-
PDF
- Split View
-
Views
-
Cite
Cite
Guangzhong Zhang, Chenjiaozi Wang, Xinghai Ren, Zaiyuan Li, Conghui Liu, Xi Qiao, Shicai Shen, Fudou Zhang, Fanghao Wan, Bo Liu, Wanqiang Qian, Inhibition of invasive plant Mikania micrantha rapid growth by host-specific rust (Puccinia spegazzinii), Plant Physiology, Volume 192, Issue 2, June 2023, Pages 1204–1220, https://doi.org/10.1093/plphys/kiad186
- Share Icon Share
Abstract
Mikania micrantha Kunth is a fast-growing global invasive weed species that causes severe damage to natural ecosystems and very large economic losses of forest and crop production. Although Puccinia spegazzinii can effectively inhibit the growth of M. micrantha and is used as a biological control strain in many countries, the mechanism of inhibiting the growth of M. micrantha is not clear. Here, we used a combination of phenotypic, enzyme activity, transcriptomic, and metabolomic approaches to study the response of M. micrantha after infection by P. spegazzinii. In the early stages of rust infection, jasmonic acid (JA), jasmonoyl-isoleucine (JA-Ile), and salicylic acid (SA) levels in infected leaves were significantly lower than those in uninfected leaves. In teliospore initial and developed stages of P. spegazzinii, JA and JA-Ile levels substantially increased by more than 6 times, which resulted in a significant decrease in the accumulation of defense hormone SA in infected leaves of M. micrantha. The contents of plant growth-promoting hormones were significantly reduced in the infected plants as a result of substantial downregulation of the expression of key genes related to hormone biosynthesis. Furthermore, rust infection led to high levels of reactive oxygen species in chloroplasts and the destruction of chlorophyll structure, which also led to decreased photosynthetic gene expression, net photosynthetic rate, activity of Rubisco, and levels of important organic acids in the Calvin cycle. We hypothesized that after P. spegazzinii infection, JA or JA-Ile accumulation not only inhibited SA levels to promote rust infection and development, but also impeded the rapid growth of M. micrantha by affecting plant growth hormones, carbon, and nitrogen metabolic pathways.
Introduction
Mikania micrantha is a fast-growing vine that is one of the top 100 most severe invasive species in the world (Lowe et al. 2000). It is widely distributed in tropical and subtropical regions of Asia and Oceania, causing serious damage to natural ecosystems (Day et al. 2016) and severe economic losses associated with forest and crop production (Macanawai et al. 2012) as a result of its extremely fast growth rate, production of a large number of wind-borne seeds, and its ability to reproduce via stem segments (Day et al. 2016). Recent studies have shown that the rapid growth of M. micrantha is related to its higher photosynthetic rate and increased nitrogen availability in the soil (Liu et al. 2020).
Puccinia spegazzinii (Uredinales: Pucciniaceae) is a rust fungus used for the biological control of M. micrantha and has a short life cycle and strong specificity (Ellison et al. 2008; Day and Riding 2019). P. spegazzinii can infect the leaves, petioles, and stems of M. micrantha and has strong environmental tolerance (Barreto and Evans 1995), causing the host plant tissue to develop lesions, become stunted, wither, or die. A previous study showed that the growth rate and dry weight (DW) of M. micrantha after infection by P. spegazzinii were generally decreased, and the number of nodes also decreased (Day et al. 2013). P. spegazzinii potential as a biological control agent is because it is particularly damaging to the leaves, stems, and petioles of M. micrantha, reducing growth rates and flowering (Barreto and Evans 1995; Day et al. 2013; Day and Riding 2019). Therefore, fungi for the biological control of M. micrantha have been introduced into 9 countries for M. micrantha management (Day and Riding 2019). Although much research has been conducted on P. spegazzinii, the mechanism through which it inhibits M. micrantha growth remains unclear.
Rust-producing fungi (Pucciniales) compose the largest group of plant pathogens and constitute one of the most devastating threats to agricultural crops worldwide (Lorrain et al. 2019). Rust can affect the growth of plants, leading to yield losses, of which disruption in photosynthesis is considered to be among the main reasons (Carretero et al. 2011; He et al. 2019; Yang and Luo 2021). Studies have shown that the net photosynthetic rate of plants obviously decreases in response to rust stress, which may be caused by decreases in stomatal conductance, green leaf area, and chlorophyll level (Moerschbachepr et al. 1993; Kumudini et al. 2008; Carretero et al. 2011; Chen et al. 2015, 2020; Eberl et al. 2018), and there is also much evidence that the factors of photosynthesis itself also play important roles. In a compatible system, rust infection easily damaged PSII in leaves to reduce photosynthesis; this occurred by phosphorylating PsbO, an external member of PSII (Wang et al. 2019), declining the PSII reactive center protein (D1 protein) (Chen et al. 2015, 2020), and reducing the photochemical efficiency and electron transfer efficiency of PSII (Kumudini et al. 2008; Navarro et al. 2019; Chen et al. 2020). In compatible interactions between Melampsora medusae and poplar (Populus spp.), the expression levels of genes related to photosynthesis, including chlorophyll a/b binding proteins and photosynthetic reaction center proteins, were significantly downregulated after rust infection (Miranda et al. 2007; Rinaldi et al. 2007). However, several physiological traits and the expression of genes related to the influence of rust on photosynthesis have not been completely evaluated at the leaf level, and few studies have investigated the influence of rust on the photosynthesis of plant leaves, especially carbon fixation.
Numerous studies have shown that salicylic acid (SA) and jasmonic acid (JA) play central roles in plant defense responses, as these hormones mediate various aspects of biotic and abiotic stress responses. Furthermore, pathogen infections can also cause changes in host growth-related hormone levels, which may lead to enhanced host defenses in the form of hormone crosstalk or may be a virulence strategy of microorganisms to interfere with plant development, leading to plant susceptibility and promoting disease development (Spoel and Dong 2008; Robert-Seilaniantz et al. 2011; Shigenaga and Argueso 2016). In the interaction of necrotic fungi with their hosts, increased levels of auxins and cytokinins or their enhanced signaling can lead to increased plant resistance (Llorente et al. 2008; Qi et al. 2012; Qiao et al. 2020). This may be related to the positive interaction of JA and auxin, as well as cytokinin and SA, in regulating plant resistance to necrotizing pathogens (Choi et al. 2010; Qi et al. 2012; Jiang et al. 2013; Kunkel and Johnson 2021). Conversely, many plant pathogens can directly synthesize auxin and cytokinins or invoke plant auxin biosynthesis as well as modulate auxin signaling to render the host more susceptible to infection (Chen et al. 2007; Lee et al. 2009; Pertry et al. 2010; Yin et al. 2014; Kunkel and Johnson 2021). In addition, the infection of pathogenic bacteria can also lead to a decrease in growth-related hormone contents or the obstruction of hormone signal transduction, which is related to the balance between growth and defense responses (Jiang et al. 2017). In response to a pathogen attack, plants allocate more resources to defensive responses and decrease the supply of nutrients and energy to less urgent physiological processes such as photosynthesis and plant growth (Huot et al. 2014; Takatsuji 2017). Many studies have shown that SA and JA also have antagonistic effects on growth-related hormones (Junichi and Jiro 1982; Wang et al. 2007; Yang et al. 2012; Zhang et al. 2020).
In this study, a combination of transcriptomic, metabolomic, and phenotypic approaches was used to study the changes in M. micrantha infected with P. spegazzinii, such as the synthesis and signal transduction of growth- and defense-related hormones, photosynthesis, and nitrogen transport and assimilation. This study provides valuable information for the biological control of M. micrantha and information about the molecular mechanism by which rust influences the growth of its host.
Results
Growth inhibition of M. micrantha in response to P. spegazzinii infection
It has been observed that P. spegazzinii could complete its life cycle within 19 to 22 d. The life cycle involved 3 main stages: (i) the early stage of infection (24, 48, and 96 h post-infection, marked as EL24, EL48, and EL96); (ii) then, light green telia is convex on the underside of the symptomatic leaves, which was defined as the teliospore initials (TIs); and (iii) thereafter, teliospore developed (TD) and matured with the telia turned dark brown, called TD stage (Fig. 1A). After teliospores mature, basidiospores sprouted from telia embedded in host tissues for the next infection cycle.
