-
PDF
- Split View
-
Views
-
Cite
Cite
Xiaodong Liu, Jian Huang, Sriram Parameswaran, Toshiro Ito, Brandon Seubert, Max Auer, Amy Rymaszewski, Gengxiang Jia, Heather A. Owen, Dazhong Zhao, The SPOROCYTELESS/NOZZLE Gene Is Involved in Controlling Stamen Identity in Arabidopsis, Plant Physiology, Volume 151, Issue 3, November 2009, Pages 1401–1411, https://doi.org/10.1104/pp.109.145896
- Share Icon Share
Abstract
The stamen, which consists of an anther and a filament, is the male reproductive organ in a flower. The specification of stamen identity in Arabidopsis (Arabidopsis thaliana) is controlled by a combination of the B genes APETALA3 (AP3) and PISTILLATA, the C gene AGAMOUS (AG), and the E genes SEPALLATA1 (SEP1) to SEP4. The “floral organ-building” gene SPOROCYTELESS/NOZZLE (SPL/NZZ) plays a central role in regulating anther cell differentiation. However, much less is known about how “floral organ identity” and floral organ-building genes interact to control floral organ development. In this study, we report that ectopic expression of SPL/NZZ not only affects flower development in the wild-type background but also leads to the transformation of petal-like organs into stamen-like organs in flowers of ap2-1, a weak ap2 mutant allele. Moreover, our loss-of-function analysis indicates that the spl/nzz mutant enhances the phenotype of the ag weak allele ag-4. Furthermore, ectopic expression and overexpression of SPL/NZZ altered expression of AG, SEP3, and AP2 in rosette leaves and flowers, while ectopic expression of SPL/NZZ resulted in ectopic expression of AG and SEP3 in the outer whorls of flowers. Our results indicate that the SPL/NZZ gene is engaged in controlling stamen identity via interacting with genes required for stamen identity in Arabidopsis.
The Arabidopsis (Arabidopsis thaliana) flower contains four types of organs that are arranged in four concentric whorls. Four sepals are found in the outermost whorl, four petals in the second whorl, six stamens in whorl 3, and two carpels in the innermost whorl. As in most angiosperms, flower development in Arabidopsis occurs in four steps: transition from vegetative growth to reproductive growth, establishment of floral meristem identity, specification of floral organ identity, and floral organ morphogenesis (Zhao et al., 2001a; Jack, 2004). Extensive molecular genetic studies have not only uncovered a large number of genes that are required for flower development but also elucidated regulatory relationships among key genes.
The ABC model describes three classes of homeotic genes, termed A, B, and C, which specify floral organ identity in four whorls (Haughn and Somerville, 1988; Bowman et al., 1991b; Coen and Meyerowitz, 1991; Meyerowitz et al., 1991; Ma, 1994; Weigel and Meyerowitz, 1994). In Arabidopsis, the class A genes APETALA1 (AP1) and AP2 are active in whorls one and two, the class B genes AP3 and PISTILATA are active in whorls two and three, and the class C gene AGAMOUS (AG) is active in whorls three and four. Class A genes alone are required for sepal identity. Class A and B genes together direct petal identity. Class B and C genes control stamen identity, while the class C gene solely specifies carpels. Moreover, class A and C genes function antagonistically (Drews et al., 1991; Mizukami and Ma, 1992). The activity of class A genes in whorls one and two prevents the function of the class C gene in the same whorls and vice versa in whorls three and four. A new addition to the ABC model is that the E genes (SEPALLATA1 [SEP1]–SEP4) are involved in specifying organ identity in all four whorls via interacting with ABC regulators (Pelaz et al., 2000; Honma and Goto, 2001; Ditta et al., 2004; Melzer et al., 2009). The ABC model is applicable to diverse plant species in terms of regulation of floral organ identity. However, much less is known about genes that are necessary for building floral organs.
Stamens are male reproductive organs in a flower. Each stamen consists of an anther, where the male gametophyte develops, and a filament, which provides water and nutrients to the anther and anchors the anther to the flower. The anther is a four-lobed structure in Arabidopsis. Each mature anther lobe is composed of five types of well-organized cell layers: epidermis, endothecium, middle layer, tapetum, and microsporocyte (pollen mother cell; Goldberg et al., 1993; Sanders et al., 1999). Microsporocytes are reproductive cells that undergo meiosis and eventually develop into pollen grains. The remaining nonreproductive (somatic) cells are required for the normal development and release of pollen. In particular, the tapetum is essential for pollen development. After the specification of stamen identity, anther development involves a series of cell division, cell differentiation, and cell death, resulting in the formation of reproductive microsporocytes and somatic cell layers. Although microarray experiments uncovered many genes that are important for anther development (Zik and Irish, 2003; Hennig et al., 2004; Wellmer et al., 2004; Lu et al., 2006; Alves-Ferreira et al., 2007; Ma et al., 2007, 2008; Wijeratne et al., 2007), so far only a few genes have been identified to play direct roles in controlling differentiation of various anther cells.
Besides genes that encode signaling proteins (Canales et al., 2002; Zhao et al., 2002; Yang et al., 2003; Albrecht et al., 2005; Colcombet et al., 2005; Hord et al., 2006; Mizuno et al., 2007; Jia et al., 2008; Zhao, 2009), the SPOROCYTELESS/NOZZLE (SPL/NZZ) gene plays a central role in controlling early anther cell differentiation (Schiefthaler et al., 1999; Yang et al., 1999). Anthers in the spl/nzz mutant do not produce microsporocytes or anther walls, suggesting that the SPL/NZZ gene is required for the differentiation of microsporocytes and anther walls, including the tapetum. The SPL/NZZ gene encodes a novel protein related to MADS box transcription factors. The direct activation of SPL/NZZ by AG is required for early anther development (Ito et al., 2004). Detected as early as the stage when stamen primordia are generated, the expression of SPL/NZZ coincides with the expression of AG during early anther development (Yanofsky et al., 1990; Bowman et al., 1991a; Drews et al., 1991; Ito et al., 2004). Therefore, we hypothesize that SPL/NZZ might be involved in controlling stamen identity.
