-
PDF
- Split View
-
Views
-
Cite
Cite
Lijun Wu, Zhijin Zhang, Haiwen Zhang, Xue-Chen Wang, Rongfeng Huang, Transcriptional Modulation of Ethylene Response Factor Protein JERF3 in the Oxidative Stress Response Enhances Tolerance of Tobacco Seedlings to Salt, Drought, and Freezing , Plant Physiology, Volume 148, Issue 4, December 2008, Pages 1953–1963, https://doi.org/10.1104/pp.108.126813
- Share Icon Share
Abstract
Abiotic stresses such as drought, cold, and salinity affect normal growth and development in plants. The production and accumulation of reactive oxygen species (ROS) cause oxidative stress under these abiotic conditions. Recent research has elucidated the significant role of ethylene response factor (ERF) proteins in plant adaptation to abiotic stresses. Our earlier functional analysis of an ERF protein, JERF3, indicated that JERF3-expressing tobacco (Nicotiana tabacum) adapts better to salinity in vitro. This article extends that study by showing that transcriptional regulation of JERF3 in the oxidative stress response modulates the increased tolerance to abiotic stresses. First, we confirm that JERF3-expressing tobacco enhances adaptation to drought, freezing, and osmotic stress during germination and seedling development. Then we demonstrate that JERF3-expressing tobacco imparts not only higher expression of osmotic stress genes compared to wild-type tobacco, but also the activation of photosynthetic carbon assimilation/metabolism and oxidative genes. More importantly, this regulation of the expression of oxidative genes subsequently enhances the activities of superoxide dismutase but reduces the content of ROS in tobacco under drought, cold, salt, and abscisic acid treatments. This indicates that JERF3 also modulates the abiotic stress response via the regulation of the oxidative stress response. Further assays indicate that JERF3 activates the expression of reporter genes driven by the osmotic-responsive GCC box, DRE, and CE1 and by oxidative-responsive as-1 in transient assays, suggesting the transcriptional activation of JERF3 in the expression of genes involved in response to oxidative and osmotic stress. Our results therefore establish that JERF3 activates the expression of such genes through transcription, resulting in decreased accumulation of ROS and, in turn, enhanced adaptation to drought, freezing, and salt in tobacco.
Regulation of transcription has proved to be a vital aspect of the complex genetic and biochemical networks involved in plant responses to stresses. Understanding the interaction between stress-responsive transcription factors and their corresponding cis-acting elements in promoters of the downstream target genes is a prerequisite to dissecting the transcriptional regulatory network. For instance, investigation of the promoters of COR genes in Arabidopsis (Arabidopsis thaliana) indicated that they are regulated in an abscisic acid (ABA)-dependent or ABA-independent pathway under conditions of cold, dehydration, and high salinity (Liu et al., 1998; Yamaguchi-Shinozaki and Shinozaki, 2006). The subfamily of ethylene response factor (ERF) proteins belongs to the ERF/APETALA2 (AP2) superfamily, which contains one highly conserved AP2 or ERF DNA-binding domain (Kizis et al., 2001; Liu et al., 2006). ERF proteins were first isolated from tobacco (Nicotiana tabacum) as GCC-box binding proteins (Ohme-Takagi and Shinshi, 1995), and consequent investigations indicate that many ERF proteins found in other plants enhance resistance to pathogens by activating the expression of pathogenesis-related genes (Guo et al., 2002; Zhang et al., 2004). Evidence accumulated over the years has proved that ERF proteins bind not only to the GCC box but also to DRE/CRT, CE1, JERE, and CT-rich elements (Liu et al., 1998; Menke et al., 1999; Niu et al., 2002; Xue and Loveridge, 2004; Tang et al., 2005), thereby enhancing plant tolerance to multiple stresses, which suggests that ERF proteins are part of the plant's response to such stresses.
Accumulated evidence indicates that stress-stimulated physiological imbalance increases the level of reactive oxygen species (ROS) in plant cells. The steady-state levels of hydrogen peroxide (H2O2), singlet oxygen, the superoxide anion (O2 −), and the hydroxyl radical depend on the balance between generation and removal, which is facilitated by the ROS-scavenging system of the cell (Bartoli et al., 2004; Gechev et al., 2006). In such a system, H2O2 plays a very important role in mediating signal transduction in response to stress in plant cells. H2O2 diffuses rapidly from its site of synthesis within subcellular microdomains, depending on its concentration, and can transmit intracellular signals to the nucleus by oxidizing various upstream components of the signaling pathway, including transcription factors that ultimately result in changes in gene expression (Mittler, 2002; Gadjev et al., 2006). Further, the expression of the ERF gene CaPF1 in Virginia pine (Pinus virginiana) enhances the level of antioxidant enzyme activities of ascorbate peroxidase, glutathione reductase, and superoxide dismutase (SOD). This results in the improved tolerance to stress (Tang et al., 2005), indicating that ERF proteins might be associated with the regulation of ROS pathway. Although many ERF proteins have been identified in the response of plants to abiotic stress, the details of involvement of an ROS-mediated regulatory module are yet to be established. An ERF protein, JERF3, was isolated from tomato (Solanum lycopersicum) using a yeast one-hybrid screen in our laboratory. It has been shown that JERF3 could be induced by ethylene, jasmonic acid, cold, salinity, and ABA, and that JERF3-expressing tobacco was better adapted to salinity in vitro (Wang et al., 2004); however, the molecular mechanism of that regulatory response remained to be elucidated. In this article, we now report that JERF3 transcriptionally regulates the expression of genes involved in plant responses to osmotic- and oxidative-related stresses; this results in decreased accumulation of ROS, thereby enhancing the adaptation to drought, salt, and freezing in tobacco.
