-
PDF
- Split View
-
Views
-
Cite
Cite
Kamil Demski, Simon Jeppson, Sten Stymne, Ida Lager, Phosphatidylcholine:diacylglycerol cholinephosphotransferase’s unique regulation of castor bean oil quality, Plant Physiology, Volume 189, Issue 4, August 2022, Pages 2001–2014, https://doi.org/10.1093/plphys/kiac209
- Share Icon Share
Abstract
Castor bean (Ricinus communis) seed oil (triacylglycerol [TAG]) is composed of ∼90% of the industrially important ricinoleoyl (12-hydroxy-9-octadecenoyl) groups. Here, phosphatidylcholine (PC):diacylglycerol (DAG) cholinephosphotransferase (PDCT) from castor bean was biochemically characterized and compared with camelina (Camelina sativa) PDCT. DAGs with ricinoleoyl groups were poorly used by Camelina PDCT, and their presence inhibited the utilization of DAG with “common” acyl groups. In contrast, castor PDCT utilized DAG with ricinoleoyl groups similarly to DAG with common acyl groups and showed a 10-fold selectivity for DAG with one ricinoleoyl group over DAG with two ricinoleoyl groups. Castor DAG acyltransferase2 specificities and selectivities toward different DAG and acyl-CoA species were assessed and shown to not acylate DAG without ricinoleoyl groups in the presence of ricinoleoyl-containing DAG. Eighty-five percent of the DAG species in microsomal membranes prepared from developing castor endosperm lacked ricinoleoyl groups. Most of these species were predicted to be derived from PC, which had been formed by PDCT in exchange with DAG with one ricinoleoyl group. A scheme of the function of PDCT in castor endosperm is proposed where one ricinoleoyl group from de novo-synthesized DAG is selectivity transferred to PC. Nonricinoleate DAG is formed and ricinoleoyl groups entering PC are re-used either in de novo synthesis of DAG with two ricinoleoyl groups or in direct synthesis of triricinoleoyl TAG by PDAT. The PC-derived DAG is not used in TAG synthesis but is proposed to serve as a substrate in membrane lipid biosynthesis during oil deposition.
Introduction
The triacylglycerols (TAGs) in seeds are synthesized by acylation of glycerol-3-phosphate (G3P) with acyl-CoA catalyzed by the G3P acyltransferase (GPAT) to form lysophosphatidic acid (LPA), which is further acylated with acyl-CoA by the LPA acyltransferase (LPAT) to phosphatidic acid (PA). The phosphate group of PA is hydrolyzed by the PA phosphohydrolase (PAP) to diacylglycerol (DAG), which is acylated with acyl-CoA catalyzed by DAG acyltransferases (DGAT) to form TAG. This pathway, named the G3P pathway or Kennedy pathway was first described by Kennedy (1961) in an animal system and shortly after in plants by Barron and Stumpf (1962). It was later shown that linoleic and linolenic acids in seeds are synthesized from oleate while esterified to phosphatidylcholine (PC) (Stymne and Appelqvist, 1978; Stymne and Appelqvist, 1980). Acyl groups are synthesized de novo in the plastid and exported to the cytosol as CoA derivatives of palmitate (16:0), stearate (18:0), and oleate (18:1). Any polyunsaturated acyl groups in TAG are produced through conversion of PC-bound 18:1 to linoleate (18:2) and further to linolenate (18:3). These acyls are then transferred from PC into TAG. Oleate can be transferred into PC by acyl-CoA:lysophosphatidylcholine acyltransferase (LPCAT) or by PC:DAG cholinephosphotransferase (PDCT). Polyunsaturated fatty acids from PC are made available for TAG synthesis by the reverse reaction of LPCAT, PDCT, and the phospholipid:diacylglycerol acyltransferase (PDAT) (Bates et al., 2013). PDCT interchanges the glycerol backbone of PC and DAG by the transfer a phosphocholine group. A mutation in PDCT gene lowers the polyunsaturated acyl content in Arabidopsis (Arabidopsis thaliana) seed TAG by 40% (Lu et al., 2009). The DAG that is derived from PC, through the action of PDCT, can be used in TAG synthesis either by DGATs or by PDAT to form TAG (Bates et al., 2013). The importance of PDCT in providing seed TAG with polyunsaturated fatty acids has been shown in a number of in vivo radioactive labeling studies (Bates et al., 2009; Lu et al., 2009; Bates and Browse, 2012).
Castor bean (Ricinus communis) seed oil has over 90% of ricinoleate (Δ12-hydroxy-9-octadecenoate). This hydroxylated acyl group is formed from 18:1 while esterified to PC by the action of a Δ12 oleate hydroxylase. The ricinoleate is rapidly transferred from PC to TAG (Bafor et al., 1991).
Formation of PC from de novo synthesized DAG with ricinoleoyl groups as well as DAG with no hydroxy fatty acids was demonstrated in microsomal fractions from developing castor endosperm (Bafor et al., 1991), indicating PDCT activity in these membranes. Expression of castor PDCT in Arabidopsis expressing the castor oleate Δ12 hydroxylase increased the total amount of ricinoleate in TAG compared with only expressing the hydroxylase (from 17% to 23%) (Hu et al., 2012). It was shown earlier that co-expression of the castor DGAT2 also increased the total amount of ricinoleate in TAG compared with only expressing the castor hydroxylase (Burgal et al., 2008). Expression of castor PDCT together with the hydroxylase and castor DGAT2 in Arabidopsis further enhanced the amount of ricinoleate in TAG (from 25% to 28.5%) (Hu et al., 2012). The function of castor PDCT is enigmatic since the amount of ricinoleate is very low in PC in castor seeds due to its rapid removal after synthesis (Bafor et al., 1991).
In this work, we demonstrate a radical difference between castor and camelina (Camelina sativa) PDCTs. Castor PDCT differs from Camelina in its high activity toward ricinoleoyl-containing DAG, in contrast to the inhibiting effects seen in Camelina PDCT activity (Lager et al., 2020). Based on the properties of castor DGAT2 and PDCT and analysis of microsomal DAG from developing castor endosperm, a scheme emerged of how these two enzymes work in concert to optimize the levels of TAG with three ricinoleoyl groups in castor, and simultaneously produce a DAG pool devoid of ricinoleoyl groups that is not used in TAG synthesis. The flow of radioactivity into lipids from [14C]G3P and acyl-CoA in microsomal fractions from developing castor endosperm was consistent with the proposed scheme.
Results
Utilization of de novo formed ricinoleoyl-containing DAG by Camelina PDCT and castor PDCT
We previously demonstrated Camelina PDCT activity using microsomes prepared from yeast (Saccharomyces cerevisiae) strain H1246 incubated with [14C]G3P and acyl-CoA (Lager et al., 2020). This yeast strain lacks DAG acylating enzymes (Sandager et al., 2002) and thus, DAG is the radioactive end product in the acylation of [14C]G3P with acyl-CoA in microsomal preparations from this strain. However, microsomal fractions from H1246 stain expressing the Camelina PDCT shunted a major part of de novo synthesized DAG into PC, in contrast to vector controls (Lager et al., 2020). We repeated these assays with Camelina PDCT and did corresponding assays with yeast H1246 expressing castor PDCT and empty plasmid. In order to optimize the assays, we also investigated if prolonged incubation time from the published 60 min (Lager et al., 2020) to 150 min increased the amount of DAG utilized by Camelina PDCT. However, the accumulation of radioactivity in PC leveled off after 60 min despite a substantial increase in DAG (Supplemental Figure S1). Therefore, further assays were done in 60 min.
Microsomal preparations from the yeast strains were assayed with [14C]G3P, nonhydroxylated acyl-CoA (18:3-CoA), ricinoleoyl-CoA, and a 2:3 mixture of these acyl-CoAs. 18:3-CoA was chosen as acyl donor since PA was more efficiently transferred to DAG than when using 18:1-CoA or 18:2-CoA (Lager et al., 2020).
Assays with microsomal fractions from H1246 with empty vector showed accumulation of radioactive PA and DAG with only small amount of radioactivity in the area where PC migrated on the thin layer chromatography plate (TLC) (Supplemental Figure S2). With 18:3-CoA, 1.5% of the total radioactivity in chloroform soluble lipids was found in this area. Corresponding figures in incubations with ricinoleoyl-CoA and mixed acyl-CoAs were 1.0% and 2.0%, respectively (Supplemental Figure S2). This radioactivity is most likely not residing in PC since Demski et al. (2021) showed that no radioactive PC was formed from added radioactive DAG in incubations with microsomal preparations of H1246 transformed with empty vector, in contrast to microsomal membranes prepared from H1246 cells transformed with Camelina PDCT. Since LPA co-chromatographed with PC in the TLC system used, we consider it most likely that the background radioactivity found in PC area of the TLC in empty plasmid assays originated from LPA.
