-
PDF
- Split View
-
Views
-
Cite
Cite
Hao-Xun Chang, Ruijuan Tan, Glen L. Hartman, Zixiang Wen, Hyunkyu Sang, Leslie L. Domier, Steven A. Whitham, Dechun Wang, Martin I. Chilvers, Characterization of Soybean STAY-GREEN Genes in Susceptibility to Foliar Chlorosis of Sudden Death Syndrome, Plant Physiology, Volume 180, Issue 2, June 2019, Pages 711–717, https://doi.org/10.1104/pp.19.00046
- Share Icon Share
Fusarium virguliforme causes sudden death syndrome (SDS) of soybean (Glycine max) in the United States. This fungal pathogen inhabits soil and produces multiple phytotoxins, which are translocated from infected roots to leaves, causing SDS foliar chlorosis and necrosis (Hartman et al., 2015). Because SDS foliar symptoms are solely induced by phytotoxins, it represents a unique pathosystem to study plant-phytotoxin interactions (Chang et al., 2016). SDS foliar symptoms typically appear near flowering through late reproductive growth stages, with chlorotic spots that gradually develop into interveinal chlorosis and necrosis (Fig. 1A). The sudden appearance of SDS foliar symptoms not only explains the origin of the disease name, but also reflects the difficulty of early detection in managing this disease. Yield reductions caused by SDS have been documented at 5% to15%, and the economic loss was estimated up to $669 million U.S. dollars in a single year (Navi and Yang, 2016). Seed treatments have been used to manage SDS, but performance differs by year and location. Alternatively, partially resistant soybean cultivars provide a sustainable option for SDS management, but the genetic architecture of SDS resistance is quantitative and complicated. Among more than 80 quantitative trait loci reported for SDS, only a few quantitative trait loci are reproducible due to the complexity of SDS etiology and environmental interactions (Chang et al., 2018).
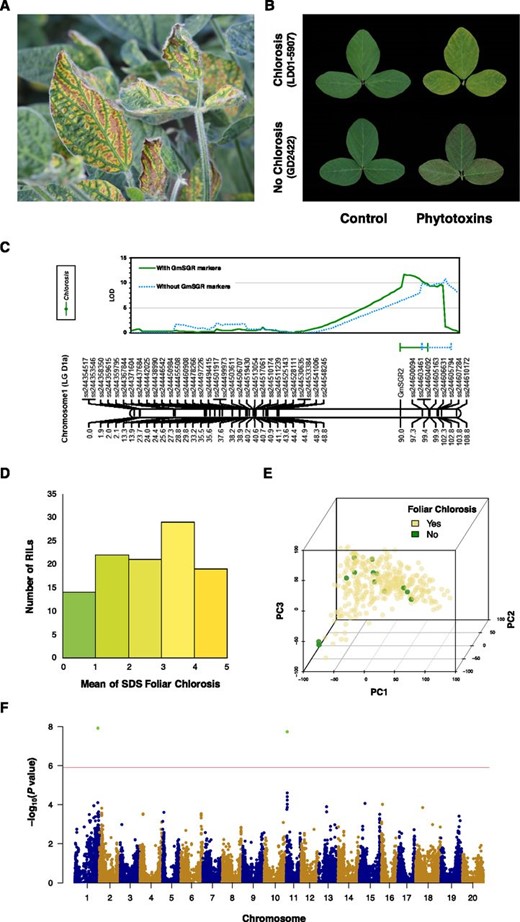
Symptomatic and genetic characterization for soybean resistance to SDS foliar chlorosis. A, Typical SDS foliar symptoms observed in fields include interveinal chlorosis and necrosis. Severely impacted leaves also display leaf marginal curling and premature defoliation. B, Soybean varieties exhibited different SDS foliar symptoms. In response to phytotoxins produced by F. virguliforme, LD01-5907 displayed chlorosis while GD2422 displayed necrosis but no chlorosis. C, Linkage mapping using the RILs derived from GD2422 (no chlorosis) × LD01-5907 (chlorosis). One locus with seven SNPs was significantly associated with the SDS foliar chlorosis. The significant threshold was determined by permutation with 1,000 iterations at logarithm of the odds 3.5. The blue line and the blue bar indicate the 95% confidence interval of the Chlorosis locus without GmSGR markers. The green line and the green bar indicate the 95% confidence interval of the Chlorosis locus with GmSGR markers. D, Frequency distribution regarding the mean of SDS foliar chlorosis in the RIL population used in linkage mapping. E, Principal component analysis showed a scattered distribution for the germplasm accessions that exhibited resistance to SDS foliar chlorosis. Zero principal component was included to control population structure. F, Manhattan plot identified two SNPs, ss715580368 on Chr01 and ss715609614 on Chr11, at false discovery rate < 0.05 (green dots) and both exceeded the Bonferroni threshold (red horizontal line).
