-
PDF
- Split View
-
Views
-
Cite
Cite
Ke Xu, Joris Jourquin, Xiangyu Xu, Ive De Smet, Ana I Fernandez, Tom Beeckman, Dynamic GOLVEN-ROOT GROWTH FACTOR 1 INSENSITIVE signaling in the root cap mediates root gravitropism, Plant Physiology, Volume 192, Issue 1, May 2023, Pages 256–273, https://doi.org/10.1093/plphys/kiad073
- Share Icon Share
Abstract
Throughout the exploration of the soil, roots interact with their environment and adapt to different conditions. Directional root growth is guided by asymmetric molecular patterns but how these become established or are dynamically regulated is poorly understood. Asymmetric gradients of the phytohormone auxin are established during root gravitropism, mainly through directional transport mediated by polarized auxin transporters. Upon gravistimulation, PIN-FORMED2 (PIN2) is differentially distributed and accumulates at the lower root side to facilitate asymmetric auxin transport up to the elongation zone where it inhibits cell elongation. GOLVEN (GLV) peptides function in gravitropism by affecting PIN2 abundance in epidermal cells. In addition, GLV signaling through ROOT GROWTH FACTOR 1 INSENSITIVE (RGI) receptors regulates root apical meristem maintenance. Here, we show that GLV-RGI signaling in these 2 processes in Arabidopsis (Arabidopsis thaliana) can be mapped to different cells in the root tip and that, in the case of gravitropism, it operates mainly in the lateral root cap (LRC) to maintain PIN2 levels at the plasma membrane (PM). Furthermore, we found that GLV signaling upregulates the phosphorylation level of PIN2 in an RGI-dependent manner. In addition, we demonstrated that the RGI5 receptor is asymmetrically distributed in the LRC and accumulates in the lower side of the LRC after gravistimulation. Asymmetric GLV-RGI signaling in the root cap likely accounts for differential PIN2 abundance at the PM to temporarily support auxin transport up to the elongation zone, thereby representing an additional level of control on the asymmetrical auxin flux to mediate differential growth of the root.
Introduction
Root tropic responses are essential for plant survival since they account for directed growth toward vital resources such as water and nutrients and away from unfavorable conditions. Directional growth changes imply the establishment of asymmetric molecular patterns across tissues in the root tip which are only partially understood. Of all plant tropic responses, the gravitropic response is probably the most extensively studied, although many aspects remain elusive. In roots, gravity perception is known to occur in statoliths-containing columella cells present in the central part of the root cap (Leitz et al. 2009; Levernier et al. 2021). The actual response (curvature formation), however, takes place in the root elongation zone as a consequence of differential cell expansion between the lower and upper sides (Wolverton et al. 2002; Miller et al. 2007). The spatial separation between gravity perception and response in the root suggests that active signaling is essential for gravitropism.
The phytohormone auxin serves as a signal molecule and plays a crucial role during plant gravitropism. Misalignment of the main root axis with respect to the gravity vector (also called gravistimulation) triggers amyloplast sedimentation and redirection of auxin gradients to the lower organ side (Friml et al. 2002; Kramer and Bennett 2006; Band et al. 2012; Su et al. 2017). The latter can be monitored by the use of auxin reporters, such as those containing the synthetic auxin-responsive promoter DR5 (Ottenschlager et al. 2003). These auxin gradients are generated by the activity of auxin transport proteins. After root gravistimulation, PIN-FORMED3 (PIN3) and PIN7 present in columella cells are polarized to the downward-oriented cell membrane, redirecting auxin fluxes to the lower root side (Friml et al. 2002; Kleine-Vehn et al. 2010). Auxin is then transported through the lateral root cap (LRC) and epidermal cells by AUX1 and PIN2 up to the elongation zone, where its accumulation inhibits cell elongation leading to the gravicurvature and reoriented growth toward gravity (Swarup et al. 2005; Su et al. 2017). After gravistimulation, PIN2 was found to be asymmetrically distributed in the root with more PIN2 at the lower side as a consequence of differential intracellular trafficking and PIN2 degradation between the upper and lower root sides (Abas et al. 2006; Kleine-Vehn et al. 2008; Leitner et al. 2012). Accordingly, chemical inhibition of PIN2 degradation led to impaired root gravitropism (Abas et al. 2006; Leitner et al. 2012). However, the molecular basis involved in determining or maintaining PIN2 asymmetry is not well understood. PIN2 distribution was shown to be regulated by Rho-like GTPase from Plants 6 (ROP6)-GTPase (Lin et al. 2012) and a recent report showed that a signaling pathway involving members of the transmembrane kinase (TMK) receptors family and membrane-associated kinase regulator2 (MAKR2) likely acts upstream of ROP6 during gravitropism (Marques-Bueno et al. 2021).
In recent years, an increasing number of cell-to-cell communication molecules have been uncovered in plants including endogenous peptides shown to participate in most of the plant developmental processes (Hirakawa and Sawa 2019; Kaufmann and Sauter 2019; Olsson et al. 2019; Jourquin et al. 2020; Jeon et al. 2021). The GOLVEN (GLV) peptides, also called CLE-LIKE (CLEL) or root meristem growth factors (RGFs), have been proposed previously to regulate root and hypocotyl gravitropism (Matsuzaki et al. 2010; Meng et al. 2012; Whitford et al. 2012). Accordingly, the overexpression of several GLV genes results in disturbed root growth patterns including exaggerated waving and curling, from which the name GOLVEN (meaning “waves” in Dutch) is derived (Meng et al. 2012; Whitford et al. 2012; Fernandez et al. 2013). GLV3 peptide treatment or gene overexpression was previously shown to affect PIN2 plasma membrane (PM) levels in root epidermal cells (Whitford et al. 2012).
GLV peptides were also found to regulate root apical meristem (RAM) maintenance by regulating the levels of the transcription factors PLETHORA1 and 2. An rgf1 rgf2 rgf3 triple mutant (corresponding to glv5 glv7 glv11) has reduced RAM size and transit amplifying cell number (Matsuzaki et al. 2010). Mutants in the TYROSYLPROTEIN SULPHOTRANSFERASE (TPST) gene, defective in the production of sulfonated and thus, bioactive GLV peptides, display similar phenotypes (Matsuzaki et al. 2010; Zhou et al. 2010). RGF1 INSENSITIVE (RGI) receptors (also called RGFRs), a subfamily of 5 leucine-rich repeat receptor-like kinases (LRR-RLK), were identified as receptors for GLV/RGF peptides (Ou et al. 2016; Shinohara et al. 2016; Song et al. 2016) and SOMATIC EMBRYOGENESIS RECEPTOR KINASEs (SERKs) serve as co-receptors of the pathway (Song et al. 2016; Ou et al. 2022). Higher-order RGI mutants display similar RAM defects as rgf and tpst mutants. It is, however, not known whether GLV function in root gravitropic responses is also mediated by the RGI receptors. Furthermore, it is at the moment unclear whether GLV signaling during these 2 processes is uncoupled or part of a chain of events. Here, we show that RGI receptors also mediate GLV signaling in Arabidopsis (Arabidopsis thaliana) root tropic responses. We found that RGI5 is expressed and asymmetrically distributed after gravistimulation in LRC cells and that GLV-RGI signaling in the root cap is essential for gravitropism but not for RAM maintenance. We propose that asymmetric GLV-RGI signaling in the LRC accounts for transient PM PIN2 abundance at the lower root side, thereby ensuring efficient auxin transport to the elongation zone during gravitropic responses.
Results
GLV signaling positively regulates root gravitropism in an RGI-dependent manner
We previously reported on the overexpression of GLV genes resulting in altered root gravitropism (Whitford et al. 2012; Fernandez et al. 2013). Still, how GLV signaling participates in the delicate root gravitropism regulatory network remains unresolved. To get insight into this process, we determined the gravitropic curvature of GLV receptor loss-of-function (lof) mutants by quantifying the gravitropic bending angles upon gravistimulation. Among the 5 receptor genes, RGI3 and RGI4 exhibited low or no expression in the root tip (Ou et al. 2016; Shinohara et al. 2016; Song et al. 2016); for that reason, we only focused on RGI1, RGI2, and RGI5. No difference between wild-type and rgi1 or rgi2 mutants could be measured, while both rgi5 mutants showed a weaker gravitropic bending (Fig. 1, A and B). The disruption of root gravitropism observed in the rgi5-1 mutant could be restored by the expression of the RGI5-GFP/Venus protein driven by its native promoter (Fig. 1, A and C). Moreover, the gravitropic defect of rgi5 mutants was further enhanced in a rgi1 rgi2 rgi5 (referred to as rgi125) triple mutant (Fig. 1, A and B). These results demonstrate that RGI1, RGI2, and RGI5 function redundantly during root gravitropism, while the analysis of single lof mutants revealed RGI5 to play a major role in this process.