In assays with microsomal preparations from yeast transformed with Camelina PDCT, 18:3-CoA and [14C]G3P, 42% of the chloroform soluble radioactivity was found in PC (Figure 1A) which is 28 times higher than the percentage in corresponding assays with empty vector (Supplemental Figure S2A). In assays with ricinoleoyl-CoA, only 5% of the radioactivity entered PC (Figure 1B), which is approximately five times more than with assays with microsomal membranes with empty vector (Supplemental Figure S2B). When 18:3-CoA and ricinoleoyl-CoA mixed in 2:3 proportions were used, equal amounts of radioactivity in DAG with no ricinoleoyl groups (0-OH-DAG) and two ricinoleoyl groups (2-OH-DAG) were formed with one-fourth of those amounts found in DAG with one ricinoleoyl group (1-OH-DAG) (Figure 1C). Only 4% of the radioactivity in chloroform soluble lipids was found in PC, a value similar to the percentage in assays with only ricinoleoyl-CoA added and 2.5 times higher than corresponding incubations with microsomes from empty vector control (Supplemental Figure S2C). The results of the assays with Camelina PDCT closely resembled the results published by Lager et al. (2020).
![Utilization of de novo synthesized DAG with and without ricinoleoyl groups by Camelina PDCT. Formation of radioactive lipids from [14C] G3P, 18:3-CoA (A) and ricinoleoyl-CoA (Ric-CoA) (B) as single acyl-CoA substrate or in mixtures in proportions 2:3 (C) in microsomal preparations of H1246 yeast expressing Camelina PDCT. 0-OH-DAG, 1-OH-DAG, and 2-OH-DAG, DAGs with no, one, and two ricinoleoyl groups, respectively; 0-OH-PC, 1-OH-PC and 2-OH-PC, PC with no, one, and two ricinoleyl groups, respectively. Average value shown ± sd, n = 3 replicates.](https://oup.silverchair-cdn.com/oup/backfile/Content_public/Journal/plphys/189/4/10.1093_plphys_kiac209/3/m_kiac209f1.jpeg?Expires=1750194010&Signature=KsdwPvc4L5AnVZre~g3mOnCHLtqnKaPLNoWXZNtBDX-Q~tww9VL5ADD8KP~NXLGpE4VynvOBTSsuz8gPKejxaoYXoFauxLdfdz3TDVMyjuaGp9FBaf6cnsuvEfSCROz-WcsVueqUpnpVmOMfjy0CW~FsfAenT~0cHiFGIOT4D1I2y9NCTW2ic6a3HWqr0W5K7XCCXSqDGOAMMaA02DQZCNwb20GkPkIbdASGAXXiWv1js7~HcFaQpioCV3q1x5pEo-zKCUPElWP3JQT-kGcrYw3AvUXM2hIoEwrYI6ZlvaKnPy1CEmh3tKAupL8j4cQkmI3q4ILZ8vJ533oAoKelng__&Key-Pair-Id=APKAIE5G5CRDK6RD3PGA)
Utilization of de novo synthesized DAG with and without ricinoleoyl groups by Camelina PDCT. Formation of radioactive lipids from [14C] G3P, 18:3-CoA (A) and ricinoleoyl-CoA (Ric-CoA) (B) as single acyl-CoA substrate or in mixtures in proportions 2:3 (C) in microsomal preparations of H1246 yeast expressing Camelina PDCT. 0-OH-DAG, 1-OH-DAG, and 2-OH-DAG, DAGs with no, one, and two ricinoleoyl groups, respectively; 0-OH-PC, 1-OH-PC and 2-OH-PC, PC with no, one, and two ricinoleyl groups, respectively. Average value shown ± sd, n = 3 replicates.
Assays with membranes from H1246 yeast expressing castor PDCT are shown in Figure 2. 18:3-CoA was metabolized similarly to the membranes with Camelina PDCT with 40% of the chloroform soluble lipid entering PC (Figure 2A). A striking difference between Camelina PDCT and castor PDCT was seen in the metabolism of ricinoleoyl-DAG when ricinoleoyl-CoA was used as a substrate (compare Figure 1B with Figure 2B). Only radioactive 2-OH-DAG of DAG species was formed and ∼56% of the chloroform soluble radioactivity entered PC, compared with 5% in Camelina PDCT assays. Although the radioactive PC was dominated by 2-OH-PC (72%), substantial amounts of radio-labeled 1-OH-PC and 0-OH-PC were found, indicating that some rearrangement of ricinoleoyl groups had occurred on the [14C]glycerol backbone of PC (Figure 2B).
![Utilization of de novo synthesized DAG with and without ricinoleoyl groups by castor PDCT. Formation of radioactive lipids from [14C]G3P, 18:3-CoA (A) and ricinoleoyl-CoA (Ric-CoA) (B), presented as single substrate or in mixtures in proportions 2:3 (C). Activities were measured in microsomal preparations of H1246 yeast expressing castor PDCT. 0-OH-DAG, 1-OH-DAG and 2-OH-DAG, DAG, with one, two and three ricinoleoyl groups, respectively; 0-OH-PC, 1-OH-PC, PC with no, one and two ricinoleoyl groups, respectively. Average value shown ± sd, n = 3 replicates.](https://oup.silverchair-cdn.com/oup/backfile/Content_public/Journal/plphys/189/4/10.1093_plphys_kiac209/3/m_kiac209f2.jpeg?Expires=1750194010&Signature=dICeNGa~V22X1VJ3cu8z330tKi4OBIcllAntFeGmBoGaOGeQLEigP90AE-qHMI72IWIt-WJcXM898g1u~BmVSvZZzVVuz8obKn-G5MaaVmH9XB0n1XZoldr~BYyDF~1HBpOZZ9cgYcEyG4YGrJSO3LVWG3VZ8pYdQJSDIB5MzXadn0OXuCEsTJh~odiONz0U5u7n286e1UjqkbQzTIk9vzKJevWMsaP6fXMQZvNLmcO1whPHYzzxIdXAo5K5kFmYuC2rNM69vBHCKnghTEyDHncXAHyJZLmLWzHTdGYMFhWFIMgSxY-2CngjAA~Va7oloV3Z9nuNi9fXB5FwOh5eCw__&Key-Pair-Id=APKAIE5G5CRDK6RD3PGA)
Utilization of de novo synthesized DAG with and without ricinoleoyl groups by castor PDCT. Formation of radioactive lipids from [14C]G3P, 18:3-CoA (A) and ricinoleoyl-CoA (Ric-CoA) (B), presented as single substrate or in mixtures in proportions 2:3 (C). Activities were measured in microsomal preparations of H1246 yeast expressing castor PDCT. 0-OH-DAG, 1-OH-DAG and 2-OH-DAG, DAG, with one, two and three ricinoleoyl groups, respectively; 0-OH-PC, 1-OH-PC, PC with no, one and two ricinoleoyl groups, respectively. Average value shown ± sd, n = 3 replicates.
When a mixture of 18:3-CoA and ricinoleoyl-CoA (in 2:3 proportion) was used in assays with yeast membranes containing castor PDCT, the relative distribution of radioactivity in the DAG species was as follows: 40% 0-OH-DAG, 14% 1-OH-DAG, and 46% 2-OH-DAG (Figure 2C). About 40% of the chloroform soluble radioactivity had entered PC, compared with 4% with Camelina PDCT. The distribution of PC species with ricinoleoyl groups showed radically different distribution compared with DAG with 61% 0-OH-PC, 30% 1-OH-PC, and 9% 2-OH-PC (Figure 2C). Thus, although the 2-OH-DAG species was efficiently used by castor PDCT (Figure 2B), these species were strongly selected against in the presence of 1-OH-DAG species, which were strongly selected for by castor PDCT.
We did similar assays with microsomal fractions from developing castor endosperm as was done with yeast microsomal fractions (Figure 3). Here, we used a 1:1 mixture of nonradioactive 18:1-CoA and ricinoleoyl-CoA together with [14C]G3P. Unlike yeast H1246 microsomes, castor microsomes contain active TAG synthesizing enzymes (PDAT and DGAT) and therefore the de novo formed DAG will not only be utilized by PDCT but also by these enzymes. Since the assays did not contain NADH, which is necessary for hydroxylation of 18:1-PC (Bafor et al., 1991), no net synthesis of ricinoleoyl-PC occurred during these assays. Since ricinoleoyl-PC is the much preferred acyl donor for castor PDAT (Dahlqvist et al., 2000; Lunn et al., 2019), it is reasonable to assume that due to the lack of net synthesis of ricinoleoyl-PC, conversion of [14C]DAG into [14C]TAG in the assays is mainly due to castor DGAT rather than PDAT activity.