Genetic approaches for resistance breeding in plants generally involve the addition of Resistance (R) loci or removal of Susceptibility (S) loci. The inheritance of the R or S loci is generally dominant and qualitative. Both R and S loci have major effects on determining disease outcome, which depends on a one-to-one molecular interaction between a pathogen avirulence effector and a plant R protein, or between a pathogen necrotrophic effector and a plant S protein. Although these models have simplified our understanding of plant-microbe interactions, plant resistance to necrotrophic pathogens is mostly quantitative and governed by numerous loci with minor effects; therefore, it is difficult to breed for resistance to necrotrophic pathogens. Accordingly, advanced knowledge of additional genetic models or molecular mechanisms is needed to understand the phenotypic observations. One of the resistance mechanisms that has received less attention is the R genes that operate by loss-of-susceptibility mechanisms. For example, in a recent study on cucumber (Cucumis sativus), Wang et al. (2019) discovered a single-nucleotide mutation in cucumber STAY-GREEN gene (CsSGR) using genetic mapping. The mutation in CsSGR caused a loss-of-susceptibility phenotype, which not only prevented chlorosis but also provided durable and broad-spectrum disease resistance. Here, we applied similar experimental approaches, using linkage mapping and a genome-wide association study (GWAS), to dissect soybean resistance to phytotoxin-induced SDS foliar chlorosis. Two soybean STAY-GREEN genes (GmSGR1 and GmSGR2) were found to be associated with development of chlorosis, and resistance to SDS foliar chlorosis was attributed to a double mutation of the STAY-GREEN genes. Unlike the cucumber CsSGR mutant that stayed green with no symptomatic defects, soybeans with a double mutation of GmSGR1 and GmSGR2 stayed green but displayed necrosis and reduced photosynthesis in response to phytotoxins produced by F. virguliforme. These results demonstrated that STAY-GREEN, as an R gene with a loss-of-susceptibility mechanism, may have different merits in resistance breeding depending on the allele types or the plant species.
Chlorosis and necrosis are two common symptoms that can be induced by phytotoxins produced by plant pathogens. Studies characterizing plant resistance to phytotoxins have identified several dominant S loci. One study in corn (Zea mays) screened foliar symptoms against a phytotoxic culture filtrate of Cochliobolus heterostrophus and mapped this response to a dominant S locus (Xiaodong et al., 2018). A study in rice (Oryza sativa) using linkage mapping for foliar necrosis caused by phytotoxins of Rhizoctonia solani identified a single dominant S locus, Rsn1 (Costanzo et al., 2011). Screening and mapping susceptibility to the phytotoxic victorin produced by Cochliobolus victoriae discovered a dominant S locus, Vb, in oats (Avena sativa; Lorang et al., 2007). Previous studies on Stagonospora nodorum blotch, a wheat (Triticum sp.) disease caused by Parastagonospora nodorum, identified a gene located in the dominant Tsn1 locus; this gene encodes a protein containing a nucleotide binding Leu-rich repeat domain and a Ser/Thr protein kinase domain that indirectly recognizes the necrotrophic effector SnToxA and triggers foliar chlorosis (Faris et al., 2010). Moreover, a wall-associated kinase encoded by a gene in the dominant Snn1 locus directly recognizes another necrotrophic effector, SnTox1, and triggers foliar necrosis (Shi et al., 2016). There are six additional necrotrophic effectors, which are paired with their corresponding dominant S loci in the wheat-P. nodorum system, and three similar interactions have been reported in wheat tan spot disease caused by Pyrenophora tritici-repentis (Liu et al., 2017). These studies provided a foundation of knowledge for the roles of S loci in plant diseases, and it has been shown that deletion of the S loci in wheat breeding lines reduces plant sensitivity to phytotoxins, demonstrating the application of modifying susceptibility in managing plant diseases (Vleeshouwers and Oliver, 2014).