RESULTS
Expression of JERF3 in Tobacco Increases Seed Germination and Root Elongation under Salt and Osmotic Stress, and Tolerance to Drought and Freezing in Seedling Stage
![Expression of JERF3 increases germination and root elongation in tobacco under salt and osmotic stress. A, Germination of tobacco seeds under 0.15 m NaCl. B, Germination of tobacco seeds under 0.2 mm mannitol. C, Elongation of tobacco roots under 0.15 m NaCl or 0.2 mm mannitol. About 80 seeds and 30 seedlings were used for each treatment. Results are the average of three replicates. Error bars indicate ±sd. [See online article for color version of this figure.]](https://oup.silverchair-cdn.com/oup/backfile/Content_public/Journal/plphys/148/4/10.1104_pp.108.126813/2/m_plphys_v148_4_1953_f1.jpeg?Expires=1750419202&Signature=iPm~-tq5M8VjEd5yviKTyh6ZP8ladIuI7WwNf6Xcjr3O-zyfHyqgKIjP1LREfPQUFty24uY~QjCd1y-rcyBuxERj1IS0AgG-VbxmZes9u0dvT1gz45qphjg65oeOr4-zFAMrONP7mRgizgHl~19~oUT6kM0uWrsCPNHXcijODHQVOzeiSekq4bj0T2AMLd7H0elCE7eVWSAjxFdVsisyZPzV1oKmTsQeAZZoTtY7KqIDbAO2R0XqpHqgM0rzGhHpwJZ0PCb~46bMYUurfyUkXuhwMAT2Y9HL8j3aZ8DwBP-pDpL91vKPuekZ7N2sHgMoPf~eKuecbxoLbOC1uCMRQQ__&Key-Pair-Id=APKAIE5G5CRDK6RD3PGA)
Expression of JERF3 increases germination and root elongation in tobacco under salt and osmotic stress. A, Germination of tobacco seeds under 0.15 m NaCl. B, Germination of tobacco seeds under 0.2 mm mannitol. C, Elongation of tobacco roots under 0.15 m NaCl or 0.2 mm mannitol. About 80 seeds and 30 seedlings were used for each treatment. Results are the average of three replicates. Error bars indicate ±sd. [See online article for color version of this figure.]
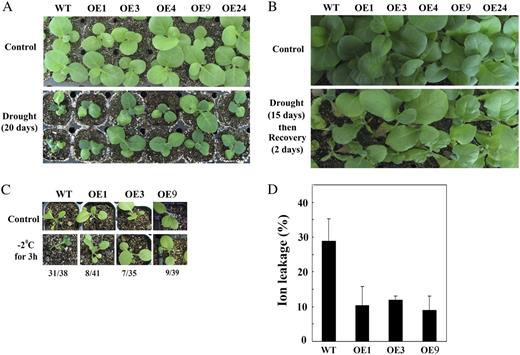
Expression of JERF3 enhances tolerance to drought and freezing in tobacco. A and B, JERF3 enhances the adaptation of tobacco to drought during 3- and 6-week-old seedlings, respectively. About 30 seedlings in triplicate were used for each line. C, Seedlings exposed to freezing (−2°C for 3 h). D, Ion leakage under −2°C for 3 h. Four-week-old seedlings were used for this assay. About 30 seedlings were used for each line. Results are the average of three replicates. Error bars indicate ±sd.
After 0.15 m NaCl treatment for 14 d, the seedling roots in the five independent OE lines were about twice as long than those in wild type (Fig. 1C). Interestingly, when subjected to higher concentrations of 0.2 mm mannitol for the same duration, roots of five independent OE lines were not only about 34% longer than those of wild type but also showed more lateral roots (Fig. 1C). Student's t tests indicate that the above data of seed germination or root elongation are not significantly different among the five independent OE lines at 95% probability, indicating that the distinctive transcript level of JERF3 (Supplemental Fig. S1A) did not correlate with the degree of tolerance in OE lines. However, there are differences (significant at 95% probability) in the seed germination or root elongation between OE lines and wild type. These results indicate that JERF3 increases the adaptation to salt and osmotic stress during seed germination and root elongation in tobacco.
Next we tested the response of wild type and the above five independent OE seedlings to drought at different stages. We found that after 3-week-old seedlings were not watered for 20 d, more than 85% of wild-type seedlings had wilted whereas growth was almost normal in the five independent OE seedlings (Fig. 2A). Interestingly, when 6-week-old seedlings were exposed to drought for 15 d, the phenotypes of wilted leaves did not show obvious differences between wild type and five independent OE seedlings. However, after rewatering for another 2 d, more than 80% of wild-type seedlings did not recover from the wilted phenotype whereas growth was back to normal in the five independent OE seedlings (Fig. 2B), indicating that JERF3 increases tolerance to drought in tobacco seedlings.
