-
PDF
- Split View
-
Views
-
Cite
Cite
Rosario Pantaleno, Denise Scuffi, Alex Costa, Elina Welchen, Roberta Torregrossa, Matthew Whiteman, Carlos García-Mata, Mitochondrial H2S donor AP39 induces stomatal closure by modulating guard cell mitochondrial activity, Plant Physiology, Volume 191, Issue 3, March 2023, Pages 2001–2011, https://doi.org/10.1093/plphys/kiac591
- Share Icon Share
Abstract
Hydrogen sulfide (H2S) is a gaseous signaling molecule involved in numerous physiological processes in plants, including gas exchange with the environment through the regulation of stomatal pore width. Guard cells (GCs) are pairs of specialized epidermal cells that delimit stomatal pores and have a higher mitochondrial density and metabolic activity than their neighboring cells. However, there is no clear evidence on the role of mitochondrial activity in stomatal closure induction. In this work, we showed that the mitochondrial-targeted H2S donor AP39 induces stomatal closure in a dose-dependent manner. Experiments using inhibitors of the mitochondrial electron transport chain (mETC) or insertional mutants in cytochrome c (CYTc) indicated that the activity of mitochondrial CYTc and/or complex IV are required for AP39-dependent stomatal closure. By using fluorescent probes and genetically encoded biosensors we reported that AP39 hyperpolarized the mitochondrial inner potential (Δψm) and increased cytosolic ATP, cytosolic hydrogen peroxide levels, and oxidation of the glutathione pool in GCs. These findings showed that mitochondrial-targeted H2S donors induce stomatal closure, modulate guard cell mETC activity, the cytosolic energetic and oxidative status, pointing to an interplay between mitochondrial H2S, mitochondrial activity, and stomatal closure.
Introduction
Most land plants have microscopic structures in the epidermal layer of leaves called stomata, consisting of a central pore surrounded by pairs of highly specialized cells, known as guard cells (GCs). Stomata are responsible for the gas exchange between the plant and the environment, controlling O2 release to the atmosphere, CO2 uptake needed for photosynthesis, and water loss through the transpiration stream (Lawson and Matthews, 2020). This tradeoff between CO2 uptake for photosynthesis, and the loss of water, is tightly controlled through the regulation of the stomatal pore width. This is possible due to the ability of GCs to perceive internal and external stimuli, which are then integrated and transduced via a complex signaling network (Hetherington and Woodward, 2003).
GCs have unique characteristics that distinguish them from their neighboring cells, including the presence of fewer and smaller chloroplasts (Wille and Lucas, 1984), a higher mitochondrial density (Willmer and Fricker, 1996), and respiratory rate (Araújo et al., 2011). These features point towards a key function of mitochondria in GC's physiological processes, and as a consequence, on stomatal movement regulation. The requirement of sucrose, starch and triacylglycerol breakdown during light-induced stomatal opening (Daloso et al., 2015; Horrer et al., 2016; McLachlan et al., 2016) highlights the role of mitochondrial metabolism in this response. In addition, it was recently shown that GC's mitochondria are the main source of cytosolic ATP, needed for stomatal opening (Lim et al., 2022). However, the role of mitochondrial activity during stomatal closure remains unexplored.
Hydrogen sulfide (H2S) is a low-weight gas endogenously synthesized in cells. It can freely diffuse across biological membranes and is considered very reactive at physiological pH and temperature conditions (Benchoam et al., 2019). H2S is the third member of the so-called “gasotransmitters” group (García-Mata and Lamattina, 2013), which also includes nitric oxide (NO) and carbon monoxide (CO). All of them share similar chemical properties and are considered relevant signaling molecules in organisms from different kingdoms (Aroca et al., 2020).
H2S participates in diverse cellular processes throughout plant's life cycle, such as germination (Baudouin et al., 2016), autophagy (Laureano-Marín et al., 2020), and stomatal closure regulation (García-Mata and Lamattina, 2010; Lisjak et al., 2010), among others. The biosynthesis of H2S occurs in different sub-cellular compartments including the cytosol, chloroplast, and mitochondria. Although H2S can participate in different types of reactions, latest reports suggest that its biological role is exerted trough persulfidation, an oxidative posttranslational modification in cysteine residues (Filipovic et al., 2018).
In animal experimental systems, mitochondrial H2S (mt-H2S) is reported to exert cytoprotective effects via the modulation of cellular ATP levels, as well as stimulating/preserving cellular bioenergetics (Szabo et al., 2014; Fox et al., 2021). The normal electron flow through the mitochondrial electron transport chain (mETC) is coupled to proton pumping, generating an electrical potential (Δψm) between both sides of the inner membrane, which is the driving force required for mitochondrial ATP synthesis by the ATP synthase complex (Braun, 2020). In prokaryotes and many metazoans including invertebrates, fish, birds, and mammals, H2S can donate electrons to the mETC (Olson and Straub, 2016). Moreover, in human cell cultures, H2S persulfidates the mitochondrial ATP synthase, increasing its activity (Módis et al., 2016); while the reduction of cytochrome c (CYTc), promotes O2 consumption (Vitvitsky et al., 2018). The use of mitochondrial-targeted H2S donors in animal systems has shown that H2S is able to restore membrane polarization, helping to preserve mitochondrial function (le Trionnaire et al., 2014; Fox et al., 2021).
On the other hand, despite advances in the knowledge of the mitochondrial H2S enzymatic source in Arabidopsis (Arabidopsis thaliana) (García et al., 2010), little is known about the effect of mt-H2S in plants. In this work, we studied the role of mt-H2S in GCs employing H2S donors targeted specifically to mitochondria. Results show that mt-H2S participates in stomatal closure induction through the modulation of mitochondrial activity in GCs.