In screening 340 accessions of the U.S. Department of Agriculture (USDA) soybean germplasm for foliar resistance to phytotoxins produced by F. virguliforme, 14 accessions were found to exhibit resistance to chlorosis. This resistance to SDS foliar chlorosis was also observed in a soybean variety GD2422 (Fig. 1B), which was a parent used in generating a recombinant inbred line (RIL) population with another parent,LD01-5907, which displayed susceptibility to SDS foliar chlorosis (Tan et al., 2018). These soybean RILs therefore became useful materials to test our hypothesis that SDS foliar chlorosis is controlled by a soybean S locus. A single Chlorosis locus on chromosome 1 (Chr01) and seven associated single nucleotide polymorphisms (SNPs) were found by conducting linkage mapping on the 129 RILs derived from GD2422 (exhibiting no chlorosis) and LD01-5907 (exhibiting chlorosis; Fig. 1C–D); Supplemental Table S1). Considering that SDS foliar chlorosis might be a qualitative trait, a GWAS was performed using binary data of SDS foliar chlorosis (absence or presence) from the USDA soybean germplasm panel of 340 individuals. GWAS identified two SNPs on Chr01 and Chr11 associated with chlorosis (Fig. 1E–F; Supplemental Table S2). The significant SNP (ss715580368) on Chr01 was located within the Chlorosis locus (Supplemental Fig. S1), and 113 predicted gene models were found in this locus. For another significant SNP (ss715609614) on Chr11, there were 26 predicted gene models within its linkage disequilibrium block (200 kb; Vuong et al., 2015). The intervals of the Chlorosis locus on Chr01 and the linkage disequilibrium block on Chr11 are syntenic to each other, and a pair of homologous genes, GmSGR1 (Glyma.11G027400) and GmSGR2 (Glyma.01G214600), which are annotated as “non-yellowing” or the STAY-GREEN gene, were found in SoyBase (Supplemental Fig. S2; Supplemental Table S3).
The stay-green phenotype was first discovered by Gregor Mendel as a discrete and recessive trait for exhibiting green cotyledons and green seed coats of pea (Pisum sativum). The phenotype was mapped to the I locus of pea and was functionally linked to the STAY-GREEN gene (Armstead et al., 2006, 2007; Sato et al., 2007). STAY-GREEN genes exist widely in different plant species, and the orthologous STAY-GREEN gene in Arabidopsis (Arabidopsis thaliana) encodes a magnesium (Mg)-dechelatase, which removes Mg from chlorophyll a in the first step of chlorophyll degradation and leaf senescence (Shimoda et al., 2016). Mutations in the Arabidopsis STAY-GREEN genes prevent chlorophyll degradation, which results in a stay-green phenotype when the plant ordinarily would be senescing. In soybean, a mutated allele (the d2 allele, which contains an insertion and exon duplication) of GmSGR1 and a mutated allele (the d1 allele, which contains a deletion of thymine that causes a frameshift in the exon) of GmSGR2 was reported (Fang et al., 2014). Soybean varieties exhibiting resistance to SDS foliar chlorosis (including the parental line GD2422 and the 14 soybean germplasm accessions in the GWAS panel) were confirmed as d1d1d2d2 double mutants by sequencing the genomic DNA of GmSGR1 and GmSGR2 (Supplemental Fig. S3; Supplemental Table S4). The population of 129 RILs was individually genotyped for the D1/d1 or D2/d2 allele by PCR and sequencing (Supplemental Table S5) and when the D1/d1 and D2/d2 alleles were used as genetic markers in a second round of linkage mapping, the mapping results discovered the same Chlorosis locus with higher logarithm of the odds, higher explained variation (R2), and a narrower confidence interval with two SNPs associated with SDS foliar chlorosis (Supplemental Fig. S4; Supplemental Table S1). The genomic DNA sequences and remapping results supported the association between soybean STAY-GREEN genes and SDS foliar chlorosis.
