs. 4 and 8F). These results suggested that CsMYB77 affected auxin homeostasis and auxin signal transduction.
Auxin transporters and IAA-amido synthetase GH3s play important roles in auxin homeostasis (Bottcher et al. 2010; Kang et al. 2013). In this study, the gene encoding the auxin transporter CsPIN5 was identified as the target gene of CsMYB77 and an IAA-amido synthetase-encoding gene GH3.6 was largely upregulated in transgenic Hongkong kumquat lines (Fig. 8). In Arabidopsis, PIN5 functions by transporting auxin from the cytoplasm to the ER, and promoting IAA conjugation by GH3s for degradation (Mravec et al. 2009). OE of PIN5 resulted in a dwarf phenotype with decreased levels of free IAA (Mravec et al. 2009). The mutants overexpressing GH3.2/YDK1 (Takase et al. 2004), GH3.6/DFL1 (Nakazawa et al. 2001), and GH3.8 (Ding et al. 2008) showed dwarf or retarded growth phenotypes consistent with decreased levels of free IAA. Meanwhile, we also overexpressed CsPIN5, which led to the same result (Fig. 10). Hence, we proposed that CsMYB77 modulated auxin homeostasis by positively regulating PIN5, which resulted in decreased IAA content, auxin responding gene expression and fruit size (Fig. 11).
However, tomato lines overexpressing CsMYB77 did not change the fruit size (Fig. 2C), indicating different regulatory mechanisms of fruit size between citrus and tomato. We aligned the promoter of SlPIN5 and found no binding sequence of CsMYB77 (Supplemental Text S1), and the expression level of SlPIN5 was no difference in OE lines compared with WT (Supplemental Fig. S7), which explained why the OE of CsMYB77 did not change tomato fruit size.
Materials and methods
Plant materials and growth conditions
The leaves of “Fengjie 72-1” orange (C. sinensis L. Osbeck) were used for gene amplification. Micro-Tom tomato (S. lycopersicum) was selected as the tomato genetic transformation material. The WT lines and the transgenic lines of tomato were cultivated at 22 °C with a photoperiod that included 16 h of light followed by 8 h of dark in a climate chamber. Hongkong kumquat (F. hindsii) was used as a genetic transformation material to verify the gene function in citrus. The WT and the transgenic lines of Hongkong kumquat were cultivated for 8 mo in a climate chamber at 25 °C with a photoperiod of 16 h of light followed by 8 h of dark. Afterwards, the WT and transgenic lines of Hongkong kumquat were transplanted to a greenhouse at 8 to 33 °C under natural light.
RNA extraction and gene expression analysis
RNA samples were isolated as described previously (Liu et al. 2007) using a FastPure Universal Plant Total RNA Isolation Kit (RC411; Vazyme Biotech). RNA quality was evaluated using Denovix 2017 (Bio-SUN). The mRNA was reverse-transcribed using the HiScriptIIQ RT SuperMix for qPCR (+gDNA wiper) kit. CsACTIN was used as the housekeeping gene. RT-qPCR was used for gene expression analysis which was performed using the QuantStudio 6 Flex real-time PCR system (Applied Biosystems, USA) and 384-well plates. All data were analyzed using the 2−ΔΔCt analysis method as described by Bustin et al. (2009). The RNA-seq raw data can be obtained from the accession number GSE125726 in NCBI (Feng et al. 2021). All primers were listed in Supplemental Table S1.
Western blotting
Five specific antibodies (against CsMYB77, CsFREE1, CsVPS23A, CsPYR1, and CsPYL4) were prepared, and the experiments were completed by the company by the company Dai-an of Wuhan, China. Protein extraction and western blotting were described previously (Cao et al. 2012). Anti-β-actin mouse mAb protein was used for actin detection (ABclonal: AC009, Wuhan, China).
Gene vector construction and transformation
To generate the CsMYB77-OE construct pBI121 (35Spro:CsMYB77), the full CsMYB77 CDS was amplified from “Fengjie 72-1” orange fruit cDNA with XbaI and SacI recombination arms using Phanta Super-Fidelity DNA Polymerase (Vazyme). The primers were listed in Supplemental Table S1. The PCR products were first cloned into p-TOPO (Aidlab, China) vectors. Then, double enzyme digestion was used to construct fusion vectors. Agrobacterium tumefaciens-mediated transformation of tomato was performed, as previously described (Wang et al. 2005). Agrobacterium tumefaciens (EHA105)-mediated transformation of Hongkong kumquat was performed, as previously described (Zhu et al. 2019). All positive plants were screened by PCR amplification of genomic DNA using the primer pairs listed in Supplemental Table S1.