We also examined the response of wild type and three one-copy insertion OE seedlings to freezing. Initially, we exposed seedlings to −10°C in the growth chamber but found no clear difference between OE lines and wild type. We then set the temperature to −2°C. As can be seen in Figure 2C, wild-type seedlings were injured after a 3-h exposure to −2°C but OE seedlings were not obviously affected. More interestingly, leakage of ions from wild-type seedlings after the treatment was 30% but that from OE seedlings was only 10% (Fig. 2D), which is consistent with the report that plasma membranes of plants are injured because of exposure to cold, resulting in leakage of ions from the cytoplasm (Gonzalez-Aguilar et al., 2000). These results suggest that JERF3 makes tobacco seedlings more tolerant to freezing by increasing the stability of the plasma membrane.
Expression of JERF3 in Tobacco Significantly Activates the Expression of Osmotic- and Oxidative-Related Genes
To investigate how JERF3 modulates plant response to drought, cold, and salt stresses, we compared the expression profiles in two independent OE seedlings with that in wild-type plants using cDNA-amplified fragment length polymorphism (AFLP; Vos et al., 1995). Using 240 primer pairs, we obtained 2,742 fragments, of which 526 were differentially expressed between OE lines and wild type (changes more than 2-fold). Compared to the transcript levels in wild type, there were 277 up-regulated genes and 249 down-regulated genes in OE lines, and approximately 23% of the up-regulated genes are believed to be involved in response to osmotic, photosynthetic carbon assimilation/metabolism, and oxidative stress.

Expression of JERF3 in tobacco enhances the expression of osmotic- and oxidative stress-related genes derived from the analysis of cDNA-AFLP under normal growth conditions. A, Confirmation of the expression of genes responsive to osmotic stress in tobacco. B, Confirmation of expression of genes related to photosynthetic carbon assimilation/metabolism. C, Confirmation of expression of genes related to oxidative stress. Transcript levels of these genes in transgenic tobacco are indicated relative to the level of wild-type tobacco taken as 100, referring to the transcripts of actin in the same samples. Error bars are based on three independent experiments.
Next we analyzed the expression of four genes involved in photosynthetic carbon assimilation/metabolism in OE seedling. It is established that NtRub-SS encodes Rubisco small subunit (Mazur and Chui 1985); ChlGaPA encodes glyceraldehyde-3-P dehydrogenase A-subunit precursor; CyGAP encodes glyceraldehyde-3-P dehydrogenase; and NtRCA342 encodes Rubisco activase precursor (Shih et al., 1986). Our results showed that the expression of these genes except ChlGaPA was increased 3 to approximately 6 times that in wild type (Fig. 3B), indicating that JERF3 confers the expression of genes in the response to photosynthetic carbon assimilation/metabolism in tobacco.
Also we analyzed the expression of seven genes involved in the response to oxidative stress in OE seedling. It has been reported that NtCA encodes carbonic anhydrase, which displays antioxidant activity and functions in the hypersensitive defense response (Slaymaker et al., 2002); NtSOD encodes SOD, which was reported to enhance oxidative stress tolerance in tobacco (Slooten et al., 1995); NtRbohD, a tobacco respiratory burst oxidase homolog, encodes an enzyme similar to the mammalian NADPH oxidase, producing active oxygen species in elicited tobacco cells (Simon-Plas et al., 2002; Morel et al., 2004). And the genes NtCAT1, NtAPX1, NtAPX2, and NtGPX encode catalase, cytosolic ascorbate peroxidase, chloroplastic ascorbate peroxidase, and glutathione peroxidase, respectively, which all are considered to use H2O2 as an electron acceptor to catalyze a number of oxidative reactions (Orvar and Ellis, 1995; Pasqualini et al., 2007). Our results confirmed, with the exception of NtCAT1 and NtRbohD, that the expression of these up-regulated genes was increased 3 to approximately 8 times above controls (Fig. 3C). Moreover, the expression of down-regulated genes was consistent with the results of cDNA-AFLP (data not shown). Therefore JERF3 regulates the expression of photosynthetic carbon assimilation/metabolism and oxidative genes as well.
Expression of JERF3 in Tomato Is Responsive to Methyl Viologen But Not H2O2 and 3-Amintriazole
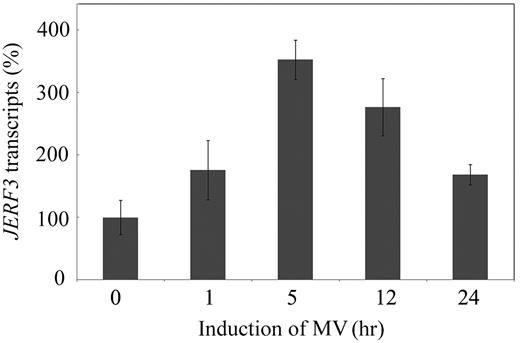
Expression of JERF3 in tomato is inducible by MV. The expression of JERF3 in tomato was detected by qPCR. The actin transcripts were used as internal control, and the expression level of JERF3 was standardized as 100 at time 0. The assay was repeated three times. The bars represent ±se.