Here, we report our characterization of the SPL/NZZ function in specifying stamen identity. We show that ectopic expression of SPL/NZZ affects both leaf and flower development. Furthermore, the ectopic expression of SPL/NZZ leads to the transformation of petal-like organs into stamen-like organs in flowers of ap2-1, a weak ap2 mutant allele. The spl/nzz mutant also enhances the phenotype of the ag weak allele ag-4. In addition, ectopic expression and overexpression of SPL/NZZ alter the expression of AG, SEP3, and AP2 in rosette leaves and flowers. Our results indicate that the “floral organ-building” gene SPL/NZZ is not only essential for early anther cell differentiation but also is engaged in controlling stamen identity in Arabidopsis.
RESULTS
Ectopic Expression and Overexpression of SPL/NZZ Cause Abnormal Plant Development
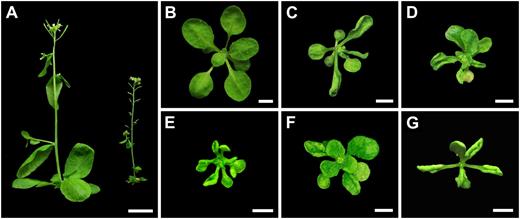
Pro35S:SPL/NZZ plants are abnormal in development. A, Compared with the wild type (left), the Pro35S:SPL/NZZ transgenic plant (right) had shorter stature and reduced fertility. B, Wild-type seedling. C to E, Pro35S:SPL/NZZ seedlings showing variabilities in their up-curled rosette leaves. F, Pro35S:SPL/NZZ-GR seedling exhibiting wrinkled and up-curled rosette leaves after treatment with DEX. G, Pro35S:AG seedling displaying up-curled rosette leaves. Bars = 1 cm (A) and 0.5 cm (B–G).
Ectopic Expression of SPL/NZZ Affects Flower Development

Scanning electron micrographs showing defects in flower development in Pro35S:SPL/NZZ plants. A, A wild-type flower showing normal petals. B, A Pro35S:SPL/NZZ flower showing narrow petals and shortened stamens. C, A narrow petal in whorl 2 of a Pro35S:SPL/NZZ flower. D, Wild-type petal epidermal cells showing uniform size and conical shape. E, Wild-type anther epidermal cells exhibiting irregular cell edges and wavy ridges. F, A narrow and up-curled petal in whorl 2 of a Pro35S:SPL/NZZ flower. G, High-magnification view of epidermal cells of the organ shown in C displaying elongated shape and uneven sizes. H, High-magnification view of epidermal cells of the organ shown in F exhibiting the resemblance to wild-type anther epidermal cells in E. Bars = 0.5 mm (A and B), 50 μm (C and F), and 10 μm (D, E, G, and H).
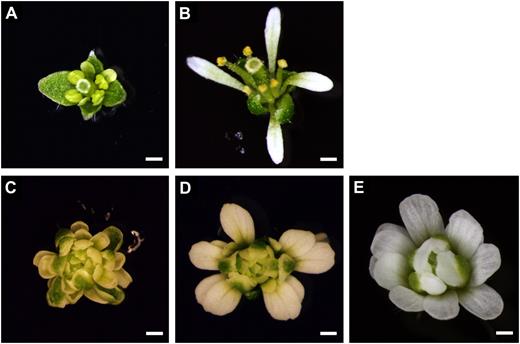
The petal phenotype caused by Pro35S:SPL/NZZ may require a functional AG. A, A Pro35S:SPL/NZZ young flower showing unclosed sepals and small petals. B, A Pro35S:SPL/NZZ flower showing four narrow petals. C, An ag-1 Pro35S:SPL/NZZ young flower. D, An ag-1 Pro35S:SPL/NZZ flower showing normal petals in the second whorl. E, An ag-1 flower. Bars = 0.5 mm.
Comparison of floral organs
Leaf like in whorl 1, sepals with leaf or stigma structures; stamen like in whorl 2, narrow, up-curled, or with stamen structures; stamen like in whorl 3, stamen with petal structures. The first 10 flowers from 10 plants were examined for each genotype.
Phenotype . | Genotype . | . | . | . | . | ||||
---|---|---|---|---|---|---|---|---|---|
. | Wild Type . | spl . | Pro35S:SPL/NZZ . | ap2-1 . | ap2-1Pro35S:SPL/NZZ . | ||||
Whorl 1 sepal | 4.00 ± 0.00 | 4.00 ± 0.00 | 4.00 ± 0.00 | 0 | 0 | ||||
Leaf like | 4.00 ± 0.00 | 4.00 ± 0.00 | |||||||
Whorl 2 petal | 4.00 ± 0.00 | 4.00 ± 0.00 | 3.47 ± 0.10 | 2.37 ± 0.11 | 0.65 ± 0.09 | ||||
Stamen like | 0 | 0 | 0.24 ± 0.06 | 0.62 ± 0.11 | 2.43 ± 0.14 | ||||
Whorl 3 stamen | 6.00 ± 0.00 | 5.44 ± 0.06 | 4.90 ± 0.12 | 5.25 ± 0.07 | 4.68 ± 0.08 | ||||
Stamen like | 0 | 0 | 0 | 0.21 ± 0.05 | 0.03 ± 0.01 | ||||
Carpel | 2.00 ± 0.00 | 2.00 ± 0.00 | 2.00 ± 0.00 | 2.00 ± 0.00 | 2.00 ± 0.00 |
Phenotype . | Genotype . | . | . | . | . | ||||
---|---|---|---|---|---|---|---|---|---|
. | Wild Type . | spl . | Pro35S:SPL/NZZ . | ap2-1 . | ap2-1Pro35S:SPL/NZZ . | ||||
Whorl 1 sepal | 4.00 ± 0.00 | 4.00 ± 0.00 | 4.00 ± 0.00 | 0 | 0 | ||||
Leaf like | 4.00 ± 0.00 | 4.00 ± 0.00 | |||||||
Whorl 2 petal | 4.00 ± 0.00 | 4.00 ± 0.00 | 3.47 ± 0.10 | 2.37 ± 0.11 | 0.65 ± 0.09 | ||||
Stamen like | 0 | 0 | 0.24 ± 0.06 | 0.62 ± 0.11 | 2.43 ± 0.14 | ||||
Whorl 3 stamen | 6.00 ± 0.00 | 5.44 ± 0.06 | 4.90 ± 0.12 | 5.25 ± 0.07 | 4.68 ± 0.08 | ||||
Stamen like | 0 | 0 | 0 | 0.21 ± 0.05 | 0.03 ± 0.01 | ||||
Carpel | 2.00 ± 0.00 | 2.00 ± 0.00 | 2.00 ± 0.00 | 2.00 ± 0.00 | 2.00 ± 0.00 |
Comparison of floral organs
Leaf like in whorl 1, sepals with leaf or stigma structures; stamen like in whorl 2, narrow, up-curled, or with stamen structures; stamen like in whorl 3, stamen with petal structures. The first 10 flowers from 10 plants were examined for each genotype.
