-
PDF
- Split View
-
Views
-
Cite
Cite
Georg F Zellmer, Yoshiyuki Iizuka, Susanne M Straub, Origin of Crystals in Mafic to Intermediate Magmas from Circum-Pacific Continental Arcs: Transcrustal Magmatic Systems Versus Transcrustal Plutonic Systems, Journal of Petrology, Volume 65, Issue 3, March 2024, egae013, https://doi.org/10.1093/petrology/egae013
- Share Icon Share
Abstract
Complex zoning in crystals including repeated resorption and overgrowth is characteristic for arc magmas and occurs in response to closed-system changes in magmatic P–T–fO2 conditions and open system processes such as magma mixing and degassing or regassing. However, over which time frame do such changes occur? Do zoning patterns record changes occurring during the polybaric ascent of magmas that carry crystals or glomerocrysts sourced from variably mushy magma reservoirs, or alternatively indicate the uptake of antecrysts that experienced long periods of cold storage in plutonic precursors? A priori, these scenarios are endmember models, with the former transcrustal magmatic systems, where the crystals record changing conditions during magma ascent or changing interstitial melt compositions, traditionally preferred over the latter, which we here term transcrustal plutonic systems. In subsolidus plutonic systems, aphyric parental melts would acquire their entirely antecrystic crystal cargo during ascent from plutonic protoliths, and only crystal rims may be related to the host magma. We discuss the evidence for dominantly plutonic antecrystic cargo in some continental arc magmas, identified by considering mineral phase proportions, hydration of crystal rims that indicate hydrothermally altered cargo picked up by fresh melts, and uranium isotope disequilibria between crystals and matrix. We then turn to two-pyroxene thermobarometry and review the evidence for plutonic antecryst dominance revealed by this method in southwest Japan and the southern Taupo Volcanic Zone. We provide additional two-pyroxene data from the Andes, the Cascades, and the Tatun Volcano Group in northern Taiwan, corroborating that the uptake of crystals by aphyric to scarcely phyric melts is prevalent in continental arc magmatic systems. Thus, in many cases transcrustal plutonic systems seem to dominate, implying that a significant proportion of parental melts of continental arc magmas are variably enriched in silica, too hot to carry crystals, and typically too hot and not hydrous enough to be generated by differentiation in frequently postulated lower crustal hot zones, as we will demonstrate here. Our data indicate that in continental subduction zones, the mantle wedge is the source of a diversity of melt compositions (low- to high-silica), irrespective of the age and temperature of the subducting slab. Before discussing some of the implications of the prevalence of non-canonical transcrustal plutonic systems for the thermal structure of the crust, magma ascent processes, volcano monitoring, economic geology, as well as the evolution of continental crustal growth and recycling through deep time, we critically evaluate this novel perspective in terms of published data that might favour more traditional supersolidus transcrustal magmatic systems. This contribution provides the community with the opportunity to consider significantly colder crustal environments than typically accepted, and outlines avenues of future research.
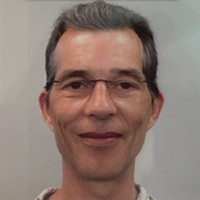
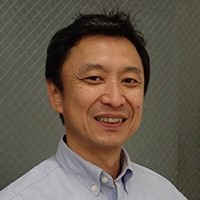
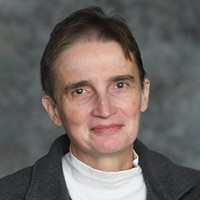
INTRODUCTION
The principles that govern magma transport from the Earth mantle through the crustal lid have been known for decades (Lister, 1990; Lister & Kerr, 1991; Rubin, 1995; Petrelli & Zellmer, 2020). In the case of subduction zones, a typical feature of arc eruptive products is the complex petrography compared to magmas erupted in other tectonic settings. Crystals in magmas have traditionally been used as faithful recorders of magmatic conditions experienced by magmas prior to their eruption (Putirka, 2008), based on experimental petrological work yielding mineral-melt equilibria at known magmatic conditions. However, natural samples from volcanic arcs typically display a highly complex petrography, indicating disequilibrium processes. Crystals often show disequilibrium textures, such as resorption zones associated with distinct chemical variations (e.g. Davidson & Tepley, 1997; Zellmer et al., 1999; Charlier & Zellmer, 2000; Zellmer & Clavero, 2006; Zellmer & Turner, 2007; Jerram & Martin, 2008; Ramos & Tepley, 2008; Streck, 2008). This complexity has been attributed to the presence of antecrysts, crystals that formed earlier from consanguineous magmas of the same system and that are taken up by new magma prior to eruption (Zellmer, 2021). Further, minerals sometimes show clear evidence for chemical disequilibrium with their host melt through the presence of reaction rims (D'Mello et al., 2021). This is typically attributed to open-system processes occurring in the overriding crust, such as mixing between magmas (e.g. Snyder, 1997; Clynne, 1999; Kent et al., 2010) or between magma and crystal mush (Pyle et al., 1988; Nakamura, 1995), or crystal uptake into the melt during crustal magma transfer or storage (e.g. Stewart et al., 1996; Dungan, 2005; Davidson et al., 2007; Streck et al., 2007; Jerram & Martin, 2008; Zellmer et al., 2014b). Linked to this interpretation of open system crustal processing of arc magmas has been the notion, held for several decades, that magmatic differentiation through crystal formation and crystal-melt separation (also known as ‘fractional crystallization’) is a fundamental crustal process, during which mafic parental magmas generated by melting in an ultramafic mantle evolve to more felsic compositions (Gill, 1981; Thorpe, 1982). There is also the notion that the crystal cargo carried by arc volcanic products may record this processing as well as the intensive parameters under which this processing occurs.
In this Perspectives article, we use the crystal size classification (Figure 1) and petrogenetic terminology of Zellmer et al. (2021). The term phenocryst is used in its original meaning of a large crystal in a fine grained or glassy groundmass (Iddings, 1892). Petrogenetically, we distinguish ‘autocrysts’ (crystals grown from the carrier melt), ‘antecrysts’ (crystals grown from consanguineous melts of the same igneous system at an earlier time), and ‘xenocrysts’ (crystals foreign to the present igneous system). To clarify, xenocrysts may form by mechanical disintegration of sedimentary or metamorphic xenoliths, while antecrysts may form by disintegration of igneous (plutonic or cumulate) xenoliths or glomerocrysts; we do not suggest the introduction of the word ‘antelith’ for consanguineous igneous xenoliths.
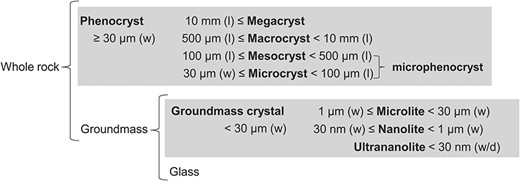
Crystal size classification of Zellmer et al. (2021), here adapted from Mujin (2022), fig. 2a). Abbreviations: l: length; w: width; d: diameter.