Expression of JERF3 in Tobacco Increases the Activities of SOD But Decreases the Accumulation of ROS
The data so far indicate that plants accumulate osmotic solutes to regulate osmotic potential under drought, salt, and cold stress (Gilmour et al., 2000). To test whether the expression of JERF3 modulates the accumulation of osmotic solutes under drought, salt, and cold stresses, we checked the Pro content of wild-type and OE seedlings. As anticipated, the contents of osmotic solutes in OE seedlings were approximately 9% higher than those in wild-type seedlings under normal growth conditions, but the differences were not clearly correlated to the stresses of drought, salt, or cold (data not shown). This might suggest that osmotic regulation is not the exclusive regulatory pathway of JERF3 in adaptation to drought, salt, and cold in tobacco. In this case, there must be an alternative regulatory pathway involving JERF3 in the plant's response to abiotic stress.
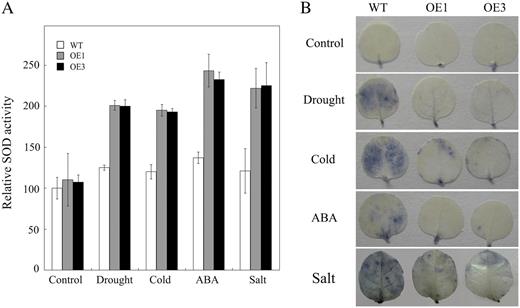
Expression of JERF3 in tobacco enhances the activity of SOD and decreases the accumulation of ROS. A, Expression of JERF3 in tobacco enhances the activities of SOD. The SOD activities were measured based on the ability of SOD to inhibit the reduction of NBT by superoxide. And the data are shown compared to the wild type of control (taken as 100) after calculation of the SOD activities each sample. Results are the average of three replicates. B, Expression of JERF3 in tobacco decreases the accumulation of ROS. The contents of O2 − in tobacco leaves were stained using NBT as substrate. Approximately 15 seedlings in triplicates were used for each line in this assay. Control indicates seedlings raised under normal conditions of growth, Drought indicates seedlings kept on tissue paper for 1 h, Cold represents seedlings exposed to 4°C for 2 d, and ABA and Salt indicate seedlings sprayed with ABA or NaCl, respectively.
Because H2O2, singlet oxygen, O2 −, and the hydroxyl radical all belong to ROS (Bartoli et al., 2004; Gechev et al., 2006), we then checked the accumulation of O2 − in OE and wild-type seedlings under dehydration, cold, salt, and ABA treatments. Under normal growth conditions, O2 − accumulated at very low levels and showed no obvious difference between wild-type and OE seedlings. This reduced ROS was not detectable in OE seedlings, while wild type still can be observed along the main vein under normal growth conditions (Fig. 5B). After exposure to dehydration, cold, salt, or ABA treatments, O2 − accumulated significantly greater quantities in wild-type plants whereas its levels in OE seedlings were lower (Fig. 5B), suggesting that JERF3 decreases the accumulation of ROS under dehydration, cold, salt, and ABA treatments.
JERF3 Transcriptionally Regulates Gene Expression through Interacting with Multiple Stress-Responsive cis-Acting Elements
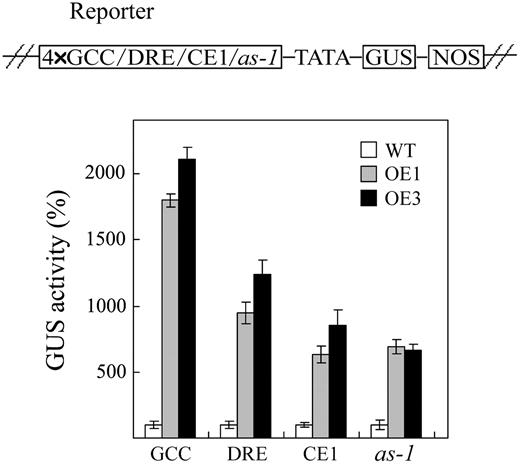
Expression of JERF3 in tobacco activates the expression of the GUS gene controlled by GCC box, DRE, CE1, or as-1. The top section displays a schematic diagram of the reporter constructs used in Agrobacterium-mediated transient expression assay. Four-times cis-elements (GCC box, DRE, CE1, or as-1) are fused upstream of 35S minimal promoter in pBI121 as reporter. The bottom section shows relative GUS activity in leaves of wild-type and OE seedlings 48 h after Agrobacterium infiltration. The data for GUS activity in OE seedling leaves are relative to that in wild type (standardized to 100, inferred to the GUS activity driven by 35S minimal promoter in pBI121). GCC, DRE, CE1, and as-1 indicate that 4× cis-elements of GCC box, DRE, CE1, and as-1 are inserted upstream of the 35S minimal promoter in pBI121 as positive reporters, respectively. Error bars indicate ±sd.