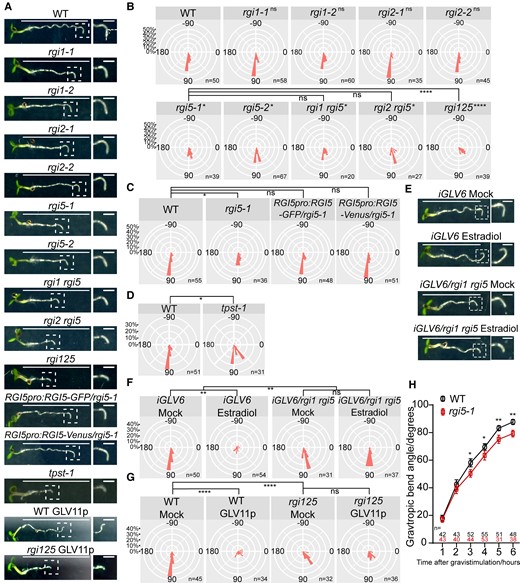
GLV signaling positively regulates root gravitropism in an RGI-dependent manner. A) and E) Representative images of 4 dag seedlings after 6 h of gravistimulation. Images at the right show a higher magnification of the bending root tip (framed in the left image); 100 nM GLV11p A) or 2 μM estradiol E) were used. Scale bars in the left images represent 1 cm and bars in the right images represent 1 mm. B) Root gravitropic bend in rgi mutants. Distribution of the root bending angle after gravistimulation for the indicated genotypes represented as nightingale plots (bin = 10°). Dunn test, preceded by Kruskal–Wallis test, was performed when comparing wild-type with rgi mutants and comparing rgi5-1 mutant with rgi1 rgi5, rgi2 rgi5, and rgi125. *P < 0.05; ****P < 0.0001; and ns indicates no significant difference (P < 0.05). Experiments were done in 3 replicates with similar results. C) Expression of RGI5-GFP/Venus driven by its native promoter in rgi5-1 complemented its gravitropism defect. Distribution of the root bending angle after gravistimulation for the indicated genotypes represented as nightingale plots (bin = 10°). Dunn test is preceded by Kruskal–Wallis test. *P < 0.05, and ns indicates no significant difference (P < 0.05). Experiments were done in 2 replicates with similar results. D) Root gravitropic bend in tpst-1 mutant. Distribution of the root bending angle after gravistimulation for the indicated genotypes represented as nightingale plots (bin = 10°). Significant differences were determined using Kolmogorov–Smirnov test, *P < 0.05. Experiments were done in 2 replicates with similar results. F) iGLV6/rgi1 rgi5 suppressed GLV6OE-induced root agravitropic phenotype upon estradiol treatment. Distribution of the root bending angle after gravistimulation for the indicated genotypes/treatment represented as nightingale plots (bin = 10°); 2 μM estradiol was used. Significant differences were determined using 2-way ANOVA and followed by the Sidak test. **P < 0.01, and ns indicates no significant difference (P < 0.05). This experiment was done 2 times with similar results. G) rgi125 suppressed GLV11p-induced agravitropic phenotype upon GLV11p treatment. Distribution of the root bending angle after gravistimulation for the indicated genotypes represented as nightingale plots (bin = 10°); 100 nM GLV11p was used. Dunn test is preceded by Kruskal–Wallis test. ****P < 0.0001, and ns indicates no significant difference (P < 0.05). Experiments were done in 3 replicates with similar results. H) Time-course analysis of gravitropic curvature in wild-type and rgi5-1 mutant. Error bars represent the standard error of the mean. Significant differences between wild type and rgi5-1 in each time point were determined using Kolmogorov–Smirnov test, *P < 0.05 and **P < 0.01. Experiments were done in 2 replicates with similar results. WT, wild type.
To verify whether or not the deviating gravitropic response was due to a defect in the GLV-RGI signaling pathway, we also measured the gravitropic bending angle in the tpst-1 mutant, a defective mutant in the GLV peptide maturation pathway. Again, a decreased bending angle was observed (Fig. 1, A and D). Furthermore, we introduced an estradiol-inducible GLV6OE line (iGLV6) (Fernandez et al. 2020) into the rgi1 rgi5 mutant and confirmed that, upon estradiol induction, the GLV6OE-associated agravitropic phenotype was strongly suppressed (Fig. 1, E and F). Additionally, rgi125 appeared to be insensitive to GLV11p treatment and suppressed the GLV11p-induced root agravitropic phenotype (Fig. 1, A and G). Together, these data suggest that GLV signaling positively regulates root gravitropism in an RGI-dependent manner.
Root gravitropism involves, in order of appearance, amyloplast-mediated gravity sensing, signal transduction, and differential cell expansion (Su et al. 2017). To determine in which of these process(es) GLV signaling might participate, we performed root gravitropism assays in a time-course comparing wild type with rgi5-1. rgi5-1 showed diminished gravitropic bending in the period from 3 to 6 h post-gravistimulation, while no differences were detected during the first 2 h (Fig. 1H). rgi5-1 is thus most likely not defective in gravity sensing, which is known to affect root gravitropic bending also during the first 2 h (Wolverton et al. 2011). Furthermore, we did not observe reduced starch granule accumulation in columella cells that could be associated with the reduced root gravitropic response of the rgi125 mutant. Instead, rather an increase in starch accumulation was apparent in rgi125 columella cells as well as in the RAM region. These data argue for the attenuated root gravitropic bending in GLV-defective mutants not to be provoked by defective gravity sensing due to less starch accumulation. In addition, no cell length differences between wild type and rgi125 were measured in the young root differentiation zone, indicating that the observed reduced root gravitropic bending angles in rgi mutants cannot be attributed to a general defect in cell elongation (Supplemental Fig. S1).
RGI5 shows asymmetric distribution during root gravitropism
Next, we analyzed in detail the expression of RGI1, RGI2, and RGI5 in the different RAM cell layers by making use of promoter-GFP lines (RGI1pro:NLS-2XGFP, RGI2pro:erGFP, and RGI5pro:NLS-2XGFP). RGI1 and RGI2 were mainly expressed in the endodermis, cortex, and epidermis with RGI1 also in columella cells, while RGI5 appeared primarily to show expression in LRC cells (Fig. 2A and Supplemental Fig. S2A). Furthermore, translational fusion constructs of RGI1 and RGI5 to GFP or Venus were transformed into the corresponding rgi mutants (RGIpro:RGI-GFP or Venus/rgi) and microscopically analyzed. Localization of both the RGI proteins overlapped with the sites of promoter activity, except that RGI1-GFP was not found in columella cells, indicating that the protein might not be stable in these cells under the assayed growth conditions (Fig. 2B and Supplemental Fig. S2B). Remarkably, we noticed that RGI5-GFP was not always evenly distributed in LRC with one side of the root cap showing stronger RGI5-GFP levels. More precisely, we observed the RGI5-GFP signal to accumulate at the concave side of LRC during root waving (Fig. 2B and Supplemental Movie S1). To determine if this pattern is specific to RGI5 or the consequence of asymmetry in the root cap due to morphological changes during root growth, we imaged another PM-localized protein BRI1-mCitrine in pBRI1-BRI1-mCitrine/bri1 plants during root waving. Unlike RGI5-GFP, the BRI1-mCitrine signal was equally distributed at both LRC sides in still and time-lapse imaging (Fig. 2B and Supplemental Movie S2). The observed asymmetry in RGI5-GFP levels, therefore, most likely represents dynamics in RGI5 protein abundance in the LRC during root growth.
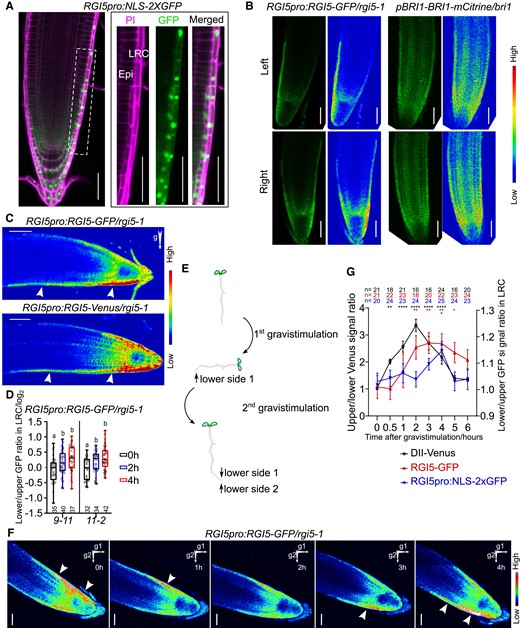
RGI5 is asymmetrically distributed in the LRC. A) Confocal image of RGI5 transcriptional reporter. Images at the right show higher magnification of the framed area (LRC cells) in the left images. Cells were counter-stained with PI. NLS, nuclear localization signal, Epi, epidermis. Scale bars represent 50 μm. B) Confocal images of RGI5pro:RGI5-GFP/rgi5-1 and pBRI1-BRI1-mCitrine/bri1 roots bending left or right during root waving. Rainbow images display high or low levels GFP/mCitrine signal as indicated. Scale bars represent 50 μm. C) Confocal images of RGI5pro:RGI5-GFP or Venus roots after 4 h gravistimulation. Arrows indicate the direction of gravity after gravistimulation and arrowheads point out RGI5 accumulation. Bars represent 50 μm. D) Quantification of the lower/upper GFP signal ratio in LRC cells after gravistimulation (2 and 4 h) compared to non-gravistimulated seedlings (0 h). For the latter, the left/right GFP signal ratio is shown. Log transformation was used after the ratio quantification. Individual data points for each seedling are shown. In the boxplot, boxes indicate ranges from the first to third quartiles, and the central lines display the median. Upper and lower whiskers extend to maximum or minimum values no further than 1.5 times IQR (interquartile range, the distance between the first and third quartiles). Significant differences were determined using 1-way ANOVA followed by a Tukey’s test. Lowercase letters indicate statistical differences between different time points (P < 0.05). Each experiment was done with 2 independent transgenic lines (9-11 and 11-2) that showed the strongest GFP signal and was repeated 4 times with similar results. E) Schematic representation of the performed experiment comprising 2 consecutive gravistimulation steps. Vertically grown RGI5pro:RGI5-GFP/rgi5-1 seedlings were turned 90 degrees clockwise for 4 h (1st gravistimulation), then turned back (2nd gravistimulation), and immediately imaged. F) Confocal images of RGI5pro:RGI5-GFP/rgi5-1 roots after the 2nd gravistimulation step in the experiment described in E). Arrows indicate gravity direction after the 1st and 2nd gravistimulation steps. Arrowheads indicated the RGI5 accumulation. This experiment was done 4 times with similar results. Scale bars represent 50 μm. G) Time-course analysis of upper/lower Venus signal ratio of DII-Venus and lower/upper GFP signal from RGI5pro:RGI5-GFP and RGI5pro:NLS-2XGFP. Error bars represent the standard error of the mean. Significant differences between each time point and time = 0 were determined by 1-way ANOVA followed by Fisher's LSD test. *P < 0.05, **P < 0.01, and ****P < 0.0001. This experiment was done 2 times with similar results.