In principle, the information carried by the crystal cargo of volcanic samples on the crustal plumbing system depends on the pressure–temperature–time histories of crystals during their growth, with resorption events representing hiatuses that by themselves also carry information on the processes operating, e.g., temperature increase through magma recharge. Considering temperature alone, two endmember states of crustal plumbing systems may be hypothesised, which we here refer to as canonical transcrustal magmatic systems and non-canonical transcrustal plutonic systems, representing high- and low-temperature scenarios, respectively (Figure 2). A range of intermediate states with intermediate temperatures may be envisaged, but focussing on the endmember scenarios provides insights into the fundamental role of crustal temperature on the dynamics of crustal plumbing systems.
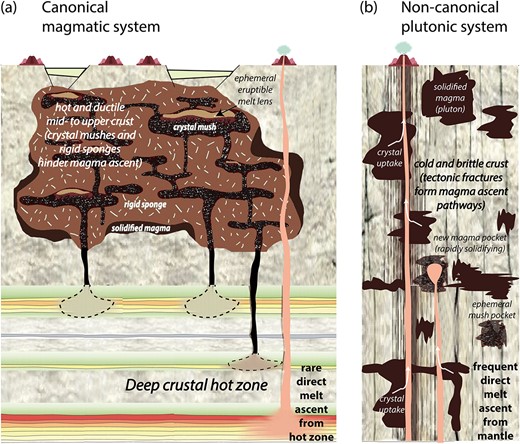
Reproduced from Coulthard Jr. et al. (2024). (a) A canonical transcrustal magmatic system, based on the deep crustal hot zone model of Annen et al. (2006) and the upper crustal magma-mush reservoir system of Miller (2016). In this largely supersolidus system, we can distinguish eruptible magmas with low (~0–5%) to intermediate (~5–15%) crystal contents from crystal mushes (~15–55%) set in a rigid sponge of >55% crystallinity (Hildreth, 2004). Note that fully solidified magmas (i.e., plutonic rocks) are restricted to the outer parts of this large upper crustal magmatic system. (b) A non-canonical transcrustal plutonic system, which lacks a deep crustal hot zone and is characterized by a brittle crust intruded by small ephemeral melt batches that rapidly cool through magmatic, mushy, and spongey states to solid plutonic rocks. These are the two conceptional endmember models of magma transfer through the crust discussed here. They govern (and are governed by) the crustal temperature structure, which in turn is ultimately related to the rate of magma influx into the crust from the melt generation region in the upper mantle.
Hypothesis I: canonical transcrustal magmatic systems
While transcrustal magmatic systems have only recently been described as such (Cashman et al., 2017), their key concept is high-temperature crustal processing and differentiation of arc magmas. From here on, we will use the shorthand ‘magmatic systems’. Magmatic systems represent a high-temperature endmember scenario, where large volumes of the crust are in supersolidus state of variable crystallinity, ranging from highly crystalline rigid sponges with interstitial melts through more melt-rich mush zones to crystal poor eruptible magma lenses (Figure 2a). The existence of magmatic systems can be regarded as the canonical view of the petrological community at present. These systems are seen as instrumental in controlling eruption initiation and inter-eruptive unrest or quiescence (as for example assumed by the new large-scale subduction zone initiative, SZ4D, see www.sz4d.org). The lower part of these systems is the deep crustal hot zone, where hot primary basalts repeatedly intrude, leading to partial melting of previous high-temperature basaltic intrusions and concomitant solidification of the new magma (Annen et al., 2006). Low-degree residual melts are enriched in silica and ascend into the mid and upper crust. Consistent with the compositional shift from lower mafic to upper felsic crust observed in arcs (Jagoutz & Klein, 2018), the upper parts of magmatic systems are thus generally more silica-rich. Here, magmas represent hot crystal mushes, i.e., frameworks of crystals with interstitial melts, environments prone to magma mingling and mixing with new ascending melt batches (Hildreth, 2007; Bergantz et al., 2015; Cashman et al., 2017). Complexly zoned crystal cargoes are seen as evidence for magma mixing resulting in crystal resorption and overgrowth (Zellmer et al., 2003; Leeman & Smith, 2018; Streck & Leeman, 2018). There is some debate regarding the melt fraction in these mush zones, which is linked to the temperature of the system and how this varies through space and time (Cooper & Kent, 2014; Barboni et al., 2016; Karakas et al., 2017). However, the general notion is that these mush zones are typically near but slightly above their solidus temperature, resulting in a significantly elevated geothermal gradient overall (Blundy, 2022, and references therein), and thus can be remobilized rapidly irrespective of the amount of pre-existing interstitial melt. One major consequence of crustal-level magmatic differentiation is the requirement for removal (via delamination from the lower crust and recycling into the mantle) of large amounts of ultralow-silica residue fractionated from basaltic primary melts (Gómez-Tuena et al., 2014; Jagoutz & Kelemen, 2015). Recent work has shown that this may be problematic in the presence of interstitial partial melts that make the bulk of this material too buoyant to be effectively removed (Bowman et al., 2021).
The notion of magmatic systems characterizing the crust is intimately bound to the idea that all magmatic differentiation occurs in the crustal lid, because parental magmas are exclusively mafic in composition. A major problem of having large volumes of the crustal lid, including in the upper crust, at mushy (i.e. supersolidus) conditions is the energy required to maintain this elevated temperature state, given the low temperature (0–50°C) boundary condition at the surface of the Earth. Recently, Glazner (2021) has shown that to maintain upper crustal magmatic mush zones, basaltic magma intrusion rates of the order of tens of kilometres per million years would be required. This is inconsistent with the geological evidence from exposed crustal sections, which do not show such thick piles of mafic intrusive rocks (Jagoutz & Behn, 2013). Thermal constraints are less limiting in the lower crust (Glazner, 2021) and, therefore, allow the existence of a deep crustal hot zone to generate differentiated magmas from parental basaltic melts (Annen et al., 2006).