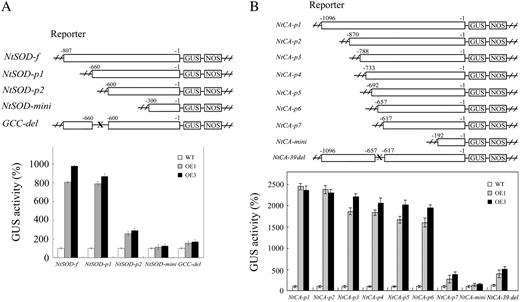
Expression of JERF3 in tobacco interacts with the promoters of NtSOD and NtCA in transient GUS assay. A and B, The top section displays a schematic diagram of the reporter constructs used in Agrobacterium-mediated transient expression assay. The promoter of NtSOD-f, NtSOD-p1, NtSOD-p2, NtSOD-mini, or removal of GCC box (GCC-del) is fused upstream of GUS gene in pCAMBIA1303. The promoter of NtCA-p1, NtCA-p2, NtCA-p3, NtCA-p4, NtCA-p5, NtCA-p6, NtCA-p7, NtCA-mini, or removal of 39-bp fragment (NtCA-39 del) is fused upstream of GUS gene in pCAMBIA1303. The chart shows relative GUS activity in leaves of wild-type and OE seedlings 48 h after Agrobacterium infiltration. The data for GUS activity in OE seedling leaves are relative to that in wild type (standardized to 100).
Interestingly, JERF3 also interacts with the full-length promoter (−1 to −1,096 bp) of NtCA (NtCA-p1). Concomitant with the deletion of the promoter, GUS activity weakened but not markedly until NtCA-p7 (−1 to −617 bp; Fig. 7B), suggesting that the deleted sequence between NtCA-p6 and NtCA-p7 might interact with JERF3. In fact, removal of the fragment in the promoter of NtCA (NtCA-39 del) significantly reduced the expression of the reporter gene (Fig. 7B). Therefore our results demonstrate that JERF3 might interact with a novel sequence of 39 bp (−617 to −657 bp, ATCTGTGATCAGCAATAATTGTTGAGTTGATTTGGAATT) located on the promoter of NtCA to activate gene expression in this manner.
DISCUSSION
It has been documented that the stress-induced increase of ROS in plant cells results from an imbalance between generation and removal. The production and accumulation of ROS cause oxidative stress under these abiotic stresses. Our earlier experiments (Wang et al., 2004) and this research with JERF3 have indicated that transcriptional regulation of JERF3 modulates the increased tolerance to drought, salt, and freezing in tobacco during germination and seedling development. Such regulation is not only through activating the expression of osmotic stress genes, but also through the activation of photosynthetic carbon assimilation/metabolism and oxidative genes. This establishes that the ERF protein JERF3 is involved in a ROS-mediated regulatory module in transcriptional networks that govern plant response to stress.
Chloroplast and mitochondria are the major sites of generation of ROS (Asada, 2006; Wormuth et al., 2007). The generation and removal of ROS is a homeostatic process during plant growth and development under normal conditions. However, this homeostasis is destroyed when plants are exposed to drought, cold, and salinity (Apel and Hirt, 2004; Bartoli et al., 2004). Cellular antioxidants, including enzymatic and nonenzymatic antioxidants, are the key to protecting the cells against damage due to ROS. Among enzymatic antioxidants, SOD playxs a pivotal role in clearing away ROS by catalyzing the highly damaging O2 − into H2O2 (Apel and Hirt, 2004). The results we obtained are consistent with the view that expressing JERF3 in tobacco greatly increases the activities of SOD, resulting in the reduced accumulation of O2 − under drought, cold, salt, and ABA treatments. To better understand the involvement of JERF3 in ROS transcriptional regulatory network, we found that the expression of JERF3 in its original tomato seedlings was inducible by the ROS-generating agent MV, suggesting that JERF3 might be associated with ROS responses. The enhanced expression of glutathione peroxidase NtGPX and cytosolic and chloroplastic ascorbate peroxidases (NtAPX1 and NtAPX2) in unstressed OE seedlings further supports the idea that JERF3 might transcriptionally regulate the expression of genes related to oxidative reactions. Furthermore, Rubisco, a key enzyme in photosynthesis, catalyzes ribulose-1,5-bisphosphate and assimilates CO2 and also catalyzes the oxygenase reaction of ribulose-1,5-bisphosphate into glycolate, which is metabolized in peroxisome (Ruuska et al., 2000; Marcus et al., 2005). This process helps scavenge ROS (Apel and Hirt, 2004). Carbonic anhydrase is important to CO2 transport because it catalyzes the reversible hydration of CO2 (Smith and Ferry, 2000) and has antioxidant activity (Slaymaker et al., 2002). In our earlier (Wang et al., 2004) and present research, we found that the ERF protein JERF3 significantly enhances tolerance to drought, freezing, and salinity in tobacco. Further research indicated that expression of photosynthetic carbon assimilation/metabolism and oxidative-related genes is up-regulated in JERF3-expressing tobacco, suggesting that JERF3 regulates photosynthesis and eliminates ROS. More importantly, we find that expressing JERF3 in tobacco activates the expression of NtSOD by interacting with the GCC box in the promoter of NtSOD, and NtCA via interaction with a 39-bp novel element, indicating that JERF3 regulates plant response to abiotic stresses mainly by modulating the expression of ROS-related genes.