![Accumulation of radioactive lipids from [14C]G3P and a mixture of 18:1-CoA and ricinoleoyl-CoA in microsomal preparation of developing castor endosperm. Incubations were performed for 30 and 60 min as indicated in the figure and contained 150 µg of castor microsomal protein, 12.5 nmol of [14C]G3P, 12.5 nmol 18:1-CoA, and 12.5 nmol of ricinoleoyl-CoA in total volume of 100 µL of 0.1 M Tris–HCl buffer, pH 7.2. A, Amount of radioactivity lipids in DAG, TAG, and PC. B, Amount of radioactivity in PA. 0-OH-DAG, 1-OH-DAG and 2-OH-DAG, DAGs with no, one and two ricinoleoyl groups, respectively. 0-OH-PC, 1-OH-PC and 2-OH-PC, PC with no, one and two ricinoleoyl groups. 0-OH-TAG, 1-OH-TAG, 2-OH-TAG and 3-OH-TAG, TAG with no, one, two and three ricinoleoyl groups, respectively. Average value shown ± sd, n = 3 replicates.](https://oup.silverchair-cdn.com/oup/backfile/Content_public/Journal/plphys/189/4/10.1093_plphys_kiac209/3/m_kiac209f3.jpeg?Expires=1750194010&Signature=lBywGnOWrOc0BWgjUPisZMzO3lB~WOsuj68oFRqp77XVZhy1rdm7VvCDMwWsJUKj1~69--yiEGJ9qptC0nHTlI7aJ1Sms3DcP-meRyzZtczuzaq884inNGT314QF3QklsyHwL0sHngcg3LfKkQXvDUH3R7yhrPr1ubqwPXMnFNiliBG3Xx7qZdBYRfY~L8xWlrJXHfv6ajhx5ZJPclvkrALx01CYjOHU3WUkpYJ6Q8ItbDpt3TYyjMc852ubZY950ZShpq2pWNVf71eEk77v8J-us3UL53H1oJqORDTiD0LoGWr1fOx7Nm85dFZH7fi5umLdJ6y68QeT7kPKIscIJA__&Key-Pair-Id=APKAIE5G5CRDK6RD3PGA)
Accumulation of radioactive lipids from [14C]G3P and a mixture of 18:1-CoA and ricinoleoyl-CoA in microsomal preparation of developing castor endosperm. Incubations were performed for 30 and 60 min as indicated in the figure and contained 150 µg of castor microsomal protein, 12.5 nmol of [14C]G3P, 12.5 nmol 18:1-CoA, and 12.5 nmol of ricinoleoyl-CoA in total volume of 100 µL of 0.1 M Tris–HCl buffer, pH 7.2. A, Amount of radioactivity lipids in DAG, TAG, and PC. B, Amount of radioactivity in PA. 0-OH-DAG, 1-OH-DAG and 2-OH-DAG, DAGs with no, one and two ricinoleoyl groups, respectively. 0-OH-PC, 1-OH-PC and 2-OH-PC, PC with no, one and two ricinoleoyl groups. 0-OH-TAG, 1-OH-TAG, 2-OH-TAG and 3-OH-TAG, TAG with no, one, two and three ricinoleoyl groups, respectively. Average value shown ± sd, n = 3 replicates.
We compared the 14C-incorporation into PA, DAG, and TAG after 30 and 60 min incubation. The majority of the radioactivity was found in PA both at 30 and 60 min, indicating that PAP was limiting in the G3P pathway in these membranes (Figure 3). Similar high accumulation of PA in castor bean microsomes fed [14C]G3P and 18:1-CoA was observed earlier (Bafor et al. 1991). After 30 min incubation, about equal amounts of radioactive 0-OH-DAG, 1-OH-DAG, 2-OH-DAG, 0-OH-PC, and 1-OH-PC were found whereas the amount of 2-OH-PC was just over detection limit (Figure 3). After 60 min of incubation, there was a near three-fold increase in radioactive 0-OH-PC whereas 1-OH-PC, 0-OH-DAG, and 2-OH-DAG remained relatively unchanged. Similar to the 30-min incubation, hardly any radioactivity was found in 2-OH-PC at this time point. Since the castor bean membranes have high lipase activity toward ricinoleoyl-PC (Bafor et al., 1991) it is likely that more 1-OH-DAG was shunted to PC between 30 and 60 min than seen in accumulated radioactivity due to the hydrolysis of the ricinoleoyl group by the phospholipase (Bafor et al., 1991). This can also be true for any radioactive 2-OH-PC formed, but the metabolism of this PC species must then be many magnitudes higher than 1-OH-PC in order to be virtually absent from this lipid. Thus, the results in membranes from castor endosperm support the finding in yeast microsomes expressing castor PDCT that it selects against 2-OH-DAG.
Very little acylation of 0-OH-DAG with 18:1-CoA to form 0-OH-TAG occurred in the castor microsomes whereas the amount of 1-OH-TAG and 2-OH-TAG nearly doubled between 30 and 60 min of incubation. The amount of 3-OH-TAG did not substantially change between these times. We, therefore, performed a 30- and 60-min incubation of castor microsomes with the same amounts of G3P and acyl-CoAs but using [14C]18:1-CoA and [14C]ricinoleoyl-CoA in the presence of nonradioactive G3P and measured the total radioactivity of [14C]18:1 and [14C]ricinoleate found in the chloroform phase (acyl-CoA does not enter that phase during lipid extraction [Stymne and Appelqvist, 1978]). There was a 60% increase in radioactivity in 18:1 between 30 and 60 min whereas the amount of radioactive ricinoleate did not substantially change during that time, demonstrating that all ricinoleoyl-CoA had been consumed during the first 30 min of incubation (Supplemental Figure S3). Since only 18:1-CoA is present between 30 and 60 min of incubation in the [14C]G3P assays, we can conclude that the increase in [14C]TAG seen between 30 and 60-min incubation (Figure 3) was due to acylation of radioactive 1-OH-DAG and 2-OH-DAG with 18:1-CoA and with very little acylation of 0-OH-DAG.
Castor bean PDCT discriminates between sn- positions of the ricinoleoyl groups in 1-OH-DAG
We determined the positional distribution of ricinoleate and nonhydroxy acyl groups in the [14C]glycerol labeled 1-OH-DAG and 1-OH-PC formed in incubations of yeast microsomes expressing the castor PDCT and with mixed 18:3-CoA and ricinoleoyl-CoA, that is, the assays presented in Figure 2C. Ricinoleic acid was found in both sn-positions in both 1-OH-DAG and 1-OH-PC but the ratio of ricinoleate to nonhydroxy fatty acids in each sn-position differed dramatically between the two lipids. Whereas 38% of the acyl groups in sn-2 of 1-OH-DAG was ricinoleate, this acyl groups made up 78% of all acyl groups in sn-2 of 1-OH-PC (Table 1). The results show that de novo synthesized 1-OH-DAG contains ricinoleate in either sn-position but the castor PDCT showed a strong preference for 1-OH-DAG with ricinoleate at the sn-2 position over that lipid with ricinoleate at the sn-1 position.
Proportion of ricinoleate at position sn-2 of [14C]glycerol labeled 1-OH-DAG and DAG derived 1-OH-PC formed from [14C]G3P and a mixture of 18:3-CoA and ricinoleoyl-CoA in yeast membranes expressing castor PDCT
[14C]Glycerol labeled lipid . | Ricinoleate (%) . |
---|---|
Sn-2-1-OH-DAG | 38.4 ± 5.7 |
Sn-2-1-OH-PC | 78.2 ± 2.9 |
[14C]Glycerol labeled lipid . | Ricinoleate (%) . |
---|---|
Sn-2-1-OH-DAG | 38.4 ± 5.7 |
Sn-2-1-OH-PC | 78.2 ± 2.9 |
Assay conditions were identical to those presented in Figure 2C. 1-OH-DAG, DAG with one ricinoleoyl group; 1-OH-PC, PC with one ricinoleoyl group. Average value shown ± sd, n = 3 replicates.
Proportion of ricinoleate at position sn-2 of [14C]glycerol labeled 1-OH-DAG and DAG derived 1-OH-PC formed from [14C]G3P and a mixture of 18:3-CoA and ricinoleoyl-CoA in yeast membranes expressing castor PDCT
[14C]Glycerol labeled lipid . | Ricinoleate (%) . |
---|---|
Sn-2-1-OH-DAG | 38.4 ± 5.7 |
Sn-2-1-OH-PC | 78.2 ± 2.9 |
[14C]Glycerol labeled lipid . | Ricinoleate (%) . |
---|---|
Sn-2-1-OH-DAG | 38.4 ± 5.7 |
Sn-2-1-OH-PC | 78.2 ± 2.9 |
Assay conditions were identical to those presented in Figure 2C. 1-OH-DAG, DAG with one ricinoleoyl group; 1-OH-PC, PC with one ricinoleoyl group. Average value shown ± sd, n = 3 replicates.
Castor DGAT2 substrate specificities and selectivities
The PDCT assays described above showed the selective transfer of 1-OH-DAG over 2-OH-DAG into PC by castor PDCT. In order to understand how castor DGAT2 and castor PDCT interact to increase the amount of ricinoleoyl groups in TAG (Hu et al., 2012), castor DGAT2 was characterized in microsomal preparations of H1246 yeast expressing castor DGAT2.