Hypothesis II: Non-canonical Transcrustal plutonic systems
An alternative transcrustal plumbing system has recently been suggested by Coulthard Jr. et al. (2024) for the southern Taupo Volcanic Zone in New Zealand, based on plagioclase diffusion chronometry of microantecrysts erupted there (Lormand et al., 2021). In this model, buoyant melt batches of variable composition may rapidly ascend though the crustal section and, due to their low volume (Zellmer & Annen, 2008), most may solidify to form plutonic rocks of a variety of compositions. Importantly, crystals in this transcrustal plutonic system may be repeatedly remobilized and redeposited by ascending melts and, therefore, resorb and overgrow intermittently en route, rather than in place. Complexly zoned crystal cargo in eruptive products thus is equally expected (Lormand et al., 2021; Zellmer et al., 2021). Higher-silica melts will rise to shallower levels due to their lower density, again explaining the overall compositional variation from mafic lower to felsic upper crust (Jagoutz & Klein, 2018). The difference to the canonical view (Hypothesis I) is that the geothermal gradient may only be marginally elevated and that most intrusions rapidly solidify, even in the lower crust where ambient temperatures may be as low as < 600°C (Whittington et al., 2009). Crystal-melt mixing replaces magma mixing (Zellmer et al., 2014a; Zellmer et al., 2014b; Zellmer et al., 2016a), and ascending magmas are not trapped by ductile mush zones. Instead, they may easily fracture through to the surface, resulting in eruptions associated with only very short-term shallow geophysical unrest (Ruprecht & Plank, 2013). From here on, we will use the shorthand ‘plutonic systems’. This is the low-temperature endmember scenario, where most of the crust is in a state well below the solidus, disturbed by ephemeral spikes of high temperature intrusions of melts into rapidly solidifying magma pockets (Figure 2b). Plutonic systems are consistent with the scenario of a long period of cold storage of crystal cargo that has been suggested on basis of differences between diffusion chronometric and radiometric ages returned by crystals from many volcanic rocks (Zellmer, 2009; Cooper & Kent, 2014). Just as magmatic systems host small amounts of solid plutonic rocks, plutonic systems contain small amounts of magmas. However, these magmas rapidly transform through mushes and rigid sponges to solid rocks. In the case of plutonic systems, if the melt phase that picks up the plutonic crystal cargo rises rapidly from the mantle, its temperature may be too high to crystallize itself en route, and all the crystals may be of antecrystic origin. Further, rapid ascent may also hinder the kinetic process of crystal resorption to progress to a degree significant enough to be identified texturally, such that euhedral crystals might still be common.
The scarcity of supersolidus conditions in transcrustal plutonic systems limits magmatic differentiation, which may only occur locally and ephemerally. What then is the origin of high-silica magmas? About 15 years ago, modelling of plate subduction and associated mantle flow (Castro & Gerya, 2008) suggested that subduction ‘melanges’, i.e., mixtures of components from the subducting slab and the adjacent mantle wedge (Marschall & Schumacher, 2012), may partially melt and generate a range of magmatic compositions (low- to high-silica) in the mantle wedge. Subcrustal generation of more felsic arc magmas has one major advantage. Similar in composition to the overriding crust, these subcrustal melts require little or no differentiation to explain the felsic crustal composition, and, therefore, no delamination of large amounts of ultramafic materials fractionated from basaltic primary melts is required. Instead, relamination of variably felsic melts generated in the mantle wedge to the base of the overriding crust is envisaged (Behn et al., 2011; Hacker et al., 2011; Kelemen & Behn, 2016), and such melts may also ascent through the crust, contributing to arc volcanism (Parolari et al., 2018; Parolari et al., 2021).
OBSERVATIONS
In this Perspectives article, we draw from several previous studies that have provided evidence for crystal uptake into sparsely phyric or aphyric melts, i.e., the prevalence of antecrysts in arc magmas, in support of non-canonical plutonic systems where high-temperature melts pick up low-temperature crystal cargo. The evidence is manifold: (I) mineral phase proportions that are inconsistent with crystal growth in equilibrium with and from magmas that carry the crystals in southwest Honshu, Japan (Zellmer et al., 2014b; Zellmer et al., 2015a), with some proportions inconsistent with the magmatic system model; (II) hydrous rims of nominally anhydrous minerals indicating shock melting of nanometre-sized hydrous alteration phases, again in southwest Honshu (Zellmer et al., 2015a); (III) 238U–234U disequilibria, attributed to hydrothermal alteration of plutonic intrusives, in mineral cargo separated from rocks with groundmasses in 238U–234U equilibrium, in the Andes (Zellmer et al., 2014a), Taiwan (Zellmer et al., 2015b), and New Zealand (Zellmer et al., 2020); and (IV) two-pyroxene pseudo-PT observations combined with MELTS modelling that are consistent with uptake of pyroxenes from a range of crustal pressures into the ascending melts in southwest Honshu (Zellmer et al., 2014b) and New Zealand (Zellmer et al., 2021), and inconsistent with simple magma mixing. For the latter approach, we here also provide new data and from the Andes, the Cascades, and Taiwan. The combined evidence shows that plutonic antecryst uptake into hot melts and thus non-canonical plutonic systems are not atypical for Circum-Pacific continental arcs.
(I) Minerals and mineral phase proportions in mafic to intermediate continental arc magmas
Due to the lack of a global database of minerals and mineral phase proportions in igneous rocks on Earth, it is difficult to quantitatively demonstrate the vast range from aphyric through monomineralic, bimineralic, and multi-mineralic andesites of continental arcs, although this diversity has been explicitly pointed out more than 40 years ago in the Setouchi volcanic belt (Tatsumi & Ishizaka, 1982), a Miocene continental arc in southwest Japan (Tatsumi & Hanyu, 2003; Tatsumi, 2006). Here, phenocrysts are absent (in aphyric rocks) or contribute to monomineralic (orthopyroxene only), bimineralic (olivine and orthopyroxene, olivine and clinopyroxene, and olivine and hornblende), and trimineralic (olivine, orthopyroxene, and clinopyroxene) assemblages. Due to the lack of reliable quantitative data on mineral proportions, in this section we continue to present mostly qualitative observations of phenocrysts and their relative abundance but extend them to more basic bulk rock compositions than the intermediate high-Mg samples studied by Tatsumi & Ishizaka (1982).
Considering basaltic arc rocks to assess the mafic compositions, we may simply turn to the basalts of the Taupo Volcanic Zone, New Zealand. These basalts range from multimineralic (Pukeonake: olivine, orthopyroxene, clinopyroxene, plagioclase; Beier et al., 2017), through trimineralic (Ohakune: olivine, orthopyroxene, clinopyroxene; Kósik et al., 2016) and bimineralic (Kakuki: olivine, plagioclase; Gamble et al., 1990) to aphyric when excluding xenocrysts (Tarawera; Cole, 1970). Monomineralic basalts, e.g. carrying olivine as only phenocryst phase, are not found in the TVZ but do of course exist elsewhere, with the perhaps most striking examples in southwest Honshu (Figure 3a), where some basalts from the Abu volcanic field and from Daisen volcano carry more than 10% olivine but no other mineral phases (Tamura et al., 2000). Thermodynamic crystallization modelling with MELTS (Ghiorso & Sack, 1995; Smith & Asimow, 2005) shows that other mineral phases such as pyroxenes and plagioclase would crystallize from the matrix or bulk rock compositions of the Daisen basalts after well below 10% of olivine crystallization (see supplementary text), providing clear evidence that these olivines cannot be the single crystallization product formed by cooling or degassing of the basaltic rocks they are found in.
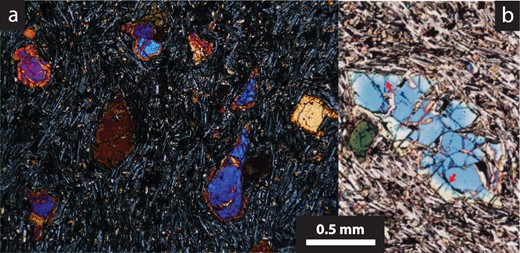
(a) Cross-polarized light image of mesocrysts of olivine in a basalt from Daisen volcano, southwest Honshu, Japan, set in a fine-grained groundmass dominated by plagioclase microlites that display a trachytic texture. (b) Cross-polarized light image of an olivine macrocryst from another Daisen basalt, displaying irregularities and discontinuities in the thickness of the rim, some of which are indicated by the arrows (adapted from Tamura et al., 2000).