We next analyzed the distribution of the RGI5-GFP/Venus protein after gravistimulation. An enrichment of the RGI5-GFP/Venus signal in the LRC at the lower root side was observed and was further confirmed by the calculation of the lower/upper RGI5-GFP/Venus ratios (Fig. 2, C and D). To further confirm the preference of RGI5 for the lower side of gravistimulated roots, we performed time-lapse imaging after 2 consecutive gravistimulation steps. More specifically, we first gravistimulated roots by turning the Petri-dish clockwise (+90 degrees) for 4 h and subsequently turned it back (counter-clockwise 90 degrees) to 0 degrees (Fig. 2E). During the first gravistimulation, the roots made a curvature, while RGI5-GFP was accumulating at the lower side. When returning the Petri-dishes to the original orientation and due to the established curvature, the lower side now becomes the upper side. Time-lapse imaging showed that the signal flipped from this new upper to the new lower LRC after the second gravistimulus (Fig. 2F and Supplemental Movie S3). These data demonstrate that the distribution of RGI5 is affected by the direction of gravity and RGI5 preferably accumulates at the lower side during root gravitropism.
In addition, RGI5 expression has been reported to be induced by auxin (Fernandez et al. 2020). Therefore, we questioned if the RGI5 asymmetry could be caused by auxin-induced RGI5 expression. To answer this, we compared the kinetics upon gravistimulation of the auxin marker DII-Venus, RGI5-GFP, and the RGI5 transcriptional reporter, RGI5pro:NLS-2XGFP. First, the auxin marker rapidly became asymmetrically distributed already after half an hour of gravistimulation. Second, the RGI5-GFP asymmetry became apparent at 2 h post-gravistimulation. Third, RGI5 transcription also displayed asymmetry but only after 4 h gravistimulation, which was much later than RGI5 protein asymmetry (Fig. 2G). RGI5 protein asymmetry thus seems to be formed ahead of higher RGI5 expression but is following the induced auxin asymmetry at the lower root side, suggesting that RGI5 asymmetry is the consequence of dynamic regulation of de novo synthesis and protein degradation at both sides of the root. Even though RGI5 asymmetry followed the auxin asymmetry in terms of time, exogenous application of the auxin analog 1-naphthaleneacetic acid (NAA) or the auxin transport inhibitor naphthylphthalamic acid (NPA) failed to break RGI5 asymmetry (Supplemental Fig. S3, A–E), indicating that the RGI5 asymmetry is most probably not a direct result of the auxin asymmetry. Consistently, RGI5 asymmetry was also unaffected in pin2 lof mutant, in which the auxin gradient is impaired (Supplemental Fig. S3, F and G).
GLV-RGI signaling in the LRC is necessary for root growth patterns but not for RAM maintenance
The asymmetrically distributed RGI5 in LRC cells prompted us to investigate whether the activation of the GLV-RGI pathway in these cells is necessary for root gravitropism and accounts for the defects observed in the lof mutants. At the same time, we intended to answer whether the function of GLV-RGI signaling in RAM maintenance as described earlier (Ou et al. 2016; Shinohara et al. 2016; Song et al. 2016) can also be mapped to these or other RAM cell layers. For this, we expressed an RGI1-Venus fusion protein in iGLV6/rgi1 rgi5 using the root cap-specific promoter SOMBRERO (SMB) (Willemsen et al. 2008). Alternatively, the RIBOSOMAL PROTEIN 5A (RPS5A) promoter was used because it shows expression in dividing cells in RAM but no or low expression in quiescent center (QC) and LRC cells (Weijers et al. 2001). The rgi1 rgi5 mutant background was chosen as it displayed mild defects in both root gravitropism and RAM size (Fig. 1, A and B; Supplemental Fig. S4). We obtained 13 SMBpro:RGI1-Venus/iGLV6/rgi1 rgi5 and 7 RPS5Apro:RGI1-Venus/iGLV6/rgi1 rgi5 independent T2 lines. Confocal imaging confirmed that RGI1-Venus expression was restricted to LRC in SMB-driven lines, while for RPS5A-driven lines, RGI1-Venus was detected in the root meristem and some inner LRC cells but not in the outmost LRC and columella cells (Fig. 3A). We then assessed the capacity to complement the root gravitropism or decreased RAM size observed in iGLV6/rgi1 rgi5 mutant without estradiol. The result showed that all SMBpro:RGI1-Venus/iGLV6/rgi1 rgi5 lines (13/13 lines) complemented the root gravitropism, while RGI1-Venus expression under RPS5A promoter did not in most of the independent lines (6/7 lines) (Fig. 3, B and C; Supplemental Fig. S5), confirming that RGI expression in LRC cells is sufficient to complement root gravitropism in this mutant. Interestingly, analysis of RAM size in these lines showed a different result. The RAM size defect in the mutant was not complemented in SMBpro:RGI1-Venus/iGLV6/rgi1 rgi5 (0/13 lines), but was complemented in RPS5pro:RGI1-Venus/iGLV6/rgi1 rgi5 lines (7/7 lines) (Fig. 3, D and E). These results indicate that GLV-RGI function in root gravitropism and RAM maintenance can be uncoupled to different RAM cell types and demonstrate that the root gravitropism defect in the mutant is not merely the consequence of reduced number of meristematic cells. Furthermore, the LRC (and especially the outmost LRC cells) seems to be important for GLV-RGI signaling in root growth patterns but not for maintaining meristematic activity. Remarkably, RGI1-Venus driven by the SMB promoter also showed asymmetric distribution and enrichment at the lower LRC side upon gravistimulation (Supplemental Fig. S6). In addition, by expressing RGI1-Venus in the root cap in a rgi125 mutant background (SMBpro:RGI1-Venus/rgi125), gravitropic defects largely disappeared while the RAM size was not affected (Supplemental Fig. S7), confirming that RGI mediates the control of separate developmental programs that take place in different cells layers. A detailed time-course of the gravitropic bending assay revealed enhanced bending in SMBpro:RGI1-Venus/rgi125 compared to that in rgi125 from 3 to 6 h post-gravistimulation (Fig. 3F). This observation is in accordance with the time-course of the gravitropic bending comparing wild-type with rgi5-1 mutants, suggesting that the GLV signaling in the root cap is rather involved in gravity signal transmission to the elongation zone and curvature response than gravity perception.
