-
PDF
- Split View
-
Views
-
Cite
Cite
Marcia GonzÁlez-Teuber, María J. Pozo, Alexander Muck, Ales Svatos, Rosa M. Adame-Álvarez, Martin Heil, Glucanases and Chitinases as Causal Agents in the Protection of Acacia Extrafloral Nectar from Infestation by Phytopathogens, Plant Physiology, Volume 152, Issue 3, March 2010, Pages 1705–1715, https://doi.org/10.1104/pp.109.148478
- Share Icon Share
Abstract
Nectars are rich in primary metabolites and attract mutualistic animals, which serve as pollinators or as an indirect defense against herbivores. Their chemical composition makes nectars prone to microbial infestation. As protective strategy, floral nectar of ornamental tobacco (Nicotiana langsdorffii × Nicotiana sanderae) contains “nectarins,” proteins producing reactive oxygen species such as hydrogen peroxide. By contrast, pathogenesis-related (PR) proteins were detected in Acacia extrafloral nectar (EFN), which is secreted in the context of defensive ant-plant mutualisms. We investigated whether these PR proteins protect EFN from phytopathogens. Five sympatric species (Acacia cornigera, A. hindsii, A. collinsii, A. farnesiana, and Prosopis juliflora) were compared that differ in their ant-plant mutualism. EFN of myrmecophytes, which are obligate ant-plants that secrete EFN constitutively to nourish specialized ant inhabitants, significantly inhibited the growth of four out of six tested phytopathogenic microorganisms. By contrast, EFN of nonmyrmecophytes, which is secreted only transiently in response to herbivory, did not exhibit a detectable inhibitory activity. Combining two-dimensional sodium dodecyl sulfate-polyacrylamide gel electrophoresis with nanoflow liquid chromatography-tandem mass spectrometry analysis confirmed that PR proteins represented over 90% of all proteins in myrmecophyte EFN. The inhibition of microbial growth was exerted by the protein fraction, but not the small metabolites of this EFN, and disappeared when nectar was heated. In-gel assays demonstrated the activity of acidic and basic chitinases in all EFNs, whereas glucanases were detected only in EFN of myrmecophytes. Our results demonstrate that PR proteins causally underlie the protection of Acacia EFN from microorganisms and that acidic and basic glucanases likely represent the most important prerequisite in this defensive function.
Plants secrete nectar to attract mutualistic animals, which mainly function as pollinators in the case of floral nectar or as defenders against herbivores in the case of extrafloral nectar (EFN; Simpson and Neff, 1981; Heil, 2008; GonzÁlez-Teuber and Heil, 2009a). Because nectars usually represent aqueous solutions of monosaccharides and disaccharides together with amino acids, they are prone to infestation by microbial organisms. When present in the nectar, fungi (GonzÁlez-Teuber et al., 2009) and yeast (Herrera et al., 2009) in particular can alter the chemical composition of the nectar and thereby reduce its suitability for the plant's animal mutualists (Herrera et al., 2008). Moreover, several phytopathogenic organisms may use the nectar-secreting tissues as entries to infect other plant organs (BubÁn et al., 2003; Farkas et al., 2007). Therefore, being an excellent growing medium for yeast, fungi, and bacteria, nectar requires an efficient antimicrobial protection.
Unfortunately, our knowledge of the means by which plants protect nectar from microorganisms is extremely limited. Although the first reports on nectar proteins date back to the 1960s and 1970s (Lüttge, 1961; Baker and Baker, 1975), most studies that considered the defensive function of nectar focused on secondary compounds such as alkaloids and phenols. These metabolites commonly protect nectar from consumption by nectar robbers (animals that feed on nectar without providing a mutualistic service to the plant [Stephenson, 1981; Johnson et al., 2006]) or limit the duration of pollinator visits (Kessler et al., 2008). Only during the last decade did a series of studies discover defensive proteins in the floral nectar of ornamental tobacco (Nicotiana langsdorffii × Nicotiana sanderae; Carter et al., 1999). In this species, floral nectar contains a limited array of proteins termed “nectarins.” Nectarins serve the protection from microbial infestation through a biochemical pathway called the nectar redox cycle (Carter and Thornburg, 2004a), in which mainly three of the five nectarins are involved: NEC1, NEC3, and NEC5. NEC1 was characterized as a manganese superoxide dismutase (Carter and Thornburg, 2000), NEC3 has carbonic anhydrase and monodehydroascorbate reductase activity (Carter and Thornburg, 2004b), and NEC5 is a Glc oxidase that functions together with NEC1 in the production of high peroxide levels (Carter and Thornburg, 2004c): nectar of ornamental tobacco can accumulate up to 4 mm hydrogen peroxide, concentrations that are clearly high enough to exhibit toxicity on microorganisms. Thus, the floral nectar of ornamental tobacco is kept free of microbes mainly via the production of small reactive oxygen species.
By contrast, a proteomic study on EFN of the ant-plant, Acacia cornigera, revealed the presence of several pathogenesis-related (PR) proteins (GonzÁlez-Teuber et al., 2009). Myrmecophytes (ant-plants) are constitutively inhabited by specialized ant species, which serve as a very efficient indirect defense against herbivores (Heil, 2008). In the most specialized cases, both the ant and the plant depend on this interaction, which thus represents an obligate mutualism. In the EFN of A. cornigera, activities of chitinase, β -1,3-glucanase, and peroxidase were detected together with proteins similar to PR-1, osmotin-like proteins, and thaumatin-like proteins (GonzÁlez-Teuber et al., 2009). Most of these proteins, however, were only investigated by tandem mass spectrometry (MS/MS) and characterized via MS-BLAST searches. Because no activity assays had been performed, the presence of these proteins could not be causally linked to the protection of EFN from microorganisms.
This study was conducted to determine whether the antimicrobial protection of Acacia EFN can be directly and exclusively allotted to the enzymatic activity of its protein fraction, which would contrast the protective strategy of this nectar from the one that has been described by Carter, Thornburg, and colleagues (Carter et al., 1999; Carter and Thornburg, 2004a). We also aimed at investigating whether Acacia EFN inhibits the growth of phytopathogens and thus can serve in the protection from infection by pathogens that may use nectaries to enter the plant (BubÁn et al., 2003). We used four sympatric Acacia species and a closely related Prosopis species, which exhibit different types of ant-plant mutualism and therefore differ in their EFN secretion schemes (Heil et al., 2004) and composition (Heil et al., 2005; GonzÁlez-Teuber and Heil, 2009b). The obligate myrmecophytes among Central American Acacia species secrete EFN constitutively at high rates, and the EFN of these species possesses a much higher level of proteins and of antimicrobial defense than the EFN of congeneric nonmyrmecophytes (GonzÁlez-Teuber et al., 2009). The nonmyrmecophytes, by contrast, secrete EFN at lower rates and only transiently in response to leaf damage; this EFN contains few proteins but high levels of Suc (Heil et al., 2005; GonzÁlez-Teuber et al., 2009).
We studied the EFN of the obligate myrmecophytes A. cornigera, Acacia hindsii, and Acacia collinsii and of the two nonmyrmecophytes Acacia farnesiana and Prosopis juliflora. Bioassays were employed to detect inhibitory activities of the nectars against phytopathogens, and in-gel assays were used to determine the presence and functionality of basic and acidic chitinases and glucanases. Size exclusion filtration and heating of the EFN was used to investigate whether the antimicrobial activity of EFN is exclusively caused by the protein fraction. The results demonstrate that the antimicrobial protection of Acacia EFN is caused by the fraction of enzymatically active PR proteins and independent of small, soluble molecules, an observation that represents, to our knowledge, a new strategy by which plants can protect nectar from infestation by potentially deleterious microorganisms.
RESULTS
Inhibitory Effects of EFN on Phytopathogens
Bioassays were performed following the disc diffusion method (Fig. 1) to investigate the biological activity against phytopathogens of EFN, which was collected from wild plants growing in the coastal area of the state of Oaxaca, Mexico. As target organisms, we used six phytopathogenic microorganisms (the oomycete Phytophthora parasitica and the fungi Fusarium oxysporum, Verticillium dahliae, Alternaria alternata, Botrytis cinerea, and Plectosphaerella cucumerina; for details, see Table I). EFN of myrmecophyte species inhibited the growth of at least four microorganisms (Table II). By contrast, no inhibitory effects were observed for nonmyrmecophyte EFN as well as for the sugar solutions, which were used as controls (Table II; Fig. 1). Size exclusion filtration and separately testing the protein fraction (greater than 5 kD) and the fraction of small metabolites revealed that only the protein fraction of EFN of the myrmecophytes inhibited the growth of P. parasitica (Table III). As a second, independent attempt, EFN was collected from the three myrmecophytes, and half of every sample was boiled before being used in bioassays. In response to heating to 100°C for 15 min, the EFNs of all three species lost their activity against the fungi A. alternata, F. oxysporum, and Botrytis capsici (Table IV).
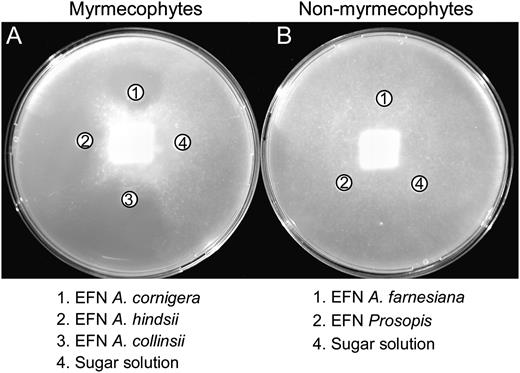
Antimicrobial effects of EFN. Inhibitory effects of EFN are shown from three myrmecophyte species, A. cornigera, A. hindsii, and A. collinsii (A), and from the two nonmyrmecophyte species, A. farnesiana and P. juliflora (B), on growth of the phytopathogen, P. parasitica.
Phytopathogens
Target species used to test the antimicrobial activity of EFN, the collection number or provider of the culture, and Acacia species infected by these pathogens are indicated.
Species | Collection No. or Source of the Culture | Species of Acacia Infected |
Phytophthora parasitica | 201, INRA, Antibes, France | A. mearnsii (Roux and Wingfield, 1997) |
Fusarium oxysporum | ZUM2407, IPO-DLO, Wageningen, The Netherlands | A. nilotica (Kapoor et al., 2004) |
Verticillium dahliae | Consejo Superior de Investigaciones Científicas, Granada, Spain | – |
Alternaria alternata | Consejo Superior de Investigaciones Científicas, Granada, Spain | Acacia trees infected by Alternaria citra (Chimwamurombe et al., 2007) |
Botrytis cinerea | 2100, Spanish Type Culture Collection, Burjassot, Spain | – |
Plectosphaerella cucumerina | University of Neuchâtel, Neuchatel, Switzerland | – |
Species | Collection No. or Source of the Culture | Species of Acacia Infected |
Phytophthora parasitica | 201, INRA, Antibes, France | A. mearnsii (Roux and Wingfield, 1997) |
Fusarium oxysporum | ZUM2407, IPO-DLO, Wageningen, The Netherlands | A. nilotica (Kapoor et al., 2004) |
Verticillium dahliae | Consejo Superior de Investigaciones Científicas, Granada, Spain | – |
Alternaria alternata | Consejo Superior de Investigaciones Científicas, Granada, Spain | Acacia trees infected by Alternaria citra (Chimwamurombe et al., 2007) |
Botrytis cinerea | 2100, Spanish Type Culture Collection, Burjassot, Spain | – |
Plectosphaerella cucumerina | University of Neuchâtel, Neuchatel, Switzerland | – |
Target species used to test the antimicrobial activity of EFN, the collection number or provider of the culture, and Acacia species infected by these pathogens are indicated.