Phenotype . | Genotype . | . | . | . | . | ||||
---|---|---|---|---|---|---|---|---|---|
. | Wild Type . | spl . | Pro35S:SPL/NZZ . | ap2-1 . | ap2-1Pro35S:SPL/NZZ . | ||||
Whorl 1 sepal | 4.00 ± 0.00 | 4.00 ± 0.00 | 4.00 ± 0.00 | 0 | 0 | ||||
Leaf like | 4.00 ± 0.00 | 4.00 ± 0.00 | |||||||
Whorl 2 petal | 4.00 ± 0.00 | 4.00 ± 0.00 | 3.47 ± 0.10 | 2.37 ± 0.11 | 0.65 ± 0.09 | ||||
Stamen like | 0 | 0 | 0.24 ± 0.06 | 0.62 ± 0.11 | 2.43 ± 0.14 | ||||
Whorl 3 stamen | 6.00 ± 0.00 | 5.44 ± 0.06 | 4.90 ± 0.12 | 5.25 ± 0.07 | 4.68 ± 0.08 | ||||
Stamen like | 0 | 0 | 0 | 0.21 ± 0.05 | 0.03 ± 0.01 | ||||
Carpel | 2.00 ± 0.00 | 2.00 ± 0.00 | 2.00 ± 0.00 | 2.00 ± 0.00 | 2.00 ± 0.00 |
Phenotype . | Genotype . | . | . | . | . | ||||
---|---|---|---|---|---|---|---|---|---|
. | Wild Type . | spl . | Pro35S:SPL/NZZ . | ap2-1 . | ap2-1Pro35S:SPL/NZZ . | ||||
Whorl 1 sepal | 4.00 ± 0.00 | 4.00 ± 0.00 | 4.00 ± 0.00 | 0 | 0 | ||||
Leaf like | 4.00 ± 0.00 | 4.00 ± 0.00 | |||||||
Whorl 2 petal | 4.00 ± 0.00 | 4.00 ± 0.00 | 3.47 ± 0.10 | 2.37 ± 0.11 | 0.65 ± 0.09 | ||||
Stamen like | 0 | 0 | 0.24 ± 0.06 | 0.62 ± 0.11 | 2.43 ± 0.14 | ||||
Whorl 3 stamen | 6.00 ± 0.00 | 5.44 ± 0.06 | 4.90 ± 0.12 | 5.25 ± 0.07 | 4.68 ± 0.08 | ||||
Stamen like | 0 | 0 | 0 | 0.21 ± 0.05 | 0.03 ± 0.01 | ||||
Carpel | 2.00 ± 0.00 | 2.00 ± 0.00 | 2.00 ± 0.00 | 2.00 ± 0.00 | 2.00 ± 0.00 |
Ectopic Expression of SPL/NZZ Enhances the ap2-1 Flower Phenotype in Whorl 2
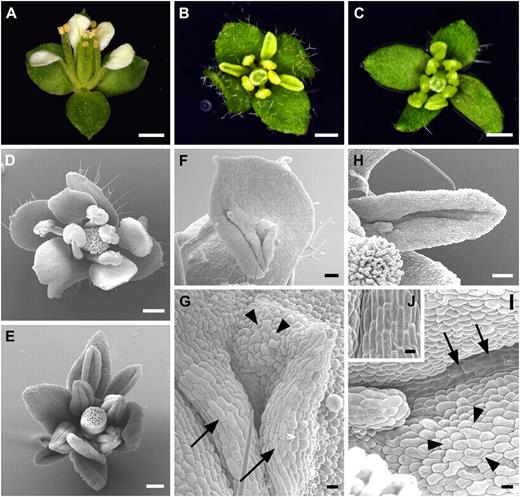
Ectopic expression of SPL/NZZ promoted stamen identity in the second floral whorl of the ap2-1 mutant. A, An ap2-1 flower showing leaf-like organs in whorl 1 and petals in whorl 2. B, An ap2-1 Pro35S:SPL/NZZ flower exhibiting narrow and up-curled petal-like organs in whorl 2. C, An ap2-1 Pro35S:SPL/NZZ flower showing all stamens in whorls two and three. D, SEM image showing a Pro35S:SPL/NZZ flower. E, SEM image exhibiting an ap2-1 Pro35S:SPL/NZZ flower with up-curled stamen-like organs in whorl 2. F and G, SEM images showing a stamen-like organ (F) as well as filament-like (arrows) and anther-like (arrowheads) epidermal cells (G; high-magnification view of F) in an ap2-1 Pro35S:SPL/NZZ flower. H and I, SEM images displaying an up-curled stamen-like organ (H) as well as filament-like (arrows) and anther-like (arrowheads) epidermal cells (I; high-magnification view of H) in an ap2-1 Pro35S:SPL/NZZ flower. J, SEM image exhibiting wild-type filament cells. Bars = 0.5 mm (A–D), 100 μm (E), 50 μm (F and H), and 10 μm (G, I, and J).