The variety of relative mineral abundances in mafic to intermediate continental arc volcanic rocks would be difficult and, in some cases, impossible to explain if crystals were deemed autocrystic and crystallization was related to the chemistry of the carrier melt of the mineral cargo alone. The presence or absence of individual phases in erupted rocks is evidently not indicative of the magmas producing these deposits alone. Mechanical processes, such as disaggregation of plutonic precursors or disintegration of subvolcanic crystal mushes, must also be considered and may in fact dominate the mineralogy and mineral proportions of volcanic arc rocks across the mafic to intermediate bulk-rock compositional range. This implies that a significant proportion (in some cases up to 100%) of the phenocrysts (sensu Iddings, 1892) carried by these arc eruptives are of antecrystic or xenocrystic origin and, therefore, that the carrier melt phase of the magma may frequently contribute little (and in some cases no) mass to the ultimate crystallinity of the eruptives. This means that carrier melts may initially be aphyric, corroborated by the presence of some aphyric eruptives, retain high temperatures, and thus have crustal residence times too short to result in cooling- or degassing-induced crystallization, processes characteristic of magmatic systems. Further, the existence of monomineralic volcanic rocks precludes the possibility that these are interstitial melts equilibrated with a monomineralic mush framework. Instead, crystals will have been picked up from crustal monomineralic and likely subsolidus cumulate layers, i.e., plutonic rocks.
Crystal uptake into arc magmas and the presence of antecrysts is widely accepted, but the nature of the crystal source (sub-solidus or super-solidus) remains debated. We, therefore, now turn to a process that cannot operate in super-solidus systems: hydrothermal alteration.
(II) Hydrous rims of nominally anhydrous minerals
To investigate the genesis of monomineralic basalts in more detail, we consider here the olivine mesocrysts in poorly vesicular basalt lavas from southwest Japan further to obtain insights into the temperature history of this crystal cargo. Figure 3 shows a variation in birefringence colours towards the crystal rims, indicating that olivine rim compositions differ from those of the core. Originally interpreted as iron-rich overgrowth rims by Tamura et al. (2000), it is evident from Figure 3b that the rims display irregularities in their thickness and discontinuities, even along straight crystal faces. Zellmer et al. (2015a) studied olivine rims of this and other basalts from southwest Honshu in detail by isotope microscopy (Yurimoto et al., 2003) and transmission electron microscopy. They showed that some samples carried olivines with rims containing micron- to submicron-wide fingers of hydrous, aluminous amorphous materials (Figure 4). These were interpreted as glasses generated through thermal shock-melting of hydrous minerals that were beginning to form in the olivine rims during hydrothermal alteration prior to uptake into their hot carrier melt. Figure 4b–e shows the isotope microscopy results of the rim of an olivine from a Mengameyama basalt in southwest Honshu, situated between the Abu volcanic field and Daisen volcano, and Figure 4f is a high-resolution TEM image of an olivine rim from a Daisen basalt, displaying the lack of lattice fringes in the amorphous material when compared to the surrounding Fe-rich olivine. Even though the crystal in Figure 4a appears euhedral, it is clear that it has experienced hydrothermal alteration prior to its uptake into the host melt, and that its residence in the magma was too short to cause resorption. Thus, despite their euhedral form, these crystals are antecrystic in nature. Hydrothermal alteration cannot operate in supersolidus mush systems. For meteoric fluids to interact with the crystals, they would have to have been stored at temperatures significantly below the solidus prior to disaggregation into their carrier melt. This clearly indicates a low-temperature origin of the crystal cargo, in support of plutonic systems. While Zellmer et al. (2015a) worked within the framework of magmatic systems and, therefore, suggested that hydrothermal alteration was restricted to the upper crust, in principle the plutonic system model would allow alteration to reach greater depths, i.e. mid- to deep crustal levels.
![(a) Backscattered electron image of an olivine rim from a Mengameyama basalt, to demonstrate features typical for basalts erupted in southwest Honshu. (b)-(e) Isotope microscopy of the area indicated by a square in panel a, using a stacked CMOS-type active pixel sensor, with brighter tones corresponding to higher ion intensity. Total ion counts are shown by greyscale bar for each image, with corresponding ion counts shown by numbers below the bar, for counting times of 70, 70, 45, and 35 s in panels b, c, d, and e, respectively. The rim of the crystal is characterized by rim-perpendicular fingers enriched in H, OH and Al and depleted in Si. The bright area in (e) represents a plagioclase microcryst close to the olivine rim. (f) TEM high resolution image of a similarly textured Fe-rich olivine rim from a Daisen basalt, southwest Japan, viewed along the [100]ol direction. The porous and amorphous (amr) nature within the low-density domains in the olivine (ol) rim is evident, as the only observed lattice fringes are from the surrounding olivine matrix. Figure modified from Zellmer et al. (2015a), figs. 5 and 9).](https://oup.silverchair-cdn.com/oup/backfile/Content_public/Journal/petrology/65/3/10.1093_petrology_egae013/9/m_egae013f4.jpeg?Expires=1750182770&Signature=R3yg~BTR8I2c9Ey~GOmHTdwZiFE0whW4aSTR79rOXT4szv4L3ooPEuONZ~MpWcFh8Y5H8tRBzRTCnRvZddATwCI59tEFxOUefp~PYNxxT16Qi61Kce2r3Kv87lZArGZ9Sr0dsX-44lsHMMLhQdCjZ~~m4E3LKG6I6Vcw7FUPno~tm4Z2Vq5eGx9ExNFC9HMWzrYGtgPwIp6IJAiZuw4eT6aAWBcbCVjyEY-2YWS3qYd96BP~Rhmf-rIis72g7pED3uWp~bCWQEPAamE~vWSbuCs9pxN5iAX1Dr3VhWGZn3ynM9ctq-BvijwUFYukEcCtPd19KO1YZtcHQ3noj3Kuyw__&Key-Pair-Id=APKAIE5G5CRDK6RD3PGA)
(a) Backscattered electron image of an olivine rim from a Mengameyama basalt, to demonstrate features typical for basalts erupted in southwest Honshu. (b)-(e) Isotope microscopy of the area indicated by a square in panel a, using a stacked CMOS-type active pixel sensor, with brighter tones corresponding to higher ion intensity. Total ion counts are shown by greyscale bar for each image, with corresponding ion counts shown by numbers below the bar, for counting times of 70, 70, 45, and 35 s in panels b, c, d, and e, respectively. The rim of the crystal is characterized by rim-perpendicular fingers enriched in H, OH and Al and depleted in Si. The bright area in (e) represents a plagioclase microcryst close to the olivine rim. (f) TEM high resolution image of a similarly textured Fe-rich olivine rim from a Daisen basalt, southwest Japan, viewed along the [100]ol direction. The porous and amorphous (amr) nature within the low-density domains in the olivine (ol) rim is evident, as the only observed lattice fringes are from the surrounding olivine matrix. Figure modified from Zellmer et al. (2015a), figs. 5 and 9).