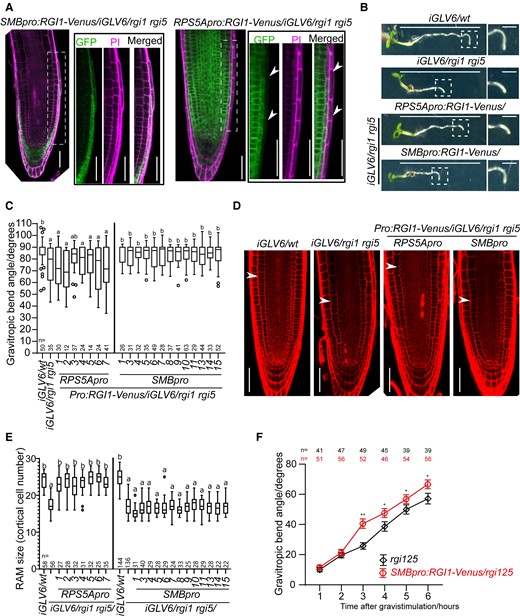
GLV-RGI signaling in the LRC is required for root gravitropism but not for RAM maintenance. A) Confocal images of SMBpro:RGI1-Venus/iGLV6/rgi1 rgi5 and RPS5Apro:RGI1-Venus/iGLV6/rgi1 rgi5 root tips. Images at the right show higher magnification of the framed area (LRC cells) in the left images. Cells were counter-stained with propidium iodide (PI). Arrowheads in RPS5Apro:RGI1-Venus/iGLV6/rgi1 rgi5 root tip indicate the LRC cells where Venus was not detected. Scale bars represent 50 μm. B) Representative images of 4 d after germination (dag) seedlings after 6 h of gravistimulation. Images at the right show a higher magnification of the bending root tip (framed in the left image). Scale bars in the left images represent 1 cm and bars in right images represent 1 mm. C) Quantification of gravitropic bending angle in the iGLV6/rgi1 rgi5 mutant transformed with RGI1-Venus expressed in the LRC (SMBpro:RGI1-Venus/iGLV6/rgi1 rgi5) or other meristematic cells (RPS5Apro:RGI1-Venus/iGLV6/rgi1 rgi5). In the boxplot, boxes indicate ranges from the first to third quartiles, and the central lines display the median. Upper and lower whiskers extend to maximum or minimum values no further than 1.5 times IQR (interquartile range, the distance between the first and third quartiles). Dunn test is preceded by Kruskal–Wallis test. Lowercase letters indicate statistical differences between genotypes (P < 0.05). Experiments were done in 2 replicates with similar results. D) Complementation of the RAM size defect in the iGLV6/rgi1 rgi5 mutant by RGI1-Venus expression in the LRC (SMB promoter) or other meristematic cells (RPS5A promoter). Confocal images show cells stained with PI in 5 dag seedlings. Arrowheads indicate the end of RAM. Scale bars represent 50 μm. E) RAM size quantification in the iGLV6/rgi1 rgi5 mutant transformed with RGI1-Venus expressed in the LRC (SMBpro:RGI1-Venus/iGLV6/rgi1 rgi5, abbreviated as SMBpro) or other meristematic cells (RPS5Apro:RGI1-Venus/iGLV6/rgi1 rgi5, abbreviated as RPS5Apro). In the boxplot, boxes indicate ranges from the first to third quartiles, and the central lines display the median. Upper and lower whiskers extend to maximum or minimum values no further than 1.5 times IQR (interquartile range, the distance between the first and third quartiles). Significant differences were determined using 1-way ANOVA and followed by a Tukey’s test. Lowercase letters indicate statistical differences between genotypes (P < 0.05). Experiments were done in 2 replicates with similar results. F) Time-course analysis of gravitropic curvature in rgi125 and SMBpro:RGI1-Venus/rgi125 mutant. Error bars represent the standard error of the mean. Significant differences between rgi125 and SMBpro:RGI1-Venus/rgi125 mutant in each time point were determined using Kolmogorov–Smirnov test, *P < 0.05 and **P < 0.01. Experiments were done in 2 replicates with similar results. WT, wild type; Pro, promoter.
GLV–RGI signaling positively regulates PIN2 PM levels and efficient auxin efflux mainly from the LRC
Auxin is the dominant messenger during root gravitropism. In this process, rootward auxin is redistributed in columella cells and transferred shootward to the elongation zone where it differentially regulates cell expansion to form the root gravitropic bend. Therefore, we analyzed cellular auxin responses as a proxy for auxin distribution in wild-type, rgi5-1, and rgi125 mutants. The ratio of the DR5-GFP signal (lower versus upper root side) followed a similar pattern in all genotypes during the first hours after gravistimulation; however, a stronger auxin asymmetry was detectable at 6 h of gravistimulation in rgi5-1 and rgi125 mutants (Fig. 4A). The longer-lasting DR5-GFP signal at the lower root side in the mutants suggests that RGI signaling might be contributing to the auxin efflux from the LRC cells to the elongating epidermal/cortical cells. In line with this thought, the difference of cortical cell lengths between the upper and lower in the elongation zone was reduced in rgi5-1 compared to that in the wild type (Supplemental Fig. S8). These data indicate that RGI signaling might be involved in supporting the shootward auxin flux during gravitropism, thereby controlling an adequate effect on cell elongation.
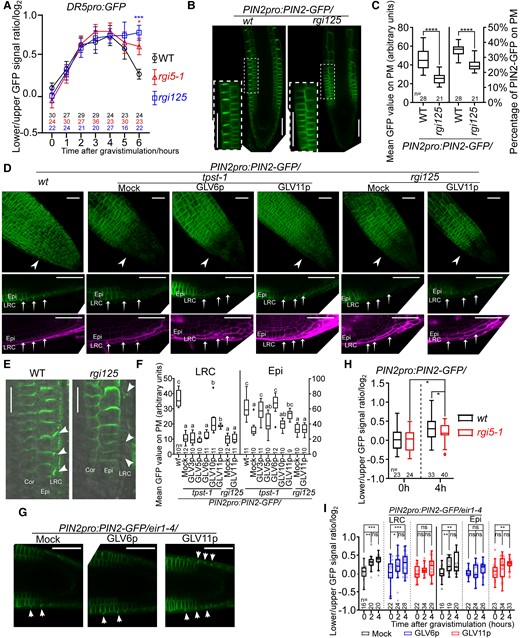
GLV-RGI signaling positively regulates PIN2 PM levels and efficient auxin efflux mainly from the LRC. A) Time-course analysis of the lower/upper DR5pro:GFP signal ratio in wild-type, rgi5-1, and rgi125 mutants. Log transformation was used after the ratio quantification. Error bars represent the standard error of the mean. Significant differences between wild type to rgi5-1 and rgi125 mutant at each time point were determined by 1-way ANOVA followed by Turkey's test. *P < 0.05 and ***P < 0.001. This experiment was done 2 times with similar results. B) Median confocal sections of PIN2-GFP in the wild type and mutant background. Inset shows a higher magnification of epidermal and cortical cells in the framed area. Scale bars represent 50 μm. C) Quantification of the mean PIN2-GFP signal at the PM alone (left) or normalized by the total PIN2-GFP signal in the cell (right). Significant differences were determined using a student's t-test. ****P < 0.0001. Experiments were done with 3 replicates with similar results. D) Confocal images of PIN2-GFP in the wild type or mutants background germinated or not in medium supplemented with GLV peptides (100 nM) as indicated. The upper row images show 3D projections. The lower 2 rows show median sections of PIN2-GFP (green) roots counterstained with PI (violet). Arrowheads and arrows point out LRC cells. Scale bars represent 50 μm. E) Confocal images of PIN2 detected by immunolocalization. Arrowheads point out LRC cells. Bars represent 20 μm. F) Complementation of the PIN2-GFP signal in the tpst-1 or rgi125 mutants on the PM of LRC cells (left) or epidermal cells (right). Roots were transferred to the medium containing 100 nM of the different peptides or peptide-free medium for 48 h as indicated. Significant differences were determined using 1-way ANOVA followed by a Tukey’s test. Lowercase letters indicate statistical differences between different treatments (P < 0.05). Experiments were done twice with similar results. G) Confocal images of 4 h gravistimulated PIN2pro:PIN2-GFP/eir1-4 root tips after mock, GLV6p, or GLV11p treatment. Arrows indicate the direction of gravity after gravistimulation. Arrowheads indicate LRC cells with high PIN2 level on PM; 100 nM GLVp was used. Bars represent 20 μm. H) Quantification of the PIN2-GFP signal ratio between left and right (0 h) or lower and upper (4 h) sides in LRC cells. Log transformation was used after the ratio quantification. Significant differences were determined using a student's t-test. *P < 0.05. Experiments were done in 2 replicates with similar results. I) Quantification of the PIN2-GFP signal ratio between left and right (0 h) or lower and upper (2 and 4 h) sides in LRC cells and epidermal cells with no peptide (Mock), 100 nM GLV6p, or 100 nM GLV11p treatment. Log-transformation was used after the ratio quantification. Individual data points for each seedling are shown. Significant differences were determined using 1-way ANOVA followed by a Tukey’s test. *P < 0.05; **P < 0.01; ***P < 0.001; and ns indicates no significant difference (P < 0.05). Experiments were done in 2 replicates with similar results. For boxplots in C), F), H), and I), boxes indicate ranges from the first to third quartiles, and the central lines display the median. Upper and lower whiskers extend to maximum or minimum values no further than 1.5 times IQR (interquartile range, the distance between the first and third quartiles). WT, wild type; Epi, epidermis; Cor, cortex.
Auxin distribution in the root tip is largely determined by auxin transporters. Thus, we introduced several PIN-GFPs, as well as AUX1-YFP, translational fusions into the rgi125 background by crossing. No difference was observed for AUX1-YFP or PIN1 between the mutant and the wild type (Supplemental Fig. S9, A and B). We observed a small increase in PIN3-GFP signal in rgi125, while PIN3-GFP showed an asymmetric distribution upon 0.5-h gravistimulation similar to that observed in the wild-type root (Supplemental Fig. S9, C–E). These results indicate that the reduced root gravitropism in rgi125 is most likely not due to a defective PIN3 relocalization and suggest that gravity sensing and early signal transduction are not affected in GLV signaling mutants.