Results
Mitochondrial H2S donors induce stomatal closure
Given the lack of information on the use of mitochondrial-targeted H2S in plants, as a first approach, we performed a dose–response assay in order to address whether mitochondrial H2S has any effect on stomatal movement. With that aim, we treated Arabidopsis thaliana epidermal peels (strips) with increasing concentrations of the mt-H2S donor AP39. Epidermal strips were incubated for 3 h in opening buffer (OB: 5 mM MES, 50 mM KCl, pH = 6.1) under white light, to open the stomatal pores, and then the strips were treated for 90 min, in the same OB, with increasing concentrations of AP39 (Figure 1A). The addition of the mt-H2S donor induced stomatal closure in a dose-dependent manner yielding a maximum effect, 30% reduction of the stomatal pore width, at a concentration of 0.1–1 μM AP39. As 100 nM was the minimal dose with the maximum effect, we selected it as the working concentration for the following assays (3.32 ± 0.84 μm and 2.35 ± 0.65 μm for mock and 100 nM AP39, respectively). Interestingly, the treatment with the master regulator of stomatal closure, abscisic acid (ABA), is reported to induce the same percentage of response (Scuffi et al., 2014). In addition, treating the epidermal strips with 100 µM of the widely used general H2S donor GYY4137 yielded a stomatal closure range comparable to that obtained with a concentration of AP39 2–3 orders of magnitude lower. To test if the mitochondrial donor was affecting GC viability, Arabidopsis epidermal strips were placed in OB and treated with 0.001–10 μM AP39 for 90 min, and then loaded with the viability fluorescent dye, Fluorescein diacetate (FDA). GC viability was quantified as the ratio between fluorescent GCs over total GCs. As observed in Figure 1B, the exposure to mt-H2S only compromised GC viability at the higher concentration assayed (10 μM), where the stomatal closure induction was suboptimal, discarding the possibility of a toxic effect of the donor molecule on stomata.
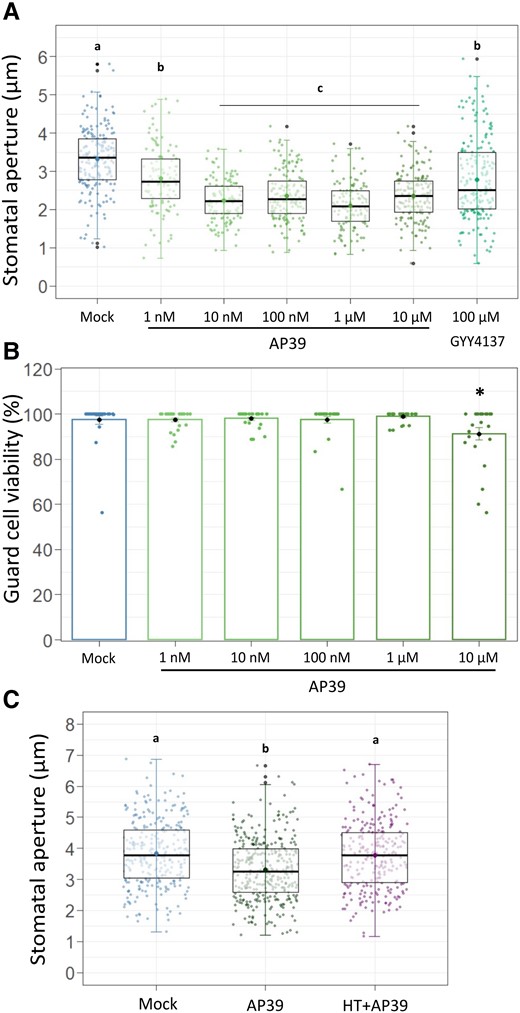
The mitochondrial H2S donor AP39 induces stomatal closure in a dose-dependent manner. Epidermal strips were excised from abaxial side from five to six weeks old Arabidopsis (Arabidopsis thaliana) leaves (ecotype Columbia -Col-0) and floated in opening buffer (OB: 5 mM MES, 50 mM KCl, pH = 6.1) for 3 h in the light. Then, they were treated for 90 min with Dimethyl sulfoxide (DMSO) 0.01% (v/v) (Mock), 100 µM of general H2S donor GYY4137, 100 nM AP39 and 200 µM of the H2S scavenger hypotaurine (HT), or with increasing concentrations of mitochondrial H2S donor AP39. Stomatal aperture was measured (A, C), or AP39-treated peels were subsequently loaded with the fluorescent dye FDA (B). Stomatal aperture values are expressed as absolute values in microns (µm) and are represented as points in the boxplots, where the box is bound by the 25th to 75th percentile (A). The line in the middle is the median, the darker point is the mean and whiskers span 10th to 90th percentile. The black points are outliers. The percentage of guard cell viability is the result of the ratio between fluorescent GCs and total GCs in the bright field (B). The bars represent the means, and the points are individual measurements. Error bars = Sd. Data are from at least three independent assays. Letters indicate statistical differences among treatments (Tukey's Method, confidence level = 0.95) A and C, Asterisks indicate statistical differences from the Mock (Dunnett's Method, P-value <0.05 = (*), confidence level = 0.95) (B).
In addition, we treated Arabidopsis peels with AP39 in the presence of the H2S scavenger hypotaurine (HT), or with two other mitochondrial-targeted H2S donors, AP123 and RT01 (Figure 1C, Supplemental Figure S1, A and B). The fact that HT impaired AP39-induced stomatal closure, and that both AP123 and RT01 showed a dose-dependent response comparable to that observed for AP39, indicate that stomatal closure was triggered by mitochondrial H2S. Finally, the same AP39 concentrations used for Arabidopsis stomatal closure assays were added to fava bean (Vicia faba) epidermal strips. As observed in Supplemental Figure S1C, Vicia epidermal strips also showed a dose-dependent stomatal response, indicating that the AP39 effect was not dependent on the plant species.
