-
PDF
- Split View
-
Views
-
Cite
Cite
Yankai Liu, Xiao-Wei Zhang, Xin Liu, Peng-Fei Zheng, Ling Su, Gui-Luan Wang, Xiao-Fei Wang, Yuan-Yuan Li, Chun-Xiang You, Jian-Ping An, Phytochrome interacting factor MdPIF7 modulates anthocyanin biosynthesis and hypocotyl growth in apple, Plant Physiology, Volume 188, Issue 4, April 2022, Pages 2342–2363, https://doi.org/10.1093/plphys/kiab605
- Share Icon Share
Abstract
Light affects many physiological and developmental processes of plants by regulating the expression and activity of light-responsive proteins. Among them, phytochrome interacting factors (PIFs) play pivotal roles in the regulation of anthocyanin accumulation and hypocotyl growth. However, the molecular mechanism is not well understood, especially in woody plants, such as apple (Malus × domestica). In this study, we identified a light-responsive PIF protein, MdPIF7, in apple and investigated the molecular mechanism of its regulation of anthocyanin biosynthesis and hypocotyl growth. We found that overexpression of MdPIF7 decreased anthocyanin accumulation in transgenic apple materials and promoted hypocotyl elongation in ectopically expressed Arabidopsis (Arabidopsis thaliana). Further investigation showed that MdPIF7 functioned by interacting with B-box 23 (MdBBX23), a positive regulator of anthocyanin biosynthesis in apple and hypocotyl growth inhibition in ectopically expressed Arabidopsis, and attenuating the transcriptional activation of MdBBX23 on LONG HYPOCOTYL 5 (MdHY5). In addition, MdPIF7 interacted with basic region leucine zipper 44 (MdbZIP44) and ethylene response factor 38 (MdERF38), two positive regulators of anthocyanin biosynthesis, and it negatively regulated MdbZIP44- and MdERF38-promoted anthocyanin accumulation by interfering with the interaction between MdbZIP44/MdERF38 and MdMYB1. Taken together, our results reveal that MdPIF7 regulates anthocyanin biosynthesis in apple and hypocotyl growth in ectopically expressed Arabidopsis through MdPIF7–MdBBX23–MdHY5 and MdPIF7–MdbZIP44/MdERF38–MdMYB1 modules. Our findings enrich the functional studies of PIF proteins and provide insights into the molecular mechanism of PIF-mediated anthocyanin biosynthesis and hypocotyl growth.
Introduction
Light is one of the most important environmental factors, not only providing energy for plant photosynthesis, but also acting as an environmental signal involved in regulating many aspects of plant growth and development, such as leaf development, stem and root growth, flower bud differentiation, and fruit development (Kami et al., 2010; Jin and Zhu, 2019; Sanchez et al., 2020; Su et al., 2021). Plants can sense light signaling through photoreceptors. Several photoreceptors have been found in plants, including phytochrome, cryptochrome, phototropin, and UV-B photoreceptor UVR8 (Chaves et al., 2011; Heijde and Ulm, 2012; Pham et al., 2018). According to the presence or absence of light during seedling growth, plants exhibit two distinct growth states, namely, photomorphogenesis and skotomorphogenesis (Josse and Halliday, 2008; Wu, 2014). Photomorphogenesis in plants is characterized by shortened hypocotyls, expanded cotyledons, and pigment accumulation (Fankhauser and Casal 2004; Liu et al., 2020). In contrast, skotomorphogenesis in plants is displayed by elongated hypocotyls, closed cotyledons, and apical hooks (Josse and Halliday, 2008; Delker et al., 2014). Therefore, light has a substantial impact on plant growth and development. Apple (Malus × domestica) is one of the leading agricultural products with international competitiveness in China. The cultivated area and yield of apple ranks first in the world and occupies an extremely important position in the fruit industry of China. In apple production, shade in the orchard affects the growth and development of fruit trees, as well as the yield and quality of fruits. An investigation on the effect of light on plant growth and the response mechanism of plants to light signaling will provide a theoretical basis for the development of new cultivation techniques for fruit trees and the cultivation of new apple varieties adapted to different light conditions.
Phytochrome is an important class of photoreceptors which is capable of sensing and responding to red and far-red signals (Bae and Choi, 2008; Pham et al., 2018). Under red light conditions, inactive red light-absorbing chromophore in the cytosol is converted to active far-red light-absorbing chromophore and then transferred to the nucleus (Klose et al., 2015). In the nucleus, phytochrome regulates the light signaling response by interacting with multiple partners (Pham et al., 2018). As an interaction partner of phytochrome, phytochrome interacting factors (PIFs) play an important role in the transition from skotomorphogenesis to photomorphogenesis in plants (Castillon et al., 2007; Leivar et al., 2008b; Shin et al., 2009). PIF proteins belong to the basic helix–loop–helix (bHLH) transcription factor (TF) subfamily and function as negative regulators of photomorphogenesis (Leivar and Quail, 2011; Leivar and Monte, 2014). PIFs regulate the expression of target genes by forming homodimers or interacting with other partners (Toledo-Ortiz et al., 2003; Bu et al., 2011; Pham et al., 2018). Recent studies have focused on the degradation mechanisms of PIF proteins under both light and dark conditions. Four kinases (CASEIN KINASE 2, BRASSINOSTEROID-INSENSITIVE 2, photo-regulatory protein kinases, and phytochromes) and four ubiquitination ligases (SCFEBF1/2, CUL3LRB, CUL3BOP, and CUL4COP1-SPA) play important roles in the regulation of the abundance of PIFs (Park et al., 2004; Pham et al., 2018; Balcerowicz, 2020). In Arabidopsis (Arabidopsis thaliana), the PIF protein family consists of eight members (AtPIF1–AtPIF8; Pham et al., 2018). Among them, PIF1, PIF3, PIF4, PIF5, and PIF7 negatively regulate plant photomorphogenesis (Huq and Quail, 2002; Monte et al., 2004; Khanna et al., 2007; Leivar et al., 2008a; Shin et al., 2009; Paik et al., 2019; Heng et al., 2019a; Yang et al., 2021). In addition to modulating plant photomorphogenesis, PIFs also play important roles in the regulation of seed germination, thermomorphogenesis, shade avoidance, anthocyanin biosynthesis, chlorophyll biosynthesis and senescence, fruit development, hormone signaling, and biotic and abiotic stress responses (Huq et al., 2004; Shin et al., 2007; Franklin et al., 2011; Hornitschek et al., 2012; de Lucas and Prat, 2014; Liu et al., 2015, 2021; Zhang et al., 2015, 2017; Quint et al., 2016; Paik et al., 2017; Gao et al., 2018; Casal and Balasubramanian, 2019; Gramegna et al., 2019; Han et al., 2019; Rosado et al., 2019; Wang et al., 2020; Job and Datta, 2021; Lee et al., 2021, Pan et al., 2021). In apple, MdPIF1 is involved in regulating seed germination, bud dormancy, and photomorphogenesis (Zhou et al., 2017). Overexpression of MdPIF4 reduces the sensitivity of apple calli to abscisic acid (Zheng et al., 2020). MdPIF3 negatively regulates cold resistance but enhances drought tolerance (Zheng et al., 2021).
In addition to the PIF proteins, B-box (BBX) proteins also play a pivotal role in the regulation of light-mediated plant growth and development (Vaishak et al., 2019; Song et al., 2020). BBX proteins belong to the zinc finger protein family with one or two BBX domains, which play essential roles in the regulation of photomorphogenesis (Khanna et al., 2009; Crocco and Botto, 2013; Gangappa and Botto, 2014). There are 32 BBX proteins (AtBBX1–AtBBX32) in Arabidopsis (Khanna et al., 2009; Gangappa and Botto, 2014). Among them, BBX4, BBX20, BBX21, BBX22, and BBX23 positively regulate photomorphogenesis, while BBX18, BBX19, BBX24, BBX25, BBX28, BBX30, and BBX31 negatively regulate photomorphogenesis (Datta et al., 2006, 2007, 2008; Indorf et al., 2007; Chang et al., 2008, 2011; Wang et al., 2011; Fan et al., 2012; Gangappa et al., 2013; Wei et al., 2016; Xu et al., 2016; Zhang et al., 2017; Job et al., 2018; Lin et al., 2018; Xu et al., 2018; Yadav et al., 2019; Heng et al., 2019a, 2019b). The BBX proteins are also involved in the biosynthesis of secondary metabolites, seed germination, cotyledon opening, thermomorphogenesis, flowering, and leaf senescence (Li et al., 2014; Ding et al., 2018; Kim et al., 2018; Xiong et al., 2019; Bai et al., 2019a, 2019b, 2019c; Ravindran et al., 2021; An et al., 2021b). In apple, MdCOL11, MdBBX1, MdBBX15, MdBBX17, MdBBX20, MdBBX22, MdBBX35, MdBBX51, and MdBBX54 positively regulate anthocyanin biosynthesis, while MdBBX25 and MdBBX37 inhibit anthocyanin accumulation (Bai et al., 2014; Fang et al., 2019; Plunkett et al., 2019; An et al., 2020a, 2020b). Overexpression of MdBBX10 enhances tolerance to salt and drought stresses (Liu et al., 2019). MdBBX37 was identified as a positive regulator in jasmonic acid-mediated cold tolerance (An et al., 2021a).
The basic leucine zipper (bZIP) TF HY5 is identified as a positive regulator of photomorphogenesis and anthocyanin biosynthesis in Arabidopsis, apple, and tomato (Solanum lycopersicum; Stracke et al., 2010; Gangappa and Botto, 2016; An et al., 2017; Liu et al., 2018; Qiu et al., 2019). Extensive studies have found that BBXs work with HY5, and the BBXs-HY5 regulatory network plays an important role in the regulation of photomorphogenesis and anthocyanin biosynthesis (Gangappa et al., 2013; Xu et al., 2016; Lin et al., 2018; Bursch et al., 2020; Xu and Deng, 2020; Zhao et al., 2020). In Arabidopsis, BBX20, BBX21, BBX22, BBX23, BBX24, BBX25, and BBX28 are interaction partners of HY5 (Datta et al., 2007, 2008; Indorf et al., 2007; Gangappa et al., 2013; Wei et al., 2016; Zhang et al., 2017; Lin et al., 2018). BBX11, BBX21, BBX22, and BBX32 directly modulate the expression of HY5 (Holtan et al., 2011; Xu et al., 2016; Xu and Deng, 2020; Zhao et al., 2020). In apple, both MdBBX22 and MdBBX37 interact with MdHY5 and affect the transcriptional activity of MdHY5 (An et al., 2020a, 2020b).