Detailed analysis of the PIN2-GFP signal revealed a small but significant reduction in rgi125 epidermal cells (Fig. 4, B and C). Strikingly, a clear decrease in the PIN2-GFP signal was observed in rgi125 LRC cells, which was further confirmed by PIN2 immunolocalization assays (Fig. 4, D–F). Similar to the rgi125 mutant, the PIN2–GFP signal was also strongly decreased in the LRC and reduced to some extent in epidermal cells of the tpst-1 mutant (Fig. 4, D and F). We tested whether the PIN2-GFP signal in tpst-1 could be restored by exogenously supplying different sulfonated synthetic GLV peptides. The addition of GLV10p and GLV11p, but not GLV3p, GLV5p, and GLV6p, partially complemented the LRC PIN2-GFP signal in tpst-1 (Fig. 4, D and F). This complementation was dose-dependent and 50 nM of GLV11p appeared to be sufficient (Supplemental Fig. S10A). Interestingly, GLV3p and GLV6p fully complemented the epidermal PIN2 PM signal, while only partial complementation was obtained with the other peptides (Fig. 4F), probably reflecting different affinities of GLVp for the RGI receptors in different cell types. In contrast to the tpst-1 mutant, the PIN2-GFP signal at the PM could not be restored in the rgi125 mutant (Fig. 4, D and F). Together, these results indicate that GLV-RGI signaling is important to maintain PIN2 PM abundance, especially in LRC cells.
PIN2 is asymmetrically distributed during gravitropism accumulating at the lower root side to facilitate auxin fluxes (Sato et al. 2015). This PIN2 asymmetry is commonly and most evidently quantified in epidermal and cortical cells (Abas et al. 2006; Kleine-Vehn et al. 2008; Baster et al. 2013; Paris et al. 2018; Retzer et al. 2019; Marques-Bueno et al. 2021). While our data suggest RGI5 to function mainly in the LRC, we focused on this tissue and confirmed asymmetry (lower versus upper side of the root) of PIN2 also in the LRC (Fig. 4, G and I). Next, to study how GLV signaling affects PIN2 distribution during the root gravitropic response, we examined PIN2 distribution when GLV signaling was impaired. However, because the rgi125 mutant showed a very weak PIN2-GFP signal in LRC, we quantified PIN2-GFP in the rgi5-1 single mutant. PIN2-GFP still accumulated in the LRC at the lower side of the root, but the asymmetry seemed to be less pronounced when compared with that in the wild type (Fig. 4H). Furthermore, we tested whether exogenous GLV peptide treatment could interfere with PIN2 distribution during gravitropism. In agreement with our complementation experiments, GLV6p treatment disrupted PIN2 asymmetry in epidermal cells but not in the LRC, while GLV11p treatment disturbed PIN2 asymmetry in the LRC and delayed it in epidermal cells (Fig. 4, G and I). These data show that balanced GLV signaling contributes to asymmetric PIN2 stabilization during gravitropic responses.
GLV-RGI signaling regulates PIN2 phosphorylation levels
The level of PIN2 polarity can be valued by determining the ratio of apical-to-lateral or basal-to-lateral PIN2-GFP signals (Glanc et al. 2018). Reduction in apical-to-lateral PIN2-GFP signal ratio was found in epidermal cells of rgi125 (Fig. 4B; Supplemental Fig. S10B), suggesting that PIN2 polarity was less pronounced in this mutant. PIN2 is constantly recycled between the PM and endomembrane compartments and its vesicle trafficking back to PM can be disturbed by Brefeldin A (BFA) treatment. Both GLV6p and GLV11p treatment inhibited the formation of PIN2 BFA bodies. Moreover, this effect was not observed in the rgi125 mutant, which itself showed an increase in PIN2 BFA bodies (Supplemental Fig. S10, C and D). A similar result was observed when GLV11p and co-treatments with the translation inhibitor cycloheximide (CHX) were performed (Supplemental Fig. S10, E and F). These data indicate that GLV signaling is involved in the regulation of PIN2 cycling likely affecting both PIN2 abundance and polarity at the PM.
PIN2 phosphorylation regulates PIN2 polarity, subcellular dynamics, and activity (Kleine-Vehn et al. 2009; Zhang et al. 2010; Barbosa et al. 2018; Han et al. 2021). The observed changes in PIN2 abundance, polarity, and trafficking upon altered GLV signaling prompted us to examine if GLV signaling regulates PIN2 phosphorylation levels. Therefore, we examined PIN2-GFP migration as an indication of phosphorylated and unphosphorylated PIN2 abundance in microsomal fractions in the presence and absence of GLV11p using the PIN2pro:PIN2-GFP/eir1-4 line. We observed a prominent increase in the proportion of phosphorylated PIN2-GFP after GLV11p treatment (Fig. 5, A and B). To further verify whether the intensity of the differential band corresponded to increased GLV-mediated PIN2-GFP phosphorylation, we performed a phosphatase treatment of the samples which resulted in a strong reduction in the upper PIN2-GFP band (Supplemental Fig. S10G). This result further confirmed that the differences observed in PIN2-GFP migration could be attributed to different phosphorylation states between peptide-treated and peptide-untreated samples. In addition, we treated wild-type and rgi125 mutant with GLV11p and detected PIN2 in microsomal fractions with an anti-PIN2 antibody using a Phos-tag gel. Again, we found that the proportion of phosphorylated PIN2 was increased after GLV11p treatment in wild-type roots and this increase appeared to be suppressed in the rgi125 mutant background (Fig. 5, C and D).
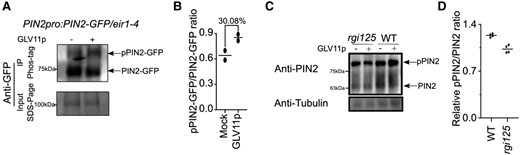
GLV-RGI signaling regulates PIN2 phosphorylation levels. A) GLV11p treatment increased the proportion of phosphorylated PIN2-GFP (pPIN2-GFP). PIN2-GFP from microsomal fraction of PIN2pro:PIN2-GFP/eir1-4 root tips treated with or without 100 nM GLV11p was immunoprecipitated and separated in a Phos-tag gel, then analyzed by Western blot with anti-GFP antibody. The IP input was separated onto an SDS-PAGE gel and PIN2-GFP protein was detected by anti-GFP antibody. Experiments were done in 2 replicates with similar results. B) Quantification of pPIN2-GFP/PIN2-GFP band intensity ratio in Western blot with Phos-tag gel. Individual data points for each experiment are shown. C) GLV11p treatment increased the proportion of phosphorylated PIN2 (pPIN2) in the wild type, but not in rgi125. Protein in the microsomal fraction from rgi125 and wild-type root tips treated with or without 100 nM GLV11p was separated in a Phos-tag gel and analyzed by Western blot with anti-PIN2 antibody. Anti-tubulin antibody was used as the loading control. Experiments were done in 4 replicates with similar results. D) Quantification of relative pPIN2/PIN2 ratio change after GLV11p treatment. Individual data points for each experiment are shown. WT, wild type.
Taken together, the physiological, microscopic, and biochemical data collectively suggest that fine-tuned GLV-RGI signaling modulates root gravitropism by regulating PIN2-dependent auxin transport mainly in LRC cells, presumably by regulating PIN2 phosphorylation status.
Discussion
GLV peptides were previously shown to function in RAM homeostasis and gravitropism (Matsuzaki et al. 2010; Whitford et al. 2012; Fernandez et al. 2013) but it was not clear whether both functions were entirely linked or represented 2 independent GLV-mediated processes. Here, we showed that both processes depend on RGI receptors but take place in different cells of the RAM. GLV signaling in the LRC is in agreement with the established function of this cell layer in gravitropic responses (Swarup et al. 2005; Su et al. 2017).
Strikingly, we observed that the RGI5 receptor is expressed in the LRC and asymmetrically distributed in this tissue during root gravistimulation. Kinematic analysis revealed that asymmetric auxin distribution preceded the RGI5 expression asymmetry, suggesting that the unequal auxin distribution between the upper and lower sides of the root is instructive for the asymmetric RGI5 distribution. However, at the protein level, RGI5 asymmetry was detected ahead of asymmetric expression of RGI5, and our data indicated that the RGI5 asymmetry could not be disturbed by pharmacologically interfering with auxin distribution in the root tip. Together, the RGI5 asymmetry seems to be regulated by gravity perception but was not the direct consequence of the asymmetric auxin distribution in the root tip upon gravistimulation. How this asymmetry is formed in the process of gravitropism remains unknown. One possibility is that a secondary messenger downstream of gravity sensing would affect RGI5 protein stability. Recent studies revealed unequal cytosolic calcium ion (Ca2+) signaling, extracellular PH changes, nitric oxide, and reactive oxygen species (ROS) distributions in the root upon gravistimulation (Monshausen et al. 2011; Krieger et al. 2016; Paris et al. 2018; Zhao et al. 2022). PHOSPHOGLUCOMUTASE (PGM) controls starch biosynthesis in columella cells and the pgm-1 mutant exhibits reduced root gravitropism (Caspar and Pickard 1989). The LAZY1-LIKE (LZY) and RCC1-like domain (RLD) proteins are other factors functioning downstream of amyloplast sedimentation that play a role in the early steps of gravity signaling (Taniguchi et al. 2017; Yoshihara and Spalding 2017; Furutani et al. 2020). In addition, ALTERED RESPONSE TO GRAVITY1 (ARG1) and ARG1-LIKE2 (ARL2) were found to act at the early steps of gravity sensing and signal transduction in a distinct pathway involving amyloplast sedimentation and also affect PIN3 relocalization upon gravistimulation (Guan et al. 2003; Harrison and Masson 2008). Thus, some of these components could be potentially involved in the establishment of RGI5 asymmetry, and their interconnection with the GLV pathway remains to be studied. Comparative analysis of RGI5 distribution between wild type and mutants in the aforementioned pathways such as the starchless mutant pgm, the lzy mutants, and arg1-2 mar2-1 mutant would offer more information to understand the mechanism that regulates RGI5 asymmetry.