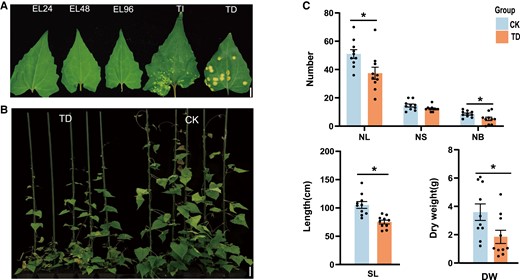
Phenotypic differences of M. micrantha in response to P. spegazzinii. A) Different developmental stages of P. spegazzinii in M. micrantha leaf, including early stage of infection (EL, 24, 48, and 96 h post-infection), TIs (7 to 8 d post-infection), and TD stage (15 to 17 d post-infection). Bar = 7 mm. B) Phenotypes of M. micrantha healthy plants (CK) and infected plants at TD stage (n = 5). The phenotype of CK was imaged under normal growth conditions, and the phenotype of TD stage was imaged at 17 d of P. spegazzinii infection. Bar = 6 cm. C) Number of leaves (NL), NS, number of branches (NB), SL, and DW of M. micrantha at CK and TD stages. Values represent the mean ± Se derived from 10 biological replicates. Statistical significance between CK and TD is indicated according to Student's t-test: *P < 0.05 .
To investigate the effect of rust infection on the growth of M. micrantha, we measured growth-related phenotypes between infected and uninfected plants by P. spegazzinii, and the growth of M. micrantha was substantially inhibited after 17 d of inoculation (Fig. 1B). The numbers of leaves and branches in M. micrantha infected with rust were significantly reduced by more than 26.7% and 39.5% (Fig. 1C; Supplemental Table S1), respectively, compared with those of uninfected plants. In addition, we also measured the total length of all the stem nodes of M. micrantha, and this length after infection was 75.1 cm on average, which was significantly reduced compared with that of the uninfected plants (Fig. 1C; Supplemental Table S1). The DW of M. micrantha after rust infection (DW = 1.3 ± 0.87 g) was less than half that of uninfected plants (DW = 3.6 ± 0.55 g; Fig. 1C; Supplemental Table S1). Although there was no significant difference in the number of nodes between the infected and uninfected plants, the number of nodes of M. micrantha infected by P. spegazzinii also decreased by 15.8% (Fig. 1C; Supplemental Table S1).
Dynamic patterns of JA and SA biosynthesis and degrading in M. micrantha during the different stages of P. spegazzinii infection
In this study, the main defense compounds—the hormone conjugate JA, jasmonoyl-isoleucine (JA-Ile), and the hormone SA—presented distinct patterns during the different stages of rust infection, including early stages of infection (EL24, EL48, and EL96), and TI and TD stages. These patterns revealed that the amounts of JA and JA-Ile in the 3 early stages (EL24, EL48, and EL96) of infected leaves were significantly lower than those in the uninfected leaves (Fig. 2A; Supplemental Table S2). The decrease in JA-Ile content might be caused by the significant upregulation of the expression level of JA-Ile degrading gene CYP94C1 in the early stages of infection (EL24 and EL48; Fig. 2B). We also found that the gene expression pattern of JA biosynthesis was consistent with the variation of JA accumulation. The expression level of JA synthetic key genes (LOX2, AOS, OPR3, and MFP2) in the infected leaves was substantially downregulated during the early stages of rust infection (Fig. 2B), leading to a significant reduction in JA accumulation.
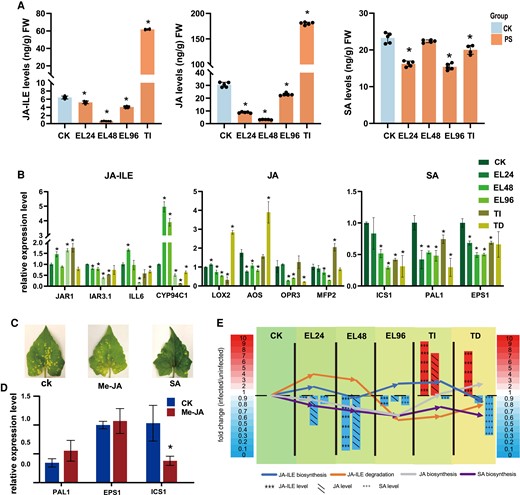
Effects of P. spegazzinii on JA, JA-Ile, and SA in M. micrantha. A) Levels of JA-Ile, JA, and SA in leaves of M. micrantha at CK, EL, and TI stages. CK indicates the uninfected plants by rust; PS indicates rust-infected leaves; EL and TI stages indicate the early stage of infection and TIs (see legend of Fig. 1). Values represent the mean ± Sd derived from 5 biological replicates. B) Relative mRNA levels of key genes involved in JA-Ile, JA, and SA biosynthesis and JA-ILE degradation in all stages of rust infection. Values represent the mean ± Sd derived from 3 biological replicates. JA-Ile synthesis gene included JAR1; JA-Ile degradation genes included IAR3.1, ILL6, and CYP94C1; JA synthesis genes included LOX2, AOS, OPR3, and MFP2; SA synthesis genes included ICS1, PAL1, and EPS1. C) Inhibitory effect of exogenous Me-JA and SA on the development of P. spegazzinii. The infected leaves were sprayed with exogenous Me-JA and SA with a concentration of 1 mol L−1 once a day, respectively. After 10 d of treatment, the leaf was collected to observe the infection status of P. spegazzinii. D) Effect of exogenous Me-JA on mRNA level of SA biosynthesis genes in M. micrantha leaves. Values represent the mean ± Sd derived from 3 biological replicates. E) Expression pattern of key genes in JA, JA-Ile, and SA biosynthesis and JA-Ile degradation pathways, and content variation of JA, JA-Ile, and JA during all stages of rust infection. The key genes of JA-Ile degradation included IAR3.1, ILL6, and CYP94C1; the key genes of JA biosynthesis included LOX2, AOS, OPR3, and MFP2; the key gene of JA-Ile biosynthesis was JAR1; the key genes of SA biosynthesis included ICS1, PAL1, and EPS1. The bars and arrow lines indicate the fold-change of metabolites content (JA, JA-Ile, and SA) and genes expression, respectively. In (A), (B), and (D), statistical significance is determined by Student's t-test: *P < 0.05.
However, the level of JA and JA-Ile represented substantial accumulation during the TI and TD stages. JA-Ile levels were 9.6 and 6.4 times higher than in uninfected leaves in TI and TD stages, respectively, while JA was 6.4 times higher than in uninfected leaves in the TD stage (Fig. 2A; Supplemental Fig. S1), respectively. It is interesting to note that the accumulation of JA-Ile in infected leaves was not only due to the upregulated expression of JA-Ile biosynthesis gene JAR1, but also due to the substantially downregulated expression of genes encoding key JA-degrading enzymes (such as CYP94C1, IAR3, and ILL6; Fig. 2B).
In contrast, the synthesis of SA was significantly inhibited during the whole stages of P. spegazzinii infection. We found that the transcription of ICS1, PAL, and EPS1, which is involved in SA biosynthesis, was significantly inhibited during the whole stages of rust infection (Fig. 2B), which ultimately led to a decrease in the SA level in the infected leaves (Fig. 2A; Supplemental Fig. S1).
To determine the role of SA and JA in the resistance to P. spegazzinii, we then treated M. micrantha leaves with exogenous SA and methyl jasmonate (Me-JA) before and after inoculation. When infected with the P. spegazzinii after 10 d, fewer pustules were produced on exogenous SA treatment plants than on the control plants, while the exogenous Me-JA treatment strain and the control strain have similarities in pustule production (Fig. 2C; Supplemental Fig. S2). In addition, the expression level of key gene ICS1 involved in SA synthesis was reduced 2- to 3-fold in Me-JA treated leaves (Fig. 2D), which suggested that the increase of JA level suppressed the expression of SA synthesizing gene ICS1 to reduce the accumulation of SA in M. micrantha leaves.