Species | Collection No. or Source of the Culture | Species of Acacia Infected |
Phytophthora parasitica | 201, INRA, Antibes, France | A. mearnsii (Roux and Wingfield, 1997) |
Fusarium oxysporum | ZUM2407, IPO-DLO, Wageningen, The Netherlands | A. nilotica (Kapoor et al., 2004) |
Verticillium dahliae | Consejo Superior de Investigaciones Científicas, Granada, Spain | – |
Alternaria alternata | Consejo Superior de Investigaciones Científicas, Granada, Spain | Acacia trees infected by Alternaria citra (Chimwamurombe et al., 2007) |
Botrytis cinerea | 2100, Spanish Type Culture Collection, Burjassot, Spain | – |
Plectosphaerella cucumerina | University of Neuchâtel, Neuchatel, Switzerland | – |
Species | Collection No. or Source of the Culture | Species of Acacia Infected |
Phytophthora parasitica | 201, INRA, Antibes, France | A. mearnsii (Roux and Wingfield, 1997) |
Fusarium oxysporum | ZUM2407, IPO-DLO, Wageningen, The Netherlands | A. nilotica (Kapoor et al., 2004) |
Verticillium dahliae | Consejo Superior de Investigaciones Científicas, Granada, Spain | – |
Alternaria alternata | Consejo Superior de Investigaciones Científicas, Granada, Spain | Acacia trees infected by Alternaria citra (Chimwamurombe et al., 2007) |
Botrytis cinerea | 2100, Spanish Type Culture Collection, Burjassot, Spain | – |
Plectosphaerella cucumerina | University of Neuchâtel, Neuchatel, Switzerland | – |
Antimicrobial effects of EFN
Inhibitory effects of EFNs from myrmecophyte species (A. cornigera, A. hindsii, and A. collinsii) and from nonmyrmecophyte species (A. farnesiana and P. juliflora) on six species of phytopathogens are represented as the percentage of the line from the center of the microbial colony toward the nectar droplet that was not occupied by the microorganism. Sugar solutions were used as a control. Values are means ± se of three biological replicates.
Treatment | P. parasitica | P. cucumerina | F. oxysporum | B. cinerea | V. dahliae | A. alternata |
A. cornigera | 29 ± 1 | 0 | 25 ± 2 | 0 | 23 ± 2 | 29 ± 2 |
A. hindsii | 23 ± 2 | 0 | 22 ± 2 | 0 | 40 ± 4 | 33 ± 2 |
A. collinsii | 23 ± 2 | 0 | 25 ± 2 | 0 | 22 ± 2 | 22 ± 2 |
A. farnesiana | 0 | 0 | 0 | 0 | 0 | 0 |
P. juliflora | 0 | 0 | 0 | 0 | 0 | 0 |
Sugar 10% | 0 | 0 | 0 | 0 | 0 | 0 |
Sugar 3% | 0 | 0 | 0 | 0 | 0 | 0 |
Treatment | P. parasitica | P. cucumerina | F. oxysporum | B. cinerea | V. dahliae | A. alternata |
A. cornigera | 29 ± 1 | 0 | 25 ± 2 | 0 | 23 ± 2 | 29 ± 2 |
A. hindsii | 23 ± 2 | 0 | 22 ± 2 | 0 | 40 ± 4 | 33 ± 2 |
A. collinsii | 23 ± 2 | 0 | 25 ± 2 | 0 | 22 ± 2 | 22 ± 2 |
A. farnesiana | 0 | 0 | 0 | 0 | 0 | 0 |
P. juliflora | 0 | 0 | 0 | 0 | 0 | 0 |
Sugar 10% | 0 | 0 | 0 | 0 | 0 | 0 |
Sugar 3% | 0 | 0 | 0 | 0 | 0 | 0 |
Inhibitory effects of EFNs from myrmecophyte species (A. cornigera, A. hindsii, and A. collinsii) and from nonmyrmecophyte species (A. farnesiana and P. juliflora) on six species of phytopathogens are represented as the percentage of the line from the center of the microbial colony toward the nectar droplet that was not occupied by the microorganism. Sugar solutions were used as a control. Values are means ± se of three biological replicates.
Treatment | P. parasitica | P. cucumerina | F. oxysporum | B. cinerea | V. dahliae | A. alternata |
A. cornigera | 29 ± 1 | 0 | 25 ± 2 | 0 | 23 ± 2 | 29 ± 2 |
A. hindsii | 23 ± 2 | 0 | 22 ± 2 | 0 | 40 ± 4 | 33 ± 2 |
A. collinsii | 23 ± 2 | 0 | 25 ± 2 | 0 | 22 ± 2 | 22 ± 2 |
A. farnesiana | 0 | 0 | 0 | 0 | 0 | 0 |
P. juliflora | 0 | 0 | 0 | 0 | 0 | 0 |
Sugar 10% | 0 | 0 | 0 | 0 | 0 | 0 |
Sugar 3% | 0 | 0 | 0 | 0 | 0 | 0 |
Treatment | P. parasitica | P. cucumerina | F. oxysporum | B. cinerea | V. dahliae | A. alternata |
A. cornigera | 29 ± 1 | 0 | 25 ± 2 | 0 | 23 ± 2 | 29 ± 2 |
A. hindsii | 23 ± 2 | 0 | 22 ± 2 | 0 | 40 ± 4 | 33 ± 2 |
A. collinsii | 23 ± 2 | 0 | 25 ± 2 | 0 | 22 ± 2 | 22 ± 2 |
A. farnesiana | 0 | 0 | 0 | 0 | 0 | 0 |
P. juliflora | 0 | 0 | 0 | 0 | 0 | 0 |
Sugar 10% | 0 | 0 | 0 | 0 | 0 | 0 |
Sugar 3% | 0 | 0 | 0 | 0 | 0 | 0 |
Antimicrobial activity against P. parasitica
Inhibitory effects of different fractions of the EFN from myrmecophytes (A. cornigera, A. hindsii, and A. collinsii) and from nonmyrmecophytes (A. farnesiana and P. juliflora) on the pathogen P. parasitica are represented as the percentage of the line from the center of the microbial colony toward the nectar droplet that was not occupied by the microorganism. Values are means ± se of three biological replicates.
Treatment | Proteins (> 5-kD Fraction) | Metabolites (< 5-kD Fraction) |
A. cornigera | 28 ± 2 | 0 |
A. hindsii | 22 ± 2 | 0 |
A. collinsii | 23 ± 2 | 0 |
A. farnesiana | 0 | 0 |
P. juliflora | 0 | 0 |
Treatment | Proteins (> 5-kD Fraction) | Metabolites (< 5-kD Fraction) |
A. cornigera | 28 ± 2 | 0 |
A. hindsii | 22 ± 2 | 0 |
A. collinsii | 23 ± 2 | 0 |
A. farnesiana | 0 | 0 |
P. juliflora | 0 | 0 |
Inhibitory effects of different fractions of the EFN from myrmecophytes (A. cornigera, A. hindsii, and A. collinsii) and from nonmyrmecophytes (A. farnesiana and P. juliflora) on the pathogen P. parasitica are represented as the percentage of the line from the center of the microbial colony toward the nectar droplet that was not occupied by the microorganism. Values are means ± se of three biological replicates.
Treatment | Proteins (> 5-kD Fraction) | Metabolites (< 5-kD Fraction) |
A. cornigera | 28 ± 2 | 0 |
A. hindsii | 22 ± 2 | 0 |
A. collinsii | 23 ± 2 | 0 |
A. farnesiana | 0 | 0 |
P. juliflora | 0 | 0 |
Treatment | Proteins (> 5-kD Fraction) | Metabolites (< 5-kD Fraction) |
A. cornigera | 28 ± 2 | 0 |
A. hindsii | 22 ± 2 | 0 |
A. collinsii | 23 ± 2 | 0 |
A. farnesiana | 0 | 0 |
P. juliflora | 0 | 0 |
Disactivation of the antimicrobial effects of EFN by heating
Inhibitory effects of EFNs from myrmecophyte species (A. cornigera, A. hindsii, and A. collinsii) on three fungal species are represented as the percentage of the line from the center of the microbial colony toward the nectar droplet that was not occupied by the microorganism. Values are means ± se of three biological replicates. Fifty percent of each sample was heated to 100°C for 15 min before the assay, and a sugar solution was used as a control.
Treatment | Temperature | F. oxysporum | B. capsici | A. alternata |
% | ||||
A. cornigera | Ambient | 29 ± 3 | 36 ± 2 | 28 ± 2 |
A. hindsii | Ambient | 25 ± 4 | 36 ± 2 | 28 ± 3 |
A. collinsii | Ambient | 23 ± 2 | 26 ± 2 | 24 ± 2 |
Sugar 10% | Ambient | 0 ± 0 | 0 ± 0 | 4 ± 1 |
A. cornigera | 100°C | 0 ± 0 | 2 ± 2 | 3 ± 2 |
A. hindsii | 100°C | 0 ± 0 | 3 ± 1 | 0 ± 0 |
A. collinsii | 100°C | 0 ± 0 | 1 ± 2 | 1 ± 2 |
Sugar 10% | 100°C | 0 ± 0 | 0 ± 0 | 1 ± 1 |
Treatment | Temperature | F. oxysporum | B. capsici | A. alternata |
% | ||||
A. cornigera | Ambient | 29 ± 3 | 36 ± 2 | 28 ± 2 |
A. hindsii | Ambient | 25 ± 4 | 36 ± 2 | 28 ± 3 |
A. collinsii | Ambient | 23 ± 2 | 26 ± 2 | 24 ± 2 |
Sugar 10% | Ambient | 0 ± 0 | 0 ± 0 | 4 ± 1 |
A. cornigera | 100°C | 0 ± 0 | 2 ± 2 | 3 ± 2 |
A. hindsii | 100°C | 0 ± 0 | 3 ± 1 | 0 ± 0 |
A. collinsii | 100°C | 0 ± 0 | 1 ± 2 | 1 ± 2 |
Sugar 10% | 100°C | 0 ± 0 | 0 ± 0 | 1 ± 1 |
Inhibitory effects of EFNs from myrmecophyte species (A. cornigera, A. hindsii, and A. collinsii) on three fungal species are represented as the percentage of the line from the center of the microbial colony toward the nectar droplet that was not occupied by the microorganism. Values are means ± se of three biological replicates. Fifty percent of each sample was heated to 100°C for 15 min before the assay, and a sugar solution was used as a control.
Treatment | Temperature | F. oxysporum | B. capsici | A. alternata |
% | ||||
A. cornigera | Ambient | 29 ± 3 | 36 ± 2 | 28 ± 2 |
A. hindsii | Ambient | 25 ± 4 | 36 ± 2 | 28 ± 3 |
A. collinsii | Ambient | 23 ± 2 | 26 ± 2 | 24 ± 2 |
Sugar 10% | Ambient | 0 ± 0 | 0 ± 0 | 4 ± 1 |
A. cornigera | 100°C | 0 ± 0 | 2 ± 2 | 3 ± 2 |
A. hindsii | 100°C | 0 ± 0 | 3 ± 1 | 0 ± 0 |
A. collinsii | 100°C | 0 ± 0 | 1 ± 2 | 1 ± 2 |
Sugar 10% | 100°C | 0 ± 0 | 0 ± 0 | 1 ± 1 |
Treatment | Temperature | F. oxysporum | B. capsici | A. alternata |
% | ||||
A. cornigera | Ambient | 29 ± 3 | 36 ± 2 | 28 ± 2 |
A. hindsii | Ambient | 25 ± 4 | 36 ± 2 | 28 ± 3 |
A. collinsii | Ambient | 23 ± 2 | 26 ± 2 | 24 ± 2 |
Sugar 10% | Ambient | 0 ± 0 | 0 ± 0 | 4 ± 1 |
A. cornigera | 100°C | 0 ± 0 | 2 ± 2 | 3 ± 2 |
A. hindsii | 100°C | 0 ± 0 | 3 ± 1 | 0 ± 0 |
A. collinsii | 100°C | 0 ± 0 | 1 ± 2 | 1 ± 2 |
Sugar 10% | 100°C | 0 ± 0 | 0 ± 0 | 1 ± 1 |
Protein Patterns and Identification of PR Proteins
One-dimensional SDS-PAGE analysis (Fig. 2) of myrmecophyte EFN revealed approximately nine to 15 clearly distinguishable major bands, which ranged from 10 to 50 kD. By contrast, EFN of the nonmyrmecophytes exhibited very few bands, which ranged from 25 to 50 kD (Fig. 2). Two-dimensional gel electrophoresis followed by matrix-assisted laser desorption/ionization time-of-flight mass spectrometry (MALDI-TOF/MS) and nanoflow liquid chromatography-tandem mass spectrometry (nanoLC-MS/MS) was employed to characterize the proteomes of the EFNs of A. hindsii and A. collinsii and of the two nonmyrmecophytes. The two-dimensional gel analysis revealed over 60 protein spots in the EFNs of the myrmecophytes, whereas between five and seven spots were observed in the EFN of nonmyrmecophytes (Fig. 3). Consistent with the patterns seen on the one-dimensional gels (Fig. 2), the molecular masses of the main proteins ranged in from 10 to 50 kD for both A. hindsii and A. collinsii and were over 25 kD in the two nonmyrmecophytes. NanoLC-MS/MS allowed the annotation of 47 protein spots from the EFNs of the myrmecophytes (Table V), whereas due to the low amount of protein present in each spot, no protein identification was possible in the EFN of A. farnesiana and P. juliflora. De novo peptide sequences were obtained from five spots in EFN of Prosopis, which are presented in Supplemental Table S1. Peptides identifying proteins for both myrmecophyte plants, A. hindsii and A. collinsii, are compiled in Supplemental Table S2.