Since we used racemic 1-OH-DAG and 2-OH-DAG prepared by lipase treatment of 2-OH-TAG from castor seed oil as acyl acceptors in our assays, we first tested if the enzyme could use sn-2,3-DAG. Sn-,1,2-sn-2,3-rac-18:1-DAG was used at half the rate of sn-1,2-18:1-DAG at the same concentration and at the same rate as half that concentration of sn-1,2-DAG (Supplemental Figure S4). Thus, it is likely that castor DGAT2 is specific for the sn-1,2 isomer of DAG. The assays were therefore adjusted to contain the same amount of sn-1,2-DAG substrates.
We measured castor DGAT2 activity with 2-OH-DAG, 1-OH-DAG, 18:1-DAG, and 18:2-DAG as single acyl acceptors and with [14C]ricinoleoyl-CoA, [14C]18:1-CoA, [14C]18:2-CoA, and [14C]18:3-CoA as single acyl donors. The highest activity was found with 2-OH-DAG as acyl acceptor and ricinoleoyl-CoA as the acyl donor (Figure 4). 18:2-CoA and 18:3-CoA were also utilized in acylation of 2-OH-DAG with an activity nearing half of the ricinoleoyl-CoA activity whereas much lower activity was seen with 18:1-CoA (Figure 4A). Ricinoleoyl-CoA was also the best acyl donor when 1-OH-DAG was the acyl acceptor (Figure 4B).
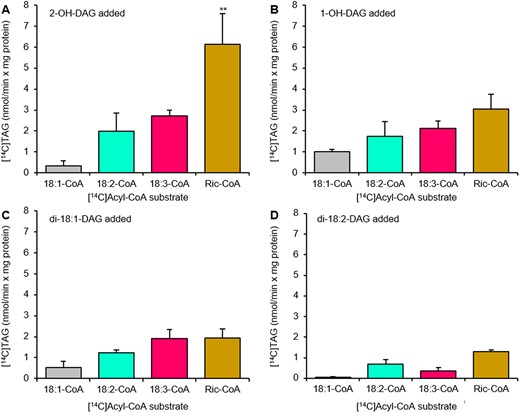
Acyl-CoA specificity of castor DGAT2 with different DAG species. Acyl-CoA specificity of castor DGAT2 was assessed with DAG with two ricinoleoyl groups (2-OH-DAG) (A), one ricinoleoyl group (1-OH-DAG) (B), di-18:1-DAG (C), and di-18:2-DAG (D) as acyl acceptors. The DGAT2 activity was measured in microsomal preparations of yeast strain H1246 expressing the castor DGAT2. Average values shown ± sd, n = 3 replicates. Double (**) asterisks indicate significant difference at P < 0.01 in one-way ANOVA followed by post hoc Tukey’s test.
Di-18:1-DAG and di-18:2-DAG were also tested as acyl acceptors (Figure 4, C and D). They were all poorer acyl acceptors than DAG with ricinoleoyl groups and ricinoleoyl-CoA was not more effective than 18:3-CoA when 18:1-DAG was used as acyl acceptor (Figure 4C). These results, which showed that castor DGAT2 also can produce TAG without ricinoleoyl groups, agree with the demonstration of in vivo TAG synthesis by this enzyme when expressed in Arabidopsis (Regmi et al., 2020).
Although the highest activity was seen with ricinoleoyl-CoA and 2-OH-DAG, yielding 3-OH-TAG, which makes up 70%–80% of the TAG species in castor (Lin et al., 2003; Du et al., 2019), the specificities for nonhydroxylated DAG and acyl-CoA species were substantial. Thus, our DGAT2 results with single acyl acceptors did not explain the very low levels of 0-OH-TAG and 1-OH-TAG seen in castor oil (Lin et al., 2003). We, therefore, performed DAG selectivity assays where either 18:1-DAG or 18:2-DAG were mixed with 1-OH-DAG and 2-OH-DAG in equimolar amounts of sn-1,2-DAG using [14C]ricinoleoyl-CoA as acyl donor (Figure 5). 2-OH-DAG was best utilized in TAG synthesis, regardless if 18:1-DAG or 18:2-DAG was used in the DAG mix (Figures 5, A and B). 1-OH-DAG was acylated at 40% of the rate of 2-OH-DAG. Remarkably, acylation of 18:1-DAG with ricinoleoyl-CoA was only 5% of the rate of acylation of 2-OH-DAG and no acylation of ricinoleoyl-CoA to 18:2-DAG could be detected (Figure 5B).
![Selectivity for DAG species by castor DGAT2 with ricinoleoyl-CoA as acyl donor. Castor DGAT2 selectivity for DAG species was assessed with [14C]ricinoleoyl-CoA as acyl donor together with an equimolar mixture of sn-1,2-DAG composed of di-18:1-DAG, 1-OH-DAG and 2-OH-DAG (A) and di-18:2-DAG, 1-OH-DAG, and 2-OH-DAG (B) as acyl acceptors. The DGAT2 activity was measured in microsomal preparations of yeast strain H1246 expressing the castor DGAT2. 1-OH-TAG, 2-OH-TAG, and 3-OH-TAG designate TAG with no, one, two, and three ricinoleoyl groups. Average values shown ± sd, n = 3 replicates. Single (*) or double (**) asterisks indicate significant difference at P < 0.05 or at P < 0.01, respectively, in one-way ANOVA followed by post hoc Tukey’s test.](https://oup.silverchair-cdn.com/oup/backfile/Content_public/Journal/plphys/189/4/10.1093_plphys_kiac209/3/m_kiac209f5.jpeg?Expires=1750194010&Signature=ZkdWegmT9MGLszzp2uFlgzK0ZXX1401jRi3cNgdDl4ibC80p6sLF4C0JoXWWHVH7aANxv9dW-JNSfVODgLiNIA~cWNQhFQuVSHNCKDhPD0AcI12bkxGfzUf7f2mwVqLYxJ1EgWazFqs4HXX5oFzfnYq2~CzhLeexOpGFrblz6XPh6eaiEI~7EtCsnIVrD2D26DZNmb6V0FiXulA~USv6zNXoTmixfnfrUtw32EwiLxnR8tvyv7xtone9GEbtfi4myUfl0OXBxWTrBRwQvJuNBHPoAHRtpDrXBdgDwrzgl62PCR0F5pZ95iJjpfpK1j4~lCN4hF6MyimUAC4zLJ0wPg__&Key-Pair-Id=APKAIE5G5CRDK6RD3PGA)
Selectivity for DAG species by castor DGAT2 with ricinoleoyl-CoA as acyl donor. Castor DGAT2 selectivity for DAG species was assessed with [14C]ricinoleoyl-CoA as acyl donor together with an equimolar mixture of sn-1,2-DAG composed of di-18:1-DAG, 1-OH-DAG and 2-OH-DAG (A) and di-18:2-DAG, 1-OH-DAG, and 2-OH-DAG (B) as acyl acceptors. The DGAT2 activity was measured in microsomal preparations of yeast strain H1246 expressing the castor DGAT2. 1-OH-TAG, 2-OH-TAG, and 3-OH-TAG designate TAG with no, one, two, and three ricinoleoyl groups. Average values shown ± sd, n = 3 replicates. Single (*) or double (**) asterisks indicate significant difference at P < 0.05 or at P < 0.01, respectively, in one-way ANOVA followed by post hoc Tukey’s test.
We also assayed castor DGAT2 activity in the same DAG mixtures with [14C]18:2-CoA as acyl donor. 2-OH-DAG was preferentially selected for with 1-OH-DAG and 0-OH-DAG both being acylated at ∼20% of that rate (Supplemental Figure S5).
Microsomal DAG from developing castor seeds is mainly composed of DAG lacking ricinoleoyl groups
The above-described PDCT experiments suggest that a proportion of the de novo synthesized 1-OH-DAG is channeled into PC via castor PDCT in castor seeds. Since the ricinoleate content in PC is very low in membranes from castor bean endosperm (Bafor et al., 1991), 1-OH-DAG would therefore be mainly exchanged for DAG with no ricinoleoyl groups. We showed that castor DGAT2 virtually excludes acylation of nonhydroxylated DAG in the presence of competing ricinoleoyl-DAG (Figure 5) which is also seen in our experiment with microsomal preparations from developing castor bean endosperm (Figure 3). Our in vitro results thus suggest that the combined action of PDCT and DGAT2 in castor endosperm would lead to a pool of nonhydroxylated DAG not utilized in TAG synthesis and arising from PC by exchange with 1-OH-DAG catalyzed by PDCT. We, therefore, analyzed the composition of the DAG pool in microsomal membranes prepared from developing castor seeds. By optimizing the separation conditions on TLC, two clearly separated 0-OH-DAG bands could be seen, one running slightly above di-18:1-DAG standard and one slightly below (Figure 6A). Quantification showed that the overwhelming part of all DAG species was the 0-OH-DAG (85%) with 1-OH-DAG at 13% and 2-OH-DAG at 2% (Figure 6B). The upper, minor 0-OH-DAG band had a fatty acid composition strongly diverting from PC by being composed of 50% saturated fatty acids (16:0 and 18:0) and 36% 18:1 whereas the lower 0-OH-DAG band had an acyl composition similar to microsomal PC (Figure 7).