To investigate the dynamic patterns of the main defense compounds, the variation of JA-Ile, JA, and SA contents, and the biosynthesis and degradation of key genes were selected to analyze the dynamic regulated relationship during the whole infection stages by P. spegazzinii. At the early stages of infection and period before teliospores formation, the decrease of SA and JA levels was due to the significant downregulation of synthetic pathway genes expression, while the upregulated expression of JA-Ile degradation pathway genes resulted in the decrease of JA-Ile accumulation (Fig. 2E). During the TI and TD stages, the amounts of JA and JA-Ile were continuously increased to suppress the key gene expression of SA synthetic, thus reducing the SA level by 6- to 7-fold in the infected leaves (Fig. 2E). Interestingly, the SA biosynthesis genes showed low expression level during the whole development stage of P. spegazzinii, resulting in the reduction of SA accumulation, which might reduce the resistance of M. micrantha to biotrophic fungus.
Infection with P. spegazzinii reduces the accumulation of growth-promoting plant hormones in M. micrantha
According to the measurements of the growth-promoting levels of hormones in leaves of M. micrantha during the whole stages of rust infection, the levels of growth-promoting hormones in the infected leaves were significantly reduced compared with those in the uninfected leaves during most stages (Fig. 3A; Supplemental Fig. S3 and Table S2). The levels of auxin (IAA) and cytokinins, which play important roles in plant growth and cell division, were significantly reduced due to rust infection. During the whole stages of rust infection, the amount of IAA was significantly decreased in the infected leaves, except for 24 h post-infection (Fig. 3A; Supplemental Fig. S3). However, the accumulation of cytokinin in infected leaves was significantly inhibited in all stages, and its content was reduced by more than 30% than that in healthy leaves (Fig. 3A; Supplemental Fig. S3). According to the analysis of transcriptome at the TD stage of infected leaves, we found that 32 genes related to plant hormone biosynthesis and signal transduction were significantly downregulated in infected leaves (Fig. 3B; Supplemental Table S3). In auxin biosynthesis pathway (Fig. 3C), the expression level of gene encoding TRYPTOPHAN AMINOTRANSFERASE OF ARABIDOPSIS 1 (TAA1) was significantly downregulated more than 3 times than that in healthy leaves (Fig. 3B). Furthermore, 3 YUC FLAVIN MONOOXYGENASES genes represented the same pattern to the TAA1, showing that the gene expression level was reduced by more than 4- to 7-fold after the rust infection (Fig. 3B). In addition, 1 AUXIN RESISTANT 1 (AUX1), 1 TRANSPORT INHIBITOR RESPONSE 1 (TIR1), 10 AUXIN-RESPONSIVE PROTEIN IAA (Aux/IAA), 2 GRETHCHEN HAGEN 3 (GH3), and 5 SMALL AUXIN UPREGULATED RNA (SAUR) genes, which were involved in regulating cell enlargement, were significantly downregulated after rust infection (Fig. 3B). The expression levels of these genes were reduced by 2.88 times, on average, in the infected leaves compared with the uninfected leaves, the level of which ranged from 1.10 to 6.07 times (Fig. 3B; Supplemental Table S3). These results indicated that the expression of key genes involved in IAA synthesis was significantly reduced in response to P. spegazzinii infection, resulting in reduced auxin levels in M. micrantha leaves to inhibit its rapid growth (Fig. 3C).
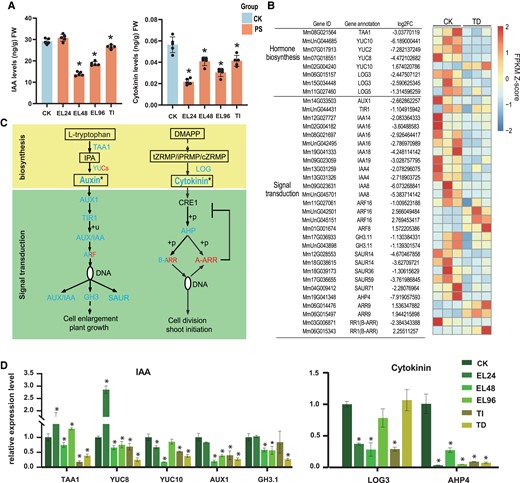
Effects of P. spegazzinii infection on growth-promoting hormones (IAA and Cytokinins) in M. micrantha leaves. A) Level of growth-promoting hormones in leaves of M. micrantha in healthy leaves (CK), early stage of rust infection (EL24, EL48, and EL96), and TI stage. PS indicates rust-infected leaves, including the rust infection stages of EL24, EL48, EL96, and TI. Values represent the mean ± Sd derived from 5 biological replicates. B) Expression patterns of DEGs involved in the biosynthesis and signal transduction of growth-promoting hormone at TD stage. The gene names and gene annotation results of M. micrantha are shown on the left. C) Comparison of growth-promoting hormone biosynthesis and signal transduction gene expression and growth-promoting hormone content at CK and TD stages. Annotated and quantified metabolites and growth-promoting hormones are framed, and the content of Auxin and cytokinin were significant significantly decreased. The expressions of 1 YUC gene, 3 ARF genes, 1 B-ARR gene and 2 ARR genes involved in Auxin and Cytokinin synthesis and signal transduction were significantly up-regulated at TD stage, while other genes were significantly down-regulated. IPA, indole-3-pyruvic acid; tZRMP/iPRMP/cZRMP, tZ/iP/cZ riboside 5′-monophosphate; DMAPP, dimethylallyl diphosphate. D) Relative mRNA levels of key genes involved in IAA and Cytokinins biosynthesis and signal transduction at all stages of rust infection. Values represent the mean ± Sd derived from 3 biological replicates. In (A) and (D), statistical significance between CK and each stage (EL24, EL48, EL96, and TI) was determined by Student's t-test: *P < 0.05.
On the other hand, the 4 key genes involved in cytokinin biosynthesis and signal transduction pathways in M. micrantha (the genes of which encode 3 copies of LONELY GUY [LOG] and 1 copy of HISTIDINE PHOSPHOTRANSFER PROTEINS [AHPs]) exhibited significant differences in expression, as these genes were significantly downregulated after rust infection (Fig. 3, B and C). The type-B ARABIDOPSIS RESPONSE REGULATORs (ARRs) are DNA-binding transcriptional activators that are required for cytokinin responses. The pattern of the expression of genes encoding type-B ARRs in M. micrantha was similar to the pattern of cytokinin levels, showing that gene expression decreased with rust infection (Fig. 3, B and C). On the other hand, the type-A ARRs act as repressors of cytokinin-activated transcription. In contrast, after rust infection, the expression of type-A ARRs genes was upregulated (Fig. 3, B and C), thus decreasing the regulation of cell division.
To further explore the influence of the whole stages of rust infection on growth hormone synthesis genes in M. micrantha, the relative expression levels of TAA1, YUC8, YUC10, AUX1, GH3.1, LOG3, and AHP4 in infected leaves were assayed by RT-qPCR with the polyubiquitin gene as a reference gene for normalization (Fig. 3D). The TAA1, YUC8, and YUC10 transcripts involved in IAA synthesis were substantially inhibited during the whole stages. In contrast, the expression level of these genes showed significant upregulation at 24 h post-infection (Fig. 3D). And the genes LOG3 and AHP4 related to cytokinins synthesis and signal transduction pathways were lowly expressed during the whole stages of rust infection. In summary, those genes expression showed the same variation pattern with the accumulation of growth hormone at different infection stages.
To prove that auxin and cytokinin could block the growth inhibition effect of M. micrantha by rust infection, we sprayed the exogenous auxin and cytokinin on the infected leaves. After 10 d, we found that the exogenous auxin and cytokinin could improve the growth of infected plants (Supplemental Fig. S4A). The growth-related phenotypes, including plant height, stem length (SL), number of stem nodes (NS), and DW, were significantly increased in infected plants (Supplemental Fig. S4B), indicating that both exogenous auxin and cytokinin could substantially block the growth inhibition of M. micrantha by P. spegazzinii.