Loss-of-Function Analysis Indicates That SPL/NZZ Is Involved in Controlling Stamen Identity
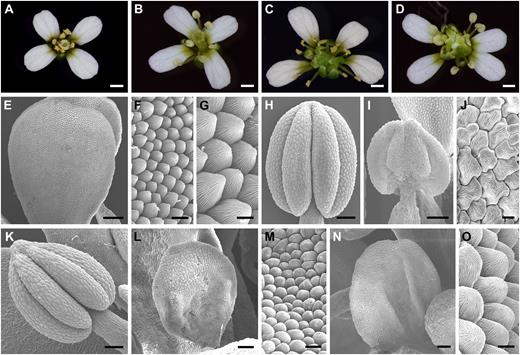
Loss-of-function analysis suggested that SPL/NZZ was involved in regulating stamen identity. A, A wild-type flower showing petals in whorl 2 and stamens in whorl 3. B, An spl flower exhibiting normal petals in whorl 2 and sterile stamens (no pollen released) in whorl 3. C, An ag-4 flower showing normal petals in whorl 2 and normal stamens in whorl 3. D, An splag-4 double mutant flower displaying normal petals in whorl 2 and stamens resembling those of spl inward whorl 2. E, SEM image exhibiting a wild-type petal. F and G, Low-magnification (F) and high-magnification (G) views of wild-type petal epidermal cells showing uniform size and cone shape. H, A wild-type anther. I, An spl anther showing flattened shape and undeveloped lobes. J, High-magnification view of epidermal cells of the spl anther, which are the same as wild-type anther epidermal cells (Fig. 2D). K, An ag-4 anther is similar to the wild-type anther. L, An splag-4 double mutant petal-like (petaloid) organ from whorl 3 showing no developed anther lobes. M, High-magnification view of epidermal cells of the organ shown in L showing the resemblance to wild-type petal epidermal cells in F. N, An splag-4 double mutant petal-like organ from whorl 3. O, High-magnification view of epidermal cells of the organ shown in N, which is similar to wild-type petal epidermal cells in G. Bars = 0.5 mm (A–D), 200 μm (E), 50 μm (L and N), 20 μm (H, I, and K), 10 μm (F and M), and 5 μm (G, J, and O).
Ectopic Expression and Overexpression of SPL/NZZ Alter the Expression of Genes Required for Stamen Identity
Previous studies showed that class B, C, and E genes cooperate to control stamen identity. The expansion of C gene function to whorls one and two leads to the formation of carpel and stamen-like structures in two outer whorls (Drews et al., 1991; Mizukami and Ma, 1992). In addition, ectopic expression of AG and SEP3 resulted in the formation of curled rosette leaves (Fig. 1G; Mizukami and Ma, 1992; Goodrich et al., 1997; Castillejo et al., 2005). Plants impaired in function of the CURLYLEAF (CLF) gene, which is required for maintaining the repression of AG epigenetically, also produced curled rosette leaves (Goodrich et al., 1997). Our results demonstrated that the ectopic expression of SPL/NZZ promotes stamen identity in the second whorl and causes the formation of curled rosette leaves. Therefore, to test how SPL/NZZ controls stamen identity, we carried out regular semiquantitative and quantitative real-time reverse transcription (RT)-PCR experiments to examine the expression of AP1, AP2, AP3, AG, SEP3, and CLF in Pro35S:SPL/NZZ and spl-D plants.

Ectopic expression and overexpression of SPL/NZZ altered the expression of genes required for stamen identity in rosette leaves and flowers. A, RT-PCR results showing greatly increased expression of SPL/NZZ, AG, and SEP3 in both Pro35S:SPL/NZZ and spl-D rosette leaves. There was no detectable change of CLF expression. B, RT-PCR results showing that expression of SPL/NZZ, AG, and SEP3 was increased in Pro35S:SPL/NZZ and spl-D flowers as well as Pro35S:SPL/NZZ sepals and petals. The expression of AP2 was slightly decreased, while the expression of AP1 and AP3 seemed unchanged. C, Quantitative real-time RT-PCR results showing the increased expression of AG in rosette leaves, flowers, sepals, and petals of Pro35S:SPL/NZZ plants. D, Quantitative real-time RT-PCR results showing the increased expression of SEP3 in rosette leaves, flowers, sepals, and petals of Pro35S:SPL/NZZ plants. E, Quantitative real-time RT-PCR results illustrating the decreased expression of AP2 in rosette leaves, flowers, sepals, and petals of Pro35S:SPL/NZZ plants. F, Semiquantitative RT-PCR results showing the increased expression of SEP3 in Pro35S:SPL/NZZ-GRag-1 inflorescences after both DEX and DEX + Cyc treatments for 4 h. The LIPASE gene was used as a control. The transcripts in wild-type rosette leaves in C to E were used as a standard for normalization. Asterisks indicate that the difference is significant (P < 0.01 or P < 0.05). REL, Relative expression level; S & P, sepal and petal; 35S:SPL, Pro35S:SPL/NZZ.