Although PIF and BBX proteins have been extensively studied in Arabidopsis, they have been less studied in woody plants, such as apple (Zhang and Hao, 2020). In this study, we identified a light responsive PIF protein, MdPIF7, in apple, which inhibited anthocyanin biosynthesis in apple and promoted hypocotyl growth in ectopically expressed Arabidopsis. As the interaction partner of MdPIF7, MdBBX23 promoted anthocyanin biosynthesis in apple and inhibited hypocotyl growth in ectopically expressed Arabidopsis. Further investigations found that MdPIF7 negatively regulated MdBBX23-mediated anthocyanin accumulation and hypocotyl elongation inhibition by attenuating the transcriptional activation of MdBBX23 on MdHY5. In addition, MdPIF7 interacted with MdbZIP44 and MdERF38, which are two positive regulators of anthocyanin biosynthesis, and it negatively regulated MdbZIP44- and MdERF38-promoted anthocyanin accumulation by interfering with the interaction between MdbZIP44/MdERF38 and MdMYB1. These results reveal two potential molecular pathways in which MdPIF7 could regulate anthocyanin biosynthesis in apple and hypocotyl growth in ectopically expressed Arabidopsis through the MdPIF7–MdBBX23–MdHY5 and MdPIF7–MdbZIP44/MdERF38–MdMYB1 modules. Our study will provide insight for further research on the biological function and mechanism of action of PIF proteins.
Results
MdPIF7 is a light-responsive protein
The PIF proteins play key roles in the regulation of plant growth and development mediated by light signaling (Leivar and Quail, 2011; Leivar and Monte, 2014; Paik et al., 2017; Balcerowicz, 2020). Although the multiple functions of PIFs have been extensively studied in model plants, they have been poorly studied in apple. In this study, the functions of the apple MdPIF7 protein were investigated. Protein sequence alignment analysis revealed that MdPIF7 had a conserved bHLH DNA binding domain (Supplemental Figure S1). Moreover, MdPIF7 had high identity with the PIF7 proteins from sweet cherry (Prunus avium) and white pear (Pyrus x bretschneideri; Supplemental Figure S2). Compared with other PIFs in apple, including MdPIF1, MdPIF3, MdPIF4, MdPIF6, and MdPIF8, no self-activation activity of MdPIF7 was detected in yeast (Supplemental Figure S3).
PIFs are considered to be negative regulators of light signaling (Balcerowicz, 2020; Sanchez et al., 2020; Xu and Deng, 2020). To study the light signaling response of MdPIF7, we examined the change in MdPIF7 gene expression under different light intensity conditions. The results showed that expression of the MdPIF7 gene was inhibited by high light intensity (Figure 1A). To detect the change in PIF7 protein abundance, we obtained the MdPIF7-labeled glutathione protein (MdPIF7-GST). Apple seedlings were treated with low and high light, and proteasome inhibitor MG132, and the total proteins of apple seedlings were extracted after different treatments. The MdPIF7–GST fusion protein was mixed with apple seedling protein extract and incubated. Samples were collected at designated intervals to detect the change in MdPIF7 protein abundance. The results showed that degradation of the MdPIF7 protein was inhibited by MG132 (Figure 1B), indicating that MdPIF7 could be degraded through the proteasome pathway. Compared with the low light treatment, stability of the MdPIF7 protein was poor after high light treatment (Figure 1B), indicating that high light treatment decreased, while low light treatment improved stability of the MdPIF7 protein. These results suggest that MdPIF7 is sensitive to light.
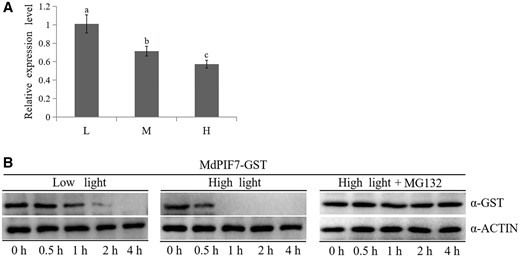
Light signaling response of MdPIF7. A, Gene expression analysis of MdPIF7 under different light intensity treatments. L, low light, photon flux density ∼80 μmol·m−2·s−1. M, moderate light, photon flux density ∼150 μmol·m−2·s−1. H, high light, photon flux density ∼300 μmol·m−2·s−1. Thirty-day-old apple tissue culture seedlings were treated with constant darkness for 24 h, followed by different light intensity conditions for 48 h and were collected to detect the expression of MdPIF7 using RT-qPCR. The expression level of low light treatment was used as the reference and set to 1. Three biological replicates were carried out with three technical repeats. Error bars denote the standard deviations. Different letters above the bars indicate significant differences (P < 0.05) obtained by one-way ANOVA with Duncan’s test. B, Stability of the MdPIF7–GST fusion protein under low and high light treatments was examined. Thirty-day-old apple tissue culture seedlings were treated with constant darkness for 24 h, followed by low and high light and proteasome inhibitor MG132 for 48 h, and the total proteins of apple seedlings after different light intensity treatments were extracted. The protein extraction solution was incubated with the MdPIF7–GST fusion protein. The mixture was collected at designated periods (0, 0.5, 1, 2, and 4 h). Western blot analysis was performed with GST-tag(12G8) mouse (code: M20007L) and ACTIN(26F7) mAb antibodies. The experiments were performed 3 times with similar results, and a representative picture is shown.
Overexpression of MdPIF7 represses anthocyanin biosynthesis in transgenic apple materials and promotes hypocotyl elongation in ectopically expressed Arabidopsis
Anthocyanin accumulation is a typical feature of most plants after light treatment (Thoma et al., 2020; An et al., 2020b). Considering the light response of MdPIF7, we investigated the effect of MdPIF7 on anthocyanin biosynthesis. MdPIF7 overexpression (MdPIF7-OX) and antisense suppression plasmids (MdPIF7-Anti) were generated and transformed into apple calli (Supplemental Figure S4A). Apple calli with different genotypes were placed on culture media and treated with light. Compared with the wild-type (WT) control, the accumulation of anthocyanin in MdPIF7-OX was reduced, while the accumulation of anthocyanin in MdPIF7-Anti was increased (Figure 2, A and B). With the increase of light intensity, the inhibitory effect of MdPIF7 weakened gradually (Supplemental Figure S5, A and B), indicating that MdPIF7 mainly acted under low light, and its inhibitory effect on anthocyanin accumulation gradually weakened with the increase of light intensity. Gene expression analysis showed that overexpression of MdPIF7 decreased the expression of anthocyanin biosynthesis-related genes (MdHY5, MdDFR, MdUF3GT, MdCHI, MdCHS, and MdANS), while suppression of MdPIF7 increased the expression of those genes (Figure 2C). In addition, MdPIF7-OX and suppression plasmids were transiently transformed into apple leaves (Supplemental Figure S4B). Apple leaves with different genotypes were placed on the culture medium and treated with light. Consistent with the results of apple calli, overexpression of MdPIF7 in apple leaves inhibited the accumulation of anthocyanin, while suppression of MdPIF7 contributed to the accumulation of anthocyanin (Figure 2, D and E). Moreover, the MdPIF7-OX viral vector (MdPIF7-pIR) was transiently injected into apple skin (Supplemental Figure S4C). Consistent with the results of apple calli and apple leaves, overexpression of MdPIF7 in apple skin negatively regulated anthocyanin biosynthesis in the injection area (Figure 2, F–G).
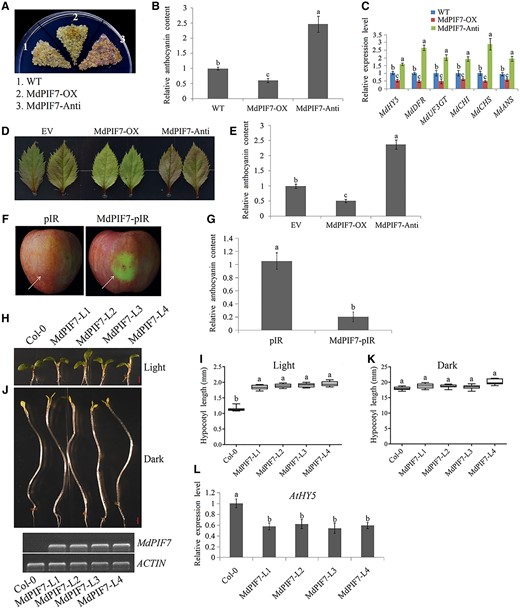
Regulation of anthocyanin biosynthesis and hypocotyl growth by MdPIF7. A, Anthocyanin accumulation phenotypes and (B) relative anthocyanin contents of WT and transgenic apple calli (MdPIF7-OX, MdPIF7-overexpressing apple calli; MdPIF7-Anti, MdPIF7 antisense suppressing apple calli). Fifteen-day-old apple calli grown on media were stored in a light incubator at 24°C with a constant light (photon flux density ∼300 μmol·m−2·s−1) for 5 d. The experiments were performed 3 times with similar results, and a representative picture is shown. The anthocyanin content of the WT was used as the reference and set to 1. Error bars denote the standard deviations (n = 3). Different letters above the bars indicate significant differences (P < 0.05) obtained by one-way ANOVA with Duncan’s test. C, Expression analysis of anthocyanin biosynthesis-related genes (MdHY5, MdDFR, MdUF3GT, MdCHI, MdCHS, and MdANS) in apple calli. The expression level of the WT was used as the reference and set to 1. Three biological replicates were carried out with three technical repeats. Error bars denote the standard deviations. Different letters above the bars indicate significant differences (P < 0.05) obtained by one-way ANOVA with Duncan’s test. D, Anthocyanin accumulation phenotypes and (E) relative anthocyanin contents of transient transformed apple leaves (EV: empty vector controls; MdPIF7-OX: MdPIF7-overexpressing apple leaves; MdPIF7-Anti, MdPIF7 antisense suppressing apple leaves). Apple leaves were stored in a light incubator at 24°C with a constant light (photon flux density ∼300 μmol·m−2·s−1) for 5 d. Each experiment contained 8–10 leaves per genotype. The experiments were performed 3 times with similar results, and a representative picture is shown. Anthocyanin content of EV was used as the reference and set to 1. Error bars denote the standard deviations (n = 28). Different letters above the bars indicate significant differences (P < 0.05) obtained by one-way ANOVA with Duncan’s test. F, Anthocyanin accumulation phenotypes and (G) relative anthocyanin contents of transient transformed apple fruits (pIR: empty vector controls; MdPIF7-pIR: MdPIF7-overexpressing apple fruits). Apple fruits were stored in a light incubator at 24°C with a constant light (photon flux density ∼300 μmol·m−2·s−1) for 5 d. Each experiment contained five to eight fruits per genotype. The experiments were performed 3 times with similar results, and a representative picture is shown. The arrow points to the injection area. Anthocyanin content of pIR was used as the reference and set to 1. Error bars denote the standard deviations (n = 21). Different letters above the bars indicate significant differences (P < 0.05) obtained by one-way ANOVA with Duncan’s test. H, Hypocotyl phenotypes and (I) lengths in 10-d-old WT (Col-0) and four independent MdPIF7-overexpressing Arabidopsis seedlings (MdPIF7-L1, L2, L3, and L4) grown in normal light conditions (80 μmol·m−2·s−1). Bar = 1 mm. Each experiment contained 10–15 seedlings per genotype. The experiments were performed 3 times with similar results, and a representative picture shown. Error bars denote the standard deviations. Different letters above the bars indicate significant differences (P < 0.05) obtained by one-way ANOVA with Duncan’s test. Center line, median; box limits, upper and lower quartiles. J, Hypocotyl phenotypes and (K) lengths in 6-d-old Col-0 and four independent MdPIF7 transgenic Arabidopsis seedlings (MdPIF7-L1, L2, L3, and L4) grown in the dark. Bar = 1 mm. Each experiment contained 10–15 seedlings per genotype. The experiments were performed 3 times with similar results, and a representative picture is shown. Error bars denote the standard deviations. Different letters above the bars indicate significant differences (P < 0.05) obtained by one-way ANOVA with Duncan’s test. Center line, median; box limits, upper and lower quartiles. L, RT-qPCR analysis showing the expression level of AtHY5 in Col-0 and transgenic Arabidopsis (grown under light). The expression level of Col-0 was used as the reference and set to 1. Three biological replicates were carried out with three technical repeats. Error bars denote the standard deviations. Different letters above the bars indicate significant differences (P < 0.05) obtained by one-way ANOVA with Duncan’s test.