Subcellular localization of CsMYB77 and CsSINAT4
The 35S:CsMYB77/CsSINAT4-GFP fusion construct was generated by inserting the CDS from CsMYB77/CsSINAT4 without a stop codon into the PRI101-GFP vector with SalI and Acc65I digestion. The CsMYB77/CsSINAT4-GFP construct was transformed into Nicotiana benthamiana leaves by Agrobacterium-mediated infiltration (Sparkes et al. 2006). Three days later, the fluorescence signal was acquired using a confocal laser scanning microscope (TCS SP8; Leica) with the following properties for GFP: laser, 488 nm, intensity, 1.0005%, collection bandwidth 505 to 545, gain, below 800, detector, PMT. The following properties for m-cherry: laser, 552 nm, intensity, 1.0005%, collection bandwidth, 595 to 635, gain, below 800, detector, PMT. The primers were listed in Supplemental Table S1 used in this experiment.
Electrophoretic mobility shift assay
The CDS of CsMYB77 was cloned into the Pet-32a vector (His-tag) with EcoRI and SalI digestion to obtain the fusion protein. Then, the 5′-FAM-labeled probes, including CsSINAT4 and CsPIN5, were synthesized and labeled by WuHan TianYi HuaYu Gene Technology Co., Ltd. The experiment was performed as previously described (Zhang et al. 2021). The primers were listed in Supplemental Table S1 used in this experiment.
Dual-luciferase assays
The full-length CsMYB77 ORF was amplified and cloned into the effector vector pGreen II62-SK via BamHI and HindIII digestion, and the promoter fragments were ligated to the pGreenII-0800-LUC vector. The constructs were transformed into A. tumefaciens GV3101 with the pSoup helper plasmid. Agrobacterium transformed with the effector and reporter construct was mixed at a ratio of 10:1 and then infiltrated into N. benthamiana leaves. The concrete operation method was performed, as previously described (Hellens et al. 2005). The pGreenII-62-SK-LUC vector without the CsMYB77 gene was used as a negative control. After 3 d of inflection, firefly luciferase and Renilla luciferase activities were assayed using dual-luciferase assay reagents (Promega) with an Infinite M200 (Tecan) plate reader. LUC was used as the reporter gene and REN was used as the internal reference gene. The activity of transactivation was presented as a ratio of LUC to REN. The primers were listed in Supplemental Table S1 used in this experiment.
Y1H assay
The full CsMYB77 CDS was amplified from the “Fengjie 72-1” orange fruit cDNA and cloned into the pGADT7 vector via BamHI and XhoI digestion. The primers were listed in Supplemental Table S1. The promoter fragment containing cis-elements was amplified from the “Fengjie 72-1” orange fruit genomic DNA and cloned into the P-AbAi vector with HindIII and KpnI digestion. The primers were listed in Supplemental Table S1. After digestion with BstBI, the bait constructs were linearized and were used to screen the appropriate aureobasidin A (AbA) concentration to avoid self-activation by the Y1H gold yeast. After 3 to 5 d, positive, negative control, and positive control yeast strains were picked and diluted in 0.9% NaCl (w/v) to an OD600 of 0.2. Five microliters of the suspension was selected on SD/-Leu medium, with or without AbA. The yeasts were grown at 30 °C for more than 3 d.
Transcription activation analysis
The pGBKT7-BD vector carrying CDS of CsMYB77 via EcoRI and BamHI digestion and its empty vector were transformed into the yeast strain AH109. After screening positive plaques on SD/-Trp medium, the positive plaques were propagated in YPDA medium, lined in SD/-Trp-Ade and SD/-Trp-Ade-His deletion medium, and cultured at 30 °C for 3 d. The primers were listed in Supplemental Table S1 used in this experiment.
Quantitation of ABA, IAA, chlorophyll, carotenoids, and ethylene content
ABA and IAA were extracted from the fruits of Hongkong kumquat lines, including the WT and transgenic lines performed by Wuhan Triploid Biotechnology Co., Ltd. (Wuhan, China). Measurement of ethylene content was described previously (Yue et al. 2020). The chlorophyll, carotenoids content were measured as described in previous literature (Zhang et al. 2021). Each sample was characterized based on 3 replicates.