To examine whether ectopic expression SPL/NZZ affects the expression of AG, SEP3, and AP2 in sepals and petals, young buds from both wild-type and Pro35S:SPL/NZZ plants were dissected and then sepals and petals were collected with the dissection microscope. Both RT-PCR and quantitative real-time RT-PCR results showed that AG and SEP3 were ectopically expressed in sepals and petals of Pro35S:SPL/NZZ flowers (Fig. 6, B–D). Additionally, the expression of AP2 was decreased in sepals and petals (Fig. 6, B and E). In summary, our results indicate that both ectopic expression and overexpression of SPL/NZZ increase the expression of AG and SEP3 in rosette leaves and flowers. Furthermore, the ectopic expression of SPL/NZZ causes ectopic expression of AG and SEP3 in flower whorls one and two, which may affect the A gene function.
To test whether SPL/NZZ directly activates the expression of AG or SEP3, the expression of AG and SEP3 was examined in nzz-2 Pro35S:SPL/NZZ-GR plants after DEX as well as both DEX and cycloheximide (Cyc) treatments. The expression of AG was induced by DEX alone, while when Cyc was present, the expression of AG did not change (data not shown). The expression of SEP3 was rapidly induced in nzz-2Pro35S:SPL/NZZ-GR inflorescences in the presence of DEX as well as both DEX and Cyc after 4 h (Fig. 6F).
To further examine whether AG is required for the formation of curled rosette leaves, the Pro35S:SPL/NZZ construct was transformed into ag-1 heterozygous plants and the spl-D mutant was crossed to ag-1 heterozygous plants. The rosette leaves in both ag-1Pro35S:SPL/NZZ and ag-1spl-D plants also exhibited the curled-leaf phenotype (Supplemental Fig. S1). However, plants with the most severe curled-leaf phenotype were not observed. Therefore, the formation of curled leaves was not fully dependent on a functional AG. Our results suggest that the induction of AG expression might be caused by the direct activation of SEP3 by SPL/NZZ in Pro35S:SPL/NZZ and spl-D plants.
DISCUSSION
Anther development entails a series of processes, including specification of stamen identity, establishment of anther adaxial and abaxial polarity, and differentiation of reproductive microsporocytes and somatic cell layers. To date, interactions between “stamen identity” and “stamen-building” genes have not been extensively studied in the context of development, although excellent advances have been made in understanding stamen identity, early anther cell differentiation, male meiosis, pollen development, and anther dehiscence (Goldberg et al., 1993; Walbot and Evans, 2003; Jack, 2004; McCormick, 2004; Scott et al., 2004; Ma, 2005; Feng and Dickinson, 2007; Borg et al., 2009; Wilson and Zhang, 2009; Zhao, 2009). Stamen identity is controlled by B, C, and E genes. The SPL/NZZ transcription factor plays a central role in regulating early anther cell differentiation, since the spl/nzz mutant anther is defective in producing the majority of anther cells, including endothecium, middle layer, tapetum, and microsporocyte (Schiefthaler et al., 1999; Yang et al., 1999). Different from SPL/NZZ, genes encoding Leu-rich repeat receptor-like protein kinases directly regulate the differentiation of fewer anther cell types in Arabidopsis (Zhao, 2009). EXCESS MICROSPOROCYTES1/EXTRA SPOROGENOUS CELLS (EMS1/EXS) and SOMATIC EMBRYOGENESIS RECEPTOR-LIKE KINASE1/2 determine the tapetum (Canales et al., 2002; Zhao et al., 2002; Albrecht et al., 2005; Colcombet et al., 2005). BARELY ANY MERISTEM1/2 is required for the differentiation of anther somatic cell layers (Hord et al., 2006). In addition, RECEPTOR-LIKE PROTEIN KINASE2 specifies middle layer cells (Mizuno et al., 2007). TAPETUM DETERMINANT1 defines the tapetum by acting as a potential ligand of EMS1/EXS (Yang et al., 2003; Jia et al., 2008). Therefore, SPL/NZZ may control anther cell differentiation by regulating a subset of downstream genes necessary for anther establishment. On the other hand, in this study, our gain-of-function and loss-of-function analyses demonstrated that SPL/NZZ is involved in controlling stamen identity. Furthermore, we showed that the ectopic expression and overexpression of SPL/NZZ altered the expression of several key genes that are required for stamen identity. Besides the role played in early anther cell differentiation, our results suggest that the floral organ-building gene SPL/NZZ might regulate stamen identity by interacting with floral organ identity genes such as AG, SEP3, and AP2.
SPL/NZZ may be involved in controlling stamen identity through interacting with AG. The AG gene, which encodes a MADS box protein, is essential for specifying stamens and carpels as well as repressing stem cell proliferation in the center of the flower (Yanofsky et al., 1990; Lenhard et al., 2001; Lohmann et al., 2001). The AG transcription factor controls early stamen development by directly activating the expression of SPL/NZZ (Ito et al., 2004). In addition, AG is necessary for regulating anther dehiscence, a late event in stamen development, by directly activating the expression of DEFECTIVEINANTHERDEHISCENCE1, which encodes a catalytic enzyme involved in the biosynthesis of jasmonic acid (Ito et al., 2007). Therefore, AG not only controls early stamen development, including stamen identity and early anther cell differentiation, but also regulates late stamen development, such as anther dehiscence. Previous studies have shown that AG is ectopically expressed in two outer whorls in ap2 mutant flowers, while plants ectopically expressing AG form ap2-like flowers, which have stamen-like structures in the second whorl (Drews et al., 1991; Mizukami and Ma, 1992). Our results showed that both ectopic expression and overexpression of SPL/NZZ result in the increased expression of AG in rosette leaves and flowers. In particular, the ectopic expression of SPL/NZZ led to ectopic expression of AG in sepals and petals. Therefore, the ectopic expression of AG may cause the flower phenotypes in Pro35S:SPL/NZZ plants and enhance the stamen phenotype in the second whorl of ap2-1Pro35S:SPL/NZZ flowers. Although Pro35S:SPL/NZZ flowers produced abnormal sepals (Fig. 3A), we have not detected carpel-like structures in whorl 1 organs of Pro35S:SPL/NZZ and ap2-1Pro35S:SPL/NZZ flowers. Hence, SPL/NZZ seemed to have no carpel identity function. It is possible that the expression level of AG might not reach the threshold for promoting the formation of carpel-like structures in sepal-like organs of both Pro35S:SPL/NZZ and ap2-1Pro35S:SPL/NZZ flowers. The other reason could be that AG was not ectopically expressed in whorl 1. In ag-1 flowers, ectopic expression of SPL/NZZ is able to induce microsporogenesis in whorl 3 petal-like structures, which, however, lack normal anther structure and filament morphology (Ito et al., 2004). In this study, we did not detect narrow petals in the second whorl of ag-1Pro35S:SPL/NZZ flowers. Although the third whorl stamens in splag-4 double mutant flowers showed petal identity, the null SPL/NZZ mutants appeared normal in stamen identity. Thus, SPL/NZZ should not play an equal role to AG in specifying stamen identity. The expression of SPL/NZZ coincides with the expression of AG during early anther development, when anther cell division and differentiation actively occur (Yanofsky et al., 1990; Bowman et al., 1991a; Drews et al., 1991; Ito et al., 2004; Xing and Zachgo, 2008). After the anther structure is established, AG is expressed in anther connective tissues and anther walls (Bowman et al., 1991a; Ito et al., 2004). Therefore, SPL/NZZ may contribute to maintaining the expression of AG during anther development.