Mitochondrial ETC complex IV and/or cytochrome C activity are required for AP39-dependent stomatal closure
In human cellular cultures, mt-H2S donors were shown to modulate mitochondrial function through the interaction with different members of the mETC (Módis et al., 2016; Vitvitsky et al., 2018). Therefore, we sought to test whether GC mitochondrial activity is involved in mt-H2S-dependent stomatal closure induction. Following that purpose, Arabidopsis epidermal strips were treated with AP39, with or without the addition of the mETC inhibitors Rotenone (Rtn), 2-Thenoyltrifluoroacetone (TTFA), Antimycin A (AA) or Potassium cyanide (KCN) (inhibitors of the mETC complexes I, II, III, and IV, respectively) (Figure 2A). Stomatal pore width measurements showed that the exposure to the inhibitors of mETC complexes I to III had no effect on AP39-dependent stomatal closure, inducing a reduction of the stomatal pore size comparable to that obtained with AP39 alone (3.51 ± 1.42 μm–3.36 ± 1.19 μm, 3.26 ± 1.07 μm–3.39 ± 1.12 μm, 3.26 ± 1.07 μm–3.24 ± 1 μm for AP39 alone or in the presence of Rtn, TTFA, or AA, respectively) (Figure 2, B–D). Interestingly, the addition of KCN impaired AP39-induced stomatal closure (2.78 ± 1.02 μm and 3.37 ± 1.08 μm for AP39 and KCN + AP39, respectively), suggesting that an active mETC complex IV is needed for mt-H2S-dependent stomatal closure (Figure 2E). To rule out a putative toxic effect of KCN on GCs, we treated epidermal strips with other widely used stomatal closure stimuli in the presence or the absence of KCN. Supplemental Figure S2 shows that induction of stomatal closure by ABA (but not by darkness) was partially inhibited by KCN (Mock = 4.07 ± 1.12 μm, ABA = 2.78 ± 0.9 μm, KCN + ABA = 3.44 ± 1.09 μm [A]; Mock = 3.99 ± 1.14 μm, darkness = 3.09 ± 0.94 μm, KCN + darkness = 3.29 ± 0.96 μm [B]). In addition, KCN treatments performed in FDA-loaded epidermal strips, showed that the fluorescence levels were not affected upon 2 h of KCN treatment, indicating that stomatal viability was not compromised by the addition of KCN (Supplemental Figure S2C).
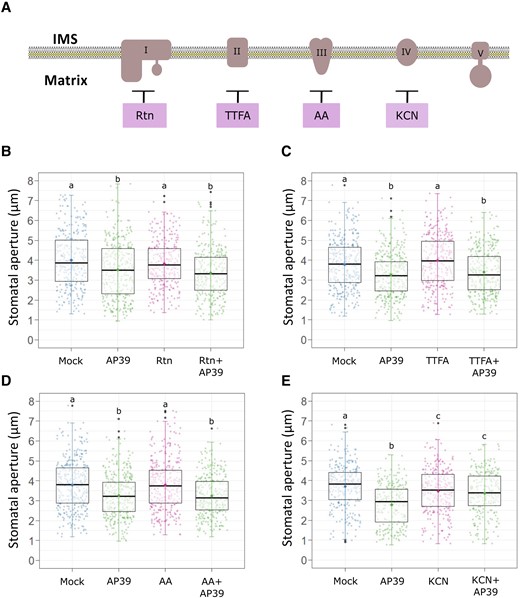
AP39-induced stomatal closure requires complex IV activity. (A), Scheme showing the sites of action of the different mETC inhibitors; Epidermal strips from the abaxial side of five to six weeks old Arabidopsis Col-0 leaves were floated in OB (5 mM MES, 50 mM KCl, pH = 6.1) for 3 h in the light and then they were treated with Dimethyl sulfoxide (DMSO) 0.01% (v/v) (Mock), 100 nM AP39, or they were pre-incubated for 30 min with the different mETC inhibitors: (B) 1 µM Rotenone (Rtn), (C) 100 µM 2-TTFA, (D) 50 µM Antimycin A (AA) or (E) 1 mM potassium cyanide (KCN), and subsequently treated or not with AP39 for 90 min. The values of stomatal aperture are expressed in microns (µm) and are represented as points in the boxplots where the box is bound by the 25th to 75th percentile. The line in the middle is the median, the darker point is the mean and whiskers span 10th to 90th percentile. The black points represent outliers. Data is the result from at least three independent assays. Letters indicate statistical differences among treatments (Tukey's Method, confidence level = 0.95). IMS, intermembrane space.
To further test the requirement of a functional mETC for AP39-triggered stomatal closure, we assessed the stomatal response in plants with a mutated Cytochrome c (CYTc). CYTc is a heme protein located in the intermembrane space (IMS) that functions as an electron carrier between complexes III and IV (Welchen and Gonzalez, 2016). Arabidopsis thaliana has two genes coding for CYTc: CYTC-1 and CYTC-2. In addition to its role as an electron carrier, CYTc is also required for complex IV stability, whose activity and amount is dependent on CYTc levels (Welchen et al., 2012). We measured the stomatal response to AP39 in single and double CYTc Arabidopsis mutants. The lines 1a and 1b are insertional mutants in the gene CYTC-1, while 2a and 2b contain insertions in the gene CYTC-2 (Welchen et al., 2012). Results in Figure 3 show that in the single mutants, AP39 induces stomatal closure comparable to that observed in WT plants, however, the stomatal response is impaired in the double mutants, indicating that CYTc is involved in AP39 response. The differences between the single and the double mutants’ response are in agreement with previous results that reported the existence of a functional redundancy between both CYTc (Welchen et al., 2012). Moreover, we observed that ABA treatment induced stomatal closure in double mutants (Supplemental Figure S3), showing that CYTc activity is needed particularly for mt-H2S signaling pathway.
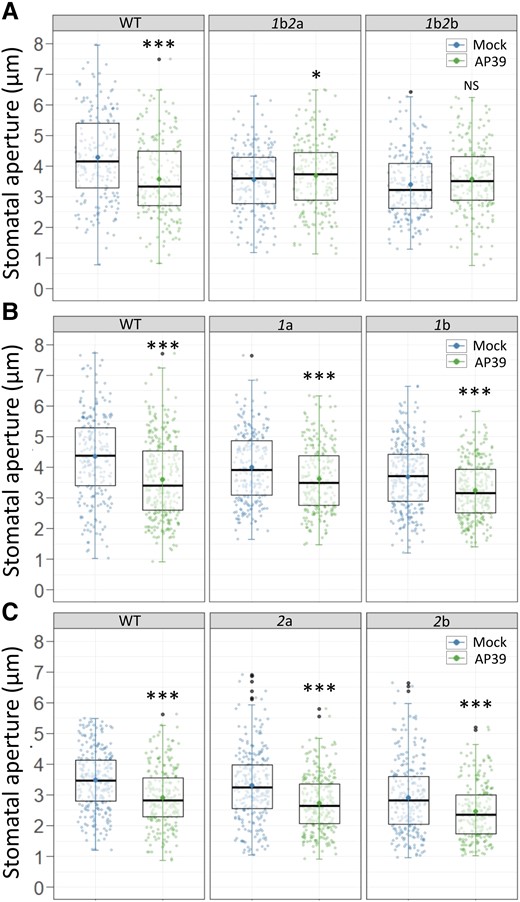
AP39-induced stomatal closure involves cytochrome c (CYTc). Epidermal strips from the abaxial side of leaves of five to six weeks old Arabidopsis double or single insertional mutants in cytochrome c CYTC-1 and CYTC-2 (1b2a and 1b2b (A), 1a and 1b (B), or 2a and 2b (C)), were floated in OB (5 mM MES, 50 mM KCl, pH = 6.1) for 3 h in the light. Then, they were treated for 90 min with Dimethyl sulfoxide (DMSO) 0.01% (v/v) (Mock) or 100 nM AP39. The values of stomatal aperture width are expressed in microns (µm) and are represented as points in the boxplots where the box is bound by the 25th to 75th percentile. The line in the middle is the median, the darker point is the mean and whiskers span 10th to 90th percentile. The black points are outliers. Data is from at least three independent assays. Asterisks indicate statistical differences with the Mock (Student's t test, confidence level = 0.95 (P-value <0.05 = (*), P-value <0.01 = (**), P-value <0.001 = (***), NS = Not significant).
