-
PDF
- Split View
-
Views
-
Cite
Cite
Eva Maleckova, Mixing it up: a chimeric tobacco-cyanobacterial photosystem II design for maximum low-light use efficiency, Plant Physiology, Volume 191, Issue 4, April 2023, Pages 2218–2220, https://doi.org/10.1093/plphys/kiad023
- Share Icon Share
Although the Green Revolution in plant breeding led to increases in food production up until the early 2000s, the benefits of such traditional methods of crop improvement have reached a plateau. Targeted engineering approaches offer a promising solution toward enhancing crop yields in the post-Green Revolution era (Maurino and Weber, 2013; Batista-Silva et al., 2020). Attempts to improve crop yields by the genetic manipulation of photosynthesis have previously been directed toward modifying the biochemical reactions of photosynthesis, including the engineering of ribulose-1,5-bisphosphate carboxylase-oxygenase (RuBisCO), the introduction of carbon concentrating mechanisms into C3 plants, and the creation of photorespiratory bypasses. The light reactions of photosynthesis have also been targeted by tweaking non-photochemical quenching (NPQ) and the cytochrome b6f complex, and by expanding the light absorption spectrum to capture more of the available light (summarized by Batista-Silva et al., 2020).
Oxygenic photosynthesis is the process of using solar energy to fix carbon dioxide (CO2) into carbohydrates while releasing oxygen as a byproduct. Photosystem II (PSII) is the site of primary charge separation but is also the most sensitive component of the photosynthetic reactions and, as such, is highly vulnerable to photodamage. Charge separation by PSII is a trade-off between photochemical productivity (water oxidation) and photoprotection (charge recombination) (Vinyard et al., 2014). Improved photoprotection thus offers another approach toward higher yields.
During evolution, phototrophic organisms developed two alternative strategies of photoprotection: algae and vascular plants developed protective mechanisms in the antenna, while prokaryotic phototrophs (cyanobacteria) evolved multiple isoforms of D1 protein, one of the PSII polypeptides (Murchie and Niyogi, 2011; Ort et al., 2015). Expression of a particular D1 isoform depends on environmental conditions, including light intensity and quality and temperature. In Synechococcus elongatus PCC 7942, both D1 isoforms are highly conserved at the protein level. In Chlamydomonas reinhardtii, two distinct amino acid residues determining high-light and low-light phenotypes have been identified (Vinyard et al., 2014).
In this issue of Plant Physiology, Zhang and colleagues investigated whether the D1 amino acid substitutions are conserved in vascular plants by re-creating previously identified substitutions at cognate positions in the D1 sequence of tobacco (Nicotiana tabacum) (Zhang et al., 2022). Because the chloroplast gene for QB protein (psbA) encoding the D1 protein is part of the plastid genome, targeted manipulations by homologous recombination are possible, and the authors generated three transplastomic, marker-free tobacco lines: a low-light isoform (LL), a high-light isoform (HL), and a control line transformed with a native psbA sequence.
LL and HL plants differed in their performance (expressed as dry weight) under several light regimes. Using controlled illumination, the performance of each line was determined in response to light intensity as well as to simulated diurnal cycling. In the presence of continuous illumination, all lines grew equally well at higher light intensities, while at low-light intensities, LL plants outperformed both HL and control lines. The better performance of LL plants under low-light conditions conforms with the enhanced growth reported for an equivalent cyanobacterial mutant under similar conditions (Sugiura et al., 2014). However, when diurnal cycling (specifically 12 h light/12 h dark) was introduced, LL plants accumulated the least biomass regardless of light intensity. The authors hypothesized that 12 h of low intensity illumination may be insufficient to sustain biomass repair and growth over the entire diurnal period.
In further studies of the observed phenotypes, the authors investigated several biophysical parameters, including PSII photochemical quantum yield, water oxidation turnover, sensitivity to photoinhibition and levels of NPQ. The maximum quantum yield (i.e. charge separation) of PSII was assessed via Fv/Fm measurement on dark-adapted leaves. The Fv/Fm parameter is a sensitive indicator of photosynthetic performance, with lower values indicating down regulation of photosynthesis or ongoing photoinhibition (Maxwell and Johnson, 2000). Fv/Fm was lower for the LL plants, which is indicative of fewer electrons entering linear electron flow and, thereby, decreasing the generation of ATP and reducing power (Gorbumov et al., 1999).
Water oxidation, as the only source of electrons for the electron transport chain, is a critical step of the photosynthetic reactions. The efficiency of water oxidation in the three lines was assessed indirectly by Fourier transformation of variable flash data and measured directly as oxygen evolution rate. After Fourier transformation, the highest peak amplitude was determined for LL plants, suggesting that a higher proportion of PSII is engaged in water oxidation in this line, an idea supported by direct measurements on isolated thylakoids under continuous illumination that revealed greater water oxidation rate per charge separation.
Photoinhibition is a result of cumulative damage to the D1 protein, and of the three lines examined, LL plants were the most susceptible to photoinhibitory light intensities (quantified as Fv/Fm after prolonged exposure to continuous high-light illumination). NPQ is one mechanism of protection from excess light, which is released as heat. While lower NPQ is advantageous under low light, insufficient NPQ capacity is detrimental to the photosynthetic machinery at higher illumination. LL plants were characterized by significantly lower NPQ levels, which explains the faster growth under low-light illumination.
The authors successfully expressed cyanobacteria-specific isoforms of D1 protein in tobacco chloroplasts, which demonstrates evolutionary preserved design of the PSII reaction center between prokaryotic ancestors and a model flowering plant. Under continuous low light, plants expressing an LL isoform of D1 protein grew better, which can be explained by less charge separation at the site of PSII, more efficient water oxidation, and less charge recombination. This however comes at the cost of limited photoprotection at high-light intensities (Figure 1). Although the expression of the LL D1 isoform improved growth under the specific artificial illumination, whether agriculture could indeed benefit from this strategy remains to be determined. Regardless, the work of Zhang et al. (2022) will serve as an inspiration to researchers interested in the targeted engineering of chloroplast genomes.
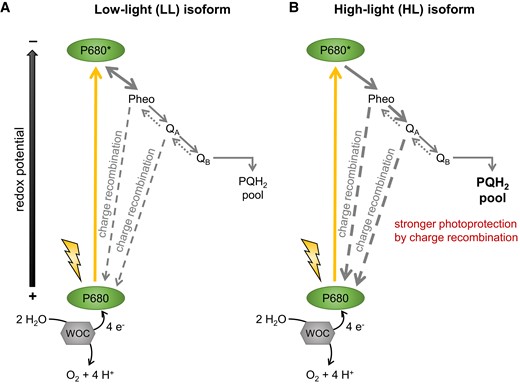
A scheme of photochemical reactions of photosystem II (PSII) and relative flux differences between low- and high-light isoforms of D1 protein. A, Expression of the low-light D1 isoform led to less charge separation by PSII, enabling more efficient water oxidation with less QA charge recombination. B, The high-light isoform of D1 protein enables more charge separation, which results in less efficient water oxidation, more charge recombination at QA and better photoprotection due to more charge recombination. Text size and line thickness indicate relative flux differences between both isoforms. P680, reaction center of photosystem II and its excited variant denotated as P680*; Pheo, pheophytin; PQH2, plastoquinol; QA, primary quinone; QB, primary quinone; WOC, water-oxidizing complex. Modified after Zhang et al. (2022).
References
Author notes
Conflict of interest statement. The author has no conflict of interest to declare.