(III) Uranium isotope disequilibria between minerals and matrix
Uranium series isotope studies have provided many insightful results in the last half century on the processes and timescales of melt generation, magma transfer, and preeruptive crystallization in various tectonomagmatic settings. We will focus here on U-series mineral-melt isochron studies that have often been employed to constrain crystallization timescales, with variable success (e.g. Allègre & Condomines, 1976; Pyle et al., 1988; Volpe & Hammond, 1991; Heath et al., 1998; Zellmer et al., 2000; Jicha et al., 2005; Zellmer et al., 2014a; Zellmer et al., 2015b). The use of 238U–230Th disequilibria in igneous rocks requires equilibrium between 238U and the immediate parent of 230Th, namely 234U. Disequilibria in the 238U–234U system, reflected in (238U/234U) activity ratios that are unequal to 1, reflect secondary alteration processes that mobilize uranium (Rosholt, 1982), which makes 238U–230Th isochron dating impossible, because this dating method, like all mineral isochron methods, does not return reliable results in open systems (Hughes & Hawkesworth, 1999). As mineral separates have low uranium concentrations, they are prone to alteration by hydrothermal fluids that typically carry high concentrations due to the complexing of uranium with fluorine and chlorine.
Many studies verify that (238U/234U) = 1 for all mineral separates prior to moving on to U-Th dating (Volpe & Hammond, 1991; Volpe, 1992; Schaefer et al., 1993; Black et al., 1998; Zellmer et al., 2000), though some do not (Heath et al., 1998; Jicha et al., 2005; Jicha et al., 2007; Jicha et al., 2009). Clearly, in the view of the previously presented evidence of hydrothermally altered anhydrous phases picked up by fresh melts (Figure 4), this analytical step should not be omitted. Quite a few studies of mineral-groundmass separates from continental arc volcanic rocks have now shown that there are cases of 238U–234U bulk rock and groundmass equilibrium whereas some of the mineral phases yielded significant 234U-disequilibria (up to excesses in δ234U of ~140‰); these include mafic to intermediate eruptive products from the Taupo Volcanic Zone, New Zealand (Zellmer et al., 2020), from Callaqui, Longquimay, and Calbuco volcanoes in the Andes (Zellmer et al., 2014a), and from recent Tatun Volcano Group (TVG) eruptives from Taiwan (Zellmer et al., 2015b). Where (230Th/232Th) activity ratios have been measured, these data can be plotted on the interpretative diagram of Rosholt (1982), and this has been done for the Andean and TVG rocks in Figure 5a. 238U–234U disequilibria are clearly evident for the Andean mineral separates, and mild but still significant (uncertainties are much smaller than symbol size) are also seen in TVG mineral separates. These disequilibria indicate variable amounts of uranium assimilation, 230Th-predominant recoil gain processes, as well as some 234U recoil loss (Figure 5b), all of which point to secondary alteration of the crystal cargo in these samples. We therefore argue that the mineral separates from these samples contained a significant proportion of crystals that had experienced hydrothermal alteration prior to uptake into their carrier melt. Again, we stress that evidence of hydrothermal alteration by meteoric fluids requires crystal storage in subsolidus plutonic rocks prior to their uptake into the carrier melts, therefore supporting a plutonic rather than magmatic crustal system architecture. The plutonic system may allow hydrothermal alteration to occur at greater depth than one would expect in a magmatic system.
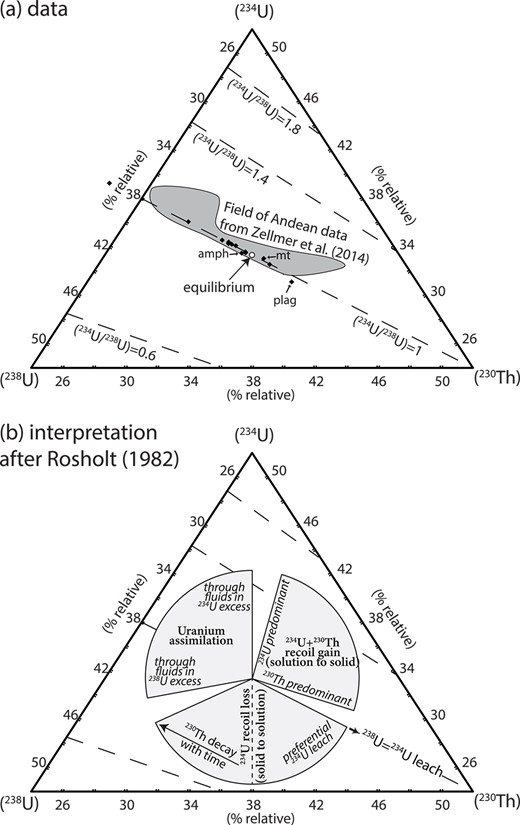
Triangular diagrams of the relative activity proportions of (238U), (234U) and (230Th), adapted from Zellmer et al. (2015b), fig. 7). (a) Tatun data, with uncertainties smaller than the symbol size. Amphibole, plagioclase and magnetite mineral separates from Mount Cising are indicated, as is the range of mineral separate data from some previously studied Andean lava flows (Zellmer et al., 2014a). (b) Interpretation of deviations from the point of equilibrium (Rosholt, 1982), see the text for the discussion.
(IV) MELTS modelling of two-pyroxene pseudo-PT observations
Given the evidence for mineral-melt disequilibrium in most arc magmas, the applicability of mineral-melt thermobarometers to obtain temperature and pressure information of pre-eruptive magma storage conditions becomes questionable. For example, there is clear evidence for clinopyroxene disequilibrium with the host rock for new data from Circum-Pacific arc volcanoes from the Andes and the Cascades obtained as part of this study based on the EnFs and DiHd equilibrium tests (Mollo et al., 2013, Mollo & Masotta, 2014, see supplementary text and Figure S1). However, there is the notion that thermobarometers based on mineral composition alone may provide constraints on the pressure and temperature conditions of the mineral cargo, and thus potentially those of the melt that formed the crystals in question, even if they are now carried as antecrystic cargo by their host. Two-pyroxene thermobarometry (Putirka, 2008) was originally developed to constrain the PT-conditions of touching orthopyroxene and clinopyroxene mineral pairs, as for example found in cumulate xenoliths or smaller glomerocrysts. This thermobarometer may thus be reliable for cumulates (e.g. Brahm et al., 2018), at least in terms of constraining temperature, if not pressure (Wieser et al., 2023). Yet, the two-pyroxene thermobarometer has also been employed using individual orthopyroxene and clinopyroxene crystals carried in the same melt, as long as they are in chemical (Fe-Mg exchange) equilibrium with one another. This is the opx–cpx matching method of Wieser et al. (2021). Here, it is implicitly assumed that orthopyroxene and clinopyroxene have formed from the same melt at the same time and under the same intrinsic growth conditions.
