-
PDF
- Split View
-
Views
-
Cite
Cite
Zhiru Bao, Ye Guo, Xiangxu Meng, Chunmei Shi, Bo Ouyang, Xiaolu Qu, Pengwei Wang, Microtubule-associated proteins MAP65-1 and SUN18/IQD26 coordinately regulate tomato fruit shape by affecting cell division, Plant Physiology, Volume 194, Issue 2, February 2024, Pages 629–633, https://doi.org/10.1093/plphys/kiad586
- Share Icon Share
Dear Editor,
Understanding the molecular basis of plant structure and development is fundamental to the genetic improvement of agricultural products. Tomato (Solanum lycopersicum) is an ideal model plant for studying climacteric fruit organogenesis and maturation (Bergougnoux 2014); its shape is an important agronomic trait related to yield, quality, and customer preference. Microtubule-associated proteins (MAPs) are key regulators of fresh fruit quantitative traits (Wu et al. 2011, 2018; Jiang et al. 2015; Wang et al. 2021). The SUN1 gene, which encodes a plant-specific MAP (also known as IQ67-domain 12, IQD12), is a conserved trait locus for tomato fruit elongation (Xiao et al. 2008). MAPs are a set of structural proteins that interact with microtubules and control microtubule assembly and organization (Olmsted 1986; Lloyd and Hussey 2001). Misexpression of Arabidopsis (Arabidopsis thaliana) MAPs, e.g. SPIRAL2 (SPR2), KINESIN LIGHT CHAIN-RELATED (KLCR) proteins, and MAP65s, causes multiple defects in cell expansion or division, resulting in abnormal organ shape (Shoji et al. 2004; Lucas and Shaw 2012; Zang et al. 2021). Functionally distinct MAPs encoded in the tomato genome mediate fruit shape formation in different ways (Yu et al. 2022; Zhang et al. 2023). Recently, we revealed the relationship between microtubule dynamics and tomato fruit shape (Bao et al. 2023); however, the mechanism by which different MAPs coordinate cell morphogenesis and division during fruit shape formation remains elusive.
Here, we generated a series of tomato mutants with impaired microtubule function through RNA interference of MICROTUBULE-ASSOCIATED PROTEIN 70-5 (MAP70-5), MICROTUBULE-DESTABILIZING PROTEIN 25 (MDP25), KLCR1, WAVE-DAMPENED 2 (WVD2), SPR2, and MAP65-1 genes. The fruits of MAP65-1 RNAi lines were substantially elongated (Supplemental Fig. S1). MAP65 proteins are conserved in most eukaryotic systems, including plants (Supplemental Fig. S2; She et al. 2019; Smertenko et al. 2000). They are phosphorylated during cytokinesis to regulate microtubule bundling and phragmoplast formation (Smertenko et al. 2006; Boruc et al. 2017). MAP65-1 was highly expressed in tomato ovaries and fruits at early developmental stages (Supplemental Fig. S3). To confirm the function of MAP65-1 in determining fruit shape, we generated map65-1 knockout lines using CRISPR–Cas9 in tomato (MicroTom; Supplemental Fig. S4); all exhibited slender ovaries and elongated fruits, with a larger fruit shape index (aspect ratio) compared with the wild type (Fig. 1, A and B), similar to the MAP65-1 RNAi mutant (Supplemental Fig. S1).
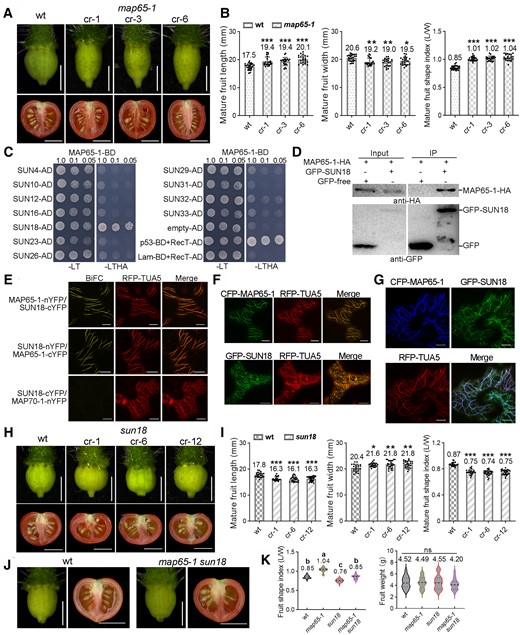
MAP65-1 interacts with SUN18 to regulate tomato fruit shape formation. A) Ovaries at the anthesis stage and mature fruits of the map65-1 mutants and wild type. B) Quantification of fruit length, width, and shape index of mature fruits in A). C) Yeast 2-hybrid assay shows that only SUN18 protein interacts with the MAP65-1 protein. The combination of p53-BD + RecT-AD is the positive control, and Lam-BD + RecT-AD is the negative control. D) Coimmunoprecipitation assay using a GFP antibody (GFP-Trap) confirmed the interaction between MAP65-1 and SUN18 (IP, immunoprecipitated fraction). MAP65-1-HA was detected using a HA antibody (top), whereas the bands of GFP-SUN18 or free GFP (negative control) were shown in the bottom panels. E) BiFC assay in N. benthamiana leaves shows that MAP65-1 and SUN18 interact at cortical microtubules (labeled by RFP-TUA5). Here, SUN18-cYFP + MAP70-1-nYFP was used as the negative control. Bars: 10 μm. F, G) Subcellular colocalization of MAP65-1 and SUN18 proteins in leaf pavement cells of N. benthamiana. RFP-TUA5 was used as the microtubule marker. Bars: 10 μm. H) Ovaries (anthesis stage) and mature fruits traits in the sun18 mutants and wt. I) The quantifications of fruit length, width, and shape index of mature fruits in H). J) Phenotypes of ovaries (anthesis stage) and mature fruits of the map65-1 sun18 double-mutant lines. K) The quantifications of shape index and weight of mature fruits in J). The bars for mature fruit are 1 cm, and for ovaries, they are 1 mm. The error bars represent mean ± Sd. N > 20 for fruit characteristic analysis. Asterisks in B, I) indicate significant differences by Student's t test, *P < 0.05, **P < 0.01, ***P < 0.001. Different letters in K) denote significant differences (P < 0.05) from Duncan's multiple tests. ns, no significant difference. The middle dotted line in the violin box K) refers to medians; the thin dotted lines refer to the median lower quartile and upper quartile; the width of the violin box represents the local distribution of feature values along the y axis.