Effects of P. spegazzinii infection on the photosynthesis of M. micrantha
To consider the P. spegazzinii infection influence on the photosynthesis of M. micrantha, we measured the accumulation of reactive oxygen species (ROS) and observed the chloroplast structure of infected leaves during the whole stages of infection. The result showed that the ROS levels of infected leaves in EL96 and TI stages were obviously higher than those in other stages based on DAB staining (Fig. 4A), indicating that the infection of P. spegazzini led to excessive accumulation of ROS in infected leaves. To further clarify where the ROS production derived from, the DCFH-DA fluorescent probe was used after rust infection. The results were consistent with those obtained by DAB staining (Fig. 4B), and strong fluorescence was observed mainly in chloroplasts, indicating that after 96 h post-infection ROS was mainly concentrated in chloroplasts of leaves (Fig. 4B). Interestingly, the levels of ROS and JA had similar variation pattern during the whole stages of rust infection, showing that the ROS level increases with the accumulation of JA (Figs. 2A and 4A). In addition, we also found that the exogenous Me-JA treatment could promote ROS accumulation in the chloroplasts in M. micrantha leaves based on DAB staining and DCFH-DA fluorescent probe (Supplemental Fig. S5). Furthermore, the chloroplast structure had been obviously destroyed after 96 h post-infection (Fig. 4C). These results might suggest that the accumulation of JA and JA-Ile may lead to the ROS burst in chloroplasts after rust infection, thus disrupting chloroplast structure.
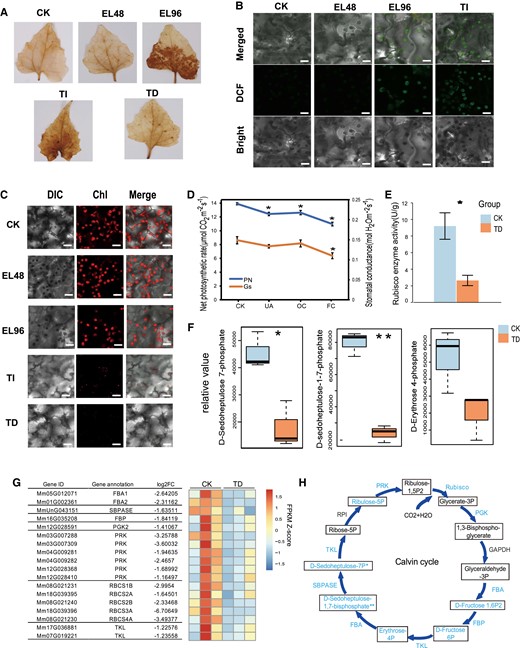
Effects of P. spegazzinii on the photosynthesis of M. micrantha. A) Diaminobenzidine (DAB) staining of ROS in leaves of M. micrantha at all stages of rust infection. For the definition of each stage, see the legend of Fig. 1. B) 2′,7′-dichloro-dihydro-fluorescein diacetate (DCFH-DA) fluorescent probe of ROS in leaves of M. micrantha in healthy leaves (CK) and infected plants (EL and TI stages of rust infection). Images were taken using confocal microscopy. Bars = 10 µm. C) The chloroplast density of M. micrantha leaves at all stages of rust infection were observed under confocal microscope. Bars = 10 µm. D) The changes in net photosynthetic rates and stomatal conductance in the leaves of M. micrantha under CK and TD stage (UA, OC, and FC). UA, the second group comprised asymptomatic areas of infected leaves; OC, infected areas with 1 pustule; FC, infected leaves with 4 pustules. Values represent the mean ± Sd derived from 5 biological replicates. E) Activity of Rubisco in the leaves of M. micrantha under CK and TD stage. Values represent the mean ± Sd derived from 5 biological replicates. F) Relative content of identified metabolites involved in the Calvin cycle in the leaves of M. micrantha under CK and TD stage is shown in the boxplot. Within each boxplot, the central line indicates the median, the bottom and top edges of the box indicate the interquartile ranges (IQRs), and the whiskers represent the maximum and minimum data points. Values were derived from 3 biological replicates. G) Expression patterns of DEGs related to the Calvin cycle at TD stage. The gene names and gene annotation results of M. micrantha are shown on the left. H) Comparison of genes and the content of metabolites involved in the Calvin cycle in leaves of M. micrantha under CK and TD stage. The metabolites annotated and quantified are framed, and decreasing contents are marked in blue. Genes with significantly downregulated expression are marked in blue. The error bars in (D) and (E) represent the means ± Sd. Statistical significance is indicated in (D), (E), (F), and (H), according to Student's t-test: *P < 0.05.
By comparing the net photosynthetic rates (Pn) between infected and uninfected leaves of M. micrantha, we found that the net photosynthetic rates of the infected leaves without pustules (UA) (12.4 μmol CO2 m−2 s−1), infected leaves with 1 pustule (OC) (12.6 μmol CO2 m−2 s−1), and infected leaves with 4 pustules (FC) (11.0 μmol CO2 m−2 s−1) after infection by P. spegazzinii were significantly reduced compared with those of the leaves of uninfected plants (CK) (13.9 μmol CO2 m−2 s−1) (Fig. 4D; Supplemental Fig. S6A and Table S4). In contrast, the stomatal conductance (Gs) of the infected leaves was not obviously different from that of the leaves of the uninfected plants. However, with the increase in pustules, the stomatal conductance of the leaves decreased. In fact, the stomatal conductance of the leaves with 4 pustules (0.11 mol H2O m−2 s−1) was significantly lower than that of normal leaves (0.15 mol H2O m−2 s−1; P = 0.011; Fig. 4D; Supplemental Table S4). In terms of the intercellular CO2 concentration and transpiration rate of leaves, we found no difference between the infected and uninfected plants except for the transpiration rate of leaves with 4 pustules (Supplemental Fig. S6B).
The fixation of carbon dioxide depends on the activity of ribulose-1,5-bisphosphate carboxylase/oxygenase (Rubisco); the activity of M. micrantha Rubisco in infected leaf tissue was nearly 3.5 times less than that in healthy plant leaves (Fig. 4E; Supplemental Table S4). In addition, the levels of important compounds of the Calvin cycle decreased significantly after rust infection (Fig. 4F). Among these compounds, the levels of D-sedoheptulose-7-phosphate and D-sedoheptulose-1–7-phosphate in infected leaves were more than 3.4 and 2.5 times less than those in healthy leaves, respectively (Fig. 4F; Supplemental Table S5). The levels of D-ribulose-5-phosphate, D-erythrose-4-phosphate, D-fructose-6-phosphate, and D-fructose-1,6-bisphosphate were reduced by 1.77 times in response to P. spegazzinii infection (Fig. 4F; Supplemental Table S5).
On the basis of contrasting gene expression in the leaf tissues between infected and uninfected plants, 18 downregulated differentially expressed genes (DEGs) were enriched in categories of genes involved in carbon fixation, namely, 5 RUBISCO genes, 2 FRUCTOSE-BISPHOSPHATE ALDOLASE (FBA) genes, 1 FRUCTOSE-1,6-BISPHOSPHATASE (SBPASE) gene, 1 PHOSPHOGLYCERATE KINASE (PGK) gene, and 6 PHOSPHORIBULOKINASE (PRK) genes (Fig. 4G; Supplemental Table S3). The expression patterns of the 5 genes encoding Rubisco copies were similar; those genes were significantly downregulated in infected plant leaves (Fig. 4G), which was consistent with the changes in the activity of Rubisco between the infected and uninfected plant leaves (Fig. 4E). Further research showed that 13 key genes involved in the Calvin cycle of photosynthesis showed significant downregulation after infection by P. spegazzinii, resulting in a significant decrease in the level of compounds in the Calvin cycle (Fig. 4H).
Furthermore, 87 of 123 (71%) genes related to the light reaction of photosynthesis were downregulated after infection by P. spegazzinii, and the expression of these genes was significantly downregulated in infected leaves (Supplemental Table S3). Seventeen genes encoding photosynthetic antenna proteins were detected in the M. micrantha genome, including LIGHT-HARVESTING COMPLEX I CHLOROPHYLL A/B BINDING PROTEIN (LHCA) and LIGHT-HARVESTING COMPLEX II CHLOROPHYLL A/B BINDING PROTEIN (LHCB); 16 genes of these genes were significantly downregulated by an average of 6.97 times (Supplemental Table S3). In addition, this study found that PSI and PSII share 66 genes, of which 49 genes, including the PSA, PSB, ATPase (such as ATPA and ATPD), and CYTOCHROME C6 (such as PETA and PETB) genes, were significantly downregulated between the CK and PS plants (Supplemental Table S3).