DAP-seq analysis
DAP-seq constructed the expression of TF protein in vitro to combine with the target genomic fragments, which can enrich the target protein-binding genomic DNA fragments. By high-throughput sequencing technology, the DNA products after DAP were sequenced and analyzed to identify the DNA-binding sites of the target protein from the whole genome. The cDNA libraries were sequenced on the Illumina sequencing platform by Genedenovo Biotechnology Co., Ltd. (Guangzhou, China). The raw data can be obtained from the accession number PRJNA928225 in NCBI.
Agrobacterium-mediated infection
The CDS of CsSINAT4 and CsPIN5 was cloned into pRI101-GFP. The accurate method of injection in tomato (Lin et al. 2022) and citrus was described previously (Yue et al. 2022). In tomato, we counted the flowering period of WT and got enough flowers after 2 wk. Each Agrobacterium strain (GV3101) was infiltrated into the fruit and carpopodium about 27 DAF, once every 3 d in different fruits. Pictures were taken, and the BR stage of the fruits was determined. In citrus, we chose 3 trees and got enough flowers, and fruits were infiltrated around 130 (for CsSINAT4) or 60 (for CsPIN5) DAF. After continuous injection, enough fruits were obtained, and the subsequent BR stage of the fruits or the fruit size were determined and photographed. The GFP fruits were used as the control in tomato and citrus. The infiltrated area was collected for performing experiments.
Statistical analysis
Origin, Excel, and SPSS software were used for the statistical analysis of the data. Comparisons between pairs of groups were performed using Student's t-test. Statistically significant differences were categorized into 2 groups: P < 0.05 and P < 0.01.
Accession numbers
Sequence data in this article can be obtained from the database Orange Genome annotation project (http://citrus.hzau.edu.cn/) with the following accession numbers: CsMYB77 (Cs3g23950), CsSINAT4 (orange1.1t02726), CsFREE1 (Cs3g26450), CsVPS23A (orange1.1t04276.1), CsPYL4 (Cs7g30500), CsPYR1 (orange1.1t01026), CsABF2 (Cs3g23480), CsABI5 (Cs6g14970), CsPIN5 (Cs6g02450), FhSINAT4 (sjg033790), FhFREE1 (sjg035190), FhVPS23A (sjg272410), FhABF2 (sjg173220), FhABI5 (sjg258630), FhPYL4 (sjg238400), FhPYR1 (sjg067470), FhPIN5 (sjg159880), and FhGH3.6 (sjg291640).
Acknowledgments
The authors thank Huiling Xiao for her help in managing the greenhouse.
Author contributions
J.W. and H.-L.Y. conceived the project. J.W. and L.Z. designed the experiment. L.Z., Y.X., Y.L., Z.Z., M.C., and H.-J.Y. collected the samples and conducted the experiments. L.Z., Y.X., and Y.L. performed the data analyses. L.Z. and J.W. wrote the manuscript. All authors read and approved the final manuscript.
Supplemental data
The following materials are available in the online version of this article.
Supplemental Figure S1. CsMYB77 amino acid sequence alignment in Arabidopsis.
Supplemental Figure S2. The positive identification of the leaves in Hongkong kumquat lines.
Supplemental Figure S3. Distribution of downstream peak genes of CsMYB77 and amino acid sequence analysis of CsSINAT4.
Supplemental Figure S4. Amino acid sequence alignment of FREE1, VPS23A, PYL4, and PYR1 in citrus and Arabidopsis.
Supplemental Figure S5. Relative expression of genes in Hongkong kumquat lines.
Supplemental Figure S6. CsPIN5 amino acid sequence alignment and relative expression level in Hongkong kumquat lines.
Supplemental Figure S7. Relative expression of SlPIN5 in tomato.
Supplemental Text S1. Promoter sequence.
Supplemental Table S1. Primers used in this study.
Supplemental Data Set 1. List of DAP-seq enriched peaks.
Funding
This research was supported by the National Natural Science Foundation of China (NSFC; 32172524), the China Agriculture Research System-Citrus (CARS-26), and the Collection, Utilization and Innovation of Germplasm Resources by Research Institutes and Enterprises of Chongqing (cqnyncw-kqlhtxm).
Data availability
The gene sequences in this article can be obtained from the Citrus Pan-genome to Breeding Database (http://citrus.hzau.edu.cn/) and Sol Genomics Network databases (https://solgenomics.net/).
Dive Curated Terms
The following phenotypic, genotypic, and functional terms are of significance to the work described in this paper:
References
Author notes
The author responsible for distribution of materials integral to the findings presented in this article in accordance with the policy described in the Instructions for Authors (https://dbpia.nl.go.kr/plphys/pages/General-Instructions) is Juxun Wu.
Conflict of interest statement. None declared.