To better understand the mechanism of MAP65-1 influence on fruit morphogenesis, we performed a yeast (Saccharomyces cerevisiae) 2-hybrid screen using a library containing a collection of SUN/IQD genes. We identified one potential MAP65-1 interactor, SUN18, which was also highly expressed in anthesis-stage ovaries and young fruits (Supplemental Fig. S5). Interaction with MAP65-1 is likely specific to individual SUN homologs (Fig. 1C); coimmunoprecipitation and bimolecular fluorescence complementation (BiFC) assays confirmed the in vivo interaction of MAP65-1 with SUN18 (Fig. 1, D and E). In Nicotiana benthamiana, tomato CFP-MAP65-1 and GFP-SUN18 colocalized with RFP-TUA5 at cortical microtubules in nondividing cells (Fig. 1, F and G). In cells undergoing cytokinesis (induced by the expression of CYCD3;1 in N. benthamiana, Xu et al. 2020), they also colocalized at various microtubule-related structures, such as the prophase band, spindle, and phragmoplast (Supplemental Fig. S6), suggesting putative functions during cell division (discussed later).
Next, we generated sun18 knockout plants using CRISPR–Cas9 (Supplemental Fig. S7). In contrast to the phenotype of map65-1 mutants, sun18 mutants exhibited significantly fatter fruits with a lower fruit shape index (Fig. 1, H and I). More interestingly, the single-mutant phenotype was restored in the map65-1 sun18 double mutant, with fruit shapes similar to the wild type (Fig. 1, J and K). This indicates that the 2 genes may have an additive effect on fruit development, possibly playing different roles in microtubule-related activities.
In-depth characterization of mutant plants by analyzing anthesis-stage ovaries showed similar shape changes as in mature fruits (Figs. 1A, H, J and 2A, B). In ovary tissue, map65-1 mutant plants displayed significantly lower cell number along the medium-lateral axis, while sun18 mutant plants showed greater cell number along the medium-lateral axis and lower cell number along the proximal-distal axis compared with wild-type plants; no significant changes were observed in map65-1 sun18 double-mutant plants (Fig. 2B). The cell shape of parenchyma tissue (measured by using the aspect ratio) was similar among genotypes (Fig. 2, C and D). These results suggest that MAP65-1 and SUN18 may function additively in shape regulation by primarily modulating cell proliferation patterns and thus fruit anisotropic growth.
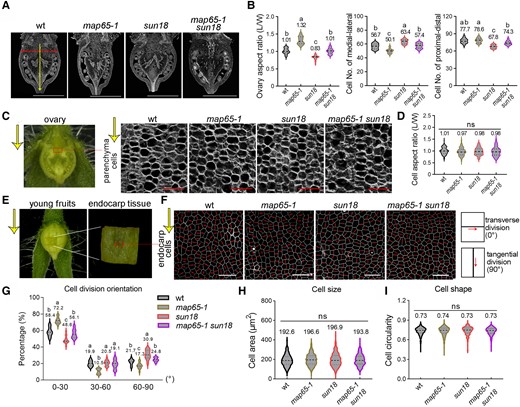
MAP65-1 and SUN18 regulate cell division patterns with little effect on cell shape. A) Paraffin sections of anthesis-stage ovaries along the longitudinal axis. B) Statistical analysis of the ovary aspect ratio, cell numbers along the medial–lateral direction, and proximal-distal of whole ovaries in different genotypes in A). C) Paraffin sections of parenchyma tissue (rectangle) from the anthesis-stage ovary in different genotypes. D) Quantitative analyses of parenchyma cell aspect ratio (as the measurement of cells shape) in C). E) Endocarp tissues were dissected from the middle part of young fruits at the active division stage (60 h after manual pollination). F) Endocarp cells in E) of different genotypes were stained by Propidium Iodine and the orientations of cell division were analyzed by using Image J. The red lines represent newly formed division planes of endocarp cells. The yellow arrows in C, E, F) indicate the fruit-growth orientation along the longitudinal axis. G to I) Quantitative analyses of endocarp cell division orientation (0° to 30°, 30° to 60°, and 60° to 90°) relative to the fruit-growth direction G), endocarp cell size H), and circularity I) in F). The bars for ovary sections are 1 mm, and parenchyma cells and endocarp cells are 50 μm. n > 1,000 cells from 6 ovaries for cell division orientation ratio analysis. n > 15 for the ovary section. n > 100 for parenchyma cells analysis. n = 600 for endocarp cell circularity analysis. Different letters of violin boxes in B, D, G, H, I) denote significant differences (P < 0.05) from Duncan's multiple tests. ns, no significant difference. The middle dotted line in the violin box refers to medians; the thin dotted lines refer to the median lower quartile and upper quartile; the width of the violin box represents the local distribution of feature values along the y axis.