The variation in hypocotyl length is also an important feature of the plant light signaling response (Botterweg-Paredes et al., 2020; Teixeira, 2020). Next, we investigated the effect of MdPIF7 on hypocotyl growth. Interestingly, compared with the WT control (Col-0), hypocotyl elongation of Arabidopsis seedlings ectopically expressing MdPIF7 was observed under light conditions (Figure 2, H and I), while hypocotyl length was not significantly different under dark conditions (Figure 2, J and K), suggesting that MdPIF7 promoted hypocotyl elongation. HY5 is a key regulator of light-mediated hypocotyl elongation inhibition (Osterlund et al., 2000; Gangappa and Botto, 2016; An et al., 2017; Xu and Deng, 2020). Reverse transcription-quantitative PCR (RT-qPCR) analysis showed that overexpression of MdPIF7 in Arabidopsis seedlings inhibited the expression of the AtHY5 gene (Figure 2L). These results suggest that MdPIF7 is a negative regulator of light signaling, including inhibition of anthocyanin accumulation in apple and promotion of hypocotyl elongation in ectopically expressed Arabidopsis.
MdPIF7 interacts with MdBBX23
BBX proteins play pivotal roles in the regulation of plant growth and development mediated by light signaling (Gangappa and Botto, 2014; Song et al., 2020). The PIF proteins act synergistically with the BBX proteins (Zhang et al., 2017; Job and Datta, 2021; Sun et al., 2021). We sought to identify the MdBBX proteins that interact with MdPIF7 and regulate anthocyanin accumulation and hypocotyl growth in apple. Previously, we cloned several MdBBX proteins in apple, including MdBBX10, MdBBX20, MdBBX22, MdBBX23, MdBBX24, MdBBX25, MdBBX33, and MdBBX43 (An et al., 2020a). The interaction between MdPIF7 and these proteins was detected by yeast two-hybrid (Y2H) assays. The result showed that MdPIF7 specifically interacted with MdBBX23 (Figure 3A). In addition, the interaction between MdPIF7 and MdBBX23 proteins was investigated by pull-down assays. The results indicated that MdPIF7 interacted with MdBBX23 in vitro (Figure 3B). Moreover, we performed bimolecular fluorescence complementation (BiFC) assays to verify the interaction between MdPIF7 and MdBBX23. Yellow fluorescent protein (YFP) fluorescence signals could only be observed when MdPIF7 and MdBBX23 were co-expressed in the nucleus of onion (Allium cepa) epidermal cells (Figure 3C), demonstrating that MdPIF7 interacted with MdBBX23 in the nucleus.
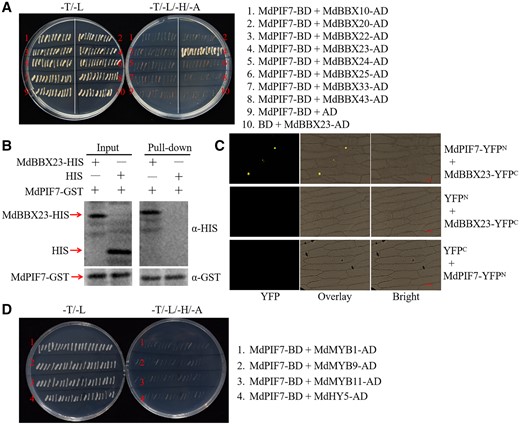
Interaction between MdPIF7 and MdBBX23. A, Y2H assays showing the interaction between MdPIF7 and the MdBBX proteins. Full-length MdPIF7 was cloned into the pGBT9 vector (MdPIF7-BD). Full-length MdBBX10, MdBBX20, MdBBX22, MdBBX23, MdBBX24, MdBBX25, MdBBX33, and MdBBX43 were cloned into the pGAD424 vector (MdBBX10-AD, MdBBX20-AD, MdBBX22-AD, MdBBX23-AD, MdBBX24-AD, MdBBX25-AD, MdBBX33-AD, and MdBBX43-AD). B, Pull-down assays showing the interaction between MdPIF7 and MdBBX23. Escherichia coli-expressed HIS or MdBBX23-HIS protein was incubated with a cobalt chelate affinity resin containing the immobilized GST-tagged MdPIF7 protein. Western blot analysis was performed with HIS-tag(10E2) mouse (code: M30111L) and GST-tag(12G8) mouse (code: M20007L) antibodies. C, BiFC assays showing the interaction between MdPIF7 and MdBBX23 in the nuclei of epidermal onion cells. Full-length MdPIF7 was cloned into the pSPYNE-35S/pUC-SPYNE vector (MdPIF7-YFPN). Full-length MdBBX23 was cloned into the pSPYCE-35S/pUC-SPYCE vector (MdBBX23-YFPC). YFP fluorescence was observed using a fluorescence microscope at the wavelength of 490–540 nm. Bars = 20 μm. D, Y2H assays showing the interaction between MdPIF7 and anthocyanin regulatory proteins including MdMYB1, MdMYB9, MdMYB11, and MdHY5. Full-length MdMYB1, MdMYB9, MdMYB11, and MdHY5 were cloned into the pGAD424 vector (MdMYB1-AD, MdMYB9-AD, MdMYB11-AD, and MdHY5-AD).
In addition to the MdBBX proteins, we also examined the interaction between MdPIF7 and several anthocyanin regulatory proteins, namely MdMYB1, MdMYB9, MdMYB11, and MdHY5, using Y2H assays (An et al., 2020a). We were unable to detect an interaction between MdPIF7 and these anthocyanin regulatory proteins (Figure 3D).
Overexpression of MdBBX23 promotes anthocyanin biosynthesis in transgenic apple materials and inhibits hypocotyl elongation in ectopically expressed Arabidopsis
The specific interaction between MdPIF7 and MdBBX23 led us to investigate the role of MdBBX23 in light signaling responses. MdBBX23 overexpression (MdBBX23-OX) and antisense suppression plasmids (MdBBX23-Anti) were constructed and transformed into apple calli (Supplemental Figure S4D). Anthocyanin coloring assays in apple calli showed that compared with the WT, overexpression of MdBBX23 increased, while suppression of MdBBX23 reduced the biosynthesis of anthocyanin (Figure 4, A and B). RT-qPCR results showed that several anthocyanin biosynthesis-related genes (MdHY5, MdDFR, MdUF3GT, MdCHI, MdCHS, and MdANS) were induced in MdBBX23-overexpressing calli, but expression of these genes (MdHY5, MdDFR, MdCHS, and MdANS) was inhibited in MdBBX23 suppression expression calli (Figure 4C). In parallel, MdBBX23-OX and suppression plasmids were transiently transformed into apple leaves (Supplemental Figure S4E). Apple leaf coloring assays showed that overexpression of MdBBX23 contributed to anthocyanin biosynthesis, while suppression of MdBBX23 inhibited anthocyanin biosynthesis in apple leaves (Figure 4, D and E). And apple skin injection assays suggested that overexpression of MdBBX23 promoted anthocyanin biosynthesis in the injection area (Figure 4, F and G; Supplemental Figure S4F), which was consistent with the results of transgenic apple calli and leaves.