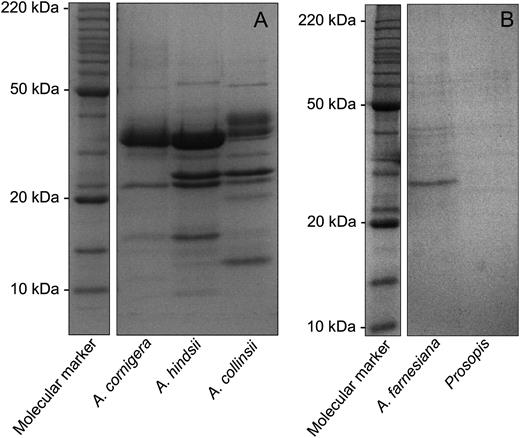
Protein patterns in EFN. SDS-PAGE (13%) profile of EFN proteins from the myrmecophyte species A. cornigera, A. hindsii, and A. collinsii (A) and the nonmyrmecophyte species A. farnesiana and P. juliflora (B). Molecular mass markers are indicated.
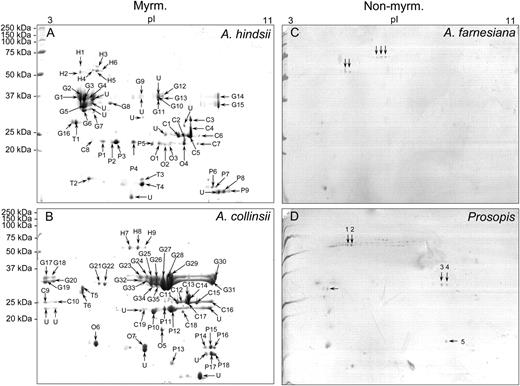
Two-dimensional gel electrophoresis. Separation of proteins in EFN from A. hindsii (A), A. collinsii (B), A. farnesiana (C), and P. juliflora (D) by two-dimensional gel electrophoresis (10% SDS-PAGE). C, Chitinase proteins; G, glucanase proteins; H, glycoside hydrolase proteins; Myrm., myrmecophytes; Non-myrm., nonmyrmecophytes; O, osmotin proteins; P, PR proteins; T, thaumatin-like proteins; U, unknown proteins.
Protein identification in EFN
Results of MS-BLAST searches using de novo peptide sequences are shown for the species A. hindsii and A. collinsii.
Spot | Acacia Species | Accession No. | Description | Plant Species | Peptide Hits MS-BLAST | MS-BLAST Score |
H1 | A. hindsii | ABP03050 | Glycoside hydrolase | Medicago trunculata | 4 | 193 |
H2 | A. hindsii | ABP03050 | Glycoside hydrolase | Medicago trunculata | 6 | 280 |
H3 | A. hindsii | ABP03050 | Glycoside hydrolase | Medicago trunculata | 5 | 275 |
H4 | A. hindsii | ABP03050 | Glycoside hydrolase | Medicago trunculata | 4 | 206 |
H5 | A. hindsii | Q8RU51 | Glucan 1,3- β -glucosidase | Oryza sativa | 12 | 535 |
H6 | A. hindsii | Q8RU51 | Glucan 1,3- β -glucosidase | Oryza sativa | 15 | 714 |
H7 | A. collinsii | Q8RU51 | Glucan 1,3- β -glucosidase | Oryza sativa | 15 | 803 |
H8 | A. collinsii | Q8RU51 | Glucan 1,3- β -glucosidase | Oryza sativa | 20 | 940 |
H9 | A. collinsii | Q8RU51 | Glucan 1,3- β -glucosidase | Oryza sativa | 11 | 532 |
G1 | A. hindsii | BAC15778 | Endo-1,3- β -glucanase | Oryza sativa | 3 | 191 |
G2 | A. hindsii | AAR26001 | Endo-1,3- β -glucanase | Glycine max | 4 | 222 |
G3 | A. hindsii | AAR26001 | Endo-1,3- β -glucanase | Glycine max | 6 | 326 |
G4 | A. hindsii | AAD10380 | β -1,3-Glucanase precursor | Oryza sativa | 4 | 151 |
G5 | A. hindsii | Q9CA15 | Endo-1,3- β -glucanase | Arabidopsis thaliana | 2 | 102 |
G6 | A. hindsii | Q9ZP12 | Glucan endo-1,3- β -d-glucosidase | Cicer arietinum | 2 | 160 |
G7 | A. hindsii | AAK97661 | β -1,3-Glucanase | Sorghum bicolor | 1 | 64 |
G8 | A. hindsii | BAC15778 | Endo-1,3- β -glucanase | Oryza sativa | 2 | 118 |
G9 | A. hindsii | BAC84500 | β -1,3-Glucanase | Oryza sativa | 1 | 81 |
G10 | A. hindsii | Q6S4I9 | Endo- β -1,3-glucanase | Glycine tabacina | 3 | 136 |
G11 | A. hindsii | Q6S9W0 | Endo-1,3- β -glucanase | Glycine max | 5 | 280 |
G12 | A. hindsii | BAE53384 | β -1,3-Glucanase | Sesbania rostrata | 1 | 89 |
G13 | A. hindsii | BAE53384 | β -1,3-Glucanase | Sesbania rostrata | 2 | 104 |
G14 | A. hindsii | BAE53384 | β -1,3-Glucanase | Sesbania rostrata | 3 | 192 |
G15 | A. hindsii | BAC15778 | Endo-1,3- β -glucanase | Oryza sativa | 4 | 202 |
G16 | A. hindsii | BAE53384 | β -1,3-Glucanase | Sesbania rostrata | 2 | 154 |
G17 | A. collinsii | Q84Y06 | β -1,3-Glucanase | Fragaria ananassa | 2 | 143 |
G18 | A. collinsii | Q6S4I9 | Endo- β -1,3-glucanase | Glycine tabacina | 3 | 176 |
G19 | A. collinsii | O49016 | β -1,3-Glucanase | Glycine max | 1 | 77 |
G20 | A. collinsii | B2NK62 | β -1,3-Glucanase | Lotus japonicus | 4 | 258 |
G21 | A. collinsii | O49012 | β -1,3-Glucanase | Glycine max | 6 | 307 |
G22 | A. collinsii | P33157 | Glucan endo-1,3- β -glucosidase | Arabidopsis thaliana | 4 | 204 |
G23 | A. collinsii | Q84Y07 | β -1,3-Glucanase | Fragaria ananassa | 3 | 156 |
G24 | A. collinsii | Q6S4J7 | Endo- β -1,3-glucanase | Glycine tabacina | 4 | 251 |
G25 | A. collinsii | Q84Y07 | β -1,3-Glucanase | Fragaria ananassa | 1 | 74 |
G26 | A. collinsii | Q6GWG6 | β -1,3-Endoglucanase | Glycine soja | 4 | 230 |
G27 | A. collinsii | Q56AP1 | β -1,3-Glucanase | Fragaria ananassa | 6 | 312 |
G28 | A. collinsii | Q84Y07 | β -1,3-Glucanase | Fragaria ananassa | 7 | 357 |
G29 | A. collinsii | Q6S4I9 | Endo- β -1,3-glucanase | Glycine tabacina | 4 | 250 |
G30 | A. collinsii | B2NK62 | β -1,3-Glucanase | Lotus japonicus | 3 | 214 |
G31 | A. collinsii | Q84Y07 | β -1,3-Glucanase | Fragaria ananassa | 5 | 260 |
G32 | A. collinsii | O49016 | β -1,3-Glucanase | Glycine max | 3 | 149 |
G33 | A. collinsii | O49016 | β -1,3-Glucanase | Glycine max | 3 | 146 |
G34 | A. collinsii | O49016 | β -1,3-Glucanase | Glycine max | 3 | 182 |
G35 | A. collinsii | Q6S4J4 | Endo- β -1,3-glucanase | Glycine latrobeana | 2 | 84 |
C1 | A. hindsii | Q43685 | Chitinase class I | Vigna unguiculata | 5 | 225 |
C2 | A. hindsii | Q8MD06 | Chitinase | Leucaena leucocephala | 6 | 317 |
C3 | A. hindsii | AAM49597 | Chitinase | Leucaena leucocephala | 6 | 292 |
C4 | A. hindsii | Q7X9R8 | Chitinase | Euonymus europaeus | 16 | 884 |
C5 | A. hindsii | ABD66068 | Chitinase | Momordica charantia | 9 | 561 |
C6 | A. hindsii | ABD66068 | Chitinase | Momordica charantia | 4 | 242 |
C7 | A. hindsii | AAG37276 | Chitinase | Fragaria ananassa | 4 | 237 |
C8 | A. hindsii | AAB41324 | Class I chitinase | Medicago sativa | 4 | 253 |
C9 | A. collinsii | Q42428 | Chitinase | Castanea sativa | 8 | 507 |
C10 | A. collinsii | Q7X9R8 | Chitinase | Euonymus europaeus | 7 | 488 |
C11 | A. collinsii | Q8MD06 | Chitinase | Leucaena leucocephala | 7 | 410 |
C12 | A. collinsii | Q42428 | Chitinase | Castanea sativa | 8 | 559 |
C13 | A. collinsii | Q7X9R8 | Chitinase | Euonymus europaeus | 9 | 564 |
C14 | A. collinsii | Q9FEW1 | Endochitinase | Nicotiana sylvestris | 13 | 683 |
C15 | A. collinsii | Q207U1 | Chitinase | Momordica charantia | 13 | 798 |
C16 | A. collinsii | Q42428 | Chitinase | Castanea sativa | 11 | 714 |
C17 | A. collinsii | Q42428 | Chitinase | Castanea sativa | 9 | 623 |
C18 | A. collinsii | Q2VAC7 | Chitinase | Ficus pumila | 2 | 111 |
C19 | A. collinsii | Q93WX9 | Endochitinase | Musa acuminata | 2 | 126 |
O1 | A. hindsii | ABC55724 | Osmotin-like protein | Fragaria ananassa | 5 | 310 |
O2 | A. hindsii | AAU95243 | Osmotin-like protein | Solanum tuberosum | 4 | 248 |
O3 | A. hindsii | AAF13707 | Osmotin-like protein | Fragaria ananassa | 4 | 232 |
O4 | A. hindsii | AAU95238 | Osmotin-like protein | Solanum phureja | 3 | 194 |
O5 | A. collinsii | A9QVJ4 | Osmotin | Piper colubrinum | 7 | 446 |
O6 | A. collinsii | Q8S4L2 | Osmotin-like protein | Solanum nigrum | 5 | 254 |
O7 | A. collinsii | Q84MK8 | Osmotin | Solanum tuberosum | 2 | 104 |
T1 | A. hindsii | AAM00216 | Thaumatin-like protein | Prunus persica | 3 | 167 |
T2 | A. hindsii | CAA48278 | Thaumatin-like protein | Oryza sativa | 1 | 95 |
T3 | A. hindsii | CAA09229 | Thaumatin-like protein | Cicer arietinum | 3 | 142 |
T4 | A. hindsii | Q93XD2 | Thaumatin-like protein | Sambucus nigra | 5 | 277 |
T5 | A. collinsii | Q2VC78 | Thaumatin-like protein | Glycine max | 2 | 125 |
T6 | A. collinsii | P83332 | Thaumatin-like protein 1 | Prunus persica | 2 | 133 |
P1 | A. hindsii | AAO22065 | NtPRp27-like protein | Solanum tuberosum | 4 | 234 |
P2 | A. hindsii | AAO22065 | NtPRp27-like protein | Solanum tuberosum | 4 | 278 |
P3 | A. hindsii | AAO22065 | NtPRp27-like protein | Solanum tuberosum | 8 | 451 |
P4 | A. hindsii | BAA81904 | NtPRp27 | Nicotiana tabacum | 6 | 338 |
P5 | A. hindsii | BAA81904 | NtPRp27 | Nicotiana tabacum | 5 | 325 |
P6 | A. hindsii | AAK30143 | Pathogenesis-related protein PR-1 | Capsicum annuum | 2 | 160 |
P7 | A. hindsii | AAK30143 | Pathogenesis-related protein PR-1 | Capsicum annuum | 2 | 172 |
P8 | A. hindsii | CAA87071 | Pathogenesis-related protein PR-1 type | Sambucus nigra | 1 | 98 |
P9 | A. hindsii | CAA52894 | PR-1b pathogenesis- related protein | Hordeum vulgare | 3 | 186 |
P10 | A. collinsii | Q84XQ4 | NtPRp27-like protein | Solanum tuberosum | 6 | 327 |
P11 | A. collinsii | Q84XQ4 | NtPRp27-like protein | Solanum tuberosum | 3 | 160 |
P12 | A. collinsii | Q84XQ4 | NtPRp27-like protein | Solanum tuberosum | 6 | 317 |
P13 | A. collinsii | Q84XQ4 | NtPRp27-like protein | Solanum tuberosum | 6 | 338 |
P14 | A. collinsii | Q84XQ4 | NtPRp27-like protein | Solanum tuberosum | 1 | 69 |