The spatial and temporal specificity of AG expression is achieved by both positive and negative regulators. The transcription factor LEAFY (LFY) is a key positive regulator of AG, which binds to cis-elements in an unusually large intron of AG to activate its expression (Schultz and Haughn, 1991; Weigel et al., 1992; Parcy et al., 1998; Busch et al., 1999). In addition, the homeodomain transcription factor WUSCHEL activates the expression of AG in the center of developing flowers via binding to the same regulatory region as LFY (Mayer et al., 1998; Lenhard et al., 2001; Lohmann et al., 2001). Also, several other positive regulators of AG have been identified with enhancer screenings. The ag-4 enhancers HUA1 and HUA2, as well as HUAENHANCER4, are involved in facilitating the processing of AG pre-mRNA, particularly splicing the large intron (Chen and Meyerowitz, 1999; Li et al., 2001; Cheng et al., 2003). The expression of AG is decreased after stage 6 in hen2-1 hua1-1 hua2-1 flowers, suggesting that HEN2 is important for maintaining the expression of AG (Western et al., 2002). Recent studies found that the bZIP transcription factor PERIANTHIA directly activates the expression of AG (Das et al., 2009; Maier et al., 2009).
AG expression is also negatively regulated by repressors. AP2 represses the expression of AG in outer whorls, possibly by binding to the AG large intron (Drews et al., 1991; Mizukami and Ma, 1992; Jofuku et al., 1994; Sieburth and Meyerowitz, 1997; Bomblies et al., 1999). Moreover, microRNA172 acts as a negative regulator of AP2 (Aukerman and Sakai, 2003; Chen, 2004; Zhao et al., 2007). LUG and SEU serve as corepressors to form a transcriptional repressor complex of AG together with DNA-binding transcription factors, including BELLRINGER, AP1, SEP3, and AGAMOUS-LIKE 24 (Liu and Meyerowitz, 1995; Conner and Liu, 2000; Bao et al., 2004; Sridhar et al., 2004, 2006; Franks et al., 2006; Gregis et al., 2006). Furthermore, polycomb group proteins CLF and EMBRYONIC FLOWER2, as well as the plant-specific protein EMF1, repress the expression of AG epigenetically (Goodrich et al., 1997; Sieburth and Meyerowitz, 1997; Aubert et al., 2001; Yoshida et al., 2001; Calonje et al., 2008).
Our results suggest that SPL/NZZ induces the expression of AG via SEP3. Both ectopic expression and overexpression of SPL/NZZ caused the increased expression of AG. Furthermore, ectopic expression of SPL/NZZ could directly induce the expression of SEP3. Previous studies showed that the ectopic expression of SEP3 is sufficient to ectopically activate AG (Castillejo et al., 2005). Depending on regulatory conditions, SEP3 may play a positive or negative role in regulating the expression of AG (Sridhar et al., 2006). Very recently, studies using the ChIP-Seq technique revealed that the SEP3 binding site is located in the second intron of AG (Kaufmann et al., 2009). AG controls early stamen development by directly activating the expression of SPL/NZZ (Ito et al., 2004). Our results suggest that the positive feed-forward regulation between AG and SPL/NZZ plays an important role in stamen identity specification and stamen morphogenesis.
It is worthwhile to point out that ectopic expression and overexpression of SPL/NZZ led to the decreased expression of AP2. AG prevents the expression of AP1 in whorls three and four of wild-type flowers (Gustafson-Brown et al., 1994). However, no report has shown that AG represses AP2 (Jofuku et al., 1994; Chen, 2004). In spl/nzz anthers, the expression of AP2 is increased 5-fold, suggesting that SPL/NZZ may repress the expression of AP2 in the third whorl (Wijeratne et al., 2007). Therefore, it might be possible that SPL/NZZ plays a direct role in negatively regulating the expression of AP2. Further studies of interactions among SPL/NZZ, AG, SEP3, and AP2 as well as identifying the target genes of SPL/NZZ should lead to a better understanding of anther development.
MATERIALS AND METHODS
Plant Materials and Growth Conditions
All Arabidopsis (Arabidopsis thaliana) plants are in the Landsberg erecta (Ler) background, except for the spl-D mutant, which is in the Columbia (Col-0) ecotype. To construct the splag-4 double mutant, pollen from the ag-4 mutant was used to pollinate spl heterozygous plants. In the F2 generation, plants exhibiting the ag-4 flower phenotype were genotyped for the spl mutant by PCR (Supplemental Table S1; Yang et al., 1999; Zhao et al., 2002). The Pro35S:SPL/NZZ-GR line was generated in the Meyerowitz Laboratory (Ito et al., 2004). Plants were grown on Metro-Mix 360 soil at 22°C under a 16-h-light/8-h-dark cycle.