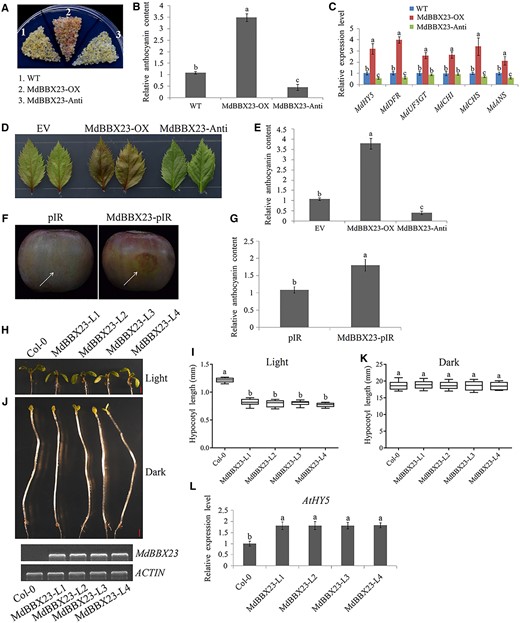
Regulation of anthocyanin biosynthesis and hypocotyl growth by MdBBX23. A, Anthocyanin accumulation phenotypes and (B) relative anthocyanin content of the WT and transgenic apple calli (MdBBX23-OX, MdBBX23-overexpressing apple calli; MdBBX23-Anti, MdBBX23 antisense suppressing apple calli). Fifteen-day-old apple calli grown on medium were stored at a light incubator at 24°C with a constant light (photon flux density ∼300 μmol·m−2·s−1) for 5 d. The experiments were performed 3 times with similar results, and a representative picture is shown. Anthocyanin content of the WT was used as the reference and set to 1. Error bars denote the standard deviations (n = 3). Different letters above the bars indicate significant differences (P < 0.05) obtained by one-way ANOVA with Duncan’s test. C, Expression analysis of anthocyanin biosynthesis-related genes (MdHY5, MdDFR, MdUF3GT, MdCHI, MdCHS, and MdANS) in apple calli. The expression level of the WT was used as the reference and set to 1. Three biological replicates were carried out with three technical repeats. Error bars denote the standard deviations. Different letters above the bars indicate significant differences (P < 0.05) obtained by one-way ANOVA with Duncan’s test. D, Anthocyanin accumulation phenotypes and E, relative anthocyanin contents of transient transformed apple leaves. Apple leaves were stored in a light incubator at 24°C with a constant light (photon flux density ∼300 μmol·m−2·s−1) for 5 d. Each experiment contained 8–10 leaves per genotype. The experiments were performed 3 times with similar results, and a representative picture is shown. Anthocyanin content of EV was used as the reference and set to 1. Error bars denote the standard deviations (n = 28). Different letters above the bars indicate significant differences (P < 0.05) obtained by one-way ANOVA with Duncan’s test. F, Anthocyanin accumulation phenotypes and (G) relative anthocyanin contents of transient transformed apple fruits (pIR: empty vector controls; MdBBX23-pIR: MdBBX23-overexpressing apple fruits). Apple fruits were stored in a light incubator at 24°C with a constant light (photon flux density ∼300 μmol·m−2·s−1) for 3 d. Each experiment contained five to eight fruits per genotype. The experiments were performed 3 times with similar results, and a representative picture is shown. The arrow points to the injection area. Anthocyanin content of pIR was used as the reference and set to 1. Error bars denote the standard deviations (n = 21). Different letters above the bars indicate significant differences (P < 0.05) obtained by one-way ANOVA with Duncan’s test. H, Hypocotyl phenotypes and (I) lengths in 10-d-old Col-0 and four independent MdBBX23-overexpressing transgenic Arabidopsis seedlings (MdBBX23-L1, L2, L3, and L4) grown in normal light conditions (80 μmol·m−2·s−1). Bar = 1 mm. Each experiment contained 10–15 seedlings per genotype. The experiments were performed 3 times with similar results, and a representative picture shown. Error bars denote the standard deviations. Different letters above the bars indicate significant differences (P < 0.05) obtained by one-way ANOVA with Duncan’s test. Center line, median; box limits, upper and lower quartiles. J, Hypocotyl phenotypes and (K) lengths in 6-d-old Col-0 and four independent MdBBX23 transgenic Arabidopsis seedlings (MdBBX23-L1, L2, L3, and L4) grown in the dark. Bar = 1 mm. Each experiment contained 10–15 seedlings per genotype. The experiments were performed 3 times with similar results, and a representative picture is shown. Error bars denote the standard deviations. Different letters above the bars indicate significant differences (P < 0.05) obtained by one-way ANOVA with Duncan’s test. Center line, median; box limits, upper and lower quartiles. L, RT-qPCR analysis of the expression level of AtHY5 in Col-0 and transgenic Arabidopsis (grown under light). The expression level of Col-0 was used as the reference and set to 1. Three biological replicates were carried out with three technical repeats. Error bars denote the standard deviations. Different letters above the bars indicate significant differences (P < 0.05) obtained by one-way ANOVA with Duncan’s test.
To investigate the effect of MdBBX23 on hypocotyl growth, we compared hypocotyl length between Col-0 and MdBBX23-overexpressing Arabidopsis. The results showed that compared with Col-0, MdBBX23-overexpressing Arabidopsis exhibited the phenotype of hypocotyl elongation inhibition under light conditions (Figure 4, H and I), while there was no significant difference in hypocotyl length between Col-0 and MdBBX23-overexpressing Arabidopsis under dark conditions (Figure 4, J and K), suggesting that MdBBX23 might be a negative regulator of hypocotyl elongation. RT-qPCR analysis showed that overexpression of MdBBX23 promoted AtHY5 expression in Arabidopsis seedlings (Figure 4L). These data suggest that MdBBX23 is a positive regulator of light signaling, including the promotion of anthocyanin biosynthesis in apple and inhibition of hypocotyl elongation in ectopically expressed Arabidopsis.
MdBBX23 directly binds to the MdHY5 promoter and activates its transcription
HY5 is a key regulator of light-mediated anthocyanin biosynthesis and hypocotyl elongation inhibition (Osterlund et al., 2000; Gangappa and Botto, 2016; An et al., 2017; Xu and Deng, 2020). The compelling inducible expression of MdHY5 by MdBBX23 made us consider whether MdBBX23 directly binds to the promoter of MdHY5 and regulates its expression (Figure 4C). BBX proteins could bind to the G-box elements in the promoters of their target genes (Vaishak et al., 2019; Song et al., 2020). To test this hypothesis, we analyzed the promoter sequence of MdHY5, and two potential MdBBX23 binding sites (G-box 1 and G-box 2) were found (Figure 5A; Supplemental Table S1). Electromobility shift assays (EMSAs) were performed to detect the binding of MdBBX23 to the MdHY5 promoter. The results showed that MdBBX23 was specifically bound to the G-box 1 site of the MdHY5 promoter, while MdPIF7 binding to the G-box sites was not detected (Figure 5B). To further confirm the binding of MdBBX23 to the promoter of MdHY5, we performed a complete EMSA. We found that binding bands existed when the MdBBX23 protein and MdHY5 promoter probe were added simultaneously (Figure 5, C and D). When competitive probes were added in proportion, the strength of binding bands decreased gradually (Figure 5D). When the MdHY5 promoter probe was mutated, the binding bands disappeared (Figure 5D). These data suggest that MdBBX23 directly binds to the MdHY5 promoter.
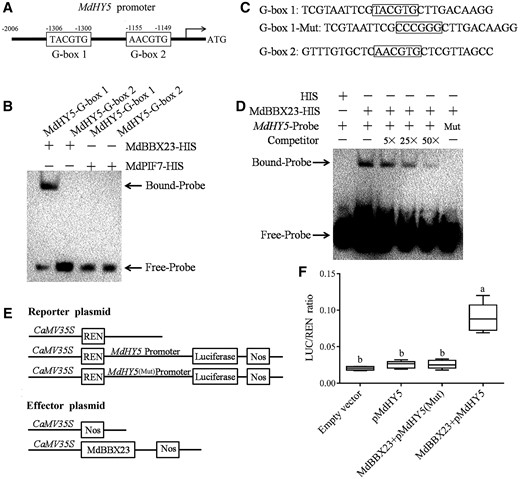
MdBBX23 mediates the expression of MdHY5. A, Diagram of the MdHY5 gene promoter region containing MdBBX23 potential binding sites (G-box 1 and G-box 2). B, EMSAs showing the MdBBX23–HIS fusion protein bound to the G-box 1 site of the MdHY5 promoter. “−” indicates the absence of corresponding proteins or probes. “+” indicates the presence of corresponding proteins or probes. C, Diagram of the MdHY5 gene promoter region containing MdBBX23 potential binding sites (G-box 1 and G-box 2). In MdHY5-Mut, the 5′-TACGTG-3′ motif was replaced with 5′-CCCGGG-3′. D, EMSAs showing the MdBBX23–HIS fusion protein bound to the MdHY5 promoter. “−” indicates the absence of corresponding proteins or probes. “+” indicates the presence of corresponding proteins or probes. “Mut” indicates the presence of mutant probes. The unlabeled probes were used as competitors. “5×”, “25×”, and “50×” represent the rates of competitor. E, Schematic representation of the LUC reporter vector containing the MdHY5 promoter and the effector vectors expressing MdBBX23 under the control of the 35S promoters. Full-length MdBBX23 was fused to pGreenII 62-SK vector. The promoter sequence of MdHY5 was cloned into the pGreenII 0800-LUC vector. pMdHY5(Mut) in which the 5′-TACGTG-3′ motif was replaced with 5′-CCCGGG-3′. F, The dual LUC assays in N. benthamiana leaves showing that MdBBX23 activates the transcription of MdHY5 and LUC/REN ratio detection to verify MdBBX23 activates the transcription of MdHY5. Empty vector, pGreenII 62-SK + pGreenII 0800-LUC; pMdHY5, pGreenII 62-SK + pMdHY5-pGreenII 0800-LUC; MdBBX23 + pMdHY5, MdBBX23-pGreenII 62-SK + pMdHY5-pGreenII 0800-LUC; MdBBX23 + pMdHY5(Mut), MdBBX23-pGreenII 62-SK + pMdHY5(Mut)-pGreenII 0800-LUC. An empty vector was used as the reference. Error bars denote the standard deviations (n = 27). Different letters above the bars indicate significant differences (P < 0.05) obtained by one-way ANOVA with Duncan’s test. Center line, median; box limits, upper and lower quartiles. The experiments were performed 3 times with similar results, and 8–10 leaves were injected in each experiment.
Dual luciferase (LUC) assays were performed to test the transcriptional regulation of MdBBX23 on MdHY5. The effector and reporter plasmids were constructed (Figure 5E), and recombinant plasmids were introduced into Agrobacterium (Agrobacterium tumefaciens) and expressed in tobacco leaves. The results of fluorescence imaging and LUC activity assays showed that MdBBX23 activated the expression of MdHY5 (Figure 5F). When the binding site was mutated, activation was abolished (Figure 5F). These data indicate that MdBBX23 activates the expression of MdHY5. Combined with the above results, we found that MdBBX23 positively regulates the expression of MdHY5 by directly binding to its promoter.
MdPIF7 negatively regulates MdBBX23-mediated anthocyanin accumulation and hypocotyl elongation inhibition
The opposite biological functions of MdPIF7 and MdBBX23 and the interaction between these two proteins prompted us to investigate their genetic relationship. Overexpression of MdPIF7 in the background of MdBBX23-overexpressing apple calli significantly decreased MdBBX23-promoted anthocyanin accumulation (Figure 6, A and B; Supplemental Figure S4, A and D). In addition, we generated MdPIF7 and MdBBX23 co-expressed apple leaves (Supplemental Figure S4, B and E). As expected, apple leaf coloring assays showed that overexpression of MdPIF7 partially reduced MdBBX23-promoted anthocyanin accumulation in apple leaves (Figure 6, C and D). These results demonstrate that MdPIF7 negatively regulates MdBBX23-promoted anthocyanin accumulation in transgenic apple calli and leaves.