Effects of P. spegazzinii infection on nitrogen-related efficiency in M. micrantha
Here, a total of 72 genes involved in nitrogen transport and assimilation were identified in the M. micrantha genome, of which the expression of 18% of these genes was significantly downregulated after infection by P. spegazzinii (Supplemental Table S3). Eight key genes related to nitrate and ammonium transport (3 copies of Nitrate transporters [NRT] genes and 5 copies of Ammonium transporters [AMT] genes) were significantly downregulated due to rust infection (Fig. 5, A and B). The expression of these genes in the infected leaves was 7.79 times lower, on average, than that in the uninfected plant leaves, the levels of which ranged from 1.57 to 23.06 times (Fig. 5B; Supplemental Table S3). However, the expression of 13 CHLORIDE CHANNEL (CLC) genes involved in nitrate transport did not change under the influence of rust infection (Supplemental Table S3).
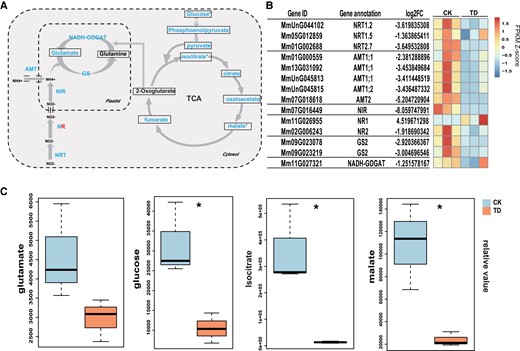
Effects of P. spegazzinii on nitrogen use efficiency in M. micrantha. A) Comparison of the expression of genes and the contents of metabolites related to nitrogen transport and assimilation, the tricarboxylic acid (TCA) cycle, and glycolysis in the leaves of M. micrantha under healthy leaves (CK) and TD stage. The metabolites annotated and quantified are framed. The levels of all metabolites except Glutamine and 2-Oxoglutarate were decreased at the TD phase. Except for one NR gene, all the genes involved in nitrogen transport and assimilation were significantly down-regulated at the TD stage. B) Expression patterns of DEGs related to nitrogen transport and assimilation. The gene names and gene annotation results of M. micrantha are shown on the left. C) Relative content of identified metabolites involved in nitrogen assimilation, the TCA cycle, and glycolysis in the leaves of M. micrantha under CK and TD stage are shown in the boxplot. Within each boxplot, the central line indicates the median, the bottom and top edges of the box indicate the IQRs, and the whiskers represent the maximum and minimum data points. Statistical significance (n = 3) is indicated in (A) and (C), according to Student's t-test: *P < 0.05.
In addition, the expression levels of 1 copy of the NITRITE REDUCTASE (NIR) gene and 1 copy of the NITRATE REDUCTASE (NR) gene were significantly downregulated in the infected leaves (Fig. 5, A and B), suggesting that the concentrations of ammonium in M. micrantha decreased after infection by P. spegazzinii. The GLUTAMINE SYNTHETASE/GLUTAMATE SYNTHASE (GS/GOGAT) cycle plays an important role in plant nitrogen metabolism (Fig. 5A). The expression patterns of 2 GS gene copies and 1 GOGAT gene copy were similar; all 3 genes were significantly downregulated in the infected leaves (Fig. 5, A and B), which reduced the glutamate concentration in the plant leaves. We also analyzed the changes in the contents of metabolites involved in glycolysis and the TCA cycle before and after P. spegazzinii infection (Fig. 5A). In this study, glucose, isocitrate, and malate levels were significantly reduced by 3.02, 27.14, and 4.6 times (Fig. 5C; Supplemental Table S5), respectively, in response to infection. In addition, pyruvate, fumarate phosphoenolpyruvate, oxaloacetate, and citrate levels also decreased by 3.02 times due to P. spegazzinii infection (Fig. 5C; Supplemental Table S5).
Discussion
P. spegazzinii is a host-specific pathogen of M. micrantha and can be safely used to control the damage to M. micrantha. (Ellison et al. 2008; Day et al. 2013). However, no studies have investigated the influence of P. spegazzinii infection on the growth of M. micrantha. In this study, we systematically studied the effects of P. spegazzinii infection on plant hormone synthesis and the metabolic pathways involving photosynthesis and carbon and nitrogen assimilation. The results showed that, by affecting multiple metabolic pathways, JA-Ile may play crucial roles in impeding the rapid growth of M. micrantha. The results certainly provide an improved understanding of the interactions between M. micrantha and P. spegazzinii and the potential of P. spegazzinii as a long-term biological control agent for M. micrantha.
Accumulation of JA/JA-Ile in infected tissues might inhibit SA production and growth-related hormone synthesis and signal transduction
Plant hormones play an important role in response to environmental stress and regulate plant growth, development, and defense ability mainly through their interactions (Pieterse et al. 2009; Santner et al. 2009; Bürger and Chory 2019). JA, a core compound, is involved in plant responses to biological and abiotic stresses via crosstalk with other plant hormone signals (Yang et al. 2019) and controls many transcriptional processes that affect plant growth and development (Howe and Jander 2008; Song et al. 2013; Wasternack 2014). JAR1 converts JA into a biologically active form of JA-Ile to activate the plant immune system (Loake et al. 2017). When M. micrantha was infected by P. spegazzinii in the 3 early stages (EL24, EL48, and EL96), the JA and JA-Ile levels were significantly decreased in infected tissues (Fig. 2E). In contrast, the JA and JA-Ile levels were substantially increased after teliospores formation, not only due to the upregulated expression of biosynthesis gene, but also due to the significantly downregulated expression of degrading genes (Fig. 2B). These results indicated that particular proteins or compounds might be released by P. spegazzinii after teliospores formation, which changed the expression patterns of synthesis and degradation genes in infected leaves, thus promoting the accumulation of JA and JA-Ile (Fig. 6).
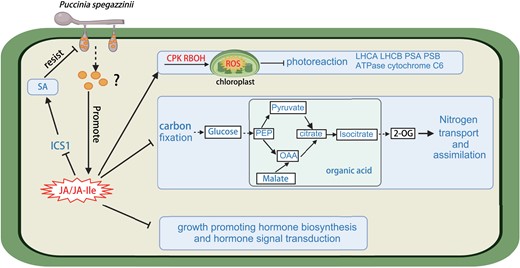
Model of the entire putative mechanism through which P. spegazzinii inhibits the growth of M. micrantha. Proteins or compounds might be released by P. spegazzinii after teliospores formation, promoting the accumulation of JA and JA-Ile in infected leaves of M. micrantha. Higher JA and JA-Ile production in infected leaves inhibits the synthesis of the defense hormone SA, thus reducing inhibitory effect of SA on teliospores development. Accumulation of JA and JA-Ile may also inhibit the synthesis of growth-related hormones and signal transduction and promote the accumulation of ROS, thereby affecting the photochemical efficiency of M. micrantha and impeding carbon and nitrogen metabolism in infected tissues. The annotated and quantified metabolites are boxed, and increases and decreases in contents are marked in red and blue, respectively. Significantly up- and downregulated genes are marked in red and blue, respectively. Significant differences are indicated, according to Student's t-test: *P < 0.05. Inhibited pathways are marked in blue. The lines with arrowheads indicate positive relationships, and the lines with blunt ends indicate inhibition or negative relationships.
In dicots, SA confers resistance to biotrophic pathogens, whereas JA and ethylene are usually associated with defense against necrotrophic pathogens. Accumulating evidence demonstrates that microbial pathogens avoid host immune activation by disrupting plant phytohormone production and signaling pathways. A classic case is coronatine, a toxin secreted by multiple plant pathogenic Pseudomonas spp., which mimics the biologically active JA derivative JA-Ile to dampen plant resistance (Uppalapati et al. 2005; Zheng et al. 2012). Coronatine exploits the antagonistic regulatory crosstalk between JA signaling and SA signaling, leading to compromised stomatal and apoplastic defenses in plants (Katsir et al. 2008; Sakata et al. 2021). Similar results were found in this study; the increased JA/JA-Ile levels significantly inhibited the expression of key gene ICS1 involved in SA synthesis (Fig. 2D), resulting in reduced SA accumulation after P. spegazzinii infection because JA–SA crosstalk blocks the accumulation of SA (An and Mou 2011). This led to the expression of 4 pathogenesis-related genes induced by SA being significantly downregulated (Supplemental Table S3). Therefore, we speculated that the teliospores development stage of rust induced more JA/JA-Ile production in infected leaves to inhibit the synthesis of defense hormone SA, thus reducing the inhibitory effect of teliospores development (Fig. 6).