P15 | A. collinsii | Q41359 | Pathogenesis-related protein PR-1 type | Sambucus nigra | 3 | 181 |
P16 | A. collinsii | Q41359 | Pathogenesis-related protein PR-1 type | Sambucus nigra | 2 | 124 |
P17 | A. collinsii | Q2XX51 | Pathogenesis-related protein 1 | Zea diploperennis | 5 | 275 |
P18 | A. collinsii | A0MZ69 | Pathogenesis-related protein 1 | Musa acuminata | 6 | 301 |
Spot | Acacia Species | Accession No. | Description | Plant Species | Peptide Hits MS-BLAST | MS-BLAST Score |
H1 | A. hindsii | ABP03050 | Glycoside hydrolase | Medicago trunculata | 4 | 193 |
H2 | A. hindsii | ABP03050 | Glycoside hydrolase | Medicago trunculata | 6 | 280 |
H3 | A. hindsii | ABP03050 | Glycoside hydrolase | Medicago trunculata | 5 | 275 |
H4 | A. hindsii | ABP03050 | Glycoside hydrolase | Medicago trunculata | 4 | 206 |
H5 | A. hindsii | Q8RU51 | Glucan 1,3- β -glucosidase | Oryza sativa | 12 | 535 |
H6 | A. hindsii | Q8RU51 | Glucan 1,3- β -glucosidase | Oryza sativa | 15 | 714 |
H7 | A. collinsii | Q8RU51 | Glucan 1,3- β -glucosidase | Oryza sativa | 15 | 803 |
H8 | A. collinsii | Q8RU51 | Glucan 1,3- β -glucosidase | Oryza sativa | 20 | 940 |
H9 | A. collinsii | Q8RU51 | Glucan 1,3- β -glucosidase | Oryza sativa | 11 | 532 |
G1 | A. hindsii | BAC15778 | Endo-1,3- β -glucanase | Oryza sativa | 3 | 191 |
G2 | A. hindsii | AAR26001 | Endo-1,3- β -glucanase | Glycine max | 4 | 222 |
G3 | A. hindsii | AAR26001 | Endo-1,3- β -glucanase | Glycine max | 6 | 326 |
G4 | A. hindsii | AAD10380 | β -1,3-Glucanase precursor | Oryza sativa | 4 | 151 |
G5 | A. hindsii | Q9CA15 | Endo-1,3- β -glucanase | Arabidopsis thaliana | 2 | 102 |
G6 | A. hindsii | Q9ZP12 | Glucan endo-1,3- β -d-glucosidase | Cicer arietinum | 2 | 160 |
G7 | A. hindsii | AAK97661 | β -1,3-Glucanase | Sorghum bicolor | 1 | 64 |
G8 | A. hindsii | BAC15778 | Endo-1,3- β -glucanase | Oryza sativa | 2 | 118 |
G9 | A. hindsii | BAC84500 | β -1,3-Glucanase | Oryza sativa | 1 | 81 |
G10 | A. hindsii | Q6S4I9 | Endo- β -1,3-glucanase | Glycine tabacina | 3 | 136 |
G11 | A. hindsii | Q6S9W0 | Endo-1,3- β -glucanase | Glycine max | 5 | 280 |
G12 | A. hindsii | BAE53384 | β -1,3-Glucanase | Sesbania rostrata | 1 | 89 |
G13 | A. hindsii | BAE53384 | β -1,3-Glucanase | Sesbania rostrata | 2 | 104 |
G14 | A. hindsii | BAE53384 | β -1,3-Glucanase | Sesbania rostrata | 3 | 192 |
G15 | A. hindsii | BAC15778 | Endo-1,3- β -glucanase | Oryza sativa | 4 | 202 |
G16 | A. hindsii | BAE53384 | β -1,3-Glucanase | Sesbania rostrata | 2 | 154 |
G17 | A. collinsii | Q84Y06 | β -1,3-Glucanase | Fragaria ananassa | 2 | 143 |
G18 | A. collinsii | Q6S4I9 | Endo- β -1,3-glucanase | Glycine tabacina | 3 | 176 |
G19 | A. collinsii | O49016 | β -1,3-Glucanase | Glycine max | 1 | 77 |
G20 | A. collinsii | B2NK62 | β -1,3-Glucanase | Lotus japonicus | 4 | 258 |
G21 | A. collinsii | O49012 | β -1,3-Glucanase | Glycine max | 6 | 307 |
G22 | A. collinsii | P33157 | Glucan endo-1,3- β -glucosidase | Arabidopsis thaliana | 4 | 204 |
G23 | A. collinsii | Q84Y07 | β -1,3-Glucanase | Fragaria ananassa | 3 | 156 |
G24 | A. collinsii | Q6S4J7 | Endo- β -1,3-glucanase | Glycine tabacina | 4 | 251 |
G25 | A. collinsii | Q84Y07 | β -1,3-Glucanase | Fragaria ananassa | 1 | 74 |
G26 | A. collinsii | Q6GWG6 | β -1,3-Endoglucanase | Glycine soja | 4 | 230 |
G27 | A. collinsii | Q56AP1 | β -1,3-Glucanase | Fragaria ananassa | 6 | 312 |
G28 | A. collinsii | Q84Y07 | β -1,3-Glucanase | Fragaria ananassa | 7 | 357 |
G29 | A. collinsii | Q6S4I9 | Endo- β -1,3-glucanase | Glycine tabacina | 4 | 250 |
G30 | A. collinsii | B2NK62 | β -1,3-Glucanase | Lotus japonicus | 3 | 214 |
G31 | A. collinsii | Q84Y07 | β -1,3-Glucanase | Fragaria ananassa | 5 | 260 |
G32 | A. collinsii | O49016 | β -1,3-Glucanase | Glycine max | 3 | 149 |
G33 | A. collinsii | O49016 | β -1,3-Glucanase | Glycine max | 3 | 146 |
G34 | A. collinsii | O49016 | β -1,3-Glucanase | Glycine max | 3 | 182 |
G35 | A. collinsii | Q6S4J4 | Endo- β -1,3-glucanase | Glycine latrobeana | 2 | 84 |
C1 | A. hindsii | Q43685 | Chitinase class I | Vigna unguiculata | 5 | 225 |
C2 | A. hindsii | Q8MD06 | Chitinase | Leucaena leucocephala | 6 | 317 |
C3 | A. hindsii | AAM49597 | Chitinase | Leucaena leucocephala | 6 | 292 |
C4 | A. hindsii | Q7X9R8 | Chitinase | Euonymus europaeus | 16 | 884 |
C5 | A. hindsii | ABD66068 | Chitinase | Momordica charantia | 9 | 561 |
C6 | A. hindsii | ABD66068 | Chitinase | Momordica charantia | 4 | 242 |
C7 | A. hindsii | AAG37276 | Chitinase | Fragaria ananassa | 4 | 237 |
C8 | A. hindsii | AAB41324 | Class I chitinase | Medicago sativa | 4 | 253 |
C9 | A. collinsii | Q42428 | Chitinase | Castanea sativa | 8 | 507 |
C10 | A. collinsii | Q7X9R8 | Chitinase | Euonymus europaeus | 7 | 488 |
C11 | A. collinsii | Q8MD06 | Chitinase | Leucaena leucocephala | 7 | 410 |
C12 | A. collinsii | Q42428 | Chitinase | Castanea sativa | 8 | 559 |
C13 | A. collinsii | Q7X9R8 | Chitinase | Euonymus europaeus | 9 | 564 |
C14 | A. collinsii | Q9FEW1 | Endochitinase | Nicotiana sylvestris | 13 | 683 |
C15 | A. collinsii | Q207U1 | Chitinase | Momordica charantia | 13 | 798 |
C16 | A. collinsii | Q42428 | Chitinase | Castanea sativa | 11 | 714 |
C17 | A. collinsii | Q42428 | Chitinase | Castanea sativa | 9 | 623 |
C18 | A. collinsii | Q2VAC7 | Chitinase | Ficus pumila | 2 | 111 |
C19 | A. collinsii | Q93WX9 | Endochitinase | Musa acuminata | 2 | 126 |
O1 | A. hindsii | ABC55724 | Osmotin-like protein | Fragaria ananassa | 5 | 310 |
O2 | A. hindsii | AAU95243 | Osmotin-like protein | Solanum tuberosum | 4 | 248 |
O3 | A. hindsii | AAF13707 | Osmotin-like protein | Fragaria ananassa | 4 | 232 |
O4 | A. hindsii | AAU95238 | Osmotin-like protein | Solanum phureja | 3 | 194 |
O5 | A. collinsii | A9QVJ4 | Osmotin | Piper colubrinum | 7 | 446 |
O6 | A. collinsii | Q8S4L2 | Osmotin-like protein | Solanum nigrum | 5 | 254 |
O7 | A. collinsii | Q84MK8 | Osmotin | Solanum tuberosum | 2 | 104 |
T1 | A. hindsii | AAM00216 | Thaumatin-like protein | Prunus persica | 3 | 167 |
T2 | A. hindsii | CAA48278 | Thaumatin-like protein | Oryza sativa | 1 | 95 |
T3 | A. hindsii | CAA09229 | Thaumatin-like protein | Cicer arietinum | 3 | 142 |
T4 | A. hindsii | Q93XD2 | Thaumatin-like protein | Sambucus nigra | 5 | 277 |
T5 | A. collinsii | Q2VC78 | Thaumatin-like protein | Glycine max | 2 | 125 |
T6 | A. collinsii | P83332 | Thaumatin-like protein 1 | Prunus persica | 2 | 133 |
P1 | A. hindsii | AAO22065 | NtPRp27-like protein | Solanum tuberosum | 4 | 234 |
P2 | A. hindsii | AAO22065 | NtPRp27-like protein | Solanum tuberosum | 4 | 278 |
P3 | A. hindsii | AAO22065 | NtPRp27-like protein | Solanum tuberosum | 8 | 451 |
P4 | A. hindsii | BAA81904 | NtPRp27 | Nicotiana tabacum | 6 | 338 |
P5 | A. hindsii | BAA81904 | NtPRp27 | Nicotiana tabacum | 5 | 325 |
P6 | A. hindsii | AAK30143 | Pathogenesis-related protein PR-1 | Capsicum annuum | 2 | 160 |
P7 | A. hindsii | AAK30143 | Pathogenesis-related protein PR-1 | Capsicum annuum | 2 | 172 |
P8 | A. hindsii | CAA87071 | Pathogenesis-related protein PR-1 type | Sambucus nigra | 1 | 98 |
P9 | A. hindsii | CAA52894 | PR-1b pathogenesis- related protein | Hordeum vulgare | 3 | 186 |
P10 | A. collinsii | Q84XQ4 | NtPRp27-like protein | Solanum tuberosum | 6 | 327 |
P11 | A. collinsii | Q84XQ4 | NtPRp27-like protein | Solanum tuberosum | 3 | 160 |
P12 | A. collinsii | Q84XQ4 | NtPRp27-like protein | Solanum tuberosum | 6 | 317 |
P13 | A. collinsii | Q84XQ4 | NtPRp27-like protein | Solanum tuberosum | 6 | 338 |
P14 | A. collinsii | Q84XQ4 | NtPRp27-like protein | Solanum tuberosum | 1 | 69 |
P15 | A. collinsii | Q41359 | Pathogenesis-related protein PR-1 type | Sambucus nigra | 3 | 181 |
P16 | A. collinsii | Q41359 | Pathogenesis-related protein PR-1 type | Sambucus nigra | 2 | 124 |
P17 | A. collinsii | Q2XX51 | Pathogenesis-related protein 1 | Zea diploperennis | 5 | 275 |
P18 | A. collinsii | A0MZ69 | Pathogenesis-related protein 1 | Musa acuminata | 6 | 301 |
Results of MS-BLAST searches using de novo peptide sequences are shown for the species A. hindsii and A. collinsii.