Clearly, this assumption may not be justifiable given the complexity of arc magmatic systems and the prevalence of antecrysts. Crystals may equally happen to be, fortuitously, in Fe-Mg exchange equilibrium if formed from different melts at different times under different intrinsic growth conditions. In this latter case, the derived PT-conditions may be entirely meaningless. Zellmer et al. (2014b) first realized this caveat when attempting to employ opx–cpx matching in mafic rocks from southwest Japan, finding PT-conditions that suggested isothermal decompression from the upper mantle to the surface (Figure 6). They recognized that these PT-conditions must be an artefact, as isothermal decompression would not result in pyroxene growth unless H2O-degassing was continuously occurring during magma ascent from great depth. They thus referred to these results as pseudo-decompression paths (PDPs). Using MELTS crystallization modelling of isobaric cooling followed by mixing of pyroxene crystals in Fe-Mg exchange equilibrium formed at different pressures, Zellmer et al. (2014b) showed that PDPs could be reproduced by mixing of crystals formed at variable depths, with pseudo-pressures and pseudo-temperatures dependent on modelled melt composition, oxygen fugacity, H2O content, and formation pressure differences of the matched oxp–cpx pairs. They also showed that in southwest Japan, PDPs could not be the result of magma mixing, based on the inability to match sample groundmass composition to mixtures of the modelled melt compositions required to crystallize the matched opx–cpx pairs. PDPs may, therefore, provide a clear indication of crystal-melt mixing rather than mixing of magmas hosting different crystal cargoes. Again, crystal-melt mixing requires the crystals to be sourced from a very crystal-rich reservoir, with little if any interstitial melt, either a slightly super-solidus rigid sponge or a subsolidus, plutonic precursor, implying a significantly lower temperature than the pseudo-temperatures would suggest. This is incompatible with mobile mush zones with significant interstitial melt, therefore further supporting the plutonic rather than the magmatic system architecture of the crust.
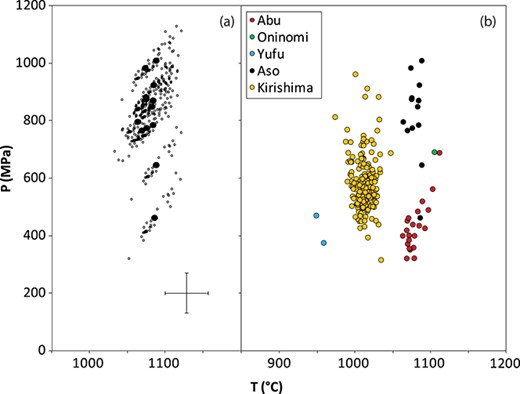
Pressure–temperature conditions estimated from manually paired orthopyroxene and clinopyroxene crystals in Fe–Mg equilibrium from southwest Japan (adapted from Zellmer et al., 2014b, fig. 4), using the equations 36 and 38 from Putirka (2008). (a) In a sample from Aso volcano, each orthopyroxene is in equilibrium with a number of clinopyroxenes, yielding several trends of positive slope (small circles). Large symbols are the P–T averages from each of these trends. The cross represents one standard deviation for each of these P–T estimates. It reflect the P–T range on each point based on the range of Fe-Mg exchange that may be considered in equilibrium, i.e. 0.95 ≤ KD(Fe-Mg) ≤ 1.23 (Putirka, 2008). (b) P–T estimates for all studied SW Japan samples that contain orthopyroxenes and clinopyroxenes. Kirishima, Aso and Abu samples yield large pressure variations at roughly constant temperatures. These trends are referred to as pseudo-decompression paths, and the 1SE uncertainty cross is based on the maximum range in opx–cpx pairs in equilibrium at Aso volcano.
Two-pyroxene pressure–temperature results from andesitic to dacitic tephras sourced from Ruapehu volcano and the Ngauruhoe cone from Tongariro volcano (Zellmer et al., 2021) equally produced large pressure ranges at what may be considered high-temperatures (c. 1000 to >1100°C) for these compositions. Here, it was shown that pressure results again represent pseudo-pressures of antecrystic crystal cargo, the presence of which is corroborated by petrographic disequilibrium textures. MELTS modelling suggested that these pseudo-pressures are a function not only of pressure but also significantly of oxygen fugacity and, therefore, cannot be taken to indicate actual crystallization depths (irrespective of recent work that points to high imprecision of pyroxene-based pressure estimates in general, Wieser et al., 2023). Strikingly, however, MELTS-modelled temperatures in this specific case were similar to those yielded by two-pyroxene thermometry, indicating that pyroxene antecrysts may indeed be high-temperature phases and thus grown in melt-dominant magmas (c. 80% residual liquid). This melt fraction is much higher than the proportion of interstitial melt purported to be present in typical arc magmatic crystal mush zones (< 55%, e.g. Bachmann & Bergantz, 2004). The high temperatures yielded by these antecrysts indicate that there was no time for Fe-Mg equilibration that would be expected during slow cooling of large magma reservoirs or mush zones. Instead cooling was rapid, as expected during crystallization following intrusion of small magma batches into cooler shallow crustal reservoirs and their rapid solidification to form solid intrusive bodies, e.g., in the form of dykes (Lormand et al., 2021). Recent diffusion chronometric work on plagioclase from the same samples indicates cooling timescales of hours to days for these antecrysts (Coulthard Jr. et al., 2024).
We here present additional two-pyroxene pseudo-PT conditions yielded by mafic to intermediate samples of Andean volcanoes (Lascar, Callaqui and Calbuco volcanoes; Figure 7), the Cascades (Mount St. Helens, Adams, Belknap, Crater Lake, Medicine Lake and Lassen volcanoes; Figure 8), and the Tatun Volcano Group in Taiwan (Mt. Hunglu, Belousov et al., 2010; Figure 9). As demonstrated in Figure S1, clinopyroxenes typically have compositions that are systematically offset in their EnFs and/or DiHd components from those expected to have formed from the composition of the bulk rocks, using the equilibrium test of Mollo et al. (2013). From a theoretical point of view, the difference between measured and predicted clinopyroxene components should be close to zero at the condition of thermodynamic equilibrium, implying that data should align along the 1:1 line. The systematic offsets are inconsistent with autocrysts settling through thermal gradients in a magma chamber, which would yield a much larger scatter (Mollo & Masotta, 2014). Thus, the data imply that these crystals cannot be considered autocrystic. Turning to the two-pyroxene thermobarometry results, while for some volcanoes they might be considered to return reasonably tight PT constraints within the claimed uncertainty of this method (e.g. Belknap and two of the Medicine Lake samples), the occurrence of clear PDPs in all others indicates that the results do not reflect natural PT conditions of pyroxene growth. Instead, they again suggest antecrystic crystal cargo dominates the crystallinity in most mafic to intermediate volcanic rocks from Circum-Pacific continental arcs. Variations in PDPs due to differences in oxygen fugacity, H2O content, and formation pressure ranges, and crystal uptake from solid plutonic precursors into aphyric melts, as previously shown in southwest Japan, result in the observed pseudo-PT variations. Previous work on the Andean volcanoes has shown that the intensive parameters vary significantly during the genesis of their magmas (Rea, 2009; Stechern, 2015; Morgado et al., 2019). Our data suggest that the same is true for the Cascades. Crystal-melt mixing rather than the mixing of magmas carrying crystal cargo of variable compositions in many of the Circum-Pacific continental subduction zone volcanoes again corroborates the dominance of plutonic rather than magmatic systems.