Suspecting that differences in cell number along different axes may be directly attributed to changes in cell division, we analyzed the directions of the division planes in different genetic backgrounds. Most tissues in tomato fruits are thick and complex, making it technically difficult to image through parenchyma tissue without causing damage; therefore, endocarp tissue, which is easily accessible for light microscopy (Fig. 2E), was used for further study. Active cell division was observed in fruit at 60 h after hand-pollination, and the orientation of the cell division plane was determined using PI staining followed by ImageJ analysis. In agreement with changes in fruit shape, sun18 mutant plants exhibited greater tangential division (angles between 60° and 90° relative to the growth direction) than the wild-type control; in contrast, the map65-1 mutant displayed more transverse division (0° to 30°) in endocarp tissue. Interestingly, the direction of cell division was restored to the wild-type phenotype in the map65-1 sun18 double mutant (Fig. 2, F and G). We suspect that similar variations were present in other fruit tissues. In contrast, cell size and shape (indicated by cell area and circularity) in the endocarp were not statistically different among all genotypes (Fig. 2, F, H, and I). These data confirm that MAP65-1 and SUN18 likely regulate fruit shape by modulating cell division patterns but not changing cell morphology.
We observed no obvious differences in leaf morphology or plant development between wild-type and mutant plants (Supplemental Fig. S8, A and B), indicating that SUN18 and MAP65-1 mainly contribute to fruit shape formation with little effect on the overall development of tomato. Our study suggests that both MAP65-1 and SUN18 contribute to the pattern of cell division in tomato fruits, and genetic manipulation of certain MAPs could be useful in molecular breeding programs for agronomic trait improvement of fruit crops.
Acknowledgments
We thank the College of Horticulture and Forestry Sciences, Huazhong Agricultural University, for providing us the core facilities for performing microscopy. We thank the College of Life Science and Technology, Huazhong Agricultural University, for providing us the core facilities for microscopy. We also thank Katharina Bürstenbinder (Leibniz Institute of Plant Biochemistry) for her advice on this work.
Author contributions
Z.B. and P.W. conceived and designed the project. Z.B. conducted the experiments and data analysis. Y.G., C.S., and X.M. participated in some experiments. Z.B. and Y.G. wrote the manuscript. P.W., X.Q., and B.O. revised the manuscript.
Supplemental data
The following materials are available in the online version of this article.
Supplemental Figure S1. Fruit morphology of different MAP RNAi mutants.
Supplemental Figure S2. Phylogenetic tree of MAP65 proteins in tomato and Arabidopsis.
Supplemental Figure S3. RT-qPCR analysis of MAP65-1 expression in tomato at different developmental stages.
Supplemental Figure S4. Genotype characterization of map65-1 mutants in T2 generation.
Supplemental Figure S5. RT-qPCR analysis of SUN18 expression in tomato at different developmental stages.
Supplemental Figure S6. Subcellular colocalization of MAP65-1 and SUN18 proteins during cell division in N. benthamiana.
Supplemental Figure S7. Characterization of sun18 mutants.
Supplemental Figure S8. Tomato seedlings of different genotypes at vegetative and reproductive growth stages.
Supplemental Table S1. Primers used for generating constructs.
Supplemental Table S2. Primers used for RT-qPCR.
Funding
The project was supported by National Natural Science Foundation of China (NSFC) grants (nos. 32261160371 and 92254307), the Fundamental Research Funds for the Central Universities (2662023PY011 and Horti-PY-2023-001), the Foundation of Hubei Hongshan Laboratory (2021hszd016) to P.W., and the Natural Science Foundation of Hubei Province (2021CFB142) to Z.B.
Data availability
Data generated in this study are available upon reasonable request.
Dive Curated Terms
The following phenotypic, genotypic, and functional terms are of significance to the work described in this paper:
References
Author notes
The author responsible for distribution of materials integral to the findings presented in this article in accordance with the policy described in the Instructions for Authors (https://dbpia.nl.go.kr/plphys/pages/General-Instructions) is Pengwei Wang ([email protected]).
Conflict of interest statement. None declared.