In addition, the mean separation for SDS foliar chlorosis showed that a single D1 allele (GmSGR2 on Chr01) was sufficient to contribute equivalent chlorosis severity of the parental line LD01-5907, whereas a single D2 allele (GmSGR1 on Chr11) contributed half of the chlorosis severity (Fig. 2A). This observation was consistent with the GWAS results that the phenotypic variance explained by the SNP on Chr01 was estimated to be higher than the SNP on Chr11 (Supplemental Table S2). Expression analysis revealed that up-regulation of GmSGR1 and GmSGR2 in LD01-5907 was significantly higher than in GD2422 in response to phytotoxins produced by F. virguliforme (Fig. 2B–C). Similar up-regulation levels and expression patterns over 3 d in both LD01-5907 and GD2422 were observed for GmSGR1 and GmSGR2, indicating partial redundancy between GmSGR1 and GmSGR2. To further confirm that the stay-green phenotype was due to the lack of chlorophyll degradation, a time-course experiment was conducted to quantify the relative chlorophyll content. When grown through to maturity, control plants of LD01-5907 gradually displayed chlorosis as part of normal senescence, but phytotoxins accelerated chlorophyll degradation within 3 d (Fig. 3A). On the other hand, the control and phytotoxin-treated plants of GD2422 maintained their relative chlorophyll content over time (Fig. 3B). Accordingly, this resistance to SDS foliar chlorosis was caused by a failure of leaf senescence and chlorophyll degradation due to double mutations in soybean STAY-GREEN genes. In other words, phytotoxins produced by F. virguliforme induce leaf senescence and chlorophyll degradation to induce SDS foliar chlorosis.
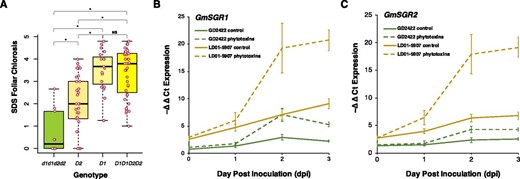
Mean separation and gene expression of soybean STAY-GREEN genes GmSGR1 and GmSGR2. A, Mean separation of SDS foliar chlorosis by the D1 allele (GmSGR2) and D2 allele (GmSGR1). One-way ANOVA was used to test the significance of mean separation in SDS foliar chlorosis severity to alleles. *Significant difference at P < 0.01; NS indicates a nonsignificant difference. The bars indicate the standard errors of SDS foliar chlorosis for each allele. B, GmSGR1 expression in GD2422 and LD01-5907 in response to phytotoxins produced by F. virguliforme. C, GmSGR2 expression in GD2422 and LD01-5907 in response to phytotoxins produced by F. virguliforme. The bars indicate the standard errors of GmSGR1 or GmSGR2 expression for each time point and treatment.
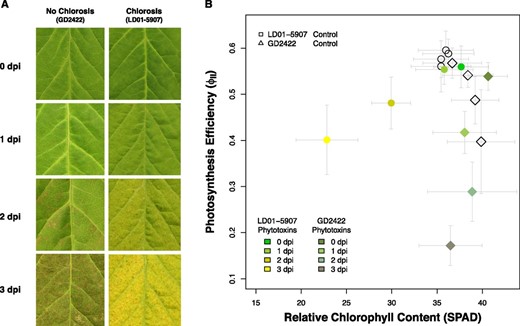
Photosynthesis efficiency and relative chlorophyll content in response to the culture filtrates of F. virguliforme. dpi, Days post inoculation. A, Development of SDS foliar chlorosis or necrosis over 3 d in response to phytotoxins produced by F. virguliforme. B, Soybean variety LD01-5907 (wild type) exhibited gradual chlorophyll degradation induced by phytotoxins produced by F. virguliforme. Soybean variety GD2422 (d1d1d2d2 genotype) maintained its relative chlorophyll content (SPAD, Soil-Plant Analyses Development), but the photosynthesis efficiency decreased significantly (one-way ANOVA test, P < 0.01). The vertical and horizontal bars indicate the standard errors of the photosynthesis efficiency and the relative chlorophyll content for each time point and treatment.