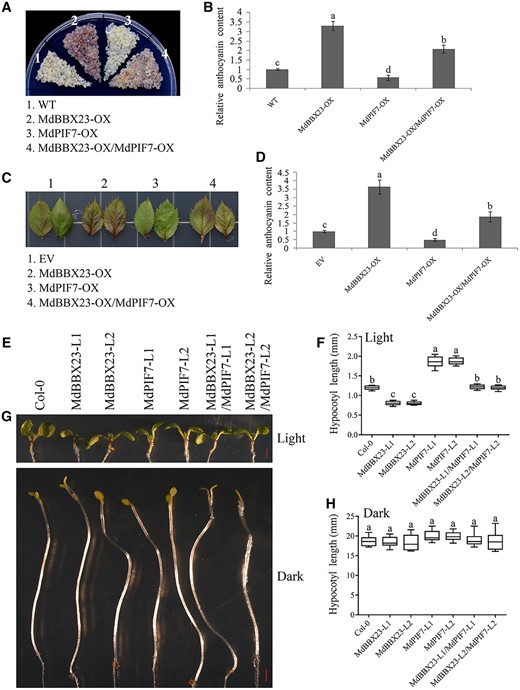
The relationship between MdBBX23 and MdPIF7 in the regulation of anthocyanin biosynthesis and hypocotyl growth. A, Anthocyanin accumulation phenotypes and (B) relative anthocyanin contents of WT and transgenic apple calli (MdBBX23-OX, MdBBX23-overexpressing apple calli; MdPIF7-OX, MdPIF7-overexpressing apple calli; MdBBX23-OX/MdPIF7-OX: overexpression of MdPIF7 in the background of MdBBX23-OX). Fifteen-day-old apple calli grown on media were stored in a light incubator at 24°C with constant light (photon flux density ∼300 μmol·m−2·s−1) for 5 d. The experiments were performed 3 times with similar results, and a representative picture is shown. Anthocyanin content of the WT was used as the reference and set to 1. Error bars denote the standard deviations (n = 3). Different letters above the bars indicate significant differences (P < 0.05) obtained by one-way ANOVA with Duncan’s test. C, Anthocyanin accumulation phenotype and (D) relative anthocyanin content of transient transformed apple leaves (EV: empty vector controls; MdBBX23-OX: MdBBX23-overexpressing apple leaves; MdPIF7-OX: MdPIF7-overexpressing apple leaves; MdBBX23-OX/MdPIF7-OX: MdBBX23 and MdPIF7 co-expression apple leaves). Apple leaves were stored in a light incubator (photon flux density ∼300 μmol·m−2·s−1) at 24°C with constant light for 5 d. Each experiment contained 8–10 leaves per genotype. The experiments were performed 3 times with similar results, and a representative picture is shown. Anthocyanin content of EV was used as the reference and set to 1. Error bars denote the standard deviations. Different letters above the bars indicate significant differences (P < 0.05) obtained by one-way ANOVA with Duncan’s test. E, Hypocotyl phenotypes and (F) lengths in 10-d-old Col-0 and transgenic Arabidopsis seedlings grown in normal light conditions (80 μmol·m−2·s−1). MdBBX23-L1 and -L2, MdBBX23-overexpressing Arabidopsis seedlings; MdPIF7-L1 and -L2, MdPIF7-overexpressing Arabidopsis seedlings; MdBBX23-L1/MdPIF7-L1 and MdBBX23-L2/MdPIF7-L2, MdBBX23 and MdPIF7 co-expression Arabidopsis seedlings. Bar = 1 mm. Each experiment contained 10–15 seedlings per genotype. The experiments were performed 3 times with similar results, and a representative picture is shown. Error bars denote the standard deviations. Different letters above the bars indicate significant differences (P < 0.05) obtained by one-way ANOVA with Duncan’s test. Center line, median; box limits, upper and lower quartiles. G, Hypocotyl phenotypes and (H) lengths in 6-d-old Col-0 and transgenic Arabidopsis seedlings grown in the dark. Bar = 1 mm. Each experiment contained 10–15 seedlings per genotype. The experiments were performed 3 times with similar results, and a representative picture is shown. Error bars denote the standard deviations. Different letters above the bars indicate significant differences (P < 0.05) obtained by one-way ANOVA with Duncan’s test. Center line, median; box limits, upper and lower quartiles.
To determine the effect of MdPIF7 on MdBBX23-regulated hypocotyl growth, we obtained the co-expression Arabidopsis lines of MdPIF7 and MdBBX23 (MdBBX23-L1/MdPIF7-L1 and MdBBX23-L2/MdPIF7-L2) by hybridization. The hypocotyl lengths of hybrid Arabidopsis were intermediate between MdPIF7 and MdBBX23 single transgenic lines under light conditions (Figure 6, E and F); however, there was no significant difference in hypocotyl length between hybrid Arabidopsis and MdPIF7 and MdBBX23 single transgenic lines under dark conditions (Figure 6, G and H). These results demonstrate that MdPIF7 negatively regulates MdBBX23-mediated hypocotyl elongation inhibition in ectopically expressed Arabidopsis.
MdPIF7 attenuates the transcriptional activity of MdBBX23 on MdHY5
To elucidate the molecular mechanism by which MdPIF7 negatively regulates the biological functions of MdBBX23, we investigated the effect of MdPIF7 on the binding strength of MdBBX23 on the MdHY5 promoter. We found that MdBBX23 directly bound the promoter of MdHY5, but the binding of MdPIF7 to the G-box sites of MdHY5 promoter was not detected (Figure 7, A and B). With the addition of MdPIF7 in proportion, the binding strength of MdBBX23 to the MdHY5 promoter gradually weakened (Figure 7, A and B).
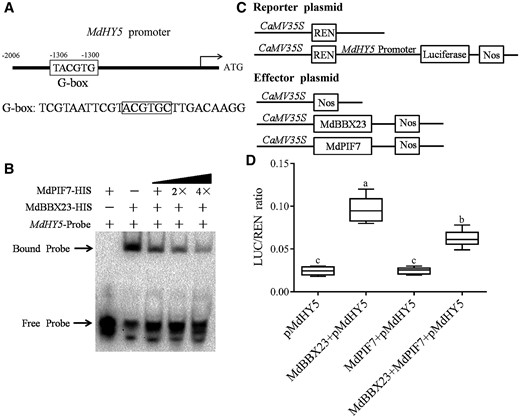
MdPIF7 affects the transcriptional activity of MdBBX23 on MdHY5. A, Diagram of the MdHY5 gene promoter region containing MdBBX23 binding sites (G-box). B, EMSAs show that MdPIF7 affects the binding of MdBBX23 to the MdHY5 promoter. “−” indicates the absence of corresponding proteins. “+” indicates the presence of corresponding proteins or probes. “2×” and “4×” indicated increased protein content. C, Schematic representation of the LUC reporter vector containing the MdHY5 promoter and the effector vectors expressing MdBBX23 or MdPIF7 under the control of 35S promoters. D, LUC/REN ratio detection to verify that MdPIF7 affects the transcriptional activity of MdBBX23 on MdHY5. pMdHY5, pGreenII 62-SK + pMdHY5-pGreenII 0800-LUC; MdBBX23 + pMdHY5, MdBBX23-pGreenII 62-SK + pMdHY5-pGreenII 0800-LUC; MdPIF7 + pMdHY5, MdPIF7-pGreenII 62-SK + pMdHY5-pGreenII 0800-LUC; MdPIF7 + MdBBX23 + pMdHY5, MdPIF7-pGreenII 62-SK + MdBBX23-pGreenII 62-SK + pMdHY5-pGreenII 0800-LUC. pMdHY5 was used as the reference. The experiments were performed 3 times with similar results, and 8–10 N. benthamiana leaves were injected in each experiment. Error bars denote the standard deviations. Different letters above the bars indicate significant differences (P < 0.05) obtained by one-way ANOVA with Duncan’s test. Center line, median; box limits, upper and lower quartiles.
To further investigate the effect of MdPIF7 on the transcriptional activity of MdBBX23 on MdHY5, we performed dual LUC assays, and effector and reporter plasmids were constructed (Figure 7C). The results showed that MdBBX23 could activate the expression of MdHY5, while MdPIF7 could not (Figure 7D). The simultaneous expression of MdBBX23 and MdPIF7 attenuated the activation of MdBBX23 on MdHY5 (Figure 7D). These results suggest that MdPIF7 negatively regulates MdBBX23-mediated anthocyanin accumulation and hypocotyl elongation inhibition by attenuating the transcriptional activation of MdBBX23 on MdHY5.
MdPIF7 interacts with MdbZIP44 and MdERF38
In addition to the MdBBX proteins, we previously identified several anthocyanin regulatory proteins in apple, including MdbZIP44, MdWRKY40, MdERF38, and MdTCP46 (An et al., 2020b). In this study, we examined the interaction between MdPIF7 and these anthocyanin regulatory proteins using Y2H assays. The results showed that MdPIF7 interacted with MdbZIP44 and MdERF38 in yeast cells (Figure 8A). As a parallel experiment, BiFC assays were performed to further verify the interaction between MdPIF7 and MdbZIP44/MdERF38. The results showed that MdPIF7 interacted with MdbZIP44 and MdERF38 in the nucleus of onion epidermal cells (Figure 8B). These results indicate that MdPIF7 interacts with MdbZIP44 and MdERF38.
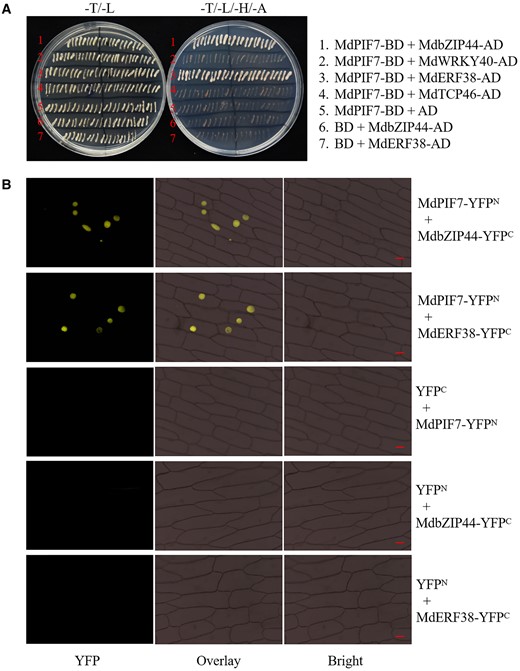
Interaction of MdPIF7 with MdbZIP44/MdERF38. A, Y2H assays showing the interaction of MdPIF7 with MdbZIP44/MdERF38. Full-length MdbZIP44, MdWRKY40, MdERF38, and MdTCP46 were cloned into the pGAD424 vector (MdbZIP44-AD, MdWRKY40-AD, MdERF38-AD, and MdTCP46-AD). B, BiFC assays showing the interaction of MdPIF7 with MdbZIP44/MdERF38 in the nuclei of onion epidermal cells. Full-length MdbZIP44 and MdERF38 were cloned into the pSPYCE-35S/pUC-SPYCE vector (MdbZIP44-YFPC and MdERF38-YFPC). YFP fluorescence was observed using a fluorescence microscope at the wavelength of 490–540 nm. Bars = 20 μm.