On the other hand, in the leaves treated with Me-JA, the expression levels of key genes YUC8, YUC10, TAA1, and LOG3 involved in the synthesis of IAA and cytokinin were significantly reduced (Supplemental Fig. S7). This indicates that the accumulation of JA/JA-Ile after P. spegazzinii infection might be antagonistic to the biosynthesis of auxin and cytokinins, thus inhibiting the rapid growth of M. micrantha, which is consistent with the findings of previous studies showing that JA has antagonistic effects on growth-promoting hormones, regulating plant growth (Chen et al. 2011; Yang et al. 2012; Jiang et al. 2014; Campos et al. 2016; Jang et al. 2017; Um et al. 2018).
The increase in JA/JA-Ile in infected tissues may promote ROS accumulation, thus affecting the photochemical efficiency of M. micrantha
Chloroplasts, as plant-specific organelles, are responsible for energy capture and transduction during photosynthesis. Chloroplasts are also a primary source of intracellular ROS, which can act as signaling intermediates involved in cell death (Van Breusegem and Dat 2006). Under normal conditions, the formation of ROS in chloroplasts is minimized by complex and refined antioxidant regulatory mechanisms, resulting in redox homeostasis (Apel and Hirt 2004). Due to the reduction of oxygen in photosynthesis and the presence of chlorophyll and its derivatives, large amounts of ROS are produced in chloroplasts, which might slow growth or contribute to cell death (Tanaka and Tanaka 2006). After Arabidopsis (Arabidopsis thaliana) was treated with Me-JA, the production of ROS was shown to first occur in mitochondria and, subsequently, in chloroplasts. Thereafter, the continuous accumulation of ROS in cells dramatically reduced the photochemical efficiency before an obvious disruption in chloroplast morphology (Zhang and Xing 2008).
In line with previous researchers, we observed that JA/JA-Ile accumulation in M. micrantha leaf tissue after P. spegazzinii infection promoted the upregulation of key genes calcium-dependent protein kinases (CPK) and respiratory burst oxidase homolog (RBOH) involved in ROS synthesis, which may promote ROS levels in cells (Fig. 6; Supplemental Fig. S8). According to the assay of DAB staining and DCFH-DA fluorescent probe, the ROS levels of infected leaves in EL96 and TI stages were obviously higher than those in other stages, and mainly concentrated in chloroplasts (Fig. 4, A and B). And there were similar variation patterns between the accumulation of ROS and JA/JA-Ile, indicating that the ROS level increases may due to the accumulation of JA/JA-Ile in the infected leaves (Figs. 2A and 4A). Further analysis also proved that JA/JA-Ile had a substantial promoting effect on the ROS burst (Supplemental Fig. S5). Next, we were surprised to find that the chloroplast structure was seriously damaged in the rust-infected leaves, possibly due to a great deal of ROS production (Fig. 4C).
Upon further analysis of photosynthesis-related gene expression, we found that 71% of genes related to the light reactions of photosynthesis were significantly downregulated in the leaf tissues after infection by P. spegazzinii. The expression of almost all the genes encoding photosynthetic antenna proteins in M. micrantha, including LIGHT-HARVESTING COMPLEX I CHLOROPHYLL A/B BINDING PROTEIN (LHCA) and LIGHT-HARVESTING COMPLEX II CHLOROPHYLL A/B BINDING PROTEIN (LHCB), was significantly inhibited by an average of 3.37 times after P. spegazzinii infection. In addition, the expression patterns of key genes related to PHOTOSYSTEM I, PHOTOSYSTEM II, ATPASE, and CYTOCHROME C6 displayed were similar; the genes were significantly downregulated due to P. spegazzinii infection. According to the above evidence, we hypothesized that after pathogen infection, the accumulation of JA/JA-Ile induced the accumulation of ROS, which reduced the photochemical efficiency (Fig. 6) and thus slowed the rapid growth of M. micrantha.
JA/JA-Ile overaccumulation may block the metabolism of carbon and nitrogen in infected tissues
More than 90% of plant dry matter consists of carbon-containing compounds, so carbon nutrition plays an extremely important role in plant biological activities. A decrease in carbon, which forms the basic skeleton of plants, reduces the accumulation of organic compounds in plants. Therefore, photosynthetic carbon metabolism plays an important role in plant growth and development. In this study, our results show that the reduction in the photosynthetic rate was due to the destruction of chloroplast structure, the decreased enzyme activity of Rubisco, and the inhibition of stomatal conductance. In addition, we also observed that the expression of almost all the key genes involved in the Calvin cycle was significantly downregulated after P. spegazzinii infection, which resulted in a marked reduction in important metabolites. According to previous studies (Beltrano et al. 1998; Yadav et al. 2005; Bilgin et al. 2010; Nabity et al. 2013; Quan et al. 2018), this probably occurred because JA-Ile overaccumulation after P. spegazzinii infection may inhibit stomatal dynamics and the expression of genes involved in photosynthetic carbon metabolism, thus reducing carbon fixation in M. micrantha (Fig. 6).
Carbon and nitrogen metabolic balance is essential for every biological system, since all major cellular components, including genetic material, proteins, pigments, energy carrier molecules, etc., are derived from carbon and nitrogen. Photosynthesis plays a key role in nitrogen utilization, as photosynthesis provides not only reducing power and ATP for the assimilation of inorganic nitrogen (Nunes-Nesi et al. 2010) but also carbon skeletons composed of organic acids for the assimilation of (Hanning and Heldt 1993). This process involves intermediates such as glucose, phosphoenolpyruvate, pyruvate, malate, oxaloacetate, and citrate and, ultimately, the synthesis of 2-oxoglutarate from isocitrate (Hanning and Heldt 1993; Szal and Podgórska 2012; Baslam et al. 2020). In this study, the inhibition of glycolysis, the Calvin cycle, and the TCA cycle resulted in a significant decrease in the level of intermediate products in the leaves after P. spegazzinii infection. The levels of intermediate products, such as glucose, isocitrate, malate, citrate, and pyruvate, in the infected leaves were more than 1.57 to 27.14 times lower than those in the uninfected plant leaves. As a result, the accumulation of organic acids (carbon skeletons) was reduced in the infected leaves, which inhibited the glutamine synthetase-glutamate synthase (GS-GOGAT) cycle and ultimately to a reduced concentration of nitrogen assimilate production (glutamate) in M. micrantha leaves. Furthermore, the reduction in nitrogen assimilation efficiency inhibited the expression of genes encoding nitrate-nitrogen and ammonia-nitrogen transporters, thus affecting the carbon and nitrogen metabolic balance (Fig. 6) in leaves infected by P. spegazzinii.
Materials and methods
Plant growth conditions and rust inoculation
Seeds of M. micrantha (M. micrantha Kunth) were sown in 15-cm diameter pots that contained soil. The seedlings were allowed to grow under a 25 °C/18 °C day/night temperature, a 16-h light/8-h dark photoperiod, and 60% humidity for 30 d.
P. spegazzinii IMI 393075, a rust-causing fungus (Pucciniales), was introduced into China from Australia. For inoculation, plants with 8 leaves were selected. Plant tissues (leaves, stems, and petioles) with mature P. spegazzinii pustules, indicated by a coppery-brown appearance, were suspended over healthy plants under specific environmental conditions (22 °C, 100% humidity, 48 h of darkness; for details, see Ellison et al. 2008; Day and Riding 2019). After 48 h, the plants were removed, transferred to the greenhouse, and allowed to grow under natural conditions. Then, we divided the plants into 2 groups: an uninfected group and a group infected with P. spegazzinii.