Vector Construction and Plant Transformation
The cDNA of SPL/NZZ was amplified by Phusion High-fidelity DNA polymerase (Supplemental Table S1; New England Biolabs) and then was cloned into the pENTR TOPO vector (Invitrogen). After verification by sequencing, the cDNA fragment was introduced into the Gateway binary vector (a gift from Dr. T. Nakagawa, Shimane University) by an LR recombination reaction using Gateway LR Clonase II enzyme mix (Invitrogen). The resulting construct was introduced into Agrobacterium tumefaciens strain GV3101. Wild-type, ap2-1 mutant, and ag-1 heterozygous plants were transformed. T0 seeds were screened for transformants on half-strength Murashige and Skoog agar plates containing 50 mg L−1 kanamycin and 50 mg L−1 hygromycin (Clough and Bent, 1998). The Pro35S:AG construct was kindly provided by Dr. Hong Ma (Penn State University).
DEX Treatment
The Pro35S:SPL/NZZ-GR T3 seedlings were screened on half-strength Murashige and Skoog plates containing 10 μg mL−1 phosphinothricin. The phosphinothricin-resistant seedlings were transferred to soil. Seedlings were then treated with a DEX solution containing 10 μm DEX and 0.015% Silwet L-77 and a mock solution (0.015% Silwet L-77 and the same concentration of ethanol used for dissolving DEX; Wagner et al., 1999; Ito et al., 2004). Rosette leaves were collected for total RNA extraction after 6 h, 12 h, 3 d, and 6 d. Inflorescences of nzz-2 Pro35S:SPL/NZZ-GR and ag-1Pro35S:SPL/NZZ-GR plants were treated similarly for 4 h.
Phenotype Analyses and Microscopy
Micrographs were taken with an Olympus DP70 digital camera through a stereomicroscope (Olympus SZX-RFL). Samples for SEM were fixed, dried, dissected, and coated as described previously (Bowman et al., 1989; Zhao et al., 2001b). Specimens were then examined using a Hitachi S-570 scanning electron microscope.
RT-PCR and Quantitative Real-Time RT-PCR
Two-week-old rosette leaves and young buds from Ler, Col-0, Pro35S:SPL/NZZ, spl-D, and ag-1Pro35S:SPL/NZZ-GR plants were collected. To examine gene expression in sepals and petals, about 500 Ler and Pro35S:SPL/NZZ young buds were dissected, and then sepals and petals were collected using a dissection microscope (Olympus SZ51). Total RNAs were extracted from different plant tissues using the RNeasy Plant Mini Kit (Qiagen). RNA concentrations were measured with a NanoDrop ND-1000 spectrophotometer. RT reactions were carried out using the QuantiTect Reverse Transcription Kit (Qiagen).
Primers for regular RT-PCR are listed in Supplemental Table S1. PCR cycles varied with expression levels for examined genes: for ACTIN2, 21 cycles in rosette leaves, flowers, sepals, and petals; for SPL/NZZ, 28 cycles in rosette leaves, flowers, sepals, and petals; for AG, 33 cycles in leaves, sepals, and petals and 29 cycles in flowers; for CLF, 33 cycles in leaves; for AP1, 28 cycles in flowers and 26 cycles in sepals and petals; for AP2, 28 cycles in flowers, sepals, and petals; for AP3, 26 cycles in flowers and 30 cycles in sepals and petals; for SEP3, 34 cycles in leaves and 28 cycles in flowers, sepals, and petals.
Quantitative real-time PCR was performed with a DNA Engine Opticon 2 system (Bio-Rad) using Fast SYBR Green PCR Master Mix (Applied Biosystems). The ACTIN2 gene was used as a control. The quantitative RT-PCR results were analyzed as described previously (Pfaffl et al., 2002). Three independent experiments were repeated. Each value indicates the average and se.
Supplemental Data
The following materials are available in the online version of this article.
Supplemental Figure S1. Leaf phenotypes.
Supplemental Figure S2. Semiquantitative RT-PCR.
Supplemental Table S1. Primer list.
ACKNOWLEDGMENTS
We thank S. Forst, D. Heathcote, and C. Starrett for technical assistance and critical comments on the manuscript, Y. Wang for helping with SEM, T. Schuck for plant care, and T. Nakagawa for providing Gateway binary vectors. We also thank E.M. Meyerowitz for providing the Pro35S:SPL/NZZ-GR line, H. Ma for providing the Pro35S:AG construct, and L. Qu for providing the spl-D mutant.