STAY-GREEN genes have been recently reported as a new group of R genes with loss-of-susceptibility mechanisms in cucumber against downy mildew, bacterial angular leaf spot, and anthracnose (Wang et al., 2019). Other examples of R genes with loss-of-susceptibility mechanisms include the Mildew resistance locus o (Mlo) in multiple plants for powdery mildews (Kusch and Panstruga, 2017), and Lr34 and Lr67 in wheat for rusts and powdery mildew (Krattinger et al., 2009; Moore et al., 2015). Unlike the S genes described in the wheat-P. nodorum pathosystem, which depends on the mechanism of necrotrophic effector-triggered susceptibility that requires a specific and compatible interaction between a necrotrophic effector and its corresponding S gene, R genes with loss-of-susceptibility mechanisms provide broad-spectrum disease resistance and have been suggested to be more durable. For instance, barley (Hordeum vulgare) Mlo has been used for over 20 years (Kusch and Panstruga, 2017), and cucumber STAY-GREEN has been used for more than 50 years (Wang et al., 2019). However, most plant varieties containing R genes with loss-of-susceptibility mechanisms were reported to have some phenotypic defects. Barley varieties with the mlo locus displayed necrotic flecks and yield loss (Kusch and Panstruga, 2017; Wang et al., 2019), and wheat varieties containing Lr34 or Lr67 displayed leaf tip necrosis (Krattinger et al., 2009; Moore et al., 2015). In our study, soybean varieties carrying STAY-GREEN alleles with a loss-of-susceptibility mechanism (d1d1d2d2 genotype) exhibited interveinal necrosis, and the leaves became dehydrated and fragile in response to the phytotoxin treatment. Moreover, the parent GD2422 (d1d1d2d2 genotype) was more susceptible in SDS field trials than another parent, LD01-5907 (Tan et al., 2018), and phytotoxins decreased photosynthesis efficiency in GD2422 faster than in LD01-5907 (Fig. 3B). It has been suggested that overgeneration of reactive oxygen species causes toxicity and induces necrosis when a plant is senescent but chlorophyll is not being degraded (Mur et al., 2010). Alternatively, the foliar necrosis in the STAY-GREEN mutants of soybean may be species dependent. For example, it has been reported that STAY-GREEN mutants of soybean were susceptible to drought, but STAY-GREEN mutants of rice and sorghum (Sorghum bicolor) were tolerant to drought (Luquez and Guiamét, 2002). In addition, the function of STAY-GREEN genes may be diversified in legume species as the STAY-GREEN mutations not only disabled chlorophyll degradation in leaves, but also reduced nodule senescence in roots (Zhou et al., 2011).
Although it remains possible that additional S loci exist in soybean that are recognized by necrotrophic effectors or phytotoxins produced by F. virguliforme to trigger foliar chlorosis, our genetic mapping attributed the phenotypic difference in our RIL population and GWAS panel to the layer of STAY-GREEN genes instead of the layer of necrotrophic effector-triggered susceptibility for SDS foliar chlorosis (Fig. 4). It is worth noting that soybean STAY-GREEN genes were rarely detected in previous SDS resistance mapping studies (Chang et al., 2018), even when the same RIL population was screened in the fields (Tan et al., 2018). Although the environment and other factors may play an important role in field screening, a possible reason could be the method and precision in phenotyping. When screening SDS resistance in the fields, most studies used the disease index system, considering a combination of disease severity (including chlorosis, necrosis, wilting, and defoliation) and normalized to the disease occurrence (percentage of SDS in a field); on the other hand, this study phenotyped specifically for SDS foliar chlorosis (Supplemental Methods), which may enable the detection of soybean STAY-GREEN genes. Future studies deploying precise and reproducible phenotyping methods may further illuminate the genetic architecture of SDS resistance and may explore if any soybean germplasm carries S loci to support the existence of a necrotrophic effector-triggered susceptibility mechanism in the SDS foliar necrosis or chlorosis.