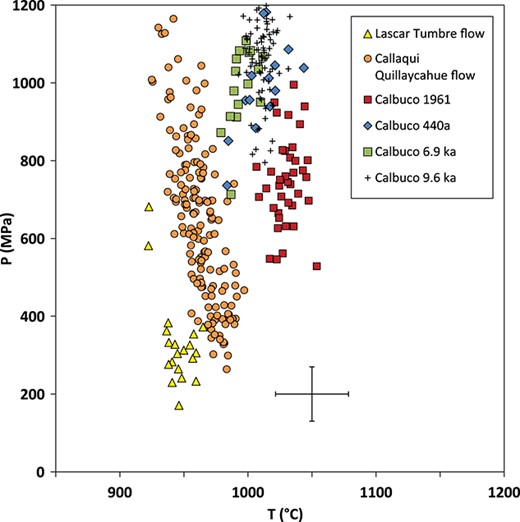
PT conditions returned by the Andes, computed using the iterative algorithm implemented in the Python3 tool Thermobar (v.0.07, Wieser et al., 2021), plotting orthopyroxene-based averages as in Figure 6b, up to 1200°C and 1.2 GPa. 1SE uncertainty cross taken to be the same as in Figure 6a. Sampling details, EPMA analytical methods and data provided in the supplement.
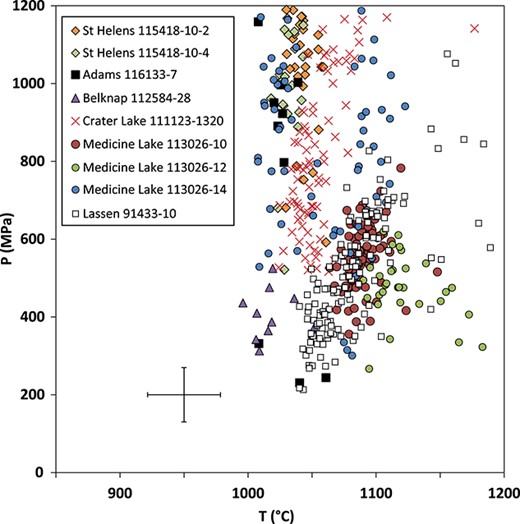
PT conditions returned by the Cascades, computed using the iterative algorithm implemented in the Python3 tool Thermobar (v.0.07, Wieser et al., 2021), plotting orthopyroxene-based averages as in Figure 6b, up to 1200°C and 1.2 GPa. 1SE uncertainty cross taken to be the same as in Figure 6a. Sampling details, EPMA analytical methods and data provided in the supplement.
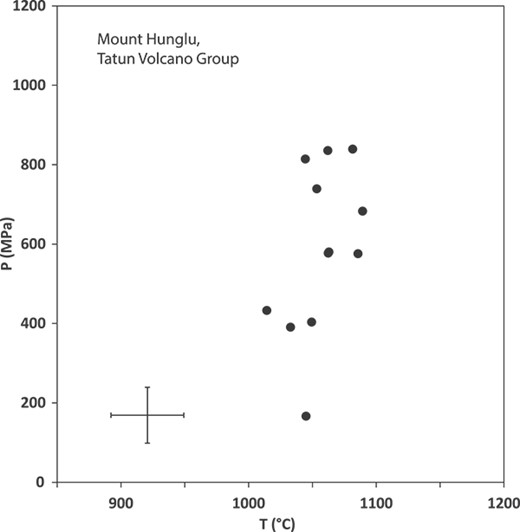
PT conditions returned from Mount Hunglu, Tatun Volcano Group, Taiwan, computed using the iterative algorithm implemented in the Python3 tool Thermobar (v.0.07, Wieser et al., 2021), plotting orthopyroxene-based averages as in Figure 6b, up to 1200°C and 1.2 GPa. 1SE uncertainty cross taken to be the same as in Figure 6a. Sampling details, EPMA analytical methods and data provided in the supplement.
Two-pyroxene pressure uncertainties
Pressure uncertainties on the two-pyroxene thermobarometer are quoted at approximately ±300 MPa (Putirka, 2008). Ad hoc, the observed pressure variations in the two-pyroxene results in Figures 6–9 might, therefore, be ascribed to data scatter on clinopyroxenes and orthopyroxenes formed under identical intensive conditions from the same melt, at a median pressure point. However, if this were the case, we would expect approximately Gaussian pressure distributions of c. 600 MPa for each sample, with data clustering around the median pressure. This is not the case. Looking at the pressure distributions in detail, we see that the samples yield pressure ranges between 250 and 900 MPa, with very few displaying a c. 600 MPa range. Further, distributions around the median pressure are far from Gaussian. The pressures are either evenly distributed, or bimodal, with clusters at higher or lower ends of the range.
The pressure ranges are, therefore, clearly pseudo-pressure ranges, and have no relationship to two-pyroxene crystallization pressures at a single crustal depth. Zellmer et al. (2014b) showed that oxygen fugacity has a strong effect on pseudo-pressure ranges, with lower fugacities yielding greater variations. As suggested above, our data are the result of a combination of crystals formed at different intensive conditions from different residual melt compositions, which were combined in the finally erupted sample. Therefore, the pseudo-pressure uncertainties quoted on Figures 6–9 reflect the pressure range on each point based on the range of Fe-Mg exchange that may be considered in equilibrium, i.e. 0.95 ≤ KD(Fe-Mg) ≤ 1.23 (Putirka, 2008).
Two-pyroxene temperature uncertainties
Temperature uncertainties on the two-pyroxene thermobarometer are quoted at approximately ±60 K (Putirka, 2008). Ad hoc, considering all samples, the temperatures returned lie mostly within a supersolidus range of 950–1100°C, i.e. might be ascribed to a supersolidus magmatic plumbing systems reflecting high-temperature crystallisation conditions. However, looking at the detail, we would, in this case, expect approximately Gaussian temperature scatters displaying a c. 120 K range for each sample, which clearly is not observed. The temperature variations are typically much lower, within a < < 100 K range.
The temperatures are, therefore, clearly pseudo-temperatures and have no relationship to a two-pyroxene crystallization temperature of formation. Zellmer et al. (2014b) showed that magma H2O contents have a strong effect on pseudo-temperatures, with higher H2O contents yielding lower pseudo-temperatures. Pseudo-temperatures are an artefact of the combination of crystals formed at different, often much lower temperatures, from different residual melt compositions, and at different intensive conditions. Therefore, the pseudo-temperature uncertainties quoted in Figures 6–9 again reflect the temperature range on each point based on the range of Fe–Mg exchange that may be considered in equilibrium, i.e. 0.95 ≤ KD (Fe–Mg) ≤ 1.23 (Putirka, 2008).