After being detected by a receptor, ROS continues transferring the signal through two main pathways. In one, a group of transcription factors participating in different cellular pathways is activated by a mitogen-activated protein kinase cascade (Pitzschke and Hirt, 2006). In the other, the level of cellular calcium is changed after a receptor detects ROS and the pathway of calcium/calmodulin is activated (Mittler et al., 2004). For example, ROS may activate the Ca2+-permeable channels in plant membranes and induce stomatal closure by increasing the level of cellular calcium (Mori and Schroeder, 2004), which is further modulated by ABA (Mustilli et al., 2002; Apel and Hirt, 2004). The stability of the plasma membrane under cold conditions in JERF3-expressing tobacco indicates that regulation of JERF3 by oxidative stress might occur via a similar ROS-regulatory pathway. The involvement of JERF3 in ROS is also supported by the fact that JERF3 interacts with the ROS-responsive as-1 element in a transient assay, consistent with the report that a bZIP-type protein, which interacts with as1/ocs-like elements in the promoters of target genes, might be a component of the ROS-mediated pathway (Cheng et al., 2007). However, the predicted regulatory relationship between ROS-bZIP1 and the as1/ocs element-containing genes awaits further confirmation. Therefore, to our knowledge, it is a novel conclusion that the interaction of JERF3 with the promoters of genes related to oxidative stress regulates the expression of these genes. This results in enhanced SOD activities, consequently decreased accumulation of ROS and thereby enhancing tolerance to drought, salt, and freezing.
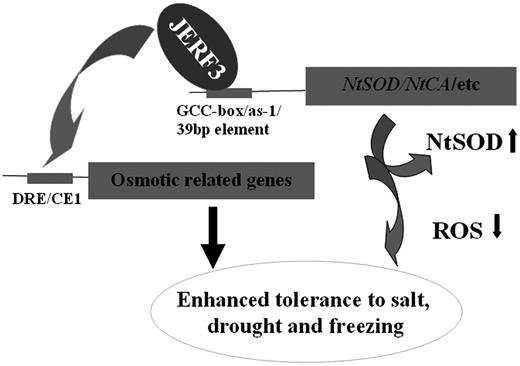
Transcriptional regulatory module of JERF3 in ROS-mediated stress responses.
MATERIALS AND METHODS
Plant Material and Growth Conditions
JERF3-expressing tobacco (Nicotiana tabacum; Wang et al., 2004) and wild-type tobacco (cv NC89) were grown in a growth chamber at 25°C under a 16-h photoperiod. T2 (OE4 and OE24 with two-copy insertions) and T3 (OE1, OE3, and OE9 with one-copy insertion) transgenic tobacco plants were used for the experiments. JERF3-expressing tobacco and wild-type tobacco are referred to as OE and wild type, respectively.
Salt, Drought, and Freezing Stress Assays
All seeds in the following assays were surface sterilized and kept at 4°C for 2 d to break dormancy (Mukhopadhyay et al., 2004) before subjecting them to any of the experimental treatments. For the germination assay, the tobacco seeds were plated in one-half Murashige and Skoog medium (0.6% agar) plus mannitol or NaCl, and the number of germinated seeds was counted every day. For root elongation assay, the germinated seeds were first placed upright in Murashige and Skoog plates until the roots were about 0.5 cm and the seedlings then transferred to the same medium supplemented with mannitol or NaCl and placed upright in the growth chamber. The root lengths were recorded before and after the treatments.
For drought assays, both 3-week-old and 6-week-old OE and wild-type seedlings in pots were adequately watered and then water was withheld for 15 to approximately 20 d. For freezing assays, 3-week-old OE lines and wild type in pots were placed in a growth chamber at −2°C for 3 h. For electrolyte leakage assay, seedlings of 3-week-old NC89 wild-type and OE seedlings were placed at −2°C in a growth chamber for 0, 2, 4, 8, and 12 h to determine electrolyte leakage as described by Guo et al. (2002). The percentage of electrolyte leakage was calculated as the ratio of the conductivity before autoclaving to that after autoclaving.
cDNA-AFLP Assay
Total RNA was extracted from unstressed 3-week-old tobacco plants using Trizol (Invitrogen) according to the manufacturer's recommendations. cDNA was synthesized from 1 μg of total RNA using 200 units of moloney murine leukemia virus (M-MLV) reverse transcriptase (Promega) following the manufacturer's instructions. For synthesis of the second strand, M-MLV RTase cDNA synthesis kit (TaKaRa) was used. cDNA-AFLP assay was performed as described by Vos et al. (1995).
Confirmation of Gene Expression Derived from cDNA-AFLP Using qPCR Amplifications
Total RNA was extracted from unstressed 3-week-old tobacco plants using Trizol (Invitrogen) according to the manufacturer's recommendations. cDNA from unstressed 3-week-old seedlings was synthesized from 1 μg of total RNA using 200 units of M-MLV reverse transcriptase (Promega) following the manufacturer's instructions. After reverse transcription, qPCRs were performed using an ABI Prism 7000 system (Applied Biosystems). The relative transcript abundance for some genes derived from cDNA-AFLP analysis is relative to the actin transcript levels measured in the same sample. The primers used in this article for qPCR are listed in Supplemental Table S1.