AP39 hyperpolarizes mitochondrial membrane potential in GCs
The requirement of normal CYTc/complex IV activity for AP39-dependent stomatal closure prompted us to analyse the effect of AP39 on mitochondrial inner membrane potential (Δψm) as an indicator of mETC activity (Zorova et al., 2018). For this purpose, Arabidopsis epidermal strips, expressing a mitochondrial-targeted GFP (mito-GFP) (Logan and Leaver, 2000), were loaded with the fluorescent probe red tetramethylrhodamine, methyl ester (TMRM), which accumulates, reversibly, in the mitochondrial matrix depending on the polarization state of the inner membrane (Brand and Nicholls, 2011). TMRM fluorescence intensity increases upon mitochondrial membrane hyperpolarization and decreases when the mitochondrial membrane is less polarized, while GFP intensity is not dependent on the variation of mitochondrial membrane potential. Mito-GFP epidermal strips were pre-incubated in the dark in OB supplemented with TMRM and then treated with DMSO 0.01% (Mock) or AP39. The ratio between TMRM and GFP fluorescence intensity was calculated for each GC (Figure 4). AP39 increased the TMRM/GFP ratio on GCs (Figure 4B), and hence the GCs Δψm, suggesting an mt-H2S-dependent increase on mETC activity. In order to measure another indicator of mitochondria activity, we studied the effect of AP39 on GCs cytosolic ATP pool.
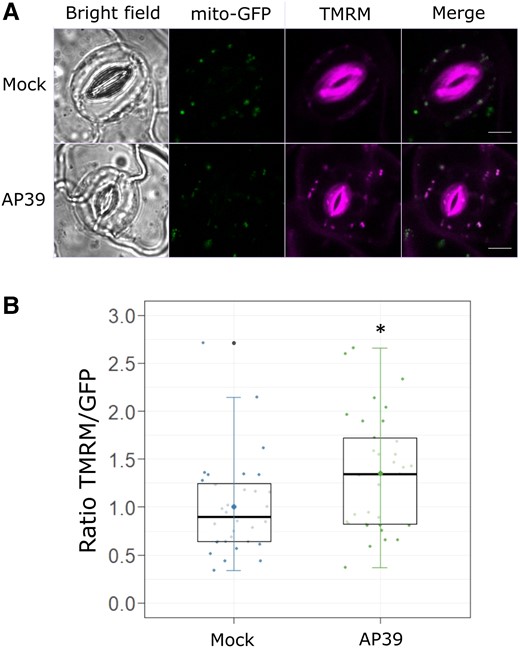
AP39 hyperpolarizes mitochondrial inner membrane potential in GCs. Epidermal peels from abaxial side of five to six weeks old Arabidopsis mito-GFP leaves were incubated in OB (5 mM MES, 50 mM KCl, pH = 6.1) with 500 nM Tetramethylrhodamine methyl ester (TMRM) in the dark, and then treated with Dimethyl sulfoxide (DMSO) 0.01% (v/v) (Mock) or 100 nM AP39 for 90 min. A, Representative false-colored images of GCs treated with the Mock or AP39. B, The ratios between TMRM and GFP fluorescence intensities (TMRM/GFP) were calculated for each guard cell, normalized to the Mock and represented as individual points in the boxplots, where the box is bound by the 25th to 75th percentile. The line in the middle is the median, the darker point is the mean and whiskers span 10th to 90th percentile. The black points are outliers. Data are for at least 30 GCs per treatment and three independent assays. Asterisks indicate statistical differences with the Mock (Student's t test, confidence level = 0.95 (P-value <0.05 = (*)). Scale bars: 5 μm.
AP39 increases ATP levels in GCs
We used Arabidopsis plants expressing the genetically encoded FRET-based sensor ATeam1.03-nD/na, which contains a ε-subunit fragment of ATP synthase from Bacillus sp. PS3, and two fluorophores, a monomeric super-enhanced cyan fluorescent protein (mseCFP) and a circularly permuted monomeric Venus (cp173-mVenus) (Imamura et al., 2009; Kotera et al., 2010). ATeam1.03-nD/Na recognizes MgATP−², the biologically relevant portion of ATP pool, and the interaction leads to a conformational modification of the protein, increasing the FRET efficiency. The simultaneous detection of both fluorescence emissions and the calculation of the Venus/CFP ratio of GCs provides a dynamic readout of the MgATP−² levels in cytosol.
Arabidopsis epidermal strips expressing ATeam 1.03-nD/na were placed in OB for at least 60 min in the dark, to avoid the effects of active photosynthesis (de Col et al., 2017), and then treated or not with AP39. The Venus/CFP ratio calculated for each GC shows that AP39 increased the cytosolic MgATP−² levels, compared with steady conditions (Figure 5), providing further evidence on the effect of mt-H2S on the modulation of mitochondrial bioenergetics.