The P. spegazzinii could complete its life cycle within 19 to 22 d. The life cycle involved 3 main stages: (i) the early stage of infection (EL, 0 to 4 d after infection); (ii) then, light green telia is convex on the underside of the symptomatic leaves, which was defined as the TIs (5 to 10 d after infection); and (iii) thereafter, teliospore developed and matured with the telia turned dark brown, called TD stage. (11 to 22 d after infection). In this study, we collected infected leaves at 5 stages of rust infection, including 24, 48, and 96 h post-infection (EL24, EL48, and EL96), 7 to 8 d post-infection (TI), and 15 to 17 d post-infection (TD). The pustules were cut from the leaves to eliminate the metabolites of the rust contaminating the leaf samples and discarded. Leaves of uninfected plants were also collected to serve as controls. All the collected leaf samples were flash frozen in liquid nitrogen and then stored at −80 °C for subsequent metabolite (2 plants used per biological replicate and 3 biological replicates) and plant hormone measurements (2 plants used per biological replicate and 5 biological replicates) and transcriptome sequencing (2 plants used per biological replicate and 3 biological replicates).
The treatment of exogenous hormones
For the inhibitory effect of exogenous hormones on rust infection, we use different exogenous plant hormones to spray the infected leaves of M. micrantha with the same growth condition. The infected leaves of M. micrantha were sprayed with exogenous Me-JA and SA with a concentration of 1 mol L−1 once a day. The experiment carried out 5 biological repeats for each treatment. After 10 d of treatment, it was collected to observe the infection status of P. spegazzinii.
To investigate the effect of plant growth-promoting hormone on rust-infected plants, we used appropriate concentration levels (10−5 and 2 mg L−1) of exogenous IAA and Cytokinin to spray on the infected plants of M. micrantha every day. The experiment had 3 biological replicates for each treatment. After 10 d of treatment, it was collected to measure the vegetative traits of M. micrantha.
Vegetative trait measurements
In this study, the vegetative traits, including the number of branches, SL, number of leaves, and NS, of the plants in the uninfected and infected groups were recorded; specifically, the average of these traits of the above plants at 17 d after infection by P. spegazzinii was recorded. One plant from each plot of the uninfected and infected groups was harvested for the measurement of aboveground DW. These vegetative traits for each group were measured for 10 biological replicates.
Chloroplast observation, DCFH-DA, and DAB staining
The chlorophyll fluorescence assay was performed using a Leica confocal laser scanning microscope (laser = 640 nm for chloroplast observation and 488 nm for ROS assay, intensity = 50%, collection bandwidth = 10 nm, gains = 695) according to the manufacturer's instructions. For ROS detection assay, 0.1% (v/v) diaminobenzidine (DAB) A solution was prepared using 50 mM Tris-HCl (pH = 4.5). The leaves of M. micrantha were completely immersed in DAB solution and dyed overnight at 25 °C away from light. Anhydrous ethanol was used for decolorization several times, and pictures were taken when the chlorophyll was completely removed and the leaves were transparent. ROS measurements were performed by the fluorescence assay. Mycelia were stained with 2′,7′-dichloro-dihydro-fluorescein diacetate for 20 min and visualized under a Leica Laser scanning confocal microscope to assess the presence of H2O2.
Gas exchange measurements
To investigate the effects of infection on the gas exchange of M. micrantha, uninfected and infected leaves at 17 d after inoculation were divided into 4 groups. The first group comprised leaves of uninfected plants (CK); the second group comprised asymptomatic areas of infected leaves (UA); the third group comprised infected areas with 1 pustule (OC); and the fourth group comprised infected areas of infected leaves with 4 pustules (FC). A portable photosynthesis system LI-6400XT (LI-COR, Inc., USA) was used to measure the gas exchange parameters of the 4 groups of leaves in the afternoon (14:00). During the measurements of net photosynthetic rate (Pn), intercellular carbon dioxide concentration (Ci), stomatal conductance (Cond), and transpiration rate (Trmmol), a saturating photosynthetic photon flux density (1,500 μmol m−2 s−1) was emitted from a red and blue (665 and 470 nm, respectively) LED light source (6400-02B) integrated into the LI-6400XT leaf measurement chamber. The area of leaves was a constant 6 cm2. The concentration of CO2 flowing into the leaf chamber was maintained at 400 μmol mol−1, the leaf temperature was maintained at ∼28 °C, and the humidity was 50% to 70%. The photosynthetic traits for each group were measured for 5 biological replicates.
Measurements of metabolites and plant hormones
The composition of metabolites in the TD stage was analyzed by quasi-targeted metabolomics according to the method reported (Want et al. 2013). Fresh plant samples (100 mg) were grounded with liquid nitrogen and the homogenate was resuspended with prechilled 500 μL 80% (v/v) methanol and 0.1% (v/v) formic acid by well vortexing. The samples were incubated on ice for 5 min and then were centrifuged at 15,000 rpm, 4 °C for 10 min. Finally, the supernatant was injected into an LC–MS/MS system for analysis, which was performed using an ExionLC AD system (AB-SCIEX) coupled to a QTRAP 6500 + mass spectrometer (AB-SCIEX) by staff at Novogene Co., Ltd (Beijing, China).
The metabolites of each sample were detected using multiple reaction monitoring, which was based on the contents of the Novogene in-house database. The data files generated by HPLC–MS/MS were processed using SCIEX OS version 1.4 to integrate and correct the peaks. The main parameters were as follows: minimum peak height, 500; signal/noise ratio, 5; and Gaussian smooth width, 1. The area of each peak represents the relative content of the corresponding substance. We used the Kyoto Encyclopedia of Genes and Genomes database (http://www.genome.jp/kegg/), Human Metabolome Database (http://www.hmdb.ca/), and LIPID MAPS database (http://www.lipidmaps.org/) to annotate the metabolites in this study. Metabolites with a P-value <0.05, a variable importance in the projection (VIP) ≥1, and a fold-change (FC) ≥2 or ≤0.5 were considered to be differentially accumulated metabolites. VIP refers to the variable projection importance of the first principal component of the PLS-DA model and represents the contribution of metabolites to the groupings.
The quantification of endogenous auxin (indole-3-acetic acid, IAA) and Cytokinin in the TD stage was performed by ELISA kits (Jiangsu Meimian Industrial Co., Ltd). Fresh M. micrantha leaves were crushed to a powder in liquid nitrogen. The powder (0.1 g) and 0.9 mL of PBS buffer (0.1 mol L−1; pH 7.4) were mixed together. After centrifugation at 3,000 × g at 4 °C for 15 min, the supernatant was collected in a centrifuge tube and placed in an ice bath. The contents of auxin and cytokinin were determined by using ELISA kits (Meimian Biotechnology Co., Ltd, Yancheng, China) according to the manufacturer's instructions. Each treatment included 5 replicates, and the experiment was repeated 5 times.
The quantification of endogenous auxin (indole-3-acetic acid, IAA), Cytokinin, JA, JA-ILE, and SA in the early stage of infection (EL24, EL48, and EL96) and TI stage was performed by Wuhan Metware Biotechnology Co., Ltd (Wuhan, China) using an LC–MS/MS platform as described by Izumi et al. (2009). Take 5 mg of fresh plant sample, freeze it in liquid nitrogen immediately, grind it into powder, and extract it with 1 mL methanol/water/formic acid (15:4:1, v/v/v) at 4 °C; 10 μL internal standard (IS) mixed solution (100 ng mL−1) was added into the extract as ISs for the quantification. The mixture was vortexed for 10 minute and centrifuged for 5 min (12000 r/min, and 4°C). The supernatant was transferred to clean plastic microtubes, evaporated to dryness, dissolved in 100 μL 80% methanol (V/V) and filtered through a 0.22 μm membrane filter for further LC-MS/MS analysis. The sample extracts were analyzed using a UPLC-ESI-MS/MS system (UPLC, ExionLC AD, https://sciex.com.cn/; MS, Applied Biosystems 500 Triple Quadrupole, https://sciex.com.cn/). The analysis conditions are as described by Guo et al. (2021). Standard curves consisting of 0.02, 0.05, 0.1, 0.2, 0.5, 1, 2, 5, 10, 20, 30, 50,100, 200, 400, 800, 1,600, and 2,000 ng mL−1 of IAA, Cytokinin, JA, JA-ILE, and SA were used to quantify each compound.