LITERATURE CITED
Albrecht C, Russinova E, Hecht V, Baaijens E, de Vries S (
Alves-Ferreira M, Wellmer F, Banhara A, Kumar V, Riechmann JL, Meyerowitz EM (
Aubert D, Chen L, Moon YH, Martin D, Castle LA, Yang CH, Sung ZR (
Aukerman MJ, Sakai H (
Bao X, Franks RG, Levin JZ, Liu Z (
Bomblies K, Dagenais N, Weigel D (
Borg M, Brownfield L, Twell D (
Bowman JL, Drews GN, Meyerowitz EM (
Bowman JL, Smyth DR, Meyerowitz EM (
Bowman JL, Smyth DR, Meyerowitz EM (
Busch MA, Bomblies K, Weigel D (
Calonje M, Sanchez R, Chen L, Sung ZR (
Canales C, Bhatt AM, Scott R, Dickinson H (
Castillejo C, Romera-Branchat M, Pelaz S (
Chen X (
Chen X, Meyerowitz EM (
Cheng Y, Kato N, Wang W, Li J, Chen X (
Clough SJ, Bent AF (
Coen ES, Meyerowitz EM (
Colcombet J, Boisson-Dernier A, Ros-Palau R, Vera CE, Schroeder JI (
Conner J, Liu Z (
Das P, Ito T, Wellmer F, Vernoux T, Dedieu A, Traas J, Meyerowitz EM (
Ditta G, Pinyopich A, Robles P, Pelaz S, Yanofsky MF (
Drews GN, Bowman JL, Meyerowitz EM (
Feng X, Dickinson HG (
Franks RG, Liu Z, Fischer RL (
Goldberg RB, Beals TP, Sanders PM (
Goodrich J, Puangsomlee P, Martin M, Long D, Meyerowitz EM, Coupland G (
Gregis V, Sessa A, Colombo L, Kater MM (
Gustafson-Brown C, Savidge B, Yanofsky MF (
Haughn GW, Somerville C (
Hennig L, Gruissem W, Grossniklaus U, Kohler C (
Honma T, Goto K (
Hord CL, Chen C, Deyoung BJ, Clark SE, Ma H (
Ito T, Ng KH, Lim TS, Yu H, Meyerowitz EM (
Ito T, Wellmer F, Yu H, Das P, Ito N, Alves-Ferreira M, Riechmann JL, Meyerowitz EM (
Jack T (
Jia G, Liu X, Owen HA, Zhao D (
Jofuku KD, den Boer BG, Van Montagu M, Okamuro JK (
Kaufmann K, Muino JM, Jauregui R, Airoldi CA, Smaczniak C, Krajewski P, Angenent GC (
Lenhard M, Bohnert A, Jurgens G, Laux T (
Li J, Jia D, Chen X (
Li LC, Qin GJ, Tsuge T, Hou XH, Ding MY, Aoyama T, Oka A, Chen Z, Gu H, Zhao Y, et al (
Liu Z, Meyerowitz EM (
Lohmann JU, Hong RL, Hobe M, Busch MA, Parcy F, Simon R, Weigel D (
Lu XC, Gong HQ, Huang ML, Bai SL, He YB, Mao X, Geng Z, Li SG, Wei L, Yuwen JS, et al (
Ma H (
Ma H (
Ma J, Duncan D, Morrow DJ, Fernandes J, Walbot V (
Ma J, Skibbe DS, Fernandes J, Walbot V (
Maier AT, Stehling-Sun S, Wollmann H, Demar M, Hong RL, Haubeiss S, Weigel D, Lohmann JU (
Mayer KF, Schoof H, Haecker A, Lenhard M, Jurgens G, Laux T (
McCormick S (
Melzer R, Verelst W, Theissen G (
Meyerowitz EM, Bowman JL, Brockman LL, Drews GN, Jack T, Sieburth LE, Weigel D (
Mizukami Y, Ma H (
Mizuno S, Osakabe Y, Maruyama K, Ito T, Osakabe K, Sato T, Shinozaki K, Yamaguchi-Shinozaki K (
Parcy F, Nilsson O, Busch MA, Lee I, Weigel D (
Pelaz S, Ditta GS, Baumann E, Wisman E, Yanofsky MF (
Pfaffl MW, Horgan GW, Dempfle L (
Sanders PM, Bui AQ, Weterings K, McIntire KN, Hsu YC, Lee PY, Truong MT, Beals TP, Goldberg RB (
Schiefthaler U, Balasubramanian S, Sieber P, Chevalier D, Wisman E, Schneitz K (
Schultz EA, Haughn GW (
Scott RJ, Spielman M, Dickinson HG (
Sieburth LE, Meyerowitz EM (
Sieburth LE, Running MP, Meyerowitz EM (
Sridhar VV, Surendrarao A, Gonzalez D, Conlan RS, Liu Z (
Sridhar VV, Surendrarao A, Liu Z (
Wagner D, Sablowski RW, Meyerowitz EM (
Walbot V, Evans MM (
Weigel D, Alvarez J, Smyth DR, Yanofsky MF, Meyerowitz EM (
Weigel D, Meyerowitz EM (
Wellmer F, Riechmann JL, Alves-Ferreira M, Meyerowitz EM (
Western TL, Cheng Y, Liu J, Chen X (
Wijeratne AJ, Zhang W, Sun Y, Liu W, Albert R, Zheng Z, Oppenheimer DG, Zhao D, Ma H (
Wilson ZA, Zhang DB (
Xing S, Zachgo S (
Yang SL, Xie LF, Mao HZ, Puah CS, Yang WC, Jiang L, Sundaresan V, Ye D (
Yang WC, Ye D, Xu J, Sundaresan V (
Yanofsky MF, Ma H, Bowman JL, Drews GN, Feldmann KA, Meyerowitz EM (
Yoshida N, Yanai Y, Chen L, Kato Y, Hiratsuka J, Miwa T, Sung ZR, Takahashi S (
Zhao D (
Zhao D, Wang GF, Speal B, Ma H (
Zhao D, Yu Q, Chen C, Ma H (
Zhao D, Yu Q, Chen M, Ma H (
Zhao L, Kim Y, Dinh TT, Chen X (
Zik M, Irish VF (
Author notes
This work was supported by the National Science Foundation (grant no. IOS–0721192 to D.Z.), the Research Growth Initiative Program at the University of Wisconsin-Milwaukee (to D.Z.), the Shaw Scientist Award from the Greater Milwaukee Foundation (to D.Z.), and the American Society of Plant Biologists Summer Undergraduate Research Fellowship (to A.R.).
Present address: Department of Biochemistry, University of Alberta, Edmonton, Alberta, Canada T6G 2H7.
Present address: Department of Genetics, University of Wisconsin, Madison, WI 53706–1574.
Corresponding author; e-mail [email protected].
The author responsible for distribution of materials integral to the findings presented in this article in accordance with the policy described in the Instructions for Authors (www.plantphysiol.org) is: Dazhong Zhao ([email protected]).
The online version of this article contains Web-only data.
Open Access articles can be viewed online without a subscription.