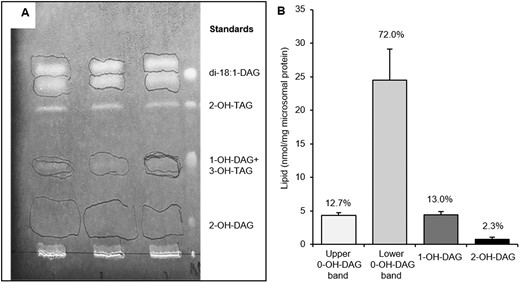
Separation and quantification of DAG species in microsomal preparations of castor bean endosperm. A, TLC separation of DAG species in microsomal preparation of developing castor endosperm visualized in UV-light after sprayed with primuline. Areas containing the separated DAG species are encircled with pencil and were removed for analysis of acyl composition and quantity. B, The amounts of separated DAG species from (A) as determined by GC. The relative contribution (%) of each DAG species is indicated in the figure. 0-OH-DAG, 1-OH-DAG, and 2 OH-DAG designate DAG with no, one and two ricinoleoyl groups respectively. Average values shown ± sd, n = 3 replicates.
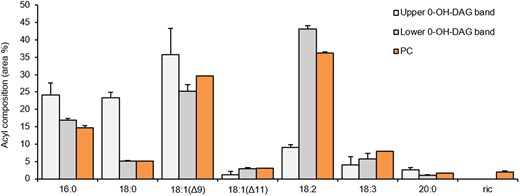
Acyl composition of PC and two TLC separated 0-OH-DAG bands in microsomal preparations from developing castor bean endosperm. The PC species and 0-OH-DAG species were separated in two different TLC system, in situ methylated, and the acyl composition determined by GC. The two separated 0-OH-DAG bands were from TLC plate presented in Figure 6A. 0-OH-DAG designate DAG with no ricinoleoyl group. Average values shown ± sd, n = 3 replicates.
We determined how much 0-OH-DAG the castor oil contained by using a TLC system that separated the DAG from the 2-OH-TAG. We found that 0-OH-DAG was ∼4.27 mol% of the 2-OH-TAG (Supplemental Table S1). Since 2-OH-TAG species make up ∼20% of the total oil (Lin et al., 2003; Du et al., 2019), the 0-OH-DAG content made up ∼0.85 mol% of total oil, which is six times more than the 2-OH-DAG reported by Lin et al. (2003).
The positional distribution of nonhydroxylated acyl groups in 2-OH-TAG in castor oil
Lin and Arcinas (2007) reported the positional location of nonhydroxylated acyl chain in 2-OH-TAG from castor oil using electrospray ionization-MS3 of lithium adducts. It was reported that over 90% of 18:1, 18:2, and 18:3 were located in the sn-2 position and 78% of the 16:0 was also found in this position. However, when preparing 1-OH-DAG and 2-OH-DAG for standards by treating 2-OH-TAG from castor oil with TAG lipase from Rhizomucor miehei, we found a somewhat higher amount of 2-OH-DAG formed than 1-OH-DAG, which is not expected if the nonhydroxylated acyl group was mainly located at the sn-2 position. We, therefore, measured lipid products produced by the TAG lipase from 2-OH-TAG (Figure 8A). The results showed that 78% of the monoacylglycerol (MAG) produced contained a ricinoleoyl group. This will mean that the sn-1 + sn-3 positions will contain 39% of nonhydroxylated acyl groups, that is, 78% of the nonhydroxylated acyl groups in 2-OH-TAG. If the lipase had a very strong selectivity for nonhydroxylated acyl groups over ricinoleoyl groups, this could partly explain the deviation of our results from the published results (Lin and Arcinas, 2007). We therefore lipase treated an equimolar mixture of tri-18:1-TAG and tri-ricinoleoyl-TAG and found that there were no substantial differences in the production of DAG and MAG between the two substrates (Figure 8B). Thus, the lipase used was completely nondiscriminatory for hydroxylated and nonhydroxylated acyl groups. We are not experts in MS re-arrangements with lithium adducts to be able to find errors in the published results (Lin and Arcinas, 2007) but our lipase experiments suggest that these earlier results need to be reexamined.

Positional distribution of nonhydroxylated fatty acids in 2-OH-TAG from castor oil and the nondiscrimative nature of the TAG lipase. A, Lipid products from 2-OH-TAG from castor oil treated with lipase from R. miehei. B, Lipid products from an equimolar mixture of tri-18:1-TAG and 3-OH-TAG treated with lipase from R. miehei The 2-OH-TAG and 3-OH-TAG were first isolated from castor oil by TLC, eluted and quantified by GC before used for lipase assays. 0-OH, 1-OH, 2-OH, and 3-OH indicate the absence of ricinoleoyl groups, 1 ricinoleoyl group, 2 ricinoleoyl groups, and 3 ricinoleoyl groups, respectively, in TAG, DAG, and MAG. Average values shown ± sd, n = 3 replicates from the same lipase-treated sample.
Ricinoleoyl groups at sn-1 position of PC is efficiently removed in castor bean microsomes
We found that 43% of the acyl groups in the sn-1 + sn-3 positions of 2-OH-TAG are nonhydroxylated, but we do not know the distribution of these between the sn-positions. Our DGAT2 assays suggest that ricinoleoyl-CoA is the preferred acyl donor in acylating both 1-OH-DAG and 2-OH-DAG. It is, therefore, likely that most of 1-OH-DAG molecules that are de novo synthesized have the nonhydroxylated acyl group in the sn-1 position. We found that castor PDCT much prefers that stereoisomer of 1-OH-DAG, although 1-OH-DAG with ricinoleate at sn-1 is still used to some extent (Table 1). Since sn-2 position of 2-OH-TAG has 14% of nonhydroxylated acyl groups, the same percentage of 2-OH-TAG formed must be derived from de novo DAG with the nonhydroxylated acyl group in sn-2 position. Accordingly, ricinoleate might be in either sn-positions of PC when 1-OH-DAG is interconverted with PC. If present in sn-2 position, the ricinoleoyl group is specifically removed and used for TAG synthesis, either by a castor bean lipase (Bafor et al., 1991), PDAT (Dahlqvist et al., 2000), or reverse action of LPCAT (Lager et al., 2013). In order to investigate if ricinoleoyl group at sn-1 position of PC is also specifically removed by castor enzymes, we incubated microsomal fractions from developing castor endosperm with [14C]18:1-CoA and either sn-1-18:1-LPC or sn-1-ricinoleoyl-LPC. About 70% of the added [14C]18:1-CoA was acylated by castor LPCAT to yield sn-1-18:1-sn-2-[14C]18:1-PC. Ricinoleoyl-LPC was a poorer acyl acceptor for the castor LPCAT with only 45% of the added [14C]18:1-CoA acylated to ricinoleoyl-LPC and with 40% incorporated into PC by acylation of endogenous LPC or by reverse LPCAT reaction (Lager et al., 2013; Figure 9). Of the PC formed from 18:1-LPC, 1% was converted to sn-2-[14C]18:1-LPC within 30 min whereas 43% of the sn-1-ricinoleoyl-sn-2[14C]18:1-PC was converted to sn-2-[14C]18:1-LPC during that period. Thus, castor endosperm has efficient systems to specifically remove ricinoleoyl groups also from position sn-1 of PC, which might be the same mechanisms that previously have been shown to operate on position sn-2 of PC (Bafor et al., 1991; Dahlqvist et al. 2000; Lager et al., 2013).
![Distribution of [14C]18:1 in PC and LPC after acylation with added sn-1-18:1-LPC or sn-1-ricinoleoyl-LPC in microsomal preparations from developing castor bean endosperm. Castor microsomal preparations (150 µg of microsomal protein) were incubated with 15 nmol of either sn-1-18:1-LPC or 15 nmol sn-1-ricinoleoyl-LPC (ric-LPC) and 12 nmol of [14C]18:1-CoA as indicated in the figure for 30 min. 0-OH-PC and 1-OH-PC designate phsophatidylcholine with no and one ricinoleoyl group, respectively; LPC, lysophosphatidylcholine. Average values shown ± sd, n = 3 replicates.](https://oup.silverchair-cdn.com/oup/backfile/Content_public/Journal/plphys/189/4/10.1093_plphys_kiac209/3/m_kiac209f9.jpeg?Expires=1750194010&Signature=ZghMyT6ozawUJqzFntxPigZUotVwy2hu6c8Dh2CJbkOestYbpJYFsB2po5m5xHLf9Wa3KQajvIfI6teWkFxiZjrqr0~ej4cL9anm6U6FN9~NPB3egOTLiYnz7zm5lHVUdPG4bMG0j8uxk5LdRiqXAbJ4uouRWyXLJ3yIIymDbG12Y-xGCOb5js-g-p5lXih7Z3jHyv~7DJf60arctO~h84TwTni63ykBkeKhJezSwmE8gumHuPy6S3YI7xK-gjOj8jaox5ZQyjAaTuxTVo3MvGmZbza43haZWUJT-PReR6Mtwx7uPwd7HODKBEjB0XR7DGnChJBt7dndk~r6IfcJTg__&Key-Pair-Id=APKAIE5G5CRDK6RD3PGA)
Distribution of [14C]18:1 in PC and LPC after acylation with added sn-1-18:1-LPC or sn-1-ricinoleoyl-LPC in microsomal preparations from developing castor bean endosperm. Castor microsomal preparations (150 µg of microsomal protein) were incubated with 15 nmol of either sn-1-18:1-LPC or 15 nmol sn-1-ricinoleoyl-LPC (ric-LPC) and 12 nmol of [14C]18:1-CoA as indicated in the figure for 30 min. 0-OH-PC and 1-OH-PC designate phsophatidylcholine with no and one ricinoleoyl group, respectively; LPC, lysophosphatidylcholine. Average values shown ± sd, n = 3 replicates.