Identification of genes related to hormone synthesis, photosynthesis, and nitrogen use efficiency in the M. micrantha genome
The sequences of genes that are involved in the patterning pathway of hormone synthesis and signaling, carbon dioxide fixation, and nitrogen transport/assimilation in A. thaliana were downloaded from The Arabidopsis Information Resource (TAIR) database (http://www.arabidopsis.org/). The gene sequences of M. micrantha were aligned to those genes of A. thaliana using BLASTP (Ye et al. 2006) with a threshold of E-value ≤10−20 and ≥70% sequence coverage (Altschul et al. 1997).
Transcriptome sequencing and identification of DEGs
RNA was extracted from uninfected and infected leaf tissues using TRIzol reagent (Invitrogen, Carlsbad, CA, USA) according to the manufacturer's instructions. The purity of the extracted RNAs was assessed on 1% (w/v) agarose gels as well as by a NanoPhotometer spectrophotometer (IMPLEN, Los Angeles, CA, USA). The RNA integrity was assessed by the RNA Nano 6000 Assay Kit of an Agilent Bioanalyzer 2100 system (Agilent Technologies, Santa Clara, CA, USA). Sequencing libraries were generated using a NEBNext Ultra RNA Library Prep Kit following the manufacturer's instructions. The library fragments were purified with an AMPure XP system (Beckman Coulter, Beverly, USA). Then, 3 μL of USER Enzyme (NEB, USA) was used with size-selected, adaptor-ligated cDNA at 37 °C for 15 min followed by 5 min at 95 °C before PCR. Then, PCR was performed in conjunction with Phusion High-Fidelity DNA polymerase, universal PCR primers, and Index (X) Primer. Finally, we used an AMPure XP system to purify the PCR products. The cDNA library quality was assessed on an Agilent Bioanalyzer 2100 system. Finally, the contents of the cDNA library were sequenced on an Illumina HiSeq platform (Illumina, Inc., San Diego, CA, USA) with 150 bp paired-end reads by staff at Novogene Biotech Co., Ltd, Tianjin (http://www.novogene.com).
To eliminate errors generated in sequencing, unreliable reads were filtered according to the following criteria: (i) having a 50% base Phred quality score <20% and (ii) containing more than 10% ambiguous N residues. After filtering, the high-quality reads were mapped to the reference genome sequence of M. micrantha, which was downloaded from the NCBI database (PRJNA528368) using HISAT2 v2.0.5 (Pertea et al. 2016) with the default parameters. The mapped read counts were calculated using StringTie v1.1.3 (Pertea et al. 2016), and the expression levels were calculated using the fragments per kilobase million method. Then, the DESeq2 package version 3.8.6 (Love et al. 2014) was used to identify the DEGs according to the following parameters: |log2(fold-change)| ≥ 1 and false discovery rate < 0.05.
Determination of 1,5-ribulose-diphosphate carboxylase/oxygenase (Rubisco) activities
Rubisco activity was determined by kit micro-method (Shanghai Acmec Biochemical) according to Sawada et al. (2003) and slightly modified. The extract was centrifuged at 10,000 × g for 10 min under 4 °C, and the supernatant was taken and incubated at 0 °C crushing ice for 30 min before determination. The working solution was prepared according to the instructions: add 20 μL extraction of samples and other reaction media into the 96-well transparent plate successively, which were measured at 25 °C in the reaction medium containing. Record the absorbance value A1 at 20 s at 340 nm and A2 at 5 min 20 s, and calculate ΔA = ΔA (measured) − ΔA (blank).
where V is the total volume of the reaction system, 2.14 × 10−4 L; ε the NADH molar extinction coefficient, 6.22 × 103 L mol−1 cm−1; d the 96-well plate light diameter, 0.6 cm; V0 the sample volume added, 0.02 mL; V1 the volume of extractive liquid added, 1 mL; T the reaction time, 5 min; and W the samples quality, g.
RT-qPCR analysis
RT-qPCR analyses were performed on CFX Connect Real-Time PCR Detection System (Bio-Rad, Hercules, CA, USA) using PerfectStart Green qPCR SuperMix (TransGen Biotech, Beijing, China) following the manufacturer's instructions. The PCR amplification program was 94 °C (30 s), followed by 40 cycles at 94 °C (5 s), 50 °C (15 s), and 72 °C (10 s). Fold changes in gene expression level were calculated using the 2−ΔΔCT method. The polyubiquitin gene was used as a candidate reference gene (Czechowski et al. 2005). The primers used in this study are given in Supplemental Table S6.
Statistical analyses
Data were analyzed using the IBM SPSS Statistics version 26.0 (International Business Machines Corporation, USA). Student's t-test was used to evaluate the difference between the 2 groups. P < 0.05 was considered significantly different.
Accession numbers
Sequence data of leaf samples used in this study have been deposited at the National Center for Biotechnology Information (NCBI, https://www.ncbi.nlm.nih.gov) under the accession number PRJNA528368.
Author contributions
X.H.R. and X.Q. carried out the sample collection and rust inoculations. G.Z.Z., Z.Y.L., and C.H.L. provided technical support for the bioinformatics analysis. G.Z.Z., C.J.Z.W., and S.C.S. provided technical support for experiments. G.Z.Z., C.J.Z.W., and B.L. wrote the manuscript. W.Q.Q., B.L., F.D.Z., and F.H.W. designed the experiments. W.Q.Q. and B.L. coordinated the project and revised the paper. All the authors contributed to the article and approved the submitted version.
Supplemental data
The following materials are available in the online version of this article.
Supplemental Figure S1. Relative level of JA, JA-Ile, and SA in leaves of M. micrantha at TD stage.
Supplemental Figure S2. Inhibitory effect of exogenous methyl jasmonate (Me-JA) and salicylic acid (SA) on the development of P. spegazzinii.
Supplemental Figure S3. Relative level of growth-promoting hormones (IAA and Cytokinins) in leaves of M. micrantha at TD stage.
Supplemental Figure S4. Phenotypic differences of M. micrantha under growth-promoting hormones (IAA and cytokinins) treatment to P. spegazzinii.
Supplemental Figure S5. Accumulation of ROS in M. micrantha leaves after Me-JA treatment for 24 h by DAB staining and DCFH-DA fluorescent probe.
Supplemental Figure S6. Effect of P. spegazzinii infection on gas exchange in M. micrantha leaves.
Supplemental Figure S7. Effects of exogenous Me-JA spraying on the mRNA levels of key synthetic genes for IAA (YUC8, YUC10, and TAA1) and cytokinin synthesis (LOG3).
Supplemental Figure S8. The expression pattern of M. micrantha genes for calcium-dependent protein kinases (CPKs) and respiratory burst oxidase homolog (RBOH) involved in ROS synthesis at TD stage.
Supplemental Table S1. Effects of growth phenotype (n = 10) in M. micrantha after infection by P. spegazzinii.
Supplemental Table S2. Plant hormone (auxin, cytokinin, jasmonic acid, jasmonate-isoleucine, and salicylic acid) content in leaves of M. micrantha at different stages of rust infection.
Supplemental Table S3. The details of differentially expressed genes (DEGs) involved in hormone biosynthesis, signal transduction, light and dark reactions, and nitrogen use pathways.
Supplemental Table S4. Effect of P. spegazzinii infection on net photosynthetic rate, stomatal conductance, intercellular carbon dioxide concentration, transpiration rate, and Rubisco enzyme activity of M. micrantha.
Supplemental Table S5. Metabolite contents in leaves of M. micrantha at TD stage under CK and PS conditions.
Supplemental Table S6. The primers used in this research.
Funding
The work was funded by the National Key R&D Program of China (2021YFC2600100 & 2021YFC2600101).
Data availability
The data that support the findings of this study are available from the corresponding author upon reasonable request.
References
Author notes
These authors contributed equally to this work.The author responsible for distribution of materials integral to the findings presented in this article in accordance with the policy described in the Instructions for Authors (https://dbpia.nl.go.kr/plphys/pages/General-Instructions) are Bo Liu ([email protected]), Wanqiang Qian ([email protected]).
Conflict of interest statement. The authors have no conflict of interest to declare.