Our data indicate that GLV signaling is likely not acting at the level of gravity sensing in columella cells since PIN3-GFP asymmetry was not affected in the rgi125 mutant. We noticed a slightly enhanced PIN3-GFP signal and potentially increased starch granules in rgi125 columella cells. However, this could be a secondary consequence of reduced ROS due to a GLV-RGI defect or auxin accumulation in columella cells (due to a defect in auxin transport though LRC) rather than the cause of the observed root gravitropic defect (Pasternak et al. 2005; Blomster et al. 2011; Yamada et al. 2020). Auxin was shown to regulate starch biosynthesis and a previous report showed that the pin2 mutant exhibited higher auxin accumulation and increased starch granule accumulation in columella cells, which is in agreement with this hypothesis (Zhang et al. 2019).
In agreement with previous results (Whitford et al. 2012), our data corroborate that GLV-RGI signaling plays an important role in regulating PIN2 abundance at the PM. More importantly, by using lof mutants, not available at the time of the previous study, our analysis revealed a major function for this pathway in the LRC rather than the epidermis, although a minor effect in epidermal cells was also observed. The (partial) complementation of PIN2 abundance in the tpst-1 mutant by the addition of sulfonated GLV10p and GLV11p at the nanomolar concentrations corresponds with the measured binding affinity of GLV11p/RGF1p to the RGI receptors (Song et al. 2016). Since supplementing GLVp to the medium only partially complemented PIN2 levels in the tpst-1 mutant, additional sulfonated molecules (possibly other peptides) may also be required. The use of lof mutants also demonstrated that GLV-RGI signaling is needed to keep a critical level of PIN2 at PM in LRC cells which is likely necessary for rapid and sharp gravitropic responses.
The dynamics of PIN proteins are known to be highly regulated by phosphorylation and for now, many PIN phosphorylation regulators have been characterized. The D6 PROTEIN KINASE, PINOID, and WAG1 and WAG2 directly phosphorylate PIN2 at serine 222 (S222), S237, S258, and S310 to activate PIN2 auxin transport activity (Zourelidou et al. 2014). Also, PINOID/WAG1/WAG2 controls PIN2 apical polar localization by regulating PIN2 phosphorylation (Dhonukshe et al. 2010). MAP KINASE KINASE 7 (MKK7) and its downstream target kinases MITOGEN-ACTIVATED PROTEIN KINASE3 (MPK3) and MPK6 phosphorylate PIN1 to control PIN1 polarity (Jia et al. 2016). MKK7-mediated PIN1 phosphorylation sites are also conserved in PIN2 at threonine 233 (T233), T254, and T306. Membrane-localized proteins are also found to regulate PIN2. CDPK-RELATED KINASE5 (CRK5) directly phosphorylates PIN2 and regulates PIN2 abundance on PM to control its polarity and trafficking. Accordingly, the crk5-1 mutant exhibited reduced PIN2 abundance and changed PIN2 polarity in epidermal and cortical cells, thus disturbing root gravitropism (Rigo et al. 2013). The CRK5-related PIN2 phosphorylation sites are not known yet, but CRK5 has been shown to phosphorylate PIN1, PIN4, and PIN7 at the sites that are also conserved in PIN2 (S258, S259, S301, and S302) (Baba et al. 2019). CANALIZATION-RELATED AUXIN-REGULATED MALECTIN-TYPE RLK (CAMEL) was reported to phosphorylate PIN1 to regulate PIN1 trafficking and polarity at sites that are also conserved in PIN2 (T192, T240, S246, T263, and S419) (Hajny et al. 2020). Activated TMK1 phosphorylates MARK2 which then releases from TMK1 to cytosol and negatively regulates ROP6 to control PIN2 dynamic distribution upon gravitropism (Marques-Bueno et al. 2021). Our data show that GLV-RGI signaling controls PIN2 PM abundance, likely by upregulating PIN2 phosphorylation although the identity of GLV-mediated PIN2 phosphorylation sites needs further investigation. One possibility is that RGI receptors, upon GLV perception, directly phosphorylate PIN2. The other possibility is that RGI receptors indirectly regulate PIN2 phosphorylation via one of the factors mentioned above. For example, MPK6 is a known downstream effector of the GLV pathway (Fernandez et al. 2020; Lu et al. 2020; Shao et al. 2020) and may phosphorylate PIN2 when GLV-RGI signaling is activated.
The positive GLV-RGI regulation of PIN2 PM abundance and the observed RGI5 asymmetry coinciding with PIN2 accumulation is in line with a model in which asymmetric GLV-RGI signaling is needed to maintain PIN2 PM levels and auxin transport up to the elongation zone at the concave root side during root directional growth. Such a temporal endorsement of auxin efflux into the elongation zone might be required to obtain well-balanced levels of auxin and the appropriate degree of cell elongation in the elongation zone at the lower side of the root. This hypothesis is supported by the exaggerated bending resulting in root curling when GLV signaling is experimentally boosted such as in GLV overexpression lines and upon GLV peptide treatment and, vice versa, by the reduced root waving and gravitropic response in mutants with reduced GLV signaling such as tpst-1and rgi125.
In summary, we hypothesize the existence of a peptide receptor signaling in the LRC that contributes to an appropriate degree of bending during root growth. Important questions, however, remain unanswered and it will be challenging to determine the driving factor behind the RGI5 protein asymmetry as well as to identify which cells in the RAM, by secreting GLV peptides, are triggering RGI signaling in the LRC.
Materials and methods
Growing conditions and plant material
Arabidopsis (Arabidopsis thaliana) Col 0 seedlings were sown on solid ½ MS medium (Duchefa Biochemie B.V.) containing 1% sucrose (VWR), 0.1 g l−1 Myo-inositol (Sigma-Aldrich), 0.5 g l−1 2-(N-morpholino) ethanesulfonic acid (MES) (Duchefa Biochemie B.V.), and 1.0% Plant Tissue Culture Agar (Lab M, MC029). Seeds were stratified for at least 2 d at 4°C and plants were grown at 21°C under continuous light conditions.
Transcriptional reporters for RGI1 and RGI5 (RGIpro:NLS-2XGFP) were previously reported (Fernandez et al. 2020). The RGI2pro-erGFP (also called proRCH1-erGFP) was previously described and kindly donated by Prof. Reinze Heidstra (Santuari et al. 2016). To generate the RGI5pro:RGI5-GFP or Venus construct, the RGI5 coding sequence was PCR amplified from root cDNA with flanking attB1 and B2 recombination sites and cloned into pDONR221 to generate pEN-L1-RGI5-L2. The previously described pEN-L4-RGI5pro-R1 entry clone (Fernandez et al. 2020), pEN-L1-RGI5-L2 and pEN-R2-F-L3 (Karimi et al. 2007), or pEN-R2-Venus-L3 (Mylle et al. 2013) were recombined into the pB7m34GW vector using LR recombination reaction (Invitrogen) to generate the RGI5pro:RGI5-GFP or Venus constructs. These were transformed into the rgi5-1 mutant by floral dipping. To generate RGI1pro:RGI1-GFP, the genomic RGI1 sequence (from ATG to stop) was amplified and cloned into pDONR221. The resulting entry clone was recombined with pEN-L4-RGI1pro-R1 (Fernandez et al. 2020), pEN-R2-F-L3, and pB7m34GW. RGI1pro:RGI1-GFP was transformed into the rgi1-1 mutant.
The SMB promoter (3,071 bp) in pDONR P4P1r was kindly donated by Prof. Moritz Nowack. The pEN-L4-RPS5A-R1, containing the RPS5A promoter (1,689 bp before ATG), and the pEN-L1-RGI1-R2, containing the RGI1 coding sequence, entry clones have been previously described (Fernandez et al. 2020). pEN-L4-RPS5Apro-R1 or pEN-L4-SMBpro-R1 were recombined with pEN-L1-RGI1-L2 and pEN-R2-Venus-L3 in the pB7m34GW-FAST vector by LR recombination reaction (Invitrogen) to generate the RPS5Apro:/SMBpro:RGI1-Venus cassette that was transformed into the iGLV6/rgi1 rgi5 or rgi125 lines. GFP-positive T2 seeds were selected with Leica-GFP bino and were used for RAM size measurement or gravitropic bending assay.