Spot | Acacia Species | Accession No. | Description | Plant Species | Peptide Hits MS-BLAST | MS-BLAST Score |
H1 | A. hindsii | ABP03050 | Glycoside hydrolase | Medicago trunculata | 4 | 193 |
H2 | A. hindsii | ABP03050 | Glycoside hydrolase | Medicago trunculata | 6 | 280 |
H3 | A. hindsii | ABP03050 | Glycoside hydrolase | Medicago trunculata | 5 | 275 |
H4 | A. hindsii | ABP03050 | Glycoside hydrolase | Medicago trunculata | 4 | 206 |
H5 | A. hindsii | Q8RU51 | Glucan 1,3- β -glucosidase | Oryza sativa | 12 | 535 |
H6 | A. hindsii | Q8RU51 | Glucan 1,3- β -glucosidase | Oryza sativa | 15 | 714 |
H7 | A. collinsii | Q8RU51 | Glucan 1,3- β -glucosidase | Oryza sativa | 15 | 803 |
H8 | A. collinsii | Q8RU51 | Glucan 1,3- β -glucosidase | Oryza sativa | 20 | 940 |
H9 | A. collinsii | Q8RU51 | Glucan 1,3- β -glucosidase | Oryza sativa | 11 | 532 |
G1 | A. hindsii | BAC15778 | Endo-1,3- β -glucanase | Oryza sativa | 3 | 191 |
G2 | A. hindsii | AAR26001 | Endo-1,3- β -glucanase | Glycine max | 4 | 222 |
G3 | A. hindsii | AAR26001 | Endo-1,3- β -glucanase | Glycine max | 6 | 326 |
G4 | A. hindsii | AAD10380 | β -1,3-Glucanase precursor | Oryza sativa | 4 | 151 |
G5 | A. hindsii | Q9CA15 | Endo-1,3- β -glucanase | Arabidopsis thaliana | 2 | 102 |
G6 | A. hindsii | Q9ZP12 | Glucan endo-1,3- β -d-glucosidase | Cicer arietinum | 2 | 160 |
G7 | A. hindsii | AAK97661 | β -1,3-Glucanase | Sorghum bicolor | 1 | 64 |
G8 | A. hindsii | BAC15778 | Endo-1,3- β -glucanase | Oryza sativa | 2 | 118 |
G9 | A. hindsii | BAC84500 | β -1,3-Glucanase | Oryza sativa | 1 | 81 |
G10 | A. hindsii | Q6S4I9 | Endo- β -1,3-glucanase | Glycine tabacina | 3 | 136 |
G11 | A. hindsii | Q6S9W0 | Endo-1,3- β -glucanase | Glycine max | 5 | 280 |
G12 | A. hindsii | BAE53384 | β -1,3-Glucanase | Sesbania rostrata | 1 | 89 |
G13 | A. hindsii | BAE53384 | β -1,3-Glucanase | Sesbania rostrata | 2 | 104 |
G14 | A. hindsii | BAE53384 | β -1,3-Glucanase | Sesbania rostrata | 3 | 192 |
G15 | A. hindsii | BAC15778 | Endo-1,3- β -glucanase | Oryza sativa | 4 | 202 |
G16 | A. hindsii | BAE53384 | β -1,3-Glucanase | Sesbania rostrata | 2 | 154 |
G17 | A. collinsii | Q84Y06 | β -1,3-Glucanase | Fragaria ananassa | 2 | 143 |
G18 | A. collinsii | Q6S4I9 | Endo- β -1,3-glucanase | Glycine tabacina | 3 | 176 |
G19 | A. collinsii | O49016 | β -1,3-Glucanase | Glycine max | 1 | 77 |
G20 | A. collinsii | B2NK62 | β -1,3-Glucanase | Lotus japonicus | 4 | 258 |
G21 | A. collinsii | O49012 | β -1,3-Glucanase | Glycine max | 6 | 307 |
G22 | A. collinsii | P33157 | Glucan endo-1,3- β -glucosidase | Arabidopsis thaliana | 4 | 204 |
G23 | A. collinsii | Q84Y07 | β -1,3-Glucanase | Fragaria ananassa | 3 | 156 |
G24 | A. collinsii | Q6S4J7 | Endo- β -1,3-glucanase | Glycine tabacina | 4 | 251 |
G25 | A. collinsii | Q84Y07 | β -1,3-Glucanase | Fragaria ananassa | 1 | 74 |
G26 | A. collinsii | Q6GWG6 | β -1,3-Endoglucanase | Glycine soja | 4 | 230 |
G27 | A. collinsii | Q56AP1 | β -1,3-Glucanase | Fragaria ananassa | 6 | 312 |
G28 | A. collinsii | Q84Y07 | β -1,3-Glucanase | Fragaria ananassa | 7 | 357 |
G29 | A. collinsii | Q6S4I9 | Endo- β -1,3-glucanase | Glycine tabacina | 4 | 250 |
G30 | A. collinsii | B2NK62 | β -1,3-Glucanase | Lotus japonicus | 3 | 214 |
G31 | A. collinsii | Q84Y07 | β -1,3-Glucanase | Fragaria ananassa | 5 | 260 |
G32 | A. collinsii | O49016 | β -1,3-Glucanase | Glycine max | 3 | 149 |
G33 | A. collinsii | O49016 | β -1,3-Glucanase | Glycine max | 3 | 146 |
G34 | A. collinsii | O49016 | β -1,3-Glucanase | Glycine max | 3 | 182 |
G35 | A. collinsii | Q6S4J4 | Endo- β -1,3-glucanase | Glycine latrobeana | 2 | 84 |
C1 | A. hindsii | Q43685 | Chitinase class I | Vigna unguiculata | 5 | 225 |
C2 | A. hindsii | Q8MD06 | Chitinase | Leucaena leucocephala | 6 | 317 |
C3 | A. hindsii | AAM49597 | Chitinase | Leucaena leucocephala | 6 | 292 |
C4 | A. hindsii | Q7X9R8 | Chitinase | Euonymus europaeus | 16 | 884 |
C5 | A. hindsii | ABD66068 | Chitinase | Momordica charantia | 9 | 561 |
C6 | A. hindsii | ABD66068 | Chitinase | Momordica charantia | 4 | 242 |
C7 | A. hindsii | AAG37276 | Chitinase | Fragaria ananassa | 4 | 237 |
C8 | A. hindsii | AAB41324 | Class I chitinase | Medicago sativa | 4 | 253 |
C9 | A. collinsii | Q42428 | Chitinase | Castanea sativa | 8 | 507 |
C10 | A. collinsii | Q7X9R8 | Chitinase | Euonymus europaeus | 7 | 488 |
C11 | A. collinsii | Q8MD06 | Chitinase | Leucaena leucocephala | 7 | 410 |
C12 | A. collinsii | Q42428 | Chitinase | Castanea sativa | 8 | 559 |
C13 | A. collinsii | Q7X9R8 | Chitinase | Euonymus europaeus | 9 | 564 |
C14 | A. collinsii | Q9FEW1 | Endochitinase | Nicotiana sylvestris | 13 | 683 |
C15 | A. collinsii | Q207U1 | Chitinase | Momordica charantia | 13 | 798 |
C16 | A. collinsii | Q42428 | Chitinase | Castanea sativa | 11 | 714 |
C17 | A. collinsii | Q42428 | Chitinase | Castanea sativa | 9 | 623 |
C18 | A. collinsii | Q2VAC7 | Chitinase | Ficus pumila | 2 | 111 |
C19 | A. collinsii | Q93WX9 | Endochitinase | Musa acuminata | 2 | 126 |
O1 | A. hindsii | ABC55724 | Osmotin-like protein | Fragaria ananassa | 5 | 310 |
O2 | A. hindsii | AAU95243 | Osmotin-like protein | Solanum tuberosum | 4 | 248 |
O3 | A. hindsii | AAF13707 | Osmotin-like protein | Fragaria ananassa | 4 | 232 |
O4 | A. hindsii | AAU95238 | Osmotin-like protein | Solanum phureja | 3 | 194 |
O5 | A. collinsii | A9QVJ4 | Osmotin | Piper colubrinum | 7 | 446 |
O6 | A. collinsii | Q8S4L2 | Osmotin-like protein | Solanum nigrum | 5 | 254 |
O7 | A. collinsii | Q84MK8 | Osmotin | Solanum tuberosum | 2 | 104 |
T1 | A. hindsii | AAM00216 | Thaumatin-like protein | Prunus persica | 3 | 167 |
T2 | A. hindsii | CAA48278 | Thaumatin-like protein | Oryza sativa | 1 | 95 |
T3 | A. hindsii | CAA09229 | Thaumatin-like protein | Cicer arietinum | 3 | 142 |
T4 | A. hindsii | Q93XD2 | Thaumatin-like protein | Sambucus nigra | 5 | 277 |
T5 | A. collinsii | Q2VC78 | Thaumatin-like protein | Glycine max | 2 | 125 |
T6 | A. collinsii | P83332 | Thaumatin-like protein 1 | Prunus persica | 2 | 133 |
P1 | A. hindsii | AAO22065 | NtPRp27-like protein | Solanum tuberosum | 4 | 234 |
P2 | A. hindsii | AAO22065 | NtPRp27-like protein | Solanum tuberosum | 4 | 278 |
P3 | A. hindsii | AAO22065 | NtPRp27-like protein | Solanum tuberosum | 8 | 451 |
P4 | A. hindsii | BAA81904 | NtPRp27 | Nicotiana tabacum | 6 | 338 |
P5 | A. hindsii | BAA81904 | NtPRp27 | Nicotiana tabacum | 5 | 325 |
P6 | A. hindsii | AAK30143 | Pathogenesis-related protein PR-1 | Capsicum annuum | 2 | 160 |
P7 | A. hindsii | AAK30143 | Pathogenesis-related protein PR-1 | Capsicum annuum | 2 | 172 |
P8 | A. hindsii | CAA87071 | Pathogenesis-related protein PR-1 type | Sambucus nigra | 1 | 98 |
P9 | A. hindsii | CAA52894 | PR-1b pathogenesis- related protein | Hordeum vulgare | 3 | 186 |
P10 | A. collinsii | Q84XQ4 | NtPRp27-like protein | Solanum tuberosum | 6 | 327 |
P11 | A. collinsii | Q84XQ4 | NtPRp27-like protein | Solanum tuberosum | 3 | 160 |
P12 | A. collinsii | Q84XQ4 | NtPRp27-like protein | Solanum tuberosum | 6 | 317 |
P13 | A. collinsii | Q84XQ4 | NtPRp27-like protein | Solanum tuberosum | 6 | 338 |
P14 | A. collinsii | Q84XQ4 | NtPRp27-like protein | Solanum tuberosum | 1 | 69 |
P15 | A. collinsii | Q41359 | Pathogenesis-related protein PR-1 type | Sambucus nigra | 3 | 181 |
P16 | A. collinsii | Q41359 | Pathogenesis-related protein PR-1 type | Sambucus nigra | 2 | 124 |
P17 | A. collinsii | Q2XX51 | Pathogenesis-related protein 1 | Zea diploperennis | 5 | 275 |
P18 | A. collinsii | A0MZ69 | Pathogenesis-related protein 1 | Musa acuminata | 6 | 301 |
Spot | Acacia Species | Accession No. | Description | Plant Species | Peptide Hits MS-BLAST | MS-BLAST Score |
H1 | A. hindsii | ABP03050 | Glycoside hydrolase | Medicago trunculata | 4 | 193 |
H2 | A. hindsii | ABP03050 | Glycoside hydrolase | Medicago trunculata | 6 | 280 |
H3 | A. hindsii | ABP03050 | Glycoside hydrolase | Medicago trunculata | 5 | 275 |
H4 | A. hindsii | ABP03050 | Glycoside hydrolase | Medicago trunculata | 4 | 206 |
H5 | A. hindsii | Q8RU51 | Glucan 1,3- β -glucosidase | Oryza sativa | 12 | 535 |
H6 | A. hindsii | Q8RU51 | Glucan 1,3- β -glucosidase | Oryza sativa | 15 | 714 |
H7 | A. collinsii | Q8RU51 | Glucan 1,3- β -glucosidase | Oryza sativa | 15 | 803 |