Discussion
Castor PDCT utilizes de novo formed ricinoleoyl-containing DAGs and selects against 2-OH-DAG
In-line with previous results (Lager et al., 2020), de novo synthesized DAG from [14C]G3P and 18:3-CoA was readily utilized by Camelina PDCT in microsomal preparations of yeast H1246 expressing the enzyme whereas ricinoleoyl-containing DAG was hardly used and inhibited the utilization of 18:3-DAG. Castor PDCT, on the other hand, used ricinoleoyl-containing DAG species as efficiently as 18:3-DAG. It is particularly notable that the distribution of radioactive PC species with no, one or two ricinoleoyl groups deviated strongly from the distribution of radioactive DAG species in assays with a mix of 18:3-CoA and ricinoleoyl-CoA. The castor PDCT showed an over 10-fold selectivity for DAG species with one ricinoleoyl group over DAG species with two hydroxy acyl groups. Similar to [14C]G3P experiments in yeast, microsomal preparations from developing castor endosperm confirmed the strong selection against 2-OH-DAG in the castor PDCT reaction.
Expression of the castor PDCT in Arabidopsis expressing the castor hydroxylase increased both the amount of ricinoleoyl groups in TAG and the total TAG content in Arabidopsis seeds (Hu et al., 2012). Bates and Browse (2012) found a 70% reduction in the flux of DAG into PC, a catabolism of ricinoleoyl-containing DAG, and a decreased oil content in Arabidopsis seeds expressing the castor hydroxylase compared with control plants. It is likely that the increased amount of ricinoleoyl groups in TAG and restored oil content seen in Arabidopsis expressing the castor PDCT together with the hydroxylase (Hu et al., 2012) is mainly due to the ability of castor PDCT to use ricinoleoyl-containing DAG as well as DAG without these acyl groups, in contrast to PDCT from Arabidopsis and Camelina.
Castor DGAT2 shows an extreme selectivity for ricinoleoyl-containing DAG species
It can be assumed that acylation of nonhydroxylated DAG is minute in castor bean since only 0.28% of all TAG species in castor seeds have one ricinoleoyl group and 0.02% TAG are without ricinoleoyl groups (Lin et al., 2003). The amount of ricinoleoyl groups in castor PC is very low (Figure 7), due to its rapid removal after synthesis. We can postulate from our in vitro experiments in yeast membranes that castor PDCT will preferentially channel de novo synthesized 1-OH-DAG into PC in exchange for DAG with no ricinoleoyl groups. Thus, the resulting DAG pool should be enriched with nonhydroxylated DAG in castor endosperm. DGAT2 is strongly expressed during castor seed development and has been shown to substantially increase the amount of hydroxylated acyl groups in seed TAG when expressed in Arabidopsis together with the castor Δ12 hydroxylase (Burgal et al., 2008). Our selectivity studies of castor DGAT2 showed that DAG with no ricinoleoyl groups (either di-18:1-DAG or di-18:2-DAG) were, more or less, excluded from acylation with ricinoleoyl groups in the presence of ricinoleoyl-containing DAG. Thus, selectivity data suggest that when ricinoleoyl-CoA is the acyl donor, the acylation of DAG with no ricinoleoyl groups is very low. Our [14C[G3P assays using microsomal fraction of developing castor endosperm showed similar acylation of radioactive 1-OH-DAG and 2-OH-DAG, but not 0-OH-DAG, with 18:1-CoA.
0-OH-DAG dominate the DAG pool in microsomal membranes from developing castor endosperm
Our results suggest that castor PDCT decreases the amount of DAG with one ricinoleoyl group by selective transfer to PC by PDCT. The ricinoleoyl group of this PC could then be utilized by PDAT to form TAG (Dahlqvist et al., 2000) or recycled into the acyl-CoA pool (Lager et al., 2013) to be re-utilized by the G3P pathway to synthesize primarily DAG with two ricinoleoyl groups. However, in such a scenario the DAG pool derived from PC through the action of PDCT would be enriched with species with no ricinoleoyl groups. We therefore analyzed the composition of the endogenous DAG pool in microsomal preparations from developing castor seeds. By optimizing the TLC separation method, microsomal 0-OH-DAG separated into two bands. The results showed that the overwhelming part of all DAG species (85%) had no ricinoleoyl groups. The upper band of 0-OH-DAG had a fatty acid composition strongly diverting from the lower band with 50% of saturated acyl groups (16:0 and 18:0) and 36% of 18:1 whereas the lower band had a fatty acid composition similar to microsomal PC. DAG with one ricinoleoyl group made up 13% of the total DAGs whereas DAG with two ricinoleoyl groups constituted only 2%. Since TAG with three ricinoleoyl groups made up ∼80% of all TAG species in castor oil (Lin et al., 2003) also 80% of all DAG species that were utilized in TAG synthesis must have been DAG with two ricinoleoyl groups. The very low levels of 2-OH-DAG species in microsomal membranes of castor endosperm indicate a near complete removal of them by acylation catalyzed by castor DGAT and PDAT. The very high amount of DAG with no ricinoleoyl groups in microsomes from castor endosperm and the very low amount (less than half a percentage) of TAG with one or no ricinoleoyl group in castor oil (Lin et al., 2003) indicate that acylation of such DAG species by castor DGAT2 (and PDAT) hardly occurs, which is also corroborated by our DGAT2 selectivity results as well as the [14C]G3P experiment in microsomal fractions of castor endosperm.
Although the lower 0-OH-DAG band is likely to be derived from PC by interconversion of mainly 1-OH-DAG catalyzed by PDCT, we can only speculate about the origin of the upper 0-OH-DAG band. That band had ∼50% of 16:0 and18:0 in about equal amounts, suggesting that the sn-1 position is only containing these fatty acids with 18:1 making up ∼70% of the acyl groups at position sn-2. No known membrane lipids have similar acyl composition and thus it is unlikely that this DAG is derived from any of them. We suggest that this DAG species is derived from de novo synthesis.
It is apparent from the TAG species composition of castor oil that 0-OH-DAG derived from PC through exchange with 1-OH-DAG and via (assumed) de novo synthesis is not utilized in TAG synthesis. Therefore, it would be expected to increase throughout the period of oil deposition in the endosperm. We note that the levels of total DAG species in the microsomes from developing castor endosperm were not higher than in other microsomal membranes, such as in yeast (Demski et al., 2021). When analyzing the 0-OH-DAG content in castor oil, we found it to constitute ∼0.85 mol % of all lipid species. Although this is the third most abundant lipid after 3-OH-TAG and 2-OH-TAG in castor oil (Lin et al., 2003), much more 0-OH-DAG would be expected to be formed if PDCT, as we propose, plays a substantial role in decreasing the amount of 2-OH-TAG. Some of the 0-OH-DAGs are likely to be utilized in membrane lipid synthesis during oil deposition but we do not exclude that there are mechanisms that trigger their catabolism when exceeding a certain level in the membranes.
Positional location of nonhydroxylated acyl groups in 2-OH-TAG is very different from previously reported
In sharp contrast to previous publications (Lin and Arcinas, 2007), we found that the nonhydroxylated acyl group was up to 78% located in the sn-1 + sn-3 positions of 2-OH-TAG and thus making up around 39% of total acyl groups in these two positions. Before a complete stereo-specific determination of 2-OH-TAG is done, we cannot with certainty say how much of this is nonhydroxylated acyl groups in position sn-1. It should be noted that the only published method for total stereospecific analysis of TAG (Brockerhoff, 1965) cannot be used for DAG-containing hydroxylated acyl groups due to derivatization of the hydroxy group in the acyl chain. Our castor DGAT2 assays strongly indicate that sn-3 position is primary acylated with ricinoleoyl groups. Expression of castor PDAT in Arabidopsis together with expression of castor GPAT9, castor LPAT2, and the Δ12 hydroxylase indicate that also castor PDAT has a strong selectivity for ricinoleoyl groups in the acylation of the sn-3 position (Lunn et al., 2019). Thus, it is reasonable to assume that most of the nonhydroxylated acyl groups are in the sn-1 and not sn-3 position of 2-OH-TAG and thus produced from 1-OH-DAG with nonhydroxylated acyl groups in sn-1 position. Analyses of the positional distribution of the ricinoleoyl and nonhydroxylated acyl group in 1-OH-DAG entering PC in assays with castor PDCT in yeast microsomal membranes suggest that the castor PDCT has a strong preference for 1-OH-DAG with nonhydroxylated acyl groups in sn-1 position, that is, the assumed major 1-OH-DAG species produced in castor bean. Since we found that 22% of the sn-2 position of 2-OH-TAG is nonhydroxylated acyl groups, a minor amount of de novo produced 1-OH-DAG and DAG derived 1-OH-PC will have the ricinoleate in the sn-1 position. We showed that castor bean microsomal fractions efficiently remove ricinoleate also from the sn-1 position of PC.