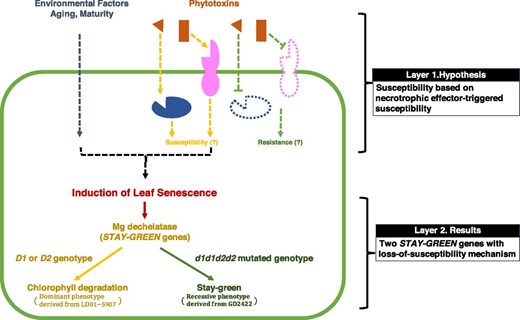
Schematic model of phytotoxin-induced SDS foliar chlorosis. The molecular mechanism for the susceptibility to SDS foliar chlorosis was hypothesized on the interaction between a cytoplasmic effector (orange triangle) and a cytoplasmic S receptor (blue ellipse) or an apoplastic effector (orange rectangle) and an intermembrane S receptor (pink ellipse). However, the phenotypic and genetic differences derived from GD2422 (no chlorosis) × LD01-5907 (chlorosis) RIL population and the USDA germplasm panel were attributed to the soybean STAY-GREEN genes with a loss-of-susceptibility mechanism rather than the S genes with a necrotrophic effector-triggered susceptibility mechanism.
While the role of STAY-GREEN genes with a loss-of-susceptibility mechanism has become apparent in arresting chlorosis development, it is worth noting that the breeding merits of STAY-GREEN genes with a loss-of-susceptibility mechanism can differ depending on the allele types or the plant species. Because the loss-of-susceptibility mechanism of CsSGR with a Q108R mutation exhibited no defective symptoms with durable resistance for disease management (Wang et al., 2019), engineering soybean STAY-GREEN genes using a different mutated allele (such as Q108R instead of d1d1d2d2) to lose susceptibility may provide resistance to SDS foliar chlorosis without detrimental agronomic traits.
SUPPLEMENTAL DATA
The following supplemental information is available:
Supplemental Methods- Experimental procedures.
Supplemental Table S1. Significant SNPs in the linkage mappings.
Supplemental Table S2. Significant SNPs in the genome-wide association study.
Supplemental Table S3. Twenty-six candidate gene models on chromosome 11.
Supplemental Table S4. Primers used in this study.
Supplemental Table S5. Phenotypes and genotypes for the 129 RILs.
Supplemental Figure S1. Candidate genes within the Chlorosis locus on chromosome 1.
Supplemental Figure S2. Candidate genes within the LD region centering ss715609614 on chromosome 11.
Supplemental Figure S3. Genomic sequences of GmSGR1 and GmSGR2.
Supplemental Figure S4. The interval of Chlorosis locus with and without GmSGR markers.
LITERATURE CITED
Author notes
This work was supported in part by Michigan Soybean Promotion Committee, North Central Soybean Research Program, and USDA-ARS.
Author for contact: [email protected].
Senior author.
The author responsible for distribution of materials integral to the findings presented in this article in accordance with the policy described in the Instructions for Authors (www.plantphysiol.org) is: Martin I. Chilvers ([email protected]).
H.-X.C. contributed to the photosynthesis measurements and wrote the manuscript; H.-X.C., R.T., and Z.W. contributed to the linkage mapping; H.-X.C. and G.L.H. phenotyped the U.S. Department of Agriculture germplasms and contributed to the genome-wide association study; H.-X.C. and H.S. contributed to the expression analyses; H.-X.C. and M.I.C. phenotyped the recombinant inbred line population; R.T., Z.W., and D.W. developed the recombinant inbred line population and performed genotyping; R.T., G.L.H., Z.W., H.S., L.L.D., S.A.W., D.W., and M.I.C. reviewed and edited the article; M.I.C. coordinated and supervised the research project.
Articles can be viewed without a subscription.