H8 | A. collinsii | Q8RU51 | Glucan 1,3- β -glucosidase | Oryza sativa | 20 | 940 |
H9 | A. collinsii | Q8RU51 | Glucan 1,3- β -glucosidase | Oryza sativa | 11 | 532 |
G1 | A. hindsii | BAC15778 | Endo-1,3- β -glucanase | Oryza sativa | 3 | 191 |
G2 | A. hindsii | AAR26001 | Endo-1,3- β -glucanase | Glycine max | 4 | 222 |
G3 | A. hindsii | AAR26001 | Endo-1,3- β -glucanase | Glycine max | 6 | 326 |
G4 | A. hindsii | AAD10380 | β -1,3-Glucanase precursor | Oryza sativa | 4 | 151 |
G5 | A. hindsii | Q9CA15 | Endo-1,3- β -glucanase | Arabidopsis thaliana | 2 | 102 |
G6 | A. hindsii | Q9ZP12 | Glucan endo-1,3- β -d-glucosidase | Cicer arietinum | 2 | 160 |
G7 | A. hindsii | AAK97661 | β -1,3-Glucanase | Sorghum bicolor | 1 | 64 |
G8 | A. hindsii | BAC15778 | Endo-1,3- β -glucanase | Oryza sativa | 2 | 118 |
G9 | A. hindsii | BAC84500 | β -1,3-Glucanase | Oryza sativa | 1 | 81 |
G10 | A. hindsii | Q6S4I9 | Endo- β -1,3-glucanase | Glycine tabacina | 3 | 136 |
G11 | A. hindsii | Q6S9W0 | Endo-1,3- β -glucanase | Glycine max | 5 | 280 |
G12 | A. hindsii | BAE53384 | β -1,3-Glucanase | Sesbania rostrata | 1 | 89 |
G13 | A. hindsii | BAE53384 | β -1,3-Glucanase | Sesbania rostrata | 2 | 104 |
G14 | A. hindsii | BAE53384 | β -1,3-Glucanase | Sesbania rostrata | 3 | 192 |
G15 | A. hindsii | BAC15778 | Endo-1,3- β -glucanase | Oryza sativa | 4 | 202 |
G16 | A. hindsii | BAE53384 | β -1,3-Glucanase | Sesbania rostrata | 2 | 154 |
G17 | A. collinsii | Q84Y06 | β -1,3-Glucanase | Fragaria ananassa | 2 | 143 |
G18 | A. collinsii | Q6S4I9 | Endo- β -1,3-glucanase | Glycine tabacina | 3 | 176 |
G19 | A. collinsii | O49016 | β -1,3-Glucanase | Glycine max | 1 | 77 |
G20 | A. collinsii | B2NK62 | β -1,3-Glucanase | Lotus japonicus | 4 | 258 |
G21 | A. collinsii | O49012 | β -1,3-Glucanase | Glycine max | 6 | 307 |
G22 | A. collinsii | P33157 | Glucan endo-1,3- β -glucosidase | Arabidopsis thaliana | 4 | 204 |
G23 | A. collinsii | Q84Y07 | β -1,3-Glucanase | Fragaria ananassa | 3 | 156 |
G24 | A. collinsii | Q6S4J7 | Endo- β -1,3-glucanase | Glycine tabacina | 4 | 251 |
G25 | A. collinsii | Q84Y07 | β -1,3-Glucanase | Fragaria ananassa | 1 | 74 |
G26 | A. collinsii | Q6GWG6 | β -1,3-Endoglucanase | Glycine soja | 4 | 230 |
G27 | A. collinsii | Q56AP1 | β -1,3-Glucanase | Fragaria ananassa | 6 | 312 |
G28 | A. collinsii | Q84Y07 | β -1,3-Glucanase | Fragaria ananassa | 7 | 357 |
G29 | A. collinsii | Q6S4I9 | Endo- β -1,3-glucanase | Glycine tabacina | 4 | 250 |
G30 | A. collinsii | B2NK62 | β -1,3-Glucanase | Lotus japonicus | 3 | 214 |
G31 | A. collinsii | Q84Y07 | β -1,3-Glucanase | Fragaria ananassa | 5 | 260 |
G32 | A. collinsii | O49016 | β -1,3-Glucanase | Glycine max | 3 | 149 |
G33 | A. collinsii | O49016 | β -1,3-Glucanase | Glycine max | 3 | 146 |
G34 | A. collinsii | O49016 | β -1,3-Glucanase | Glycine max | 3 | 182 |
G35 | A. collinsii | Q6S4J4 | Endo- β -1,3-glucanase | Glycine latrobeana | 2 | 84 |
C1 | A. hindsii | Q43685 | Chitinase class I | Vigna unguiculata | 5 | 225 |
C2 | A. hindsii | Q8MD06 | Chitinase | Leucaena leucocephala | 6 | 317 |
C3 | A. hindsii | AAM49597 | Chitinase | Leucaena leucocephala | 6 | 292 |
C4 | A. hindsii | Q7X9R8 | Chitinase | Euonymus europaeus | 16 | 884 |
C5 | A. hindsii | ABD66068 | Chitinase | Momordica charantia | 9 | 561 |
C6 | A. hindsii | ABD66068 | Chitinase | Momordica charantia | 4 | 242 |
C7 | A. hindsii | AAG37276 | Chitinase | Fragaria ananassa | 4 | 237 |
C8 | A. hindsii | AAB41324 | Class I chitinase | Medicago sativa | 4 | 253 |
C9 | A. collinsii | Q42428 | Chitinase | Castanea sativa | 8 | 507 |
C10 | A. collinsii | Q7X9R8 | Chitinase | Euonymus europaeus | 7 | 488 |
C11 | A. collinsii | Q8MD06 | Chitinase | Leucaena leucocephala | 7 | 410 |
C12 | A. collinsii | Q42428 | Chitinase | Castanea sativa | 8 | 559 |
C13 | A. collinsii | Q7X9R8 | Chitinase | Euonymus europaeus | 9 | 564 |
C14 | A. collinsii | Q9FEW1 | Endochitinase | Nicotiana sylvestris | 13 | 683 |
C15 | A. collinsii | Q207U1 | Chitinase | Momordica charantia | 13 | 798 |
C16 | A. collinsii | Q42428 | Chitinase | Castanea sativa | 11 | 714 |
C17 | A. collinsii | Q42428 | Chitinase | Castanea sativa | 9 | 623 |
C18 | A. collinsii | Q2VAC7 | Chitinase | Ficus pumila | 2 | 111 |
C19 | A. collinsii | Q93WX9 | Endochitinase | Musa acuminata | 2 | 126 |
O1 | A. hindsii | ABC55724 | Osmotin-like protein | Fragaria ananassa | 5 | 310 |
O2 | A. hindsii | AAU95243 | Osmotin-like protein | Solanum tuberosum | 4 | 248 |
O3 | A. hindsii | AAF13707 | Osmotin-like protein | Fragaria ananassa | 4 | 232 |
O4 | A. hindsii | AAU95238 | Osmotin-like protein | Solanum phureja | 3 | 194 |
O5 | A. collinsii | A9QVJ4 | Osmotin | Piper colubrinum | 7 | 446 |
O6 | A. collinsii | Q8S4L2 | Osmotin-like protein | Solanum nigrum | 5 | 254 |
O7 | A. collinsii | Q84MK8 | Osmotin | Solanum tuberosum | 2 | 104 |
T1 | A. hindsii | AAM00216 | Thaumatin-like protein | Prunus persica | 3 | 167 |
T2 | A. hindsii | CAA48278 | Thaumatin-like protein | Oryza sativa | 1 | 95 |
T3 | A. hindsii | CAA09229 | Thaumatin-like protein | Cicer arietinum | 3 | 142 |
T4 | A. hindsii | Q93XD2 | Thaumatin-like protein | Sambucus nigra | 5 | 277 |
T5 | A. collinsii | Q2VC78 | Thaumatin-like protein | Glycine max | 2 | 125 |
T6 | A. collinsii | P83332 | Thaumatin-like protein 1 | Prunus persica | 2 | 133 |
P1 | A. hindsii | AAO22065 | NtPRp27-like protein | Solanum tuberosum | 4 | 234 |
P2 | A. hindsii | AAO22065 | NtPRp27-like protein | Solanum tuberosum | 4 | 278 |
P3 | A. hindsii | AAO22065 | NtPRp27-like protein | Solanum tuberosum | 8 | 451 |
P4 | A. hindsii | BAA81904 | NtPRp27 | Nicotiana tabacum | 6 | 338 |
P5 | A. hindsii | BAA81904 | NtPRp27 | Nicotiana tabacum | 5 | 325 |
P6 | A. hindsii | AAK30143 | Pathogenesis-related protein PR-1 | Capsicum annuum | 2 | 160 |
P7 | A. hindsii | AAK30143 | Pathogenesis-related protein PR-1 | Capsicum annuum | 2 | 172 |
P8 | A. hindsii | CAA87071 | Pathogenesis-related protein PR-1 type | Sambucus nigra | 1 | 98 |
P9 | A. hindsii | CAA52894 | PR-1b pathogenesis- related protein | Hordeum vulgare | 3 | 186 |
P10 | A. collinsii | Q84XQ4 | NtPRp27-like protein | Solanum tuberosum | 6 | 327 |
P11 | A. collinsii | Q84XQ4 | NtPRp27-like protein | Solanum tuberosum | 3 | 160 |
P12 | A. collinsii | Q84XQ4 | NtPRp27-like protein | Solanum tuberosum | 6 | 317 |
P13 | A. collinsii | Q84XQ4 | NtPRp27-like protein | Solanum tuberosum | 6 | 338 |
P14 | A. collinsii | Q84XQ4 | NtPRp27-like protein | Solanum tuberosum | 1 | 69 |
P15 | A. collinsii | Q41359 | Pathogenesis-related protein PR-1 type | Sambucus nigra | 3 | 181 |
P16 | A. collinsii | Q41359 | Pathogenesis-related protein PR-1 type | Sambucus nigra | 2 | 124 |
P17 | A. collinsii | Q2XX51 | Pathogenesis-related protein 1 | Zea diploperennis | 5 | 275 |
P18 | A. collinsii | A0MZ69 | Pathogenesis-related protein 1 | Musa acuminata | 6 | 301 |
Spots excised from two-dimensional gels were analyzed by nanoLC-MS/MS, and the fragment spectral data were subjected to searches in the “planta” subdatabase of the European Bioinformatics Institute (http://www.ebi.ac.uk) using the ProteinLynx GLOBAL SERVER 2.3 (Waters; http://www.labbay.eu/software/protein-lynx-global-server-20). Proteins most commonly found in EFN of myrmecophyte Acacia plants belonged to the following families of PR proteins: PR-2 (β -1,3-glucanases), PR-3, -4, -8, and -11 (chitinases), and PR-1 and -5 (thaumatin-like and osmotin-like proteins). Several proteins other than PR proteins were also identified in EFN from A. hindsii and A. collinsii and classified within the glucosidases (EC 3.2.1), for example, as glycoside hydrolase and glucan-1,3- β -glucosidase (Table V).
In order to quantify the extent to which chitinases and glucanases contributed to the total amount of EFN proteins, the PD Quest 7.3.0 program (Bio-Rad) was used to conduct a relative quantification by determining the volume of each spot as optical density multiplied by its area (mm2). For A. hindsii, glucanase proteins contributed approximately 52% ± 2.1% to the total proteins, whereas chitinases contributed approximately 16% ± 2.6%. For A. collinsii, glucanase proteins contributed approximately 60% ± 2% and chitinases contributed approximately 19% ± 1.6% (n = 3 gels representing biological replicates).