MdPIF7 negatively regulates MdbZIP44- and MdERF38-promoted anthocyanin accumulation in transgenic apple calli
MdbZIP44 and MdERF38 promoted anthocyanin biosynthesis by interacting with MdMYB1 and enhancing the transcriptional activity of MdMYB1 to its target genes (An et al., 2020b). To determine the effect of MdPIF7 on MdbZIP44- and MdERF38-promoted anthocyanin accumulation, we obtained transgenic apple calli with co-expression of MdbZIP44 and MdPIF7 (MdbZIP44-OX/MdPIF7-OX) and co-expression of MdERF38 and MdPIF7 (MdERF38-OX/MdPIF7-OX) (Supplemental Figure S4, G and H). Apple calli coloring assays showed that overexpression of MdPIF7 in the background of MdbZIP44- and MdERF38-overexpressing apple calli significantly reduced MdbZIP44- and MdERF38-promoted anthocyanin accumulation (Figure 9, A–D).
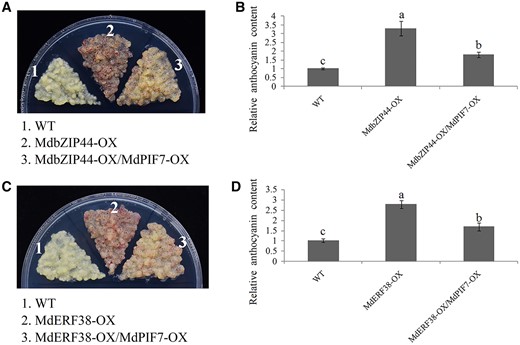
Relationship between MdPIF7, MdbZIP44, and MdERF38 in the regulation of anthocyanin biosynthesis. A, Anthocyanin accumulation phenotype and (B) relative anthocyanin content of the WT and transgenic apple calli (MdbZIP44-OX, MdbZIP44-overexpressing apple calli; MdbZIP44-OX/MdPIF7-OX: overexpression of MdPIF7 in the background of MdbZIP44-OX). Fifteen-day-old apple calli grown on media were stored in a light incubator at 24°C with constant light (photon flux density ∼300 μmol·m−2·s−1) for 5 d. The experiments were performed 3 times with similar results, and a representative picture is shown. Anthocyanin content of the WT was used as the reference and set to 1. Error bars denote the standard deviations (n = 3). Different letters above the bars indicate significant differences (P < 0.05) obtained by one-way ANOVA with Duncan’s test. C, Anthocyanin accumulation phenotype and (D) relative anthocyanin content of the WT and transgenic apple calli (MdERF38-OX, MdERF38-overexpressing apple calli; MdERF38-OX/MdPIF7-OX: overexpression of MdPIF7 in the background of MdERF38-OX). Fifteen-day-old apple calli grown on media were stored in a light incubator at 24°C with constant light (photon flux density ∼300 μmol·m−2·s−1) for 5 d. The experiments were performed 3 times with similar results, and a representative picture is shown. Anthocyanin content of WT was used as the reference and set to 1. Error bars denote the standard deviations (n = 3). Different letters above the bars indicate significant differences (P < 0.05) obtained by one-way ANOVA with Duncan’s test.
MdPIF7 interferes with the interaction between MdbZIP44/MdERF38 and MdMYB1
To uncover the molecular mechanism through which MdPIF7 negatively regulates MdbZIP44 and MdERF38-mediated anthocyanin accumulation, we examined the effect of MdPIF7 on the interaction between MdbZIP44/MdERF38 and MdMYB1. Competitive binding assays revealed that with the addition of MdPIF7, the interaction intensity between MdbZIP44/MdERF38 and MdMYB1 gradually weakened (Figure 10, A and B), but the interaction intensity between MdWRKY40/Teosinte branched1/Cycloidea/Proliferating 46 (MdTCP46) and MdMYB1 did not change significantly (Supplemental Figure S6). Further, LUC complementation imaging assays showed that the addition of MdPIF7 decreased the luminescence interaction between MdbZIP44/MdERF38 and MdMYB1 (Figure 10, C and D). These results demonstrate that MdPIF7 interferes with the interaction between MdbZIP44/MdERF38 and MdMYB1.
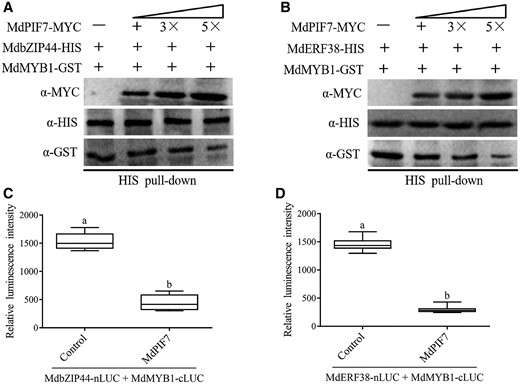
MdPIF7 affects the interaction between MdbZIP44/MdERF38 and MdMYB1. A, Competitive binding assays. The mixture of MdPIF7-MYC and MdMYB1-GST was added to immobilized MdbZIP44-HIS. The gradient indicates the increasing amount of MdPIF7-MYC. Western blot analysis was performed with HIS-tag(10E2) mouse (code: M30111L), GST-tag(12G8) mouse (code: M20007L), and MYC-tag(19C2) mouse antibodies. B, Competitive binding assays. The mixture of MdPIF7-MYC and MdMYB1-GST was added to immobilized MdERF38-HIS. The gradient indicates the increasing amount of MdPIF7-MYC. Western blot analysis was performed with HIS-tag(10E2) mouse (code: M30111L), GST-tag(12G8) mouse (code: M20007L), and MYC-tag(19C2) mouse antibodies. C, MdPIF7 inhibits the interaction between MdbZIP44 and MdMYB1. Control, co-expression of MdbZIP44-nLUC/MdMYB1-cLUC and pRI101 empty vector; +MdPIF7, co-expression of MdbZIP44-nLUC/MdMYB1-cLUC and MdPIF7-pRI101. The luminescence intensity of the control was used as the reference. The experiments were performed 3 times with similar results, and 8–10 N. benthamiana leaves were injected in each experiment. Error bars denote the standard deviations. Different letters above the bars indicate significant differences (P < 0.05) obtained by one-way ANOVA with Duncan’s test. Center line, median; box limits, upper and lower quartiles. D, MdPIF7 inhibits the interactions between MdERF38 and MdMYB1. Control, co-expression of MdERF38-nLUC/MdMYB1-cLUC; +MdPIF7, co-expression of MdERF38-nLUC/MdMYB1-cLUC/MdPIF7. The luminescence intensity of the control was used as the reference. The experiments were performed 3 times with similar results, and 8–10 N. benthamiana leaves were injected in each experiment. Error bars denote the standard deviations. Different letters above the bars indicate significant differences (P < 0.05) obtained by one-way ANOVA with Duncan’s test. Center line, median; box limits, upper and lower quartiles.
Discussion
Light signaling modulates many physiological and developmental processes in plants, including anthocyanin biosynthesis and hypocotyl growth (Kami et al., 2010; Jin and Zhu, 2019; Sanchez et al., 2020). In apple production, fruit coloration is an important index to measure the quality of apple fruits, and light is the most important environmental factor affecting anthocyanin biosynthesis and fruit coloration (Honda and Moriya, 2018). In addition, shade in the orchard is the key problem that limits the yield and quality of apple fruit. Therefore, it is of practical importance to study the effect of light signaling on the growth and development of fruit trees and the response mechanism of fruit trees to light signaling to improve the shade problem in orchards and improve the yield and quality of apple fruit. In this study, we identified two light signaling regulatory proteins, MdPIF7 and MdBBX23, in apple, and we systematically elucidated the molecular mechanism through which MdPIF7–MdBBX23–MdHY5 and MdPIF7–MdbZIP44/MdERF38–MdMYB1 modules regulate anthocyanin biosynthesis in apple and hypocotyl growth in ectopically expressed Arabidopsis.
Regulation of anthocyanin accumulation and hypocotyl growth by MdBBX23
Emerging evidence has suggested that the BBX proteins play central roles in light-mediated plant growth and development (Vaishak et al., 2019; Song et al., 2020). The BBX proteins function not only by directly modulating the expression of target genes, but also by forming a complex with their interaction partners. The BBX–HY5 module especially is considered a central regulatory hub in the regulation of photomorphogenesis and anthocyanin biosynthesis (Gangappa et al., 2013; Xu et al., 2016; Lin et al., 2018; Bursch et al., 2020; Song et al., 2020; Xu and Deng, 2020; Zhao et al., 2020). In Arabidopsis, BBX11, BBX21, BBX22, and BBX32 directly modulate the expression of HY5 (Holtan et al., 2011; Xu et al., 2016; Xu and Deng, 2020; Zhao et al., 2020). BBX20, BBX21, BBX22, BBX23, BBX24, BBX25, and BBX28 form a complex with HY5 (Datta et al., 2007, 2008; Indorf et al., 2007; Gangappa et al., 2013; Wei et al., 2016; Zhang et al., 2017; Lin et al., 2018). In apple, MdCOL11, MdBBX20, MdBBX22, and MdBBX37 were identified as interaction partners of MdHY5 (Bai et al., 2014; Fang et al., 2019; An et al., 2020a, 2020b). Although the BBX–HY5 module has been extensively studied in the model plant Arabidopsis, it has not been sufficiently studied in woody plants such as apple. In this study, we identified apple BBX protein MdBBX23 as a positive regulator of anthocyanin biosynthesis in apple and hypocotyl elongation inhibition in ectopically expressed Arabidopsis (Figure 4), which is consistent with the results in Arabidopsis (Zhang et al., 2017). A previous study revealed that MdBBX23 does not interact with MdHY5 in apple (An et al., 2020a). Here, we found that MdBBX23 could directly bind to the promoter of MdHY5 to activate its expression (Figure 5). Therefore, MdBBX23 positively regulates anthocyanin accumulation and hypocotyl elongation inhibition by activating MdHY5.