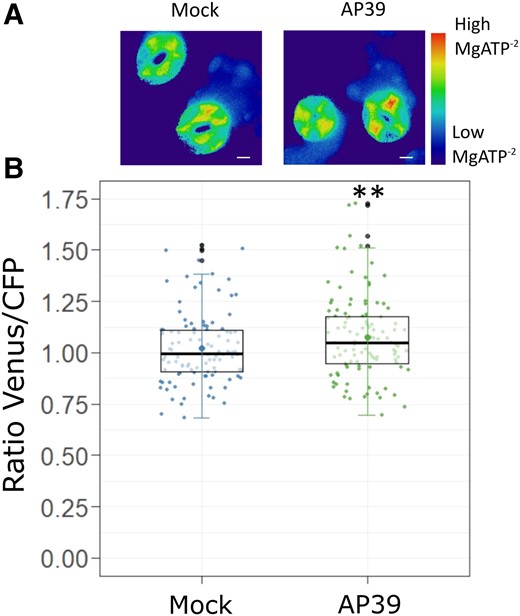
AP39 increases cytosolic MgATP−² levels in GCs. Epidermal strips from abaxial side of five to six weeks old Arabidopsis Col-0 leaves expressing the cytosolic MgATP−² sensor ATeam1.03-nD/Na were placed in OB (5 mM MES, 50 mM KCl, pH = 6.1), dark-adapted at least 60 min and treated for 90 min with Dimethyl sulfoxide (DMSO) 0.01% (v/v) (Mock) or 100 nM AP39. A, Representative false-colored ratiometric images of GCs treated with the Mock or AP39. B, The ratio between Venus and CFP fluorescence intensities (Venus/CFP) were calculated for each guard cell, normalized to the Mock and represented as individual points in the boxplots, where the box is bound by the 25th to 75th percentile. The line in the middle is the median, the darker point is the mean and whiskers span 10th to 90th percentile. The black points are outliers. Data are the result of at least 70 GCs for each treatment from three independent assays. Asterisks indicate statistical differences with the Mock (Student's t test, confidence level = 0.95 (P-value <0.01 = (**)). Scale bars: 5 μm.
AP39 increases H2O2 levels and the oxidation of glutathione in the cytosol
In different animal experimental systems, AP39 was shown to exert its effect not only through the modulation of mitochondrial activity, but also through the regulation of intracellular signaling components, among them, reactive oxygen species (ROS) (Covarrubias et al., 2019). In plants, there is no information of mt-H2S regulation, but we reported the existence of an interplay between H2S and H2O2 during stomatal closure induction. To study whether mt-H2S has any effect on ROS modulation and/or the cellular redox state, we measured cytosolic H2O2 levels and the glutathione (GSH) oxidation status in GCs treated with AP39. In order to detect in vivo and reversibly the H2O2 and the GSH/GSSH ratio dynamics in GCs, we employed Arabidopsis plants expressing the fluorescent proteins roGFP2-Orp1 and Grx1-roGFP2 (Scuffi et al., 2018; Nietzel et al., 2019), respectively. The sensor roGFP2-Orp1 is a construct that contains the yeast peroxidase Orp1 fused to redox-sensitive roGFP2, while Grx1-roGFP2 has roGFP2 fused to human glutaredoxin (Grx1) (Gutscher et al., 2009; Nietzel et al., 2019). Epidermal strips from roGFP2-Orp1 and Grx1-roGFP2 plants were treated or not with AP39 at different times, according to Scuffi et al. (2018). Figure 6 shows that AP39 induced the oxidation of both biosensors in GCs (Figure 6, A and B), indicating that mt-H2S increases the H2O2 content and the oxidation of the GSH. Furthermore, when strips were treated with AP39 in the presence of HT, there was no evident increase on the oxidation of roGFP2-Orp1 (Figure 6C) supporting the involvement of H2S in this response.
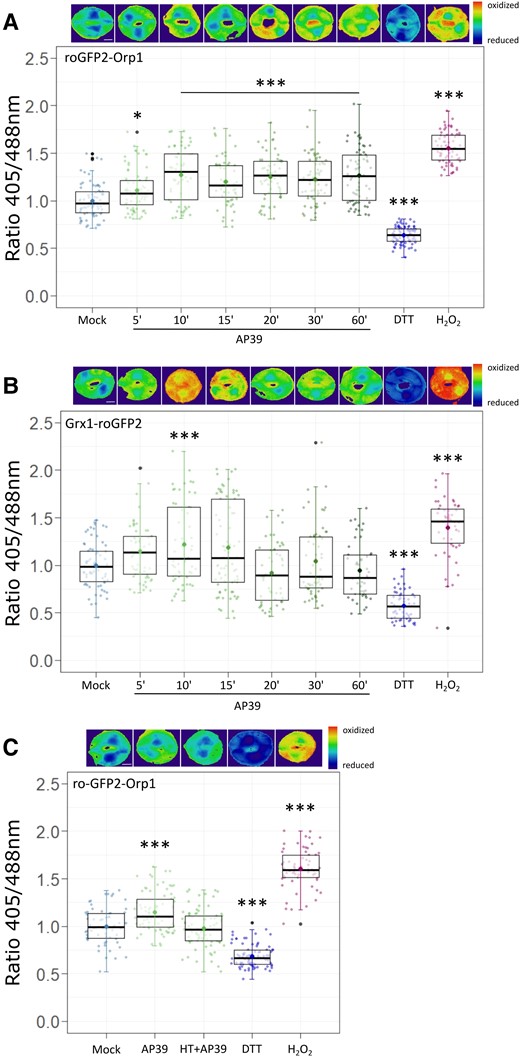
AP39 oxidizes cytosolic roGFP2-Orp1 and Grx1-roGFP2 in GCs. Epidermal peels from Arabidopsis Col-0 leaves expressing the cytosolic biosensors that detect H2O2 and the glutathione (GSH) oxidative state, roGFP2-Orp1 (A, C) or Grx1-roGFP2 (B), respectively, were incubated in opening buffer (OB: 5 mM MES, 50 mM KCl, pH = 6.1) for 7 h under light and then treated with: Dimethyl sulfoxide (DMSO) 0.01% (v/v) (Mock) for 60 min, 100 nM AP39 for 5, 10, 15, 20, 30, or 60 min, 20 mM Dithiothreitol (DTT) for 10 min, 1 mM H2O2 for 10 min, or 200 µM Hypotaurine (HT) and AP39 for 60 min. The ratios between emissions collected with 405/488 nm excitations were calculated for each guard cell, normalized to the Mock and represented as individual points in the boxplots, where the box is bound by the 25th to 75th percentile. The line in the middle is the median, the darker point is the mean and whiskers span 10th to 90th percentile. The black points are outliers. Data are the result of at least three independent assays. Asterisks indicate statistical differences with the Mock (Dunnett's test = (P-value <0.05 = (*), P-value <0.01 = (**), P-value <0.001 = (***)). Ratiometric images are false-colored and represent the different treatments. Scale bars: 5 μm.