Enrichment of ricinoleoyl groups by the concerted action of PDCT and DGAT2 in castor seeds
Based on the biochemical properties of castor PDCT and DGAT2, analysis of DAG species in microsomal preparations from developing castor seeds, the distribution of ricinoleoyl groups in 2-OH-TAG in castor, and published composition of castor TAG species (Lin et al., 2003; Du et al., 2019) a biosynthetic scheme for TAG synthesis in castor seeds emerges as depicted in Figure 10.
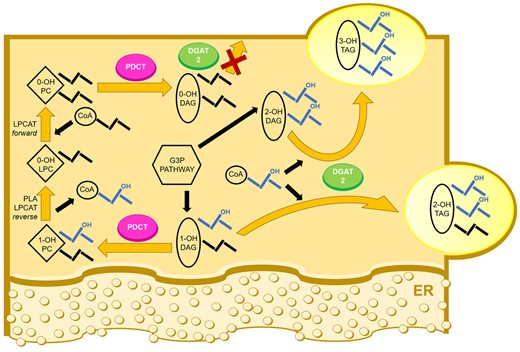
Castor PDCT and DGAT2 working in concert to enhance tri-ricinoleoyl glycerol amount in castor seeds. Proposed acyl flow in TAG synthetic pathway in castor endosperm. De novo synthesis of DAG via G3P pathway gives rise to DAG with two (2-OH-DAG) and one (1-OH-DAG) ricinoleoyl groups (mainly located in the sn-2 position). 1-OH-DAG is preferentially exchanged with PC with no ricinoleoyl groups (0-OH-PC) by PDCT. The ricinoleoyl group in 1-OH-PC derived from DAG is rapidly replaced with a nonhydroxylated acyl group and the ricinoleate is recycled in the G3P pathway to mainly yield 2-OH-DAG or used by PDAT to form 3-OH-TAG. Ricinoleoyl-containing DAG is mainly acylated with ricinoleoyl groups by DGAT2. However, DAG with no ricinoleoyl groups (0-OH-DAG) is strongly selected against in presence of DAG with ricinoleoyl groups. Thus, a DAG pool with acyl composition similar to PC and not used in TAG synthesis replaces the 1-OH-DAG shunted to PC and can be used in membrane lipid synthesis. PDAT reaction is not depicted in the figure but can be predicted to have the same high selectivity for ricinoleoyl-containing substrates as DGAT2 (Dahlqvist et al., 2000; Lunn et al. 2019). ER, endoplasmic reticulum.
No selectivity studies have been done with castor PDAT but the expression of castor PDAT together with castor GPAT9, LPAT2, and the hydroxylase suggests that castor PDAT has high selectivity for ricinoleoyl-PC over PC with other acyl groups and, similar to DGAT2, selects DAG with ricinoleoyl groups as acyl acceptors (Lunn et al., 2019).
Conclusion
Many plant species produce high amount of unusual fatty acids in their seed oil (Ohlrogge et al., 2018) and in most cases, these are not present in membrane lipids of the plant or in other tissues than the seed TAG. It is now evident that these plants not only have developed enzymes producing these unusual fatty acids but also a number of auxiliary enzymes which have been modified to efficiently channel these acids into TAGs (Cahoon and Li-Beisson, 2020). Castor bean is a case in point having ∼90% of ricinoleate in its seed oil. Castor GPAT9, LPAT2, DGAT2, PDAT, and PDCT have all shown to increase the amount of ricinoleate in seed TAG in transgenic Arabidopsis expressing the castor Δ12 oleate hydroxylase (Burgal et al., 2008; van Erp et al., 2011; Hu et al., 2012; Lunn et al., 2019). In our study, we show that castor PDCT has another function than to provide acyl groups produced on PC to DAG for further acylation to TAG (Bates et al., 2013). We have shown that castor PDCT specifically transfers 1-OH-DAG to PC in exchange for 0-OH-DAG. Due to extreme selectivity for DAG with ricinoleoyl groups, castor DGAT2 (and most likely PDAT) excludes acylation of 0-OH-DAG, which build up in the membranes.
Castor bean DGAT2 is extreme in its selectivity in the acylation of certain DAG species, which has drastic effects on the acyl composition of the TAG molecules synthesized. We have earlier shown that both DGATs and PDAT from Camelina can have vastly different specificities for different DAG species (Lager et al., 2020). In vivo radioactive labeling studies in Arabidopsis seeds concur that PC-derived DAG is a separate pool from the de novo synthesized DAG (Bates and Browse, 2012) and that different DAG pools are utilized differently by PDAT, DGAT1, and DGAT2 (Regmi et al., 2020). The hypothesized different DAG pools might partly, or entirely, be explained by different DAG selectivities by the DAG-acylating enzymes.
Materials and methods
Chemicals
Nonradioactive fatty acids, BSA (essentially fatty acid free), G3P, phospholipase C (from Clostridium perfringens), TAG lipase (from R.miehei), phospholipase A2 (from Naja mossambica), ricinoleic acid, sn-1,2-di-18:1-DAG, CoA, sn-1-18:1-LPC, and glycerophosphocholine (GPC) were obtained from Sigma Aldrich (St. Louis, MO, USA). [14C]18:1 and [14C]G3P were purchased from Perkin Elmer, [14C]ricinoleic acids were obtained from American Radiolabeled Chemicals and tri-oleoylglycerol was purchased from Larodan (Stockholm). Acyl-CoA was synthesized in a modification of the method of Sanchez et al. (1973). Sn-1,2-sn-2,3-rac-DAG with one or two ricinoleoyl groups were obtained from 2-OH-TAG from castor bean (R.communis) oil by treatment with TAG lipase and separation of resulting products TLC plates in heptane:diethyl ether:acetic acid (20:50:0.5 by vol.). Ricinoleoyl-LPC was synthesized enzymatically by acylation of GPC with ricinoleoyl-CoA in microsomes of yeast (S.cerevisiae) mutated in ALE1 (lysophospholipid acyltransferase, YOR175C) and overexpressing the GPC1 (YGR149W) gene (yeast GPC acyltransferase, GPCAT) (Glab et al., 2016). The lipids were extracted into chloroform and LPC separated from other lipids on TLC in chloroform:methanol:acetic acid:water (85:15:10:3.5 by vol.).
Gene constructs, yeast transformation, and microsomal preparation
Camelina (C.sativa) PDCT and yeast vector were designed as described by Lager et al. (2020). Castor bean PDCT and DGAT2 were S.cerevisiae codon-optimized and synthesized synthetically. Both genes were processed utilizing Gateway Cloning and finally expressed in binary vector Gateway pYES-DEST52 under the GAL1 (galactokinase, YBR020W) promoter in plasmids containing URA3 (orotidine-5′-phosphate decarboxylase, YEL021W) marker for yeast selection. Yeast transformations of TAG-deficient H1246 yeast strain (MATα are1-Δ::HIS3 are2-Δ::LEU2 dga1-Δ::KanMX4 Iro-Δ::TRP1 ADE2 ura3) (Sandager et al., 2002) and subsequent microsomal preparations were carried out as described by Jeppson et al. (2020). Yeast mutated in ale1 and overexpressing GPC1 and microsomal preparations of this yeast were produced as described by Glab et al. (2016). Microsomal preparations from developing castor bean endosperm were done as described by Bafor et al. (1991).
Enzyme assays
PDCT was assayed in microsomal preparations of PDCT/transformed yeast H1246 (100 µg microsomal protein) with de novo synthesized [14C]DAGs derived from the [14C]G3P and acyl-CoA as described by Lager et al. (2020). Incubations were performed at 30°C with shaking (1,250 rpm) for 60 min if not otherwise indicated in figures. Radioactive lipid formation from [14C]G3P and acyl-CoA in microsomal preparations from developing castor endosperm was performed similarly to the yeast membrane assays but with 150 µg of microsomal protein, 12.5 nmol [14C]G3P, 12.5 nmol 18:1-CoA, and 12.5 nmol of ricinoleoyl-CoA.