The role of MdPIF7 in the regulation of MdBBX23-mediated anthocyanin accumulation and hypocotyl growth
The PIF proteins play an important role in the transition from skotomorphogenesis to photomorphogenesis in plants (Castillon et al., 2007; Leivar et al., 2008b; Shin et al., 2009). As opposed to HY5, most of the PIF proteins are negative regulators of plant photomorphogenesis. In Arabidopsis, compared with the WT, the pif3, pif4, pif5, and pif7 mutant plants exhibited shorter hypocotyls and larger cotyledons (Huq and Quail, 2002; Monte et al., 2004; Khanna et al., 2007; Leivar et al., 2008a; Shin et al., 2009; Heng et al., 2019a; Yang et al., 2021). Apple PIF protein MdPIF1 promotes hypocotyl elongation in transgenic Arabidopsis (Zhou et al., 2017). PIF7 studies in Arabidopsis suggest that PIF7 plays an important role in the plant’s response to light, temperature, and circadian rhythms, as well as in the regulation of flowering (Leivar et al., 2008a, 2020; Kidokoro et al., 2009; Huang et al., 2018; Jiang et al., 2019; Zhang et al., 2019; Fiorucci et al., 2020; Paulišić et al., 2021; Yang et al., 2021). Here, we found that apple PIF protein MdPIF7 was a light-responsive protein and acted as a negative regulator of anthocyanin biosynthesis in apple and hypocotyl elongation inhibition in ectopically expressed Arabidopsis (Figures 1 and 2). Our results further enrich the functional study of PIF7.
The PIF proteins function by interacting with their partners (Toledo-Ortiz et al., 2003; Bu et al., 2011; Pham et al., 2018). In particular, PIFs, BBXs, and HY5 are known to interact in the regulation of anthocyanin biosynthesis, hypocotyl growth, and cotyledon opening. For instance, in Arabidopsis, the PIF3–HY5–BBXs cascade plays a key role in the regulation of photomorphogenesis (Shin et al., 2007; Zhang et al., 2017; Job and Datta, 2021). The phyB–BBX4–PIF3 module fine tunes red light-mediated photomorphogenesis (Heng et al., 2019a). BBX32, BZR1, and PIF3 regulate the opening and closing of cotyledons (Ravindran et al., 2021). In rose, PIF1, PIF3, and PIF4 suppress flowering by interacting with CO and weakening the activation activity of CO on FT (Sun et al., 2021). Here, we found that MdPIF7 interacted with MdBBX23 and antagonistically regulated anthocyanin biosynthesis and hypocotyl growth (Figures 2–4). Overexpression of MdPIF7 inhibited MdBBX23-mediated anthocyanin accumulation and hypocotyl elongation inhibition (Figure 6). We did not detect MdPIF7 binding to the G-box sites of MdHY5 promoter (Figure 5B), but observed that MdPIF7 attenuated the transcriptional activation of MdBBX23 on MdHY5 (Figure 7). The MdPIF7–MdBBX23–MdHY5 network completes the cascade regulation of anthocyanin biosynthesis and hypocotyl growth by MdPIF7 and MdBBX23.
A regulatory module of MdPIF7 modulating anthocyanin accumulation in apple
In apple, MdMYB1 and its alleles (MdMYB10 and MdMYBA) are considered to be key positive regulators of anthocyanin biosynthesis, and they facilitate anthocyanin biosynthesis by directly activating the expression of anthocyanin biosynthetic genes (Takos et al., 2006; Ban et al., 2007; Espley et al., 2007; Allan et al., 2008). Several different types of TFs, including MdbZIP44, MdWRKY40, MdERF38, and MdTCP46, have been identified to form complexes with MdMYB1 that synergistically regulate anthocyanin biosynthesis (An et al., 2020b). We found that MdPIF7 interacted with MdbZIP44 and MdERF38 (Figure 8), and overexpression of MdPIF7 reduced MdbZIP44- and MdERF38-promoted anthocyanin accumulation (Figure 9). Further studies showed that MdPIF7 negatively regulated MdbZIP44- and MdERF38-promoted anthocyanin accumulation by interfering with the interaction between MdbZIP44/MdERF38 and MdMYB1 (Figure 10). The MdPIF7–MdbZIP44/MdERF38–MdMYB1 module is a regulatory cascade for MdPIF7 to modulate anthocyanin biosynthesis in apple, and the discovery of this pathway indicates the diversity of MdPIF7 regulatory pathways.
In summary, we identified a light-responsive PIF protein, MdPIF7, and we investigated its potential regulatory cascade for modulating anthocyanin biosynthesis in apple and hypocotyl growth in ectopically expressed Arabidopsis. A hypothetical model was proposed to illustrate our findings (Figure 11). Under low light conditions, the expression of MdPIF7 is increased. MdPIF7 interacts with MdBBX23 to inhibit the transcriptional activation of MdBBX23 on MdHY5, thereby negatively regulating anthocyanin biosynthesis in apple and hypocotyl elongation inhibition in ectopically expressed Arabidopsis. Further, MdPIF7 interacts with MdbZIP44 and MdERF38 and interferes with the interaction between MdbZIP44/MdERF38 and MdMYB1, thereby negatively regulating anthocyanin accumulation in apple (Figure 11A; An et al., 2018b, 2020c). Under high light conditions, the protein stability of MdPIF7 decreases, leading to the release of MdBBX23–MdHY5 and MdbZIP44/MdERF38–MdMYB1 modules, promoting anthocyanin accumulation in apple and inhibiting hypocotyl elongation in ectopically expressed Arabidopsis (Figure 11B). The effect of light intensity on the interaction of MdPIF7 with MdBBX23 and MdbZIP44/MdERF38 remains to be further studied. MdPIF7–MdBBX23–MdHY5 and MdPIF7–MdbZIP44/MdERF38–MdMYB1 regulatory cascades ensure precise regulation of anthocyanin biosynthesis and hypocotyl growth in plants under different light intensity conditions. Two parallel regulatory pathways ensure the timely response of plants to the external environment.
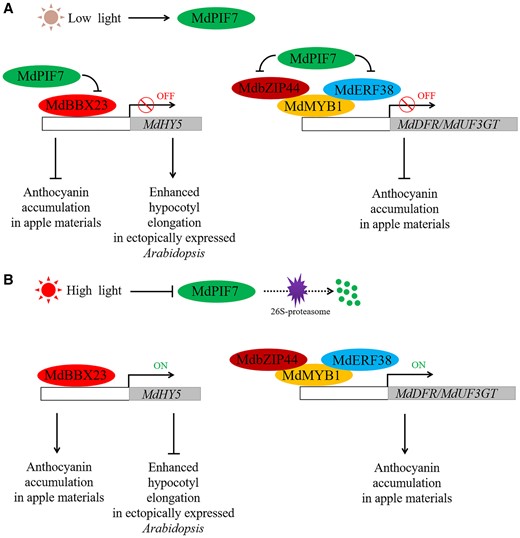
Role of MdPIF7 in the regulation of anthocyanin biosynthesis and hypocotyl growth. A, Under low light conditions, MdPIF7 interacts with MdBBX23 to inhibit the transcriptional activation of MdBBX23 on MdHY5, thereby negatively regulating anthocyanin biosynthesis in apple materials and hypocotyl elongation inhibition in ectopically expressed Arabidopsis. Furthermore, MdPIF7 interacts with MdbZIP44 and MdERF38 and interferes with the interaction between MdbZIP44/MdERF38 and MdMYB1, thereby negatively regulating anthocyanin accumulation in apple materials. B, Under high light conditions, the protein stability of MdPIF7 decreases, leading to the release of MdBBX23–MdHY5 and MdbZIP44/MdERF38–MdMYB1 regulatory cascades, promoting anthocyanin accumulation in apple materials and inhibiting hypocotyl elongation in ectopically expressed Arabidopsis.
Materials and methods
Plant materials and growth conditions
The plant materials used in this study included apple calli (Malus domestica, Orin), apple tissue culture seedlings (M. domestica, GL-3), apple fruits (M. domestica, Fuji), and Arabidopsis seedlings (A. thaliana, Col-0). The apple calli were grown in subculture medium (MS + 1.5 mg L−1 2,4-D + 0.4 mg L−1 6-BA) under dark conditions (24-h darkness, 24°C). Apple tissue culture seedlings were grown in subculture medium (MS + 1 mg L−1 6-BA + 0.2 mg L−1 NAA + 0.5 mg L−1 GA) under long-day conditions (16-h light/8-h darkness, 24°C). About 120 d after full bloom of “Fuji” apple fruits were collected for skin injection assays. Arabidopsis seedlings were grown in MS medium under long-day conditions (16-h light/8-h darkness, 22°C).
Vector construction and genetic transformation
Full-length MdPIF7, MdBBX23, MdbZIP44, and MdERF38 were cloned into the pRI101 or pCXSN-MYC vector to generate 35S:MdPIF7, 35S:MdBBX23-MYC, 35S:MdbZIP44-MYC, and 35S:MdERF38-MYC overexpression vectors. The amplified fragments of MdPIF7-Anti and MdBBX23-Anti were cloned into the pCXSN vector to generate 35S:MdPIF7-Anti and 35S:MdBBX23-Anti antisense suppression vectors. In brief, MdPIF7, MdBBX23, MdbZIP44, MdERF38, MdPIF7-Anti, and MdBBX23-Anti fragments were amplified with primers of MdPIF7-F/R, MdBBX23-F/R, MdbZIP44-F/R, MdERF38-F/R, MdPIF7-Anti-F/R, and MdBBX23-Anti-F/R. The amplified fragment was connected with the pMD18-T clone vector (Takara, Dalian, China). The positive Escherichia coli was sequenced in Sangon Biotech (Shanghai, China) Co., Ltd. The correctly sequenced plasmid and the overexpression vector were digested simultaneously. The amplified fragment was linked to the overexpression vector using T4 DNA ligase (Takara, Shiga, Japan). PCR was used to screen the recombinant plasmid. Primers are shown in Supplemental Table S2.
Transgenic apple calli were obtained by Agrobacterium-mediated transformation. WT calli were incubated with Agrobacterium for 0.5 h, then transferred to selective medium for 30 d. Transgenic calli were identified by RT-qPCR analysis (An et al., 2020a). Transgenic apple leaves were obtained by Agrobacterium-mediated transformation and vacuum extraction. Leaves with consistent growth were collected from WT apple tissue culture seedlings. The detached leaves were incubated with Agrobacterium and vacuumed for 0.5 h, then transferred to selective media for another 2 d. Transgenic leaves were identified by RT-qPCR analysis (An et al., 2020a). The virus vectors were injected into the apple skin for transient expression. Apple skin injection was identified by RT-qPCR analysis (An et al., 2018a). Transgenic Arabidopsis was obtained by inflorescence infection. Transgenic Arabidopsis was identified by RT-qPCR analysis (Clough and Bent, 1998). Primers are shown in Supplemental Table S2.