Discussion
H2S is already established as a signaling molecule with physiological roles in organisms from the five kingdoms (Olson and Straub, 2016; Aroca et al., 2020). The main enzymatic source of H2S in animal systems, Cystathionine γ-lyase (CSE), is localized in the cytosol, however, under certain stress conditions it can translocate to the mitochondria and exert different physiological roles through mt-H2S production (Fu et al., 2012). On the other hand, the role of the endogenously synthesized mt-H2S in plants remains unclear because most of the knowledge about the biological function of H2S comes from pharmacological data, using the general H2S donors NaHS and GYY4137, or from genetic data related to the cytosolic source DES1 (Scuffi et al., 2018; Shen et al., 2020).
In the present study we prove that mt-H2S, released by mitochondrial-targeted H2S delivery molecules, induces stomatal closure in a CYTc and/or complex IV dependent manner (Figures 2 and 3). The suboptimal response at the higher concentration assayed, corresponds with a reduction in guard cell viability (Figure 1B), which resembles the biphasic dose–response observed for mt-H2S in animal's mitochondria (Szabo et al., 2014). In the latter, high concentrations of mt-H2S inhibit mitochondrial activity, an effect associated with mitochondrial-derived diseases (Panagaki et al., 2019), while at low concentrations, mt-H2S has cytoprotective effects, increasing mitochondrial biogenesis and function (Fox et al., 2021). As in the above-mentioned systems, mt-H2S can act as an inorganic electron donor and interact with mETC components (Módis et al., 2016; Vitvitsky et al., 2018), we analysed the possibility of a link between stomatal closure induction and mETC activity. It has been reported that the activity of the mitochondrial complex II (succinate dehydrogenase [SDH]) is related to stomatal behavior. The heterozygous mutant SDH1-1/sdh1-1 and a line with down-regulation of SHD1-1 expression by RNA interference showed an increased stomatal conductance and stomatal aperture (Fuentes et al., 2011).
In our experimental system, either the inhibition of mitochondrial ETC complex IV or the mutation of both CYTC-1 and CYTC-2 genes, impaired AP39-dependent stomatal closure, suggesting that mt-H2S affects the last steps of mitochondrial ETC. Given that CYTc not only carries electrons to complex IV, but it is also required for the stability of this supercomplex (Welchen et al., 2012), further evidence will be needed to determine whether CYTc, complex IV, or both of them, are involved in the mt-H2S-triggered signaling pathway. These results can be associated with experiments done in human cells, where H2S stimulates O2 consumption even in the presence of AA, consistently with the entry of electrons at the level of complex IV (Vitvitsky et al., 2018).
Mitochondrial ATP production by oxidative phosphorylation (OXPHOS) has a major relevance in most eukaryotic cells as the main energetic source to drive cellular processes. OXPHOS implies the electron transport from reducing equivalents to molecular oxygen, through the mETC, establishing the Δψm required for ATP synthesis (Braun, 2020). Using the fluorescent probe TMRM in Arabidopsis mito-GFP epidermal peels, we show that AP39 hyperpolarizes Δψm in GCs (Figure 4). The increase in mitochondrial inner membrane potential, together with the requirement of CYTc/complex IV for AP39-dependent stomatal closure, suggests that mt-H2S modulates mETC activity, pointing to a relationship between mitochondrial function and stomatal movement regulation.
Cytosolic ATP pool in GCs plays a key role for light-dependent stomatal opening (Inoue and Kinoshita, 2017). ATP is needed to fuel plasma membrane H+-ATPase, which generates the electrochemical gradient that activates inward-rectifying K+ channels, increasing GC's turgor and finally opening the stomatal pore. Recent works suggested that mitochondria are the main ATP source in GCs (Lim et al., 2022), and that plasma membrane H+-ATPase AHA2 is required for ABA-induced stomatal closure (Pei et al., 2022), highlighting the relevance of mitochondrial energetic metabolism for stomatal physiology.
On the other hand, although ATP requirement for stomatal closure is less explored, the inhibition of mitochondrial ATP synthase, partially inhibits dark-mediated stomatal closure (Goh et al., 2011), indicating that mitochondrial ATP production participates in this response. Our data shows that GC cytosolic ATP levels increase in response to the mt-H2S donor (Figure 5). The shift on the Venus/CFP ratio was consistent and reproducible and is in the range of the reported biosensor response to adenine in Arabidopsis seedlings (de Col et al., 2017). Therefore, we provide insights on the relationship between mitochondrial ATP production and stomatal closure induction.
Recent reports indicate that H2S triggers stomatal closure through persulfidation of highly connected components (nodes) that includes the NADPH oxidase Respiratory Burst Oxidase Homolog D (RBOHD) (Shen et al., 2020). Persulfidation promotes RBOHD activity, stimulating the apoplastic superoxide production. Superoxide is rapidly converted to H2O2 either spontaneously or by the activity of superoxide dismutase (Chen and Yang, 2020), and then H2O2 is believed to enter GCs trough aquaporins (Rodrigues et al., 2017). In addition, the treatment of epidermal peels with general H2S donors (NaHS and GYY4137) produces an increase in cytosolic H2O2 levels in GCs (Scuffi et al., 2018), showing an interplay between ROS and H2S. On the other hand, in the last years some works have shown that mitochondrial activity is related to RBOHs-dependent ROS production. The mitochondrial enzyme NAD kinase-CaM dependent (NADKc) catalyzes NADP+ production exclusively in the presence of CaM/Ca2+. The insertional mutants in NADKc, nadkc-1 and nadkc-2, displayed a substantial reduction of extracellular ROS accumulation when seedlings were treated with the pathogen-derived peptide flagellin (flg22) (Dell’Aglio et al., 2019). Authors proposed that the NADKc-dependent NADP(H) synthesis is needed to sustain ROS burst driven by plasma membrane NADPH oxidases. Furthermore, Fabro et al., (2016) reported the same altered response in ROS production in plants mutated in the enzyme PROLINE DEHYDROGENASE (ProDH), which transfer electrons into the mETC. Despite ROS accumulation is a key mechanism that has been observed downstream of several signals that trigger stomatal closure (Arnaud and Hwang, 2015; Zhang et al., 2017; Postiglione and Muday, 2020), there are no reports showing the participation of mitochondrial activity on ROS production in GCs. Based on these pieces of evidence that connect mitochondrial function with ROS accumulation, together with the, well established, interplay between H2S and H2O2 in GCs, we analysed cytosolic H2O2 content and the GSH/GSSH ratio. In Figure 6, we show that AP39 triggers the oxidation of both roGFP2-Orp1 and Grx1-roGFP2 sensors, but their dynamics are different. After reaching a maximum oxidation at 10 min, Grx1-roGFP2 was fully reduced back to its initial state. These results indicate that the mt-H2S donor increases the H2O2 levels and the oxidation of the GSH pool in GCs, pointing to a signaling role of mitochondrial H2S over cytosolic components in the regulation of stomatal closure. Although it is tempting to link the raise of cytosolic H2O2 to its reported effect on inward-rectifying K+ channels (IKIN) activity (Köhler et al., 2003), it is difficult to propose a linear pathway in such a complex signaling network.