Activity of Glucanases and Chitinases in EFN
Native gel assays were employed to separate basic and acidic isoforms of the dominating protein classes, chitinases and glucanases, and to prove their activity. Active isoforms of acidic and basic β -1,3-glucanases and chitinases were highly represented in the EFNs of the three myrmecophytes (Fig. 4). By contrast, EFNs of nonmyrmecophytes exhibited mainly activity of several isoforms of acidic chitinases and one band representing an active basic chitinase (Fig. 4, A and B) but no bands representing active glucanases (Fig. 4, C and D).
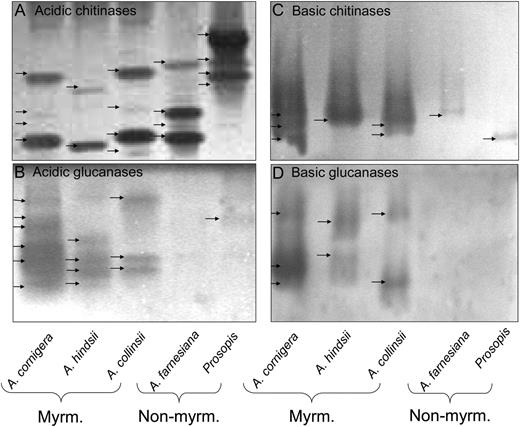
Enzyme activities in gel assays. Active isoforms of acidic chitinases (A), acidic glucanases (B), basic chitinases (C), and basic glucanases (D) in EFN of myrmecophytes (Myrm.; A. cornigera, A. hindsii, and A. collinsii) and nonmyrmecophytes (Non-myrm.; A. farnesiana and P. juliflora).
DISCUSSION
EFN of myrmecophytic Acacia species appeared free of fungi and yeast when collected under natural conditions, whereas the EFN of sympatric and closely related nonmyrmecophytes was regularly infested (GonzÁlez-Teuber et al., 2009). The purported biological activity of the nectars was investigated in bioassays on six phytopathogens, some of which have been previously reported to infect Acacia species (Roux and Wingfield, 1997; Kapoor et al., 2004). These pathogens could thus even use nectaries as entries to infect the plant tissue, as speculated earlier by BubÁn et al. (2003) and Farkas et al. (2007). EFN of myrmecophytes inhibited the growth of at least four among these species, including all those reported as pathogens of Acacia species (Table I), whereas no inhibitory effects were observed for nonmyrmecophyte EFN (Table II; Fig. 1).
We investigated the proteomes and selected enzymatic activities of both types of EFNs to elucidate whether their antimicrobial protection can be allotted unambiguously to their protein fraction. One-dimensional SDS-PAGE analysis (Fig. 2) revealed patterns that were very similar to those found in an earlier study (see Fig. 2 in GonzÁlez-Teuber et al., 2009), demonstrating that the proteome of Acacia EFN is stable under natural conditions and thus represents a species-specific trait. Many more abundant bands were detected in EFN of myrmecophytes as compared with nonmyrmecophytes. The complexity of the nectar proteome thus resembles its importance in the protection of EFN from microbial growth.
As the resolution of one-dimensional gels was too low to obtain a complete image of nectar proteomes, two-dimensional gel electrophoresis followed by MALDI-TOF/MS and LC-MS/MS was employed to characterize the proteomes of A. hindsii, A. collinsii, and the two nonmyrmecophytes (the proteome of A. cornigera EFN has already been described [GonzÁlez-Teuber et al., 2009]). The two-dimensional gel analysis revealed a much higher number of protein spots than the one-dimensional analysis (Fig. 3). PR proteins such as chitinases, glucanases, and thaumatin-like proteins clearly dominated the protein fraction both qualitatively (Table V) and quantitatively: putative glucanase proteins contributed more than 50% and putative chitinases more than 16% to the total proteins in EFN of both myrmecophytes. These values are slightly higher than those identified earlier for A. cornigera, in which glucanases represented some 40% and chitinases some 14% of the protein spots (GonzÁlez-Teuber et al., 2009).
PR proteins are currently divided into 17 families based on their structure and functional properties (Van Loon, 1999; Van Loon et al., 2006). Members of several of these families exert a damaging action on phytopathogen structures, thus exhibiting, for example, direct antifungal activity (Van Loon 1999; Van Loon et al., 2006). Interestingly, these families were represented by those proteins most commonly found in EFN of myrmecophyte Acacia plants: PR-2 (β -1,3-glucanases) and PR-3, -4, -8, and -11 (chitinases), which hydrolyze β -1,3-glucans and chitin, respectively; and PR-1 and -5 (thaumatin-like and osmotin-like proteins), which have been associated with activity against oomycetes (Van Loon et al., 2006).
The non-PR proteins found in myrmecophyte EFN were identified, for example, as glycoside hydrolase and glucan-1,3- β -glucosidase (Table V). The functions of these enzyme classes are commonly associated with carbohydrate metabolic processes (Zoran, 2008; BojarovÁ and Křen, 2009) and are likely involved in the control of the nectar's carbohydrate fraction, as described for an invertase that is also present in the EFN of Acacia myrmecophytes (Heil et al., 2005; GonzÁlez-Teuber et al., 2009).
Although SDS-PAGE analysis revealed very low abundances of proteins in the EFNs of both nonmyrmecophytes (Figs. 2 and 3), native gel assays indicated activities of several acidic chitinases and at least one basic chitinase in these EFNs (Fig. 4), whereas no clear hint of active glucanases was obtained (Fig. 4). For the EFNs of the three myrmecophytes, the activity assays confirmed the analysis of two-dimensional gels with nanoLC-MS/MS (Table V), since active isoforms of acidic and basic β -1,3-glucanases and chitinases were highly present in these EFNs (Fig. 4).
In summary, most proteins present in the EFN of myrmecophytes have been reported in the context of the defense of plants against pathogens. Do these proteins fulfill the same function in EFN, which represents a secreted liquid rather than tissue, and does the protection of EFN from microbial infestation rely completely on PR proteins? Size exclusion filtration and an experiment in which enzymes had been deactivated by heating confirmed that only the active protein fraction of EFN of the myrmecophytes inhibited phytopathogen growth (Tables III and IV). Moreover, the pathogens tested in our bioassays (Tables I and II) included the oomycete P. parasitica and different ascomycetes, which all differ in the composition of their cell walls. β -1,3-Glucans are the major component of hyphal walls of oomycetes, while ascomycetes have cell walls made of varying proportions of chitin and β -glucans (Wessels, 1994). Interestingly, the strongest inhibitory effects were found on the oomycete P. parasitica (Tables II and III). This result indicates that glucanases (the most abundant PR proteins in EFNs) were mainly responsible for the observed effects. Probably, the absence of active glucanases from the EFN of nonmyrmecophytes explains the differences in the protection from microorganisms between EFNs of both functional plant groups (Table II). Indeed, some in vitro studies showed that purified β -1,3-glucanases exhibited activity against phytopathogens such as Phytophthora and Alternaria (Tonón et al., 2002; Liu et al., 2009). Whereas the activity of plant protein extracts against different Phytophthora species lies in basic glucanase isoforms (Meins and Ahl, 1989; Kim and Hwang, 1997; Yi and Hwang, 1997), tobacco basic chitinase and glucanase isoforms exerted antifungal effects (Sela-Buurlage et al., 1993; Joosten et al., 1995; Lawrence et al., 1996). In vitro experiments have demonstrated that the antifungal effects of chitinases and β -1,3-glucanases are synergistically enhanced when both enzyme classes are present (Vogeli et al., 1988; Sela-Buurlage et al., 1993; Lawrence et al., 1996; Anfoka and Buchenauer, 1997).
In addition, PR-1 and PR-5 (thaumatin-like and osmotin-like proteins) have been associated with activity against oomycetes (Van Loon et al., 2006), and representatives of both families were detected in myrmecophyte EFN (Table V), but according to their molecular masses, they are not likely to be present in the EFNs of the nonmyrmecophytes: the latter did not show protein bands below 20 kD (Fig. 2). These PR proteins have been associated with strong antimicrobial activities in vitro (Monteiro et al., 2003) and might thus further contribute to the inhibitory action of myrmecophyte EFN against filamentous microorganisms. All these observations suggest that the activity of PR proteins underlies the protection of EFN from microorganisms and that the high protection level that can be observed in the EFNs of Acacia myrmecophytes is caused by the combined activity of several different PR proteins.
CONCLUSION
This study allows a clear allotting of the antimicrobial protection of myrmecophyte EFN to its protein fraction and demonstrates that the nectar exhibits a significant effect against purportedly phytopathogenic microorganisms. Because nonmyrmecophytes secrete EFN only transiently at low rates and in response to herbivory, these species appear not to invest much into the protection of their EFN. Several PR proteins with known antimicrobial activities were detected in myrmecophyte EFNs: an efficient protection apparently requires the combined activity of basic and acidic glucanases and chitinases, purportedly supported by the presence of thaumatin-like and osmotin-like proteins.
Nectarins, which protect tobacco floral nectar from microbial infection, exert their function via the production of reactive oxygen species (Carter and Thornburg, 2000, 2004a). By contrast, pollination drops contained PR proteins such as glucan- β -1,3-glucosidades, chitinases and thaumatin-like proteins (Wagner et al., 2007). At least some of these proteins (particularly glucan- β -1,3-glucosidases), however, are also involved in pollen development (Cheong et al., 2000; Van Loon et al., 2006) and could not be unambiguously linked to the antimicrobial protection of pollination drops.
The EFN of Acacia myrmecophytes appears protected from microbial infestation by the combined action of different PR proteins. This observation explains the high complexity of the proteomes of these EFNs, which by far exceeded those observed in tobacco floral nectar (five proteins; Carter et al., 1999) and in pollination drops of four gymnosperm species (five to 20 proteins; Wagner et al., 2007). Acacia myrmecophytes depend vitally on the antiherbivore defense that is exerted by their resident ants. Both chemical composition and amount of the EFN are important for keeping the presence and activity of ants a t the high levels that are required for plant survival (Heil et al., 2005, 2009). Therefore, the high importance of keeping the EFN free of phytopathogens and on other microorganisms, which can have negative effects on the composition of nectar chemistry, is reflected in the nectar proteome, which appears specifically tailored for antimicrobial protection.
MATERIALS AND METHODS
Study Species
This study was conducted using the three myrmecophytes Acacia cornigera, Acacia hindsii, and Acacia collinsii and the two nonmyrmecophytes Acacia farnesiana and Prosopis juliflora, which were determined following Janzen (1974) and Seigler and Ebinger (1995) and by comparison with specimens held at the Herbario MEXU at the Universidad Autónoma de México in Mexico City. The species grow sympatrically and belong to the same subfamily, the Mimosoideae. All plants used for EFN collection grew at their natural sites in the coastal area of the state of Oaxaca, Mexico, 5 km northwest of Puerto Escondido (Pacific coast; approximately 15°55′ N and 97°09′ W; elevation, 15 m). For bioassays, the following pathogenic species were obtained from the following collaborators: Phytophthora parasitica var nicotianae (isolate 201; kindly provided by P. Bonnet, INRA, Antibes, France); Fusarium oxysporum f. sp. radicis-lycopersici (ZUM 2407; collection of the IPO-DLO, Wageningen, The Netherlands); Verticillium dahliae isolated from naturally infected olive (Olea europeae) trees (kindly provided by J.M. García-Garrido, Consejo Superior de Investigaciones Científicas, Granada, Spain); Alternaria alternata isolated from naturally infected tomato (Solanum lycopersicum) plants (kindly provided by J. García, Consejo Superior de Investigaciones Científicas, Granada, Spain); Botrytis cinerea (CECT2100; Spanish Type Culture Collection, Universidad de Valencia, Burjassot, Spain); and Plectosphaerella cucumerina isolated from naturally infected Arabidopsis (Arabidopsis thaliana; Ton and Mauch-Mani, 2004; kindly provided by B. Mauch-Mani, University of Neuchâtel, Neuchatel, Switzerland).
Nectar Collection
EFN was collected in March and April 2007 and 2008 and November 2009 as follows. Branches of myrmecophytes were deprived of ants and other insects the day before nectar collection by cutting off the inhabited thorns, mechanically removing ants, and then placing the branch in a mesh bag after isolating it from the rest of the plant by applying a ring of sticky resin (Tangletrap; Tanglefoot Corp.). Branches of nonmyrmecophyte species were induced by applying 1 mmol of aqueous jasmonic acid solution (Heil et al., 2004) and then placed in mesh bags. After 1 d, the EFN was collected with a 20- μ L micropipette and stored directly at −20°C until analysis.