The rgi1-1 (Salk_020659), rgi1-2 (Salk_040393), rgi5-1 (Salk_058918), rgi5-2 (Salk_014726), and tpst-1 (SALK_009847) mutant lines as well as the iGLV6 line were previously reported (Fernandez et al. 2020). The rgi2-1 (Salk_038309) (Song et al. 2016) rgi2-2 (Salk_096206) (Shinohara et al. 2016) lines have been previously described and were ordered from the Nottingham Arabidopsis Stock Center (NASC). The PIN2pro:PIN2-GFP/eir1-4 (Xu and Scheres 2005), PIN3pro:PIN3-GFP/pin3-2 (Zadnikova et al. 2010), AUX1pro:AUX1-YFP/aux1-22 (Swarup et al. 2004), and BRI1pro::BRI1-mCitrine/bir1 (Jaillais et al. 2011) transgenic lines and eir1-1 (Luschnig et al. 1998) mutant have been previously described. The rgi1 rgi5, rgi2 rgi5, rgi125, iGLV6/rgi1 rgi5, DR5pro:GFP/rgi5-1, DR5pro:GFP/rgi125, PIN2pro:PIN2-GFP/rgi5-1, PIN2pro:PIN2-GFP/rgi125, PIN2pro:PIN2-GFP/tpst-1, PIN3pro:PIN3-GFP/rgi125, AUX1pro:AUX1-YFP/rgi125, and RGI5pro:RGI5-GFP/rgi5-1/eir1-1 lines were obtained by crossing.
Peptide treatments
GLV3p: RDD(SO3)YPIYSKPRRKPPVNN, GLV5p: DY(SO3)WKPRHHP(HYP)KNN, GLV6p: DY(SO3)RTFRRRRPVHN, GLV10p DY(SO3)PKPSTRPPRHN, and GLV11p: D(SO3)YSNPGHHPPRHN were synthesized from a commercial provider (GenScript; http://www.genscript.com/). Peptides were dissolved in potassium phosphate buffer (PH = 5.7) and added to a medium at the indicated concentrations.
Phenotypic analysis
Seedlings at 4 d after germination (dag) vertically grown on medium supplemented or not with peptides or estradiol were quickly pulled up by the cotyledons to align the root with the vertical, then rotated 90 degrees, and were kept in dark. For gravitropism assays, plates were scanned after 6 h gravitropism response. For kinetic analysis of gravitropic bending angles, plates were scanned every hour after gravistimulation. The bending angle was measured with ImageJ. Plots were generated with https://rmtrane.shinyapps.io/RootNav/.
Confocal microscopy
All confocal imaging was performed with a Zeiss 710 confocal microscope except time-lapse imaging after 2 consecutive gravistimulations for Fig. 2F and Supplemental Movie S3 which were performed with a Zeiss 900 confocal microscope setup to image vertical root growth. To measure RAM size, the cell wall from 5 dag roots was stained with propidium iodide (PI). To image starch in the root, mPS-PI staining was performed as described (Truernit et al. 2008). For cell length measurement at the beginning of the root differentiation zone, the ClearSee protocol was performed in 5 dag seedlings as previously described (Kurihara et al. 2015), and calcofluor white (Sigma) was used for cell wall staining. To measure cell elongation after gravistimulation, 4 dag roots were rotated 90 degrees for 3 h. Cell walls were stained by adding 45 μg μl−1 PI solution to the plates. After 3 min, samples were transferred to a growth chamber and imaged. The following settings were used to image fluorophores: GFP, 488 nm laser for excitation and 490 to 550 nm for detection; Venus, 514 nm laser for excitation and 519 to 543 nm for detection; mCitrine, 514 nm laser for excitation and 520 to 621 nm for detection; PI, 514 nm laser for excitation and 566 to 719 nm filter for detection; calcofluor white, 405 nm laser for excitation and 410 to 524 nm filter for detection. The 488 and 514 nm lasers were used at 20% laser power and the 405 laser was used at 10% laser power.
The PIN2-GFP signal was quantified in the PM of the LRC or epidermal cells in median confocal images using ImageJ (5 to 12 cells per root were used). Z-stacks were obtained to generate 3D projections. To determine the percentage of PIN2 on the PM, the GFP signal in the PM was divided by the total GFP signal (PM + intracellular signal). To measure PIN2 polarity index in epidermal cells, the apical GFP signal in the PM was divided by the lateral GFP signal. For peptide complementation experiments, PIN2pro:PIN2-GFP/wt, PIN2pro:PIN2-GFP/tpst-1, and PIN2pro:PIN2-GFP/rgi125 seeds were germinated on solid MS medium for 6 d then transferred to peptide-free medium or medium containing 100 nM of the indicated GLV peptide. After 48-h treatment, samples were imaged. Similarly, PIN2pro:PIN2-GFP/tpst-1 seedlings were transferred to a medium supplemented with GLV11p at different concentrations as indicated. To quantify LRC PIN2 distribution in rgi5-1 background, 6 dag PIN2pro:PIN2-GFP/wt and PIN2pro:PIN2-GFP/rgi5-1 were imaged at 0 and 4 h post-gravistimulation. To determine PIN2 distribution with GLV peptide treatment after gravistimulation, 6 dag PIN2pro:PIN2-GFP/eir1-4 seedlings were transferred from ½ MS plates to ½ MS plates with 100 nM GLV6p, 100 nM GLV11p, or no peptide and were rotated 90 degrees after 1 h treatment. Roots were imaged 0, 2, and 4 h after gravistimulation. To detect BFA bodies, 6 dag PIN2pro:PIN2-GFP/wt or PIN2pro:PIN2-GFP/rgi125 seedlings were transferred from ½ MS plates to liquid ½ MS containing no peptide, 100 nM GLV6p, or GLV11p for 1 h. About 25 μM BFA were added and samples were imaged after 30 min. To eliminate newly synthesized PIN2 accumulation in BFA bodies, PIN2pro:PIN2-GFP/eir1-4 seedlings were transferred to liquid ½ MS containing 50 μM CHX for 1 h treatment. After CHX pretreatment, no peptide or 100 nM treatment was added for 1 h followed by 25 μM BFA treatment for half an hour before imaging. Average PIN2 BFA bodies were quantified from 8 to 15 cells per root.
To image RGI5-GFP asymmetry during root waving, 5 dag vertically grown RGI5pro:RGI5-GFP/rgi5-1 or pBRI1-BRI1-mCitrine/bir1 seedlings were transferred into an imaging chamber (Thermo Scientific) and kept slanted 45 degrees. After 12 h acclimatization, samples were imaged every hour for up to 24 h.
To determine RGI5-GFP asymmetry after gravistimulation, 6 dag vertically grown RGI5pro:RGI5-GFP/rgi5-1 or RGI5pro:RGI5-Venus/rgi5-1 seedlings were rotated 90 degrees and roots were imaged 0, 2, and 4 h after gravistimulation. GFP/Venus fluorescent intensity in the LRC was measured with ImageJ in a similar area at the lower and upper sides of the root and the lower/upper GFP signal ratio was calculated. In non-gravistimulated seedlings used as control, a similar measurement was performed on the left and right sides of the root. RGI1-Venus distribution in SMBpro:RGI1-Venus/rgi1 rgi5 was determined in a similar way. To determine RGI5-GFP distribution upon gravitropism, 4 dag RGI5pro:RGI5-GFP/rgi5-1 or RGI5pro:RGI5-GFP/rgi5-1/eir1-1 roots were aligned with the vertical. After 1 h, plates were rotated 90 degrees and roots were imaged at 0 and 4 h after gravistimulation. To measure the kinetics of auxin distribution, RGI5 protein distribution, and RGI5 transcriptional changes upon gravitropism, 6 dag vertically grown DII-Venus, RGI5pro:RGI5-GFP/rgi5-1, and RGI5pro:NLS-2XGFP seedlings were rotated 90 degrees and plates were imaged at each time point. For NAA and NPA treatments, seedlings were sprayed with liquid ½ MS (same composition as before without agar) supplemented with 10 nM 1-NAA, 10 nM NPA, or an equal volume of DMSO and incubated for 1 h before gravistimulation.
To quantify the DR5pro:GFP asymmetry, 6 dag DR5pro:GFP seedlings in the wild type, rgi5-1, and rgi125 mutant background were processed as described above and imaged every hour after gravistimulation. The lower/upper GFP signal ratio was calculated by measuring the GFP signal with ImageJ in the LRC cells above the QC.
To quantify the PIN3-GFP asymmetry, 4 dag PIN3pro:PIN3-GFP/wt and PIN3pro:PIN3-GFP/rgi125 seedlings were imaged at 0 and 0.5 h gravistimulation. The lower/upper GFP signal ratio was calculated by measuring the GFP signal with ImageJ at lower outer and upper outer columella cells as described by Peter Grones (Grones et al. 2018).