Based in our findings, we propose that mitochondrially targeted H2S delivery molecule AP39 induces stomatal closure, a response that depends on a functional CYTc or/and complex IV. Additionally, this donor also hyperpolarizes the mitochondrial inner membrane, increases the cytosolic GSH oxidation, and the ATP and H2O2 content in GCs (Figure 7). Taken together, these results point towards an effect of mt-H2S over mETC at the CYTc/complex IV level that induces an increase in the mitochondrial inner membrane potential and an increase in the ATP synthesis in GCs. The modulation of mitochondrial activity by AP39 can possibly lead to the spread of the signal to other cellular compartments, increasing the oxidation of GSH pool and the H2O2 levels in GCs, finally producing stomatal closure. These observations indicate that mt-H2S has a function in the modulation of mitochondrial energetics in plants, while they also point to an active role of mitochondrial activity in stomatal closure. Nevertheless, more studies are required to unveil the gap between the mitochondrial effect of AP39 and the cytosolic H2O2 increase in GCs, as well as the relevance of this signaling pathway in a physiological context to further understand the mitochondrial role in GC's signaling network.
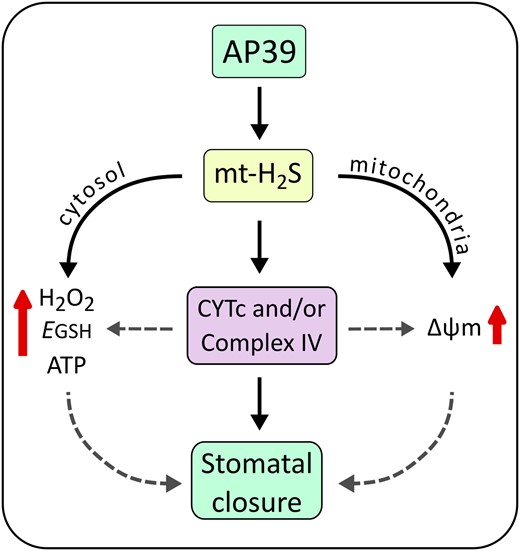
Model of mitochondrial (mt)-H2S-triggered signaling pathway in GCs. The donor AP39 accumulates in mitochondria and releases mitochondrial (mt)-H2S, triggering a signaling pathway that concludes in stomatal closure. To induce the reduction of stomatal pore size, AP39 requires the activity of cytochrome c (CYTc) and/or mitochondrial complex IV. Further, AP39 hyperpolarizes the mitochondrial membrane potential (Δψm) and increases ATP synthesis in GCs, as well as the cytosolic H2O2 levels and the oxidation of the glutathione (GSH) pool. Segmented lines suggest a possible participation of H2O2, GSH, ATP, and Δψm on stomatal closure induction, which can be modulated through CYTc/complex IV.
Materials and methods
Plant material and chemicals
Arabidopsis (Arabidopsis thaliana) wild-type Columbia-0 (Col-0) ecotype, Arabidopsis mito-GFP, Arabidopsis CYTc mutants 1a, 1b, 2a, 2b, 1b2a, and 1b2b (Welchen et al., 2012), and Arabidopsis lines expressing the biosensors ATeam1.03-nD/nA (de Col et al., 2017), roGFP2-Orp1, and Grx1-roGFP2 (Gutscher et al., 2009; Nietzel et al., 2019) (kindly provided by Prof. Markus Schwarzländer) were grown in soil:perlite:vermiculite (1:1:1, v/v/v); while fava bean (Vicia faba were grown in soil:vermiculite (1:3 v/v). All plants were placed at 20°C under short-day conditions (8-h- light/16-h-dark photoperiod, 200 µmol photons m−2 s−1), after stratification for 48 h at 4°C in the dark.
Mitochondrial H2S donors AP123 (Gerő et al., 2016) (10-(4-carbamothioylphenoxy)-10-oxodecyl) triphenylphosphonium bromide), AP39 (le Trionnaire et al., 2014), ((10-oxo-10-(4-(3-thioxo-3H-1,2-dithiol-5-yl)phenoxy) decyl) triphenylphosphonium bromide) and RT01 (Latorre et al., 2018) were synthesized as previously described by us. GYY4137 (morpholin-4-ium4 methoxyphenyl(morpholino) phosphinodithioate) was purchased from Sigma-Aldrich. Rotenone, 2-TTFA, and Antimycin A (AA) were purchased from Tecnolab S.A., while Potassium cyanide (KCN) was purchased from Mallinckrodt Pharmaceuticals. Tetramethylrhodamine methyl ester (TMRM) was purchased from Biotium.
Stomatal aperture measurements
Epidermal peels were excised from abaxial side of five to six weeks old Arabidopsis thaliana Col-0 or four-weeks old Vicia faba leaves and immediately floated in Opening Buffer (OB: 5 mM MES-KOH, 50 mM KCl, pH = 6.1) for 3 h in the light. Strips were then kept in the same OB and exposed to different treatments as indicated in the figure legends. Stomata were digitized using an AmScope MU1000 camera coupled to an Olympus CKX53 microscope with a 40 × lens (LUCPlanFLN, 0.6 numerical aperture). The stomatal aperture was measured using IMAGEJ analysis software (NIH, Bethesda, MD, USA).