Inducible Expression of JERF3 in Tomato in Response to H2O2, MV, and 3-Amintriazole
Three-week-old tomato plants (Lycopersicon esculentum ‘Lichun’) were grown in growth chambers at 25°C with a 16-h light regime with illumination from cool-white fluorescent lights of about 150 μmol m−2 s−1. For the H2O2 and MV treatments, 100 μ m H2O2 or 50 μ m MV in 0.1% Tween 20 was used to spray tomato seedling leaves (Nishizawa et al., 2008). A total of 0.1% Tween 20 solution was used to spray tomato seedling leaves as control treatment. After treatments, tomato plants were used to detect expression of JERF3 using qPCR.
Detection of SOD Activities and O2 − in Tobacco Leaves
The stress treatments were applied as described below. For the drought treatment, OE and wild-type seedlings were removed from Murashige and Skoog medium, washed with water, dried with tissue paper, and then placed on tissue paper for 1 h. For the low-temperature treatment, OE and wild-type seedlings in plates were placed at 4°C in a growth chamber for 2 d. For the ABA and salt treatments, OE and wild-type seedlings in plates were sprayed with 100 μ m ABA or 200 mm NaCl and kept under high humidity for 3 h. Activity of SOD was determined using the method of Winterbourn et al. (1975) that is based on the ability of SOD to inhibit the reduction of nitro-blue tetrazolium (NBT) by superoxide. In our assays, 0.3 g leaves from 3-week-old tobacco seedlings each sample cultured in Murashige and Skoog medium were used. Each sample of the supernatants was placed in two 1.5-mL tubes, one was in the dark as control, another under light (150 μmol m−2 s−1). After incubation at 30°C for 20 min, the tubes were placed on ice to stop the reaction. The inhibition of NBT reduction was determined at A560. Leaves of 3-week-old seedlings treated with stresses were also used to determine superoxide using NBT staining as described by Lee et al. (2002).
TAIL-PCR Assay
TAIL-PCR was performed as described by Liu et al. (1995). The genomic DNA of NC89 was used as a template. AD primers were the same as those used by Liu et al. (1995). The sequences of specific primers for NtSOD, NtCA, and JERF3 were given in Supplemental Table S1.
Agrobacterium-Mediated GUS Transient Assay
For constructing the reporter vectors, four-times-repeated sequences of cis-acting elements GCC (AGCCGCC), DRE (TACCGACAT), CE1 (TGCCACCGG), or as-1 (TGACG) were inserted upstream of the minimal TATA box (−46 to +10) to replace the cauliflower mosaic virus 35S promoter in pBI121 (CLONTECH). The plasmids were then introduced into the Agrobacterium tumefaciens strain LBA4404. Agrobacterium-mediated transient assay was performed on the leaves of 4-week-old wild-type and OE seedlings as described by Yang et al. (2000). The GUS activity was measured about 48 h later.
To ascertain whether JERF3 interacts with the upstream sequence of the ATG start code of NtCA, DNA fragments 1,096 bp (NtCA-p1), 870 bp (NtCA-p2), 788 bp (NtCA-p3), 733 bp (NtCA-p4), 692 bp (NtCA-p5), 657 bp (NtCA-p6), 617 bp (NtCA-p7), and 192 bp (NtCA-mini as a minimal promoter) upstream of the ATG start code of NtCA were inserted separately into pCAMBIA1303 using the enzymes PstI and BglII.
Similarly, a DNA fragment 807 bp (NtSOD-f), 660 bp (NtSOD-p1), 600 bp (NtSOD-p2), and 300 bp (NtSOD-mini as a minimal promoter) upstream of the ATG start code of NtSOD was inserted separately into pCAMBIA1303 using the enzymes PstI and BglII. Sequences of the primers of NtCA and NtSOD promoters were listed in Supplemental Table S1. To generate the removal of GCC box or 39-bp fragment from NtSOD-f or NtCA-p1, respectively, PCR amplifications were used with special primers listed in Supplemental Table S1 using pCAMBIA1303-NtCA-p1 or pCAMBIA1303-NtSOD-f as a template. Then PCR production was self linked with T4 DNA ligase and was followed by transformation into Escherichia coli. After sequencing confirmation, the above-constructed plasmids were then introduced separately into the A. tumefaciens strain LBA4404. Agrobacterium-mediated transient assay was performed and the GUS activity measured about 48 h later.
The GenBank accession numbers for the sequences used as materials in this article are: AY383630 (JERF3), X63603 (actin), AF127243 (NtSAM1), D13952 (TOBLT), AB049337 (NtERD10C), AF194022 (NtSPS), X02353 (NtRub-SS), M14417 (ChlGaPA), M14419 (CyGAP), U35111 (NtRCA342), AB093097 (NtSOD), AF454759 (NtCA), AJ309006 (NtRbohD), U93244 (NtCAT1), AB041518 (NtGPX), AU15933 (NtAPX1), D85912 (NtAPX2), EU342357 (NtCA promoter), EU342358 (NtSOD promoter), AF328784 (LeEIL1), and EU910896 (JERF3 promoter).
Supplemental Data
The following materials are available in the online version of this article.
Supplemental Figure S1. Expression of JERF3 in tobacco has no effect on the growth at normal growth conditions.
Supplemental Figure S2. Expression of NtSPS in response to cold.
Supplemental Figure S3. LeEIL1 interacts with the promoter of JERF3 in transient expression assay.
Supplemental Table S1. The primers used in this article.