Castor DGAT2 activity was assayed in H1246 yeast expressing the enzyme with nonradioactive DAG added in DMSO and [14C]acyl-CoA according to method described by Jeppson et al. (2019). Background assessment was performed utilizing the same reaction components and parameters, without the addition of exogenous DAG (Supplemental Table S2). The detected background was subtracted from the presented results. Description of statistical analysis and number of replicates can be found in the figure legends.
Lipid analyses
Enzyme assays were terminated by extracting the lipids into chloroform according to Bligh and Dyer (1959). The amount of radioactivity in chloroform soluble lipids was determined by liquid scintillation of an aliquot of the chloroform phase before TLC separation of the remaining lipids. The relative distribution of radioactivity in individual lipids on the TLC plates (silica gel 60) was determined in either of two ways; by electronic autoradiography using Instant Imager (Canberra Packard) or by applying standards to the sample application spot on TLC, staining the lipids with iodine and marking relevant lipid areas with a pencil. After heating the TLC plate to evaporate the iodine, the pencil-marked lipid areas were removed as well as the rest of the sample lane, and the gel fractions were counted by liquid scintillation.
Methylation of lipids was performed with 2% v/v sulfuric acid in methanol. GC analysis of fatty acid methyl esters was performed on a CP-wax 58 (FFAP-CB) column using an Agilent Technologies 8860 gas chromatograph and quantified with 17:0 methyl ester as internal standard.
The TLC plate with lipid extracts from the yeast PDCT assays was developed to half its height in chloroform:methanol:acetic acid:water (85:15:10:3.5 by vol.), then dried and fully developed in heptane:diethylether:acetic acid (70:50:1 by vol.).
In incubations with [14C]G3P and acyl-CoA in castor bean microsomes, the lipid extracts were divided into three parts. Two parts were separated on TLC using chloroform:methanol:acetic acid:water (85:15:10:3.5 by vol.) with standard PC and PA added. When the relative distribution of radioactivity in PA and PC were to be determined, the PA and PC areas as well as the rest of the lane were removed for scintillation counting as described above. Neutral lipids were separated from one-third of lipid extract by TLC with standard 0-OH-TAG, 1-OH-TAG, 2-OH-TAG, 3-OH-TAG, di-18:1-DAG, 1-OH-DAG, and 2-OH-DAG applied on the sample application spot. The plate was developed in heptane:diethyl ether: acetic acid (60:70:1 by vol.) to its full length, dried, and re-developed to its full length in the same solvent. This separated all the standard lipids from each other and radioactivity in the sample lipids was determined by liquid scintillation as described above.
When the distribution of ricinoleoyl groups in PC was to be determined, PC was located either by Instant Imager and eluted or the TLC plate was sprayed with primuline, viewed under UV light, and PC area was marked with a pencil and eluted from the gel. The isolated PC was treated with phospholipase C to yield DAG. The DAG species were separated on silica gel 60 TLC plates developed in heptane:diethyl ether:acetic acid (40:60:1 by vol.) and distribution of radioactivity in 0-OH-DAG, 1-OH-DAG, and 2-OH-DAG was either determined by liquid scintillation or by Instant Imager.
The chloroform extracts from the DGAT2 assays were separated on TLC plate developed in heptane:diethyl ether:acetic acid (20:50:0.5 by vol.) to its full length and then dried and re-developed with the same solvent mix. To identify the TAG species, nonradioactive reference standards composed of TAG with no ricinoleoyl groups, castor oil, and ricinoleic acid were used and visualized with iodine after determining their relative radioactivity with Instant Imager.
Lipids in microsomal fractions prepared from developing castor bean endosperm were extracted according to Bligh and Dyer (1959). The lipid extracts were applied on silica 60 TLC plates in chloroform:methanol:acetic acid: water (85:15:10:3.5 by vol.) to separate out the PC. Neutral lipids from microsomal fractions of castor endosperm were separated by TLC in heptane:diethyl ether:acetic acid (80:70:0.5 by vol.) by developing the plate to its full length, then drying the plate and redeveloping it in the same solvent mixture. This resolved 0-OH-DAG, 1-OH-DAG, and 2-OH-DAG from each other and separated 0-OH-DAG into two bands. The DAG species was located in UV light after spraying the plate with primuline, gel areas were removed and the lipids methylated in situ. The 1-OH-DAG partially overlapped with 3-OH-TAG (see Figure 6A). However correct quantification of 1-OH-DAG by GC could be done by ignoring the ricinoleate and multiplying the amount of nonhydroyxlated acyl groups with a factor of 2.
Positional analyses of lipids
Castor oil was separated on TLC developed in heptane:diethyl ether:acetic acid (20:50:0.5 by vol.). This separated out 1-OH-TAG, 2-OH-TAG, and 3-OH-TAG from each other. The lipids were visualized with primuline and eluted from the gel to be used as standards and their identity confirmed by GC on the basis of their ricinoleoyl content.
2-OH-TAG (1 µmol) was dissolved in 0.2 mL of diethyl ether, 1 mL of 0.1 M Tris–HCl buffer, pH 7.4 containing 5 mM CaCl2, and 200 units of R.miehei lipase was added and the solution was vigorously agitated in room temperature for 5 min. The lipids were extracted into chloroform according to Bligh and Dyer (1959) and separated on TLC in heptane:diethyl ether:acetic acid (20:50:0.5 by vol.) which separated out 1-OH-DAG, 2-OH-DAG, MAG, and OH-MAG which were either eluted to be used as standards or in situ methylated for GC analysis. To check possible specificity bias of the lipase toward ricinoleoyl groups, 2 µmol of tri-18:1-TAG and 2 µmol of 3-OH-TAG were mixed and treated as above with 400 units lipase for 10 min.
Two chloroform extracts from assays with yeast microsomes expressing castor PDCT incubated with mixed 18:3-CoA, ricinoleoyl-CoA, and [14C]G3P were pooled and separated on TLC as described previously with 30 nmol unlabeled 1-OH-DAG and 30 nmol unlabeled 18:1-PC added to the sample application spot. The PC was converted to DAG by phospholipase C and separated on the basis of its ricinoleoyl content as described previously. The 1-OH-DAG derived from PC and 1-OH-DAG formed in the assays were mixed with 1 µmol of di-18:1-DAG and 0.5 µmol of 2-OH-DAG and treated with 200 units of TAG lipase for 3 min after which the lipids were extracted into chloroform and separated on TLC in heptane:diethyl ether:acetic acid (20:50:0.5 by vol.) with 0-OH-MAG and 1-OH-MAG added as reference substances. Radioactivity in 0-OH-MAG and 1-OH-MAG was determined by liquid scintillation to give the proportion of radioactive nonhydroxylated groups and ricinoleoyl groups in sn-2 position of the [14C]glycerol labeled 1-OH-DAG and 1-OH-PC formed in the assays.
Accession numbers
Sequence data from this article can be found under the following accession numbers in the listed data libraries: RcPDCT—XM_002517597.3 (NCBI), RcDGAT2—AY916129.2 (GenBank), CsPDCT—MT130513.1 (GenBank).
Supplemental data
The following materials are available in the online version of this article.
Supplemental Figure S1. Time-course accumulation of radioactive lipids from [14C]G3P and 18:3-CoA in microsomal preparations of yeast strain H1246 expressing Camelina PDCT.
Supplemental Figure S2. Formation of radioactive lipids from [14C]G3P and acyl-CoA in microsomal preparations from H1246 cells transformed with empty vector.
Supplemental Figure S3. Accumulation of [14C]18:1 and [14C]ricinoleate in the chloroform phase from assays with nonradioactive glycerol 3-phosphate and a 1:1 mixture of [14C]18:1-CoA and [14C]ricinoleoyl-CoA in microsomal preparations of developing castor bean membranes.
Supplemental Figure S4. Castor DGAT2 is specific for sn-1,2 enantiomer of DAG.
Supplemental Figure S5. Selectivity for DAG species by castor DGAT2 with 18:2-CoA as acyl donor.
Supplemental Table S1. Amounts of DAG with no hydroxylated acyl groups (0-OH-DAG) relative to TAG with two ricinoleoyl groups (2-OH-TAG) in castor bean oil.
Supplemental Table S2. Background radioactivity (nmol/min × mg protein) co-localizing with 3-OH-TAG and 2-OH-TAG areas on TLC from assays of yeast microsomal fractions expressing DGAT2 without the addition of exogenous DAG.
Funding
This work was supported by the Swedish Foundation for Strategic Research and The Swedish Research Council for Environment, Agriculture and Spatial Planning (FORMAS) Grant 2018-01579.
Conflict of interest statement. The authors declare no conflict of interest.
K.D., S.J., S.S., and I.L. conceived the project and were all involved in the experimental work. S.S. wrote most of the manuscript with contributions from all other authors. All authors agreed to submit the manuscript for publication.
The author responsible for distribution of materials integral to the findings presented in this article in accordance with the policy described in the Instructions for Authors (https://dbpia.nl.go.kr/plphys/pages/general-instructions) is: Ida Lager ([email protected]).
References
Author notes
Senior author