Viability staining
Arabidopsis Col-0 epidermal strips were incubated for 3 h in OB in light and exposed to different treatments. Then, peels were incubated with 20 µM FDA for 10 min in the dark at room temperature and washed with fresh OB to remove excess dye. Fluorescence was imaged with a 40 × lens (LUCPlanFLN, 0.6 numerical aperture) using an Amscope MU900 camera coupled to an Olympus CKX41 microscope equipped with an Olympus U-RFLT50 lamp, a BP460–490C excitation filter and a BA520 IF barrier filter. Exposure times (from 100 to 300 ms) were adjusted depending on the probe fluorescence intensity in each experiment.
Confocal microscopy
Fluorescence images were acquired with a Nikon Eclipse C1 Plus confocal microscope. Mitochondria in GCs were imaged with a 100 × lens (Plan Apochromat Lambda, 1.45 numerical aperture, oil immersion). Epidermal peels from Arabidopsis thaliana expressing a mitochondrial-targeted GFP (mito-GFP) were floated in OB supplemented with 500 nM Tetramethylrhodamine methyl ester (TMRM) for 3 h in the dark and then treated for 90 min with Mock (DMSO 0.01% (v/v)) or 100 nM AP39. TMRM and GFP were excited at 488 nm and 561 nm, respectively, and their emissions were collected simultaneously at 515/30 and 605/75 nm. The laser intensity was set to 4% for 488 nm and 5% for 561 nm. The ratio TMRM/GFP was calculated for each GC and the values were normalized to the Mock.
Fluorescence microscopy
GCs of plants expressing ATeam1.03-nD/Na, roGFP2-Orp1 and Grx1-roGFP2 biosensors were analyzed in vivo using an inverted Nikon Ti-E fluorescence microscope coupled to a Hamamatsu ORCA-D2 Dual CCD camera. Excitation light was produced by a fluorescent lamp Prior Lumen 200 PRO (Prior Scientific) and epidermal strips were imaged using a 60 × oil immersion objective (CFI Plan APO Lambda 60 × 1.4 numerical aperture). Epidermal peels from ATeam1.03-nD/nA leaves were floated in OB a least 1 h in the dark and then treated for 1 h with DMSO 0.01% (v/v) (Mock) or 100 nM AP39. Peels were excited at 440 nm (436/20 nm), and mseCFP and cp173-mVenus emissions were collected at 483/32 nm and 542/27 nm, respectively, with a dichroic 510-nm mirror (Hamamatsu Pho-tonics) for simultaneous acquisitions. Exposure times (from 150 to 200 ms) were adjusted depending on the sensor expression level in each experiment, with a 4 × 4 pixel binning. The ratio Venus/CFP was calculated for each GC and the values were normalized to the Mock.
roGFP2-Orp1 and Grx1-roGFP2 peels were floated in OB at least 7 h in light and then treated with DMSO 0.01% (v/v) (Mock), 100 nM AP39, or AP39 and 200 µM HT for the indicated times. Sensors were excited sequentially with 470/40 nm and 405/40 nm and the emissions were collected using a 505/530 nm bandpass filter (GFP-specific filter) for both excitation wavelengths with a 2 × 2 pixel binning. For roGFP2-Orp1 and Grx1-roGFP2 experiments, the exposure times were 100 ms and 200 ms, and 50 ms and 200 ms, for 405/40 nm and 470/40 nm excitations, respectively. The ratio 405/488 nm was calculated for each GC and the values were normalized to the Mock.
Images analysis
Images were analysed using IMAGEJ software (NIH, Bethesda, MD, USA). For TMRM/GFP ratio calculation and fluorescence measurement from roGFP2-Orp1, Grx1-roGFP2 and ATeam1.03-nD/nA sensors, the background was subtracted from each channel and then the region of interest was delimited to GCs. Fluorescence was measured as the mean pixel intensity.
To obtain the ratiometric false-colored ATeam1.03-nD/nA images, background was subtracted to mseCFP and cp173-mVenus emissions in representative images, and then they were thresholded. Finally, cp173-mVenus/mseCFP (Venus/CFP in Figure 5) was generated with Image Calculator and a pseudocolor (LUT Physics) was applied to the resulting image.
Statistical analyses
Data analyses were performed using RStudio (R Foundation for Statistical Computing, Vienna, Austria. URL: https://www.R-project.org/). The statistically significant differences were analyzed using Student's t test, Tukey's Method, or Dunnett's Method, as indicated in the figure legends.
Accession numbers
Sequence data from this article can be found in the GenBank/EMBL data libraries under accession numbers At1g22840 (CYTC-1) and At4g10040 (CYTC-2).
Supplemental data
The following materials are available in the online version of this article.
Supplemental Figure S1. Mitochondrial H2S donors induce stomatal closure in a dose-dependent manner.
Supplemental Figure S2. Abscisic acid (ABA) and darkness induce stomatal closure in the presence of KCN.
Supplemental Figure S3. Abscisic acid (ABA) induces stomatal closure in cytochrome c (CYTc) double mutants 1b2a and 1b2b.
Acknowledgments
We thank to Ana M. Laxalt and the members of the Signaling Mechanisms in Plants Lab for the challenging discussion.
Funding
This work was financially supported by Universidad Nacional de Mar del Plata (UNMDP), Consejo Nacional de Investigaciones Científicas y Técnicas (CONICET), Agencia Nacional de Promoción Científica y Tecnológica and The Company of Biologists. PICT 2016 N°2553 (to C.G.M.) PICT 2018 N°1986 (to C.G.M.) and PICT 2018 N°1449 (to D.S.), PhD finishing fellowship from CONICET (to R.P.), and Traveling Fellowship from The Company of Biologists (to R.P.).
References
Author notes
R.P. performed most of the experiments, analysed the data, and wrote the article; D.S. performed experiments from Figure 1, analysed the data, and helped with the writing of the article; R.T. and M.W. contributed with the reagents and assisted in the writing of the article; E.W. provided biological material and contributed to discussion; A.C. participated in the experimental design and discussion, and C.G.M. conceived the project, analysed the data, and wrote the article.
The author responsible for distribution of materials integral to the findings presented in this article in accordance with the policy described in the Instructions for Authors (https://dbpia.nl.go.kr/plphys/pages/General-Instructions) is Carlos García-Mata ([email protected]).
Conflict of interests. M.W and R.T. have intellectual property (patents awarded) on mitochondrial and other targeted H2S donors and MW is CSO of MitoRx Therapeutics.