ACKNOWLEDGMENTS
The authors thank Professor Clive W. Lloyd and International Science Editing for improving the text.
LITERATURE CITED
Apel K, Hirt H (
Asada K (
Bartoli CG, Gomez F, Martinez DE, Guiamet JJ (
Bishopp A, Mahonen AP, Helariutta Y (
Börnke F (
Chao Q, Rothenberg M, Solano R, Roman G, Terzaghi W, Ecker JR (
Cheng C, Yun KY, Ressom HW, Mohanty B, Bajic VB, Jia Y, Yun SJ, Reyes BG (
Despres C, Chubak C, Rochon A, Clark R, Bethune T, Desveaux D, Fobert PR (
Espartero J, Pintor-Toro JA, Pardo JM (
Gadjev I, Vanderauwera S, Gechev TS, Laloi C, Minkov IN, Shulaev V, Apel K, Inze D, Mittler R, Breusegem FV (
Gechev TS, Breusegem FV, Stone JM, Denev I, Laloi C (
Gilmour SJ, Sebolt AM, Salazar MP, Everard JD, Thomashow MF (
Gonzalez-Aguilar GA, Fortiz J, Cruz R, Baez R, Wang CY (
Guo H, Ecker JR (
Guo Y, Xiong L, Ishitani M, Zhu JK (
Kasuga M, Miura S, Shinozaki K, Yamaguchi-Shinozaki K (
Kieber JJ, Rothenberg M, Roman G, Feldmann KA, Ecker JR (
Kizis D, Lumbreras V, Pages M (
Lee BH, Lee H, Xiong L, Zhu JK (
Liu Q, Kasuga M, Sakuma Y, Abe H, Miura S, Yamaguchi-Shinozaki K, Shinozaki K (
Liu Y, Zhao TJ, Liu JM, Liu WQ, Liu Q, Yan YB, Zhou HM (
Liu YG, Mitsukawa N, Oosumi T, Whittier RF (
Marcus Y, Altman-Gueta H, Finkler A, Gurevitz M (
Masuta C, Furuno M, Tanaka H, Yamada M, Koiwai A (
Mazur BJ, Chui CF (
Menke FLH, Champion A, Kijne JW, Memelink J (
Mittler R (
Mittler R, Vanderauwera S, Gollery M, Breusegem FV (
Morel J, Fromentin J, Blein JP, Simon-Plas F, Elmayan T (
Mori IC, Schroeder JI (
Mukhopadhyay A, Vij S, Tyagi AK (
Mustilli AC, Merlot S, Vavasseur A, Fenzi F, Giraudat J (
Narusaka Y, Nakashima K, Shinwari ZK, Sakuma Y, Furihata T, Abe H, Narusaka M, Shinozaki K, Yamaguchi-Shinozaki K (
Nishizawa A, Yabuta Y, Shigeoka S (
Niu X, Helentjaris T, Bate NJ (
Ohme-Takagi M, Shinshi H (
Orvar BL, Ellis BE (
Pasqualini S, Paolocci F, Borgogni A, Morettini R, Ederli L (
Pitzschke A, Hirt H (
Potuschak T, Lechner E, Parmentier Y, Yanagisawa S, Grava S, Koncz C, Genschik P (
Ruuska SA, Andrews TJ, Badger MR, Price GD, von Caemmerer S (
Shih MC, Lazar G, Goodman HM (
Simon-Plas F, Elmayan T, Blein JP (
Slaymaker DH, Navarre DA, Clark D, del Pozo O, Martin GB, Klessig DF (
Slooten L, Capiau K, Van Camp W, Van Montagu M, Sybesma C, Inze D (
Smith KS, Ferry JG (
Solano R, Stepanova A, Chao Q, Ecker JR (
Tang W, Charles TM, Newton RJ (
Tieman DM, Ciardi JA, Taylor MG, Klee HJ (
Torres-Schumann S, Godoy JA, Pintor-Toro JA (
Trevino MB, O'Connell MA (
Vos P, Hogers R, Bleeker M, Reijans M, van de Lee T, Hornes M, Frijters A, Pot J, Peleman J, Kuiper M, et al (
Wang H, Huang Z, Chen Q, Zhang Z, Zhang H, Wu Y, Huang D, Huang R (
Winterbourn CC, Hawkins RE, Brian M, Carrell RW (
Wormuth D, Heiber I, Shaikali J, Kandlbinder A, Baier M, Dietz KJ (
Xue GP, Loveridge CW (
Yamaguchi-Shinozaki K, Shinozaki K (
Yang Y, Li R, Qi M (
Zhang H, Zhang D, Chen J, Yang Y, Huang Z, Huang D, Wang XC, Huang R (
Author notes
This work was supported by the National Science Foundation of China (grant nos. 30525034 and 30671135) and the National Basic Research Program of China (grant nos. 2006CB100102 and 2007CB108801).
These authors contributed equally to the article.
Corresponding author; e-mail [email protected].
The author responsible for the distribution of materials integral to the findings presented in this article in accordance with the policy described in the Instructions for Authors (www.plantphysiol.org) is: Rongfeng Huang ([email protected]).
Some figures in this article are displayed in color online but in black and white in the print edition.
The online version of this article contains Web-only data.
Open access articles can be viewed online without a subscription.