Immunolocalization assays
To detect PIN1 or PIN2 by immunolocalization, 4 dag seedlings were harvested and immunolocalization was performed as previously described (Karampelias et al. 2018) with goat anti-PIN1 (1:600) or rabbit anti-PIN2 (1:600) as primary antibodies and AlexaFluor488 donkey anti-goat (1:600) (A-11055, ThermoFisher) or AlexaFluor555 donkey anti-rabbit (1:600) (A-31572, ThermoFisher) as secondary antibodies, respectively. AlexaFluor488 was imaged with a 488-nm laser for excitation and 525 to 580 nm for detection. AlexaFluor555 was imaged using a 561-nm laser for excitation and 580 to 680 nm for detection.
PIN2 phosphorylation assays
To determine the phosphorylation level of PM PIN2 or PIN2-GFP protein, roots tips from 6 dag wild type and rgi125 seedlings or PIN2pro:PIN2-GFP/eir1-4 seedlings were harvested after 8 h mock or 100 nM GLV11p treatment. Microsomal extraction was performed according to Abas and Luschnig (2010), with modifications. Roots were ground and 100 μl of extraction buffer (EB) [Tris-HCl (pH = 7.5, 55 mM), D-sorbitol (27.5% W/V), sodium fluoride (NaF, 55 mM), sodium molybdate (Na2MoO4, 1.1 mM), cOmplete Protease Inhibitor Cocktail (Sigma-Aldrich), PhosStop (Roche), and 1 mM phenylmethylsulfonyl fluoride (PMSF)] was added per milligram of tissue. After vortexing, samples were transferred into tubes with insoluble polyvinylpolypyrrolidone (PVPP, 50 mg per gram of tissue) that had been previously equilibrated with EB. After 5-min incubation on ice with intermittent vortexing, samples were centrifuged at 600 × g for 3 min at 4°C. The supernatant was transferred to another tube and the pellet was extracted again twice with half and one-third of the initial EB volume, respectively. All supernatants were pooled. Finally, the pellet was centrifuged at 2,000 × g for 30 s at 4°C, and the supernatant was added to the previous supernatant pool, mixed by vortex, and centrifuged at 600 × g for 3 min at 4°C. Then, the supernatant was transferred to a new tube, mixed with an equal volume of water, and transferred into different tubes (100 μl each). After being centrifuged at 21,000 × g for 2 h at 4°C, the supernatant was discarded and the microsomal pellet was washed with 150 μl wash buffer [Tris-HCl (pH = 7.5, 20 mM) and PMSF (1 mM)] and centrifuged at 21,000 × g for 45 min at 4°C. For PIN2 phosphorylation analysis in wild type and rgi125, the pellet was resuspended in 1.5 μl of sample buffer (Laemmli Sample Buffer, Biorad) per initial milligram of tissue, then centrifuged at 21,000 × g for 30 min at 4°C, and the supernatant (equivalent to 10 mg starting material) was loaded into Phos-tag precast gels (FUJIFILM Waco Chemicals). For PIN2-GFP phosphorylation analysis, the microsomal pellet was resuspended in buffer containing Tris-HCl (PH = 7.5, 10 mM), sodium chloride (NaCl, 150 mM), glycerol (10% V/V), Triton X-100 (1% V/V), NaF (20 mM), cOmplete Protease Inhibitor Cocktail, PhosStop, and PMSF (1 mM). Samples were centrifuged at 21,000 × g for 30 min at 4°C and protein concentration was measured with Qubit protein assay kit (Thermo Fischer Scientific) in the supernatant. PIN2-GFP was immunoprecipitated with GFP-trap magnetic agarose beads (gtma, Chromotek) following the manufacturer’s instructions. For phosphatase treatment, PIN2-GFP proteins bound to GFP-trap beads were washed with 500 μl beads washing buffer [Tris-HCL (PH = 7.5, 10 mM) and NaCl (150 mM)] 4 times and then were treated with reaction buffer containing 1 μl (400 U) of Lambda Protein Phosphatase (Lambda PP, NEB) for 15 min at 30 degrees. As a control, samples added to reaction buffer without Lambda PP were also treated for 15 min at 30 degrees. The reaction was stopped by washing the column with beads and washing buffer 4 times. Immunoprecipitates were loaded into Phos-tag precast gels and transferred to PVDF membranes.
PIN2 protein was detected with anti-PIN2 antibody (1:500) (Abas et al. 2006) followed by secondary horseradish peroxidase (HRP)-conjugated anti-rabbit antibody (1:10,000, NA934V; GE Healthcare). Anti-Tubulin (1:10,000, T5168-100UL; Sigma-Aldrich) was used as loading control followed by secondary HRP-conjugated anti-mouse antibody (1:10,000, NA931; GE Healthcare). PIN2-GFP protein was detected with anti-GFP antibody (1:5,000, ab290; Abcam) followed by secondary HRP-conjugated anti-rabbit antibody (1:10,000, NA934V; GE Healthcare). Quantification of protein signaling was performed using ImageJ software.
Statistical analysis
Comparisons of RAM size and fluorescence intensities between genotypes and/or treatments were performed via 1-way ANOVA followed by a Tukey's test, or 2-way ANOVA followed by a Šidák test. To compare the fluorescent intensity ratio during gravitropism, a student's t-test was performed on the log2-transformed ratio value for pairwise comparisons, and a 1-way ANOVA followed by a Tukey's test was performed on the log2-transformed ratio value in case of multiple comparisons. To compare the root bending angle during gravitropism between different genotypes or treatments, a Kolmogorov–Smirnov test was performed for pairwise comparisons, or in case of multiple comparisons, a Kruskal–Wallis test and Dunn's test were performed. To compare the mean PIN2-GFP signal at the PM, and the average PIN2 BFA bodies per cell with CHX treatment, a student's t-test was performed. For the comparison of lower/upper DR5pro:GFP ratio between the wild type and rgi5-1 and wild type and rgi125 background, a student's t-test was performed on the log2-transformed ratio value.
Accession numbers
Sequence data from this article can be found in the Arabidopsis Information Resource (http://www.arabidopsis.org/) under accession numbers: GLV6 (AT2G03830), RGI1 (AT3G24240), RGI2 (AT5G48940), RGI5 (AT1G34110), BRI1 (At4g39400), TPST (AT1G08030), SMB (AT1G79580), RPS5A (AT3G11940), PIN1 (AT1G73590), PIN2 (AT5G57090), PIN3 (AT1G70940), and AUX1 (AT2G38120).
Acknowledgments
We thank Dr. Eugenia Russinova for providing the pBRI1-BRI1-mCitrine/bri1 seeds; Dr. Moritz Nowack for providing the pEN-L4-SMBpro-R1 plasmids; Dr. Melinda Abas for providing the eir1-1 mutant and the anti-PIN2 antibody; Ren Wang, Xiangyu Xu, Davy Opdenacker, and Brigitte Van De Cotte for their technical help; and Veronique Storme for her advice on statistical analyses.
Author contributions
A.I.F. and T.B. designed the experiments. K.X., J.J., X.X., and A.I.F. performed the experiments. K.X., J.J., X.X., and I.D.S. analyzed the data. K.X., A.I.F., and T.B. wrote the manuscript.
Supplemental data
The following materials are available in the online version of this article.
Supplemental Figure S1. Presence of statoliths and cell length analysis in the rgi125 mutant compared to the wild type.
Supplemental Figure S2. Expression of RGI1 and RGI2 in the root tip.
Supplemental Figure S3. RGI5 unequal distribution is detected when auxin asymmetry is disrupted during root gravitropism.
Supplemental Figure S4. RAM sizes in GLV signaling-defective mutants.
Supplemental Figure S5. GLV-RGI signaling in LRC is necessary for root gravitropism.
Supplemental Figure S6. RGI1-Venus controlled by lateral root cap-specific promoter shows asymmetry during root gravitropism.
Supplemental Figure S7. RGI1-Venus controlled by lateral root cap-specific promoter partially complemented root gravitropism defect in rgi125 but not RAM size.
Supplemental Figure S8. Reduced difference between upper and lower cortical cell lengths in rgi5-1 elongation zone upon gravistimulation.
Supplemental Figure S9. PIN1, AUX1, and PIN3 in rgi125 mutant.
Supplemental Figure S10. GLV signaling regulates PIN2.
Supplemental Movie S1. RGI5-GFP signal flipped from one side to the other of the LRC during root waving.
Supplemental Movie S2. BRI1-mCitrine signal distribution does not change during root waving.
Supplemental Movie S3. RGI5-GFP signal enriches at the lower side of the root after gravistimulation.
Funding
This research was supported by FWO postdoctoral and project grants (A.I.F., nos. 1293817N and 456 G020918N) as well as an FWO doctoral fellowship grant (J.J., no. 1168218N), and a China Scholarship Council grant (K.X., no. 201606350134).
Data availability
The author responsible for distribution of materials integral to the findings presented in this article in accordance with the policy described in the Instructions for Authors (https://dbpia.nl.go.kr/plphys/pages/general-instructions) is Tom Beeckman ([email protected]).
References
Author notes
Ana I Fernandez and Tom Beeckman contributed equally to this work.
Conflict of interest statement. The authours declare no conflicts of interest.