RNA isolation and RT-qPCR analysis
The total RNA of apple calli, detached apple leaves, apple skin, and Arabidopsis seedlings was extracted with a TRIzol RNA separation reagent (Thermo Fisher Scientific, Waltham, MA, USA). The cDNA was obtained using a reverse transcription kit (Takara). RT-qPCR analysis was performed to examine the gene expression levels with a Real-Time PCR system (Applied Biosystems, Foster City, CA, USA). RT-qPCR was conducted with three biological replicates and three technical replicates (n = 9). MdACTIN-1 and MdACTIN-2 were used as reference genes to evaluate degree of normalization. Primers are shown in Supplemental Table S2.
Y2H assays
Full-length MdBBX10, MdBBX20, MdBBX22, MdBBX23, MdBBX24, MdBBX25, MdBBX33, MdBBX43, MdMYB1, MdMYB9, MdMYB11, MdHY5, MdbZIP44, MdWRKY40, MdERF38, and MdTCP46 were cloned into the pGAD424 vector (MdBBX10-AD, MdBBX20-AD, MdBBX22-AD, MdBBX23-AD, MdBBX24-AD, MdBBX25-AD, MdBBX33-AD, MdBBX43-AD, MdMYB1-AD, MdMYB9-AD, MdMYB11-AD, MdHY5-AD, MdbZIP44-AD, MdWRKY40-AD, MdERF38-AD, and MdTCP46-AD). Full-length MdPIF1, MdPIF3, MdPIF4, MdPIF6, MdPIF7, and MdPIF8 were cloned into the pGBT9 vector (MdPIF1-BD, MdPIF3-BD, MdPIF4-BD, MdPIF6-BD, MdPIF7-BD, and MdPIF8-BD). Primers are shown in Supplemental Table S2. Recombinant plasmids were introduced into yeast strain Y2H Gold, and the transformed yeast cells were grown on selective medium (-T/-L medium or -T/-L/-H/-A medium).
Pull-down assays
Full-length MdBBX23 and MdPIF7 were cloned into the pET32a or pGEX4T-1 vector to generate MdBBX23-pET32 and MdPIF7-pGEX4T-1. Recombinant plasmids were introduced into E. coli BL21. The MdBBX23–HIS and MdPIF7–GST fusion proteins were obtained by isopropyl-β-d-thiogalactoside (IPTG) induction. A glutathione purification kit (Thermo Fisher Scientific) was used for the pull-down assays. In brief, the MdBBX23-HIS and MdPIF7-GST proteins were incubated in a glutathione affinity gel chromatography column for 3 h. Elution samples were collected for western blot analysis with HIS-tag(10E2) mouse (code: M30111L) and GST-tag(12G8) mouse (code: M20007L) antibodies. All antibodies are provided by Abmart Biomedicine (Shanghai, China) Co., Ltd.
Competitive binding assays
Full-length MdbZIP44, MdERF38, and MdMYB1 were cloned into the pET32a or pGEX4T-1 vector to generate MdbZIP44-pET32, MdERF38-pET32a, and MdMYB1-pGEX4T-1. Recombinant plasmids were introduced into E. coli BL21. The MdbZIP44-HIS, MdERF38-HIS, and MdMYB1–GST fusion proteins were obtained by IPTG induction. The MdPIF7-MYC protein was extracted from MdPIF7-OX apple calli with an immunoprecipitation kit (Thermo Fisher Scientific). A Ni-Agarose histidine purification kit (CWBIO, Beijing, China) was used for the competitive binding assays. In brief, the MdPIF7-MYC, MdbZIP44-HIS, MdERF38-HIS, and MdMYB1-GST proteins were incubated in a polyhistidine affinity gel chromatography column for 3 h. Elution samples were collected for western blot analysis with HIS-tag(10E2) mouse (code: M30111L), GST-tag(12G8) mouse (code: M20007L), and MYC-tag(19C2) mouse antibodies. All antibodies are provided by Abmart Biomedicine (Shanghai, China) Co., Ltd.
BiFC assays
Full-length MdPIF7 was cloned into the pSPYNE-35S/pUC-SPYNE vector to generate MdPIF7-YFPN. Full-length MdBBX23, MdbZP44, and MdERF38 were cloned into the pSPYCE-35S/pUC-SPYCE vector to generate MdBBX23-YFPC, MdbZIP44-YFPC, and MdERF38-YFPC, respectively. Recombinant plasmids were introduced into A. tumefaciens strain LBA4404 and expressed in onion epidermal cells. YFP fluorescence was observed using a fluorescence microscope at a wavelength of 490–540 nm (FV3000; Olympus, Tokyo, Japan).
EMSAs
The MdBBX23–HIS fusion protein and biotin-labeled and mutant probes were prepared for EMSAs. The probes were manufactured by Sangon Biotech (Shanghai) Co., Ltd. The fusion protein and probe were incubated at 24°C for 0.5 h, and the free probe and bound probe were separated by acrylamide gel electrophoresis. The unlabeled probe was used as competitor. The probe blot was transferred onto a N+ nylon membrane and observed using a protein imaging system (ChemiDoc XRS+, Bio-Rad, Hercules, CA, USA).
Dual LUC assays
Full-length MdBBX23 and MdPIF7 were cloned into the pGreenII 62-SK vector, and the promoter sequence of MdHY5 was cloned into the pGreenII 0800-LUC vector. Recombinant plasmids were introduced into A. tumefaciens strain LBA4404 and expressed in tobacco leaves. LUC activity was determined with a LUC detection kit (Promega, Madison, WI, USA).
LUC complementation imaging assays
Full-length MdbZIP44 and MdERF38 were cloned into the pCAMBIA1300-nLUC vector, and full-length MdMYB1 was cloned into the pCAMBIA1300-cLUC vector. Recombinant plasmids were introduced into A. tumefaciens strain LBA4404 and expressed in tobacco leaves. Fluorescence was observed using an imaging system (Xenogen, Alameda, CA, USA, IVIS Spectrum), and LUC was determined with a LUC detection kit (Promega).
Coloration assays
The coloration assays of apple calli and leaves were performed as previously described (An et al., 2020a). The 15-d-old apple calli were evenly spread on subculture media and placed in the dark for one week, then placed in a light incubator (photon flux density: 300 μmol·m−2·s−1). The coloration of apple calli was observed. The detached apple leaves were tiled onto the subculture media, and placed in a light incubator (photon flux density: 300 μmol·m−2·s−1). The coloration of apple leaves was observed.
Apple skin injection assays
The apple skin injection assays were performed as previously described (An et al., 2018a, 2018b). Full-length MdPIF7 and MdBBX23 were cloned into the IL60-2 overexpression viral vector. IL60‐1was used as helper vectors. pIR: IL60-1 + IL60-2, MdPIF7-pIR: IL60-1 + MdPIF7-IL60-2, MdBBX23-pIR: IL60-1 + MdBBX23-IL60-2. pIR, MdPIF7, and MdBBX23-pIR were injected into the epidermis of apple fruit with a 1mL medical syringe. The injected apple fruits were placed in a light incubator (photon flux density: 300 μmol·m−2·s−1). Anthocyanin accumulation of apple skin was observed.
Determination of anthocyanin content
Anthocyanin determination of apple calli, detached apple leaves, or apple skin was performed as previously described (An et al., 2020a). Apple calli or detached apple leaves were incubated in anthocyanin extraction buffer. Anthocyanin was measured using a spectrophotometer (SHIMADZU, Kyoto, Japan; UV-1600).
Statistical analyses
Statistical analysis was determined by one-way analysis of variance (ANOVA) with Duncan’s test.
Accession numbers
Sequence data can be found using the accession number below: MdPIF1 (MDP0000289642), MdPIF3 (MDP0000290263), MdPIF4 (MDP0000198404), MdPIF6 (XP_008340273.1), MdPIF7 (XP_008393195.2), MdPIF8 (MDP0000439540), MdBBX10 (MDP0000733075), MdBBX20 (MDP0000177126), MdBBX22 (MDP0000298804), MdBBX23 (MDP0000222881), MdBBX24 (MDP0000800387), MdBBX25 (MDP0000901915), MdBBX33 (MDP0000697407), MdBBX43 (MDP0000140484), MdMYB1 (MDP0000259614), MdMYB9 (MDP0000210851), MdMYB11 (MDP0000437717), MdHY5 (MDP0000586302), MdbZIP44 (MDP0000521934), MdWRKY40 (MDP0000177906), MdERF38 (MDP0000153436), and MdTCP46 (MDP0000319941).
Supplemental data
The following materials are available in the online version of this article.
Supplemental Figure S1. Sequence alignment of the PIF7 proteins obtained from the NCBI database.
Supplemental Figure S2. Phylogenetic tree of 12 PIF7 proteins.
Supplemental Figure S3. Y2H assays show the autonomic activation of MdPIF proteins.
Supplemental Figure S4. Identification of the transgenic plant materials.
Supplemental Figure S5. Detection of the light-dependent regulation of anthocyanin accumulation by MdPIF7.
Supplemental Figure S6. MdPIF7 does not affect the interaction between MdWRKY40/MdTCP46 and MdMYB1.
Supplemental Table S1. The promoter sequence of MdHY5.
Supplemental Table S2. Primers used for gene expression analysis and vector construction.
Funding
This work was financially supported by grants from the National Key Research and Development Program (2018YFD1000100), Natural Science Foundation of Shandong Province (ZR2019PC004), and the open funds of the State Key Laboratory of Crop Genetics and Germplasm Enhancement (ZW202008).
Conflict of interest statement. The authors declare that they have no conflicts of interest.
J.P.A. conceived and designed the experiments. J.P.A., Y.L., and X.W.Z. performed the research. J.P.A., C.X.Y., X.L., P.F.Z., L.S., G.L.W., X.F.W., and Y.Y.L. analyzed the data. J.P.A. wrote the paper.
The author responsible for distribution of materials integral to the findings presented in this article in accordance with the policy described in the Instructions for Authors (https://dbpia.nl.go.kr/plphys/pages/general-instructions) is: Jian-Ping An ([email protected]).
References
Author notes
Senior authors.
These authors contributed equally (Y.L. and X.W.Z.).