Antimicrobial Activity of EFN
Bioassays were carried out to evaluate a putative inhibitory effect of EFN on the growth of six phytopathogens: the oomycete P. parasitica and the ascomycetes F. oxysporum, V. dahliae, A. alternata, B. cinerea, and P. cucumerina. The genera Phytophthora, Fusarium, and Verticillium have been previously described as leaf pathogens for other Acacia species (Roux and Wingfield, 1997; Kapoor et al., 2004; Chimwamurombe et al., 2007; Table I). All species were maintained on potato dextrose agar (Sigma) plates. The antimicrobial assay was performed following the disc diffusion method. Sterile filter paper discs (0.5 cm diameter, equidistantly separated) impregnated with 10 μ L of EFN were placed on the surface of a new potato dextrose agar plate. One agar plug (2.5 cm2) containing growing hyphae that were obtained from the edges of an actively growing colony was placed in the center of the plate. Plates were then incubated at room temperature for 72 h. After incubation, plates were inspected for inhibition of microbial growth around the filter paper discs. The inhibition zones indicate that the respective microorganism is susceptible to some activity present in the EFN. Inhibitory effects of EFN on the six microbial species were quantified as the percentage of the line from the center of the microbial colony toward the nectar droplet that was not occupied by the microorganism. The experiment was conducted with EFNs of all five species. Myrmecophyte EFN (A. cornigera, A. hindsii, and A. collinsii) was adjusted to a concentration of 10% (w/v) using a portable refractometer, which represents the common EFN concentration found in the field for those species. A 10% sugar solution (Fru:Glc, 1:1) was used as a control for the EFNs of these species because EFN of Acacia myrmecophytes is naturally free of Suc (Heil et al., 2005). Nonmyrmecophyte EFN (A. farnesiana and P. juliflora) was adjusted to a concentration of 3% (w/v), and a 3% sugar solution (Suc:Fru:Glc, 1:1:1) was used as a control. All assays were repeated with three biologically independent replicates for every microorganism.
To evaluate which fraction of EFN causes its putative antimicrobial effects, a membrane filtration of 5 kD (Vivaspin 500; Vivascience Sartorius Group) was used to separate the protein fraction (greater than 5 kD) from the metabolite fraction (less than 5 kD) of EFN. After centrifugation (13,000 rpm for 5 min), both fractions were obtained for each plant species, and the disc diffusion method (see above for methodological description) was carried out using P. parasitica as the target organism.
As an independent attempt to causally relate the antimicrobial activity of myrmecophyte EFN to its protein fraction, EFN was collected from the three myrmecophytes in November 2009 and subjected to disc diffusion assays with the three fungi, A. alternata, F. oxysporum, and Botrytis capsici. Half of the nectar was heated to 100°C for 15 min before placing nectar droplets onto the agar plates. The experiment was repeated with three biologically independent samples for every fungal species.
SDS-PAGE, Two-Dimensional PAGE, and MS
Before one- or two-dimensional SDS-PAGE, nectar samples were adjusted to a concentration of 3% of soluble solids (w/v) using a refractometer (10–20 μ L for myrmecophyte species and 150–200 μ L for nonmyrmecophyte species) and then precipitated with 10% TCA (v/v) at 4°C (nectar:TCA = 1:2). The mixture was incubated for 1.5 h at 4°C and centrifuged at 13,000 rpm for 15 min at 4°C. Then, the supernatant was removed and 0.5 mL of absolute ethanol was added. Samples were centrifuged at 7,000 rpm for 10 min at 4°C. Proteins (15 μ g per sample) were separated on a 13% SDS-PAGE Laemmli gel (Laemmli, 1970) and stained with Coomassie Brilliant Blue solution. The electrophoresis running conditions consisted of 130 V for 1.5 h.
Proteins in EFN of the myrmecophytes A. hindsii and A. collinsii and of the nonmyrmecophytes A. farnesiana and P. juliflora were identified with two-dimensional gel electrophoresis and MALDI-TOF/MS using three replicate gels that represented biologically independent samples. For A. farnesiana and Prosopis, only one replicate gel each was obtained, which represented a pooled sample obtained from some 20 individual plants per species. The two-dimensional PAGE procedure was according to Giri et al. (2006) with the following modifications. After water removal from the sample wells, the gel plugs were reduced using 20 μ L of 10 mm dithiothreitol in 25 mm ammonium bicarbonate for 1 h at 56°C, alkylated by 20 μ L of 55 mm iodoacetic acid at room temperature in the dark for 45 min, and rinsed with 70 μ L of 50 mm ammonium bicarbonate/50% acetonitrile two times for 20 min to remove the Coomassie Brilliant Blue stain. The second wash was done with 70 μ L of 70% acetonitrile for 20 min. The gel plugs were then air dried for 30 min and overlaid with 10 μ L of 25 mm ammonium bicarbonate containing 70 ng of porcine trypsin (sequencing grade; Promega). The microtiter plates were subsequently covered with aluminum foil, and the proteins were digested overnight at 37°C. The resulting peptides were extracted from the gel plugs by adding 50 μ L of 50% acetonitrile in 0.1% trifluoroacetic acid for 20 min and an additional extraction with 50 μ L of the same extraction buffer. The extracts were collected on an extraction microtiter plate and vacuum dried to remove any remaining liquid and the volatile ammonium bicarbonate. A MALDImicro MX mass spectrometer (Waters-Micromass) was used in reflectron mode for monitoring of protein digestion and database identification. The tryptic peptides were reconstituted in 6 μ L of aqueous 0.1% trifluoroacetic acid containing 3% MeCN.
Peptides not identified by MALDI-TOF/MS were identified de novo using LC-MS/MS (Giri et al., 2006; Pauchet et al., 2008). The aliquots of peptides (1.5–6 μ L) were injected on a nanoAcquity nanoUPLC system (Waters). A mobile phase 500 nL min−1 flow of 0.1% aqueous formic acid (15 μ L min−1 for 1 min) was used to concentrate and desalt the samples on a 20- × 0.180-mm Symmetry C18, 5- μ m particle precolumn. The samples were eluted on a 100-mm × 100- μ m i.d., 1.7- μ m BEH nanoAcquity C18 column using an increasing acetonitrile gradient in 0.1% aqueous formic acid. Phases A (0.1% formic acid) and B (100% MeCN in 0.1% formic acid) were linearly mixed using a gradient program going up to 4% phase B in A in 2 min, increasing to 35% B in 8 min, 85% B over 0.5 min, holding at 85% B for 0.5 min, finally decreasing to 1% B over 0.1 min, and holding at 1% B until the 20th min. The eluted peptides were transferred to the NanoElectroSpray source of a Synapt HDMS Q-TOF tandem mass spectrometer (Waters-Micromass) through a Teflon capillary union and a metal-coated nanoelectrospray tip (Picotip [50 × 0.36 mm, 10 μ m i.d.]; Waters). The source temperature was set to 60°C, cone gas flow was 20 L h−1, and the nanoelectrospray voltage was 3.2 kV. The TOF analyzer was used in reflectron mode. The MS/MS spectra were collected in 1-s intervals in the range of 50 to 1,600 mass-to-charge ratio. A mixture of 680 fmol μ L−1 human Glu-fibrinopeptide B and 80 fmol μ L−1 reserpine in 0.1% formic acid-acetonitrile (1:1, v/v) was infused at a flow rate of 0.5 μ L min−1 and fragmentation voltage of 24 eV through the reference NanoLockSpray source every fifth scan to compensate for mass shifts in the MS and MS/MS fragmentation mode due to temperature fluctuations.
Data were collected by MassLynx version 4.1 software. The ProteinLynx Global Server Browser version 2.3 software (both Waters) was used for baseline subtraction and smoothing, deisotoping, de novo peptide sequence identification, and database searches. The peptide fragment spectra were searched against the European Bioinformatics Institute planta-specific subdatabase downloaded on July 22, 2008 from http://www.ebi.ac.uk/. The protein database identification search parameters were as follows: peptide mass tolerance, 20 ppm and minimum two peptides found; estimated calibration error, 0.005 D; one possible missed cleavage; carbamidomethylation of Cys residues and possible oxidation of Met residues. A 0.05-D mass deviation and a calibration error of 0.005 D for de novo sequencing were allowed. The BLAST search was performed internally using the MS-BLAST algorithm (Shevchenko et al., 2001) using minimum one peptide matching at an expect score of 100, with no-gap-hspmax100-sort_by_totalscore -span1 advanced options and PAM30MS search matrix.
In-Gel Assays of Activities of Glucanases and Chitinases
Acidic and basic chitinases and glucanases were determined by native gel assays in order to detect and separate active isoforms in nectar. For the detection of acidic and neutral chitinases and glucanases, 10 μ g of proteins per sample was separated by PAGE on 15% (w/v) polyacrylamide gels under native conditions at pH 8.9 according to Davis (1964). Glycol chitin was embedded in gels at 0.01% (w/v) and used as substrate for chitinase activities. After electrophoresis, chitinase gels were incubated for 4 h at 37°C in 50 mm sodium acetate buffer, pH 5.0. For β -1,3-glucanase activities, a soluble fraction of purified β -glucans from Saccharomyces cerevisiae was used as a substrate (Grenier and Asselin, 1993). β -Glucans were incorporated at a final concentration of 0.6 mg mL−1 directly in the separation gels. After electrophoresis, glucanase gels were incubated for 3 h at 37°C in 50 mm sodium acetate buffer, pH 5.0, as well. Running conditions for electrophoresis of chitinases and glucanases were 100 V for 1.5 h. Chitinase activities on gels were revealed by fluorescent staining (10 min) using Calcofluor white M2R (0.01%, w/v) in 500 mm Tris-HCl (pH 8.9) and visualized after destaining under UV light. Glucanase activities on gels were revealed by staining the gels for 15 min with 0.025% (w/v) aniline blue fluorochrome in 150 mm K2HPO4, pH 8.6, and visualized under UV light (365 nm).
For the detection of basic chitinases and glucanases, 10 μ g of proteins per sample was analyzed by PAGE on 12% (w/v) polyacrylamide gels under native conditions at pH 4.3 as described by Reisfeld et al. (1962). For basic activities, substrates of glycol chitin and β -glucans were incorporated in an additional polyacrylamide gel (overlay gel, 7.5%) to which proteins were transferred. Transfer of proteins was done by blotting for 3 to 4 h at 37°C. After electrophoresis, separation gels (attached to a supporting glass plate) were covered with the overlay gel. Bubbles between both gels were eliminated by gently sliding the overlay gel on the top of the separated gel. Both gels together (separating gel plus overlay gel) were incubated for 3 to 4 h under moist conditions at 37°C with 50 mm sodium acetate buffer, pH 5.0. Chitinase and glucanase activities on overlay gels were revealed and visualized in the same way as for acidic/neutral activities (see above). All electrophoreses were repeated at least three times. All chemicals used were purchased from Sigma Chemical Co.
Supplemental Data
The following materials are available in the online version of this article.
Supplemental Table S1. De novo peptide sequences obtained for EFN proteins of P. juliflora.
Supplemental Table S2. De novo peptide sequences obtained for EFN proteins of A. hindsii and A. collinsii.
ACKNOWLEDGMENTS
We thank Juan Carlos Silva Bueno for his help with nectar collection and Dr. Arturo Reyes for kindly supporting our work at the Universidad del Mar, Puerto Escondido, Mexico. We are very grateful to Antje Loele and Domancar Orona for their help in the protein analysis, to Juan García and IvÁn FernÁndez for kind support in the laboratory, and to two anonymous referees for highly valuable comments on an earlier version of the manuscript.
LITERATURE CITED
Author notes
This work was supported by the Deutsche Forschungsgemeinschaft (grant no. He 3169/4–2), the Consejo Nacional de Ciencia y Tecnología de México, the German Academic Exchange Service (fellowship to M.G.-T.), and the Max Planck Society.
Corresponding author; e-mail [email protected].
The author responsible for distribution of materials integral to the findings presented in this article in accordance with the policy described in the Instructions for Authors (www.plantphysiol.org) is: Martin Heil ([email protected]).
The online version of this article contains Web-only data.
Open Access articles can be viewed online without a subscription.