DISCUSSION
Origin of the mafic crystal cargo
The observations presented here on the crystals carried by (more or less randomly selected) mafic to intermediate Circum-Pacific continental arc volcanic rocks follow two themes: (i) The prevalence of antecrysts and xenocrysts, which has been widely accepted by the petrological community, although their proportion relative to autocrysts may be debated; and (ii) the subsolidus temperature of the source from which the crystal cargo is picked up by the carrier melt, which counters the notion of canonical magmatic systems (i.e. the commonly accepted hypothesis I) and corroborates the frequent occurrence of noncanonical plutonic systems (i.e. hypothesis II). Crystal uptake from subsolidus sources is most strongly suggested by the presence of hydrothermal alteration features (hydrous rims and 234U–238U disequilibria in mineral separates) of crystals carried in unaltered magmas, as such hydrothermal alteration cannot progress in supersolidus environments. Paradoxically, uptake from low-temperature subsolidus sources is further supported based on the high temperatures yielded by of some antecrysts from intermediate magmas that in fact indicate rapid cooling to subsolidus temperature to be preserved rather than eliminated by long-term (supersolidus) diffusional equilibration.
In fact, it has been recognized before that more felsic arc magmas contain crystals that previously reached near-solidus or subsolidus conditions based on petrographic and mineral chemical evidence: examples include crystal cargo from Mount Mazama and Mount St. Helens eruptives (Bacon & Lowenstern, 2005; Claiborne et al., 2010; Wright et al., 2012). This perspective provides equivalent evidence for mafic to intermediate arc volcanic products.
Crustal plumbing systems may thus be envisaged as environments of repetitive upward transfer of small volume melts that most frequently get intruded and rapidly solidify. Part of their solidification process is the formation of small volumes of cumulates and ephemeral mush pockets. During melt ascent in this overall brittle crustal regime, plutonic and sometimes hydrothermally altered xenoliths are picked up and disintegrate into glomerocrysts, which further disintegrate into antecrystic mineral cargo carried by the melt towards its ultimate place of storage and solidification (Figure 10). The common occurrence of glomerocrysts with compound crystal chemistries that are indistinguishable from those of the phenocrysts (sensu Iddings, 1892), as for example pointed out by D'Mello et al. (2023), is consistent with glomerocryst disintegration, and this process has been described in more detail elsewhere (Zellmer et al., 2016a). Solidified plutonic rocks thus contain autocrysts formed by the solidifying magma pockets and antecrysts sourced from crustal plutons situated deeper in the crust. Occasionally, however, initially aphyric melts get transferred through to the surface, forming volcanic eruptions. These eruptives may then contain antecrystic mineral cargo picked up from many crustal plutonic rocks solidified previously, some of which may have been partially altered through hydrothermal processes.
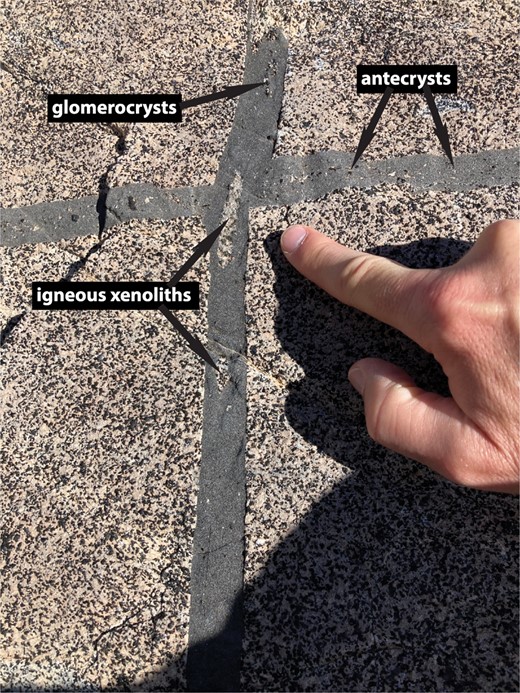
Illustration of the disintegration of igneous xenolith into glomerocrysts and antecrysts in a thin mafic dyke crosscutting the Adamello batholith, Italy. While not in the circum-Pacific setting, it provides a good example of the fundamental process at hand. Further information on these dykes can be found in Pimenta Silva et al. (2023).
If antecrysts are dominantly sourced from subsolidus lithologies, as the data presented here suggest, and if they dominate the crystal cargo of mafic to intermediate circum-Pacific continental arc magmas, this implies a scarcity (and potentially a lack) of volcanic autocrysts in arcs, as even some microlites have been shown to be of antecrystic nature (Lormand et al., 2021). It also implies a lack of long-lived, large supersolidus mid- to upper crustal mush zones that contribute crystals to the cargo of arc magmas, consistent with the thermal considerations of Glazner (2021). Thus, the erupting melts typically have not resided in such mush zones, from which they would carry crystals upwards when extracted. Instead, erupted melts will have to traverse the crust rapidly, with minimal overgrowth on picked-up subsolidus crystal cargo during late-stage degassing. In fact, given the subhedral to euhedral habit of some phenocrysts (sensu Iddings, 1892), ascent timescales appear to be too fast for the kinetic processes of crystal resorption or crystal growth to operate effectively in these melts. We note that crystal growth and resorption in natural silicate melts are typically operating at rates of <1 μm per hour (Cashman, 1990; Hammer & Rutherford, 2002; Couch et al., 2003; Mollard et al., 2012; Zellmer et al., 2016b; Zellmer et al., 2018; Lormand et al., 2020), and that typical magma ascent rates range from centimetres to tens of meters per second (Petrelli & Zellmer, 2020). This means that antecrysts carried up rapidly from 10 km depth to the surface may see <1 μm resorption, which may be easily missed by optical investigations.
Origin of the intermediate to felsic carrier melt: Deep crustal or subcrustal?
Because crystal uptake (from subsolidus lithologies into autocryst-free, i.e. initially aphyric melts) is a crustal process leading to differentiation of ascending magmas, groundmass compositions that are typically more felsic than whole rocks can in this case be considered the least rather than most “differentiated” compositions in mafic to intermediate Circum-Pacific continental arc magmas (cf. Streck et al., 2007; D'Mello et al., 2023). This implies a separate origin of magmas more felsic than typical primitive arc basalts. In the following, we consider if these felsic compositions can be produced in deep crustal hot zones (Annen et al., 2006), a scenario largely adopted by the petrological community and permissive based on thermal considerations (Glazner, 2021), or if they may be produced deeper (Castro & Gerya, 2008), a model ignored by the majority of the petrological community to date, although it does begin to gain more traction (e.g. Hacker et al., 2011; Straub et al., 2011; Timm et al., 2014; Straub et al., 2015; Straub et al., 2020; Parolari et al., 2021; Dallai et al., 2022).
Through repeated injection of mafic sills into the lower crust, the deep crustal hot zone model (Annen et al., 2006) generates a supersolidus environment in the deep crust where mixing, assimilation, storage and hybridization (MASH, Hildreth, 1981) processes are operating. This happens because still hot, subsolidus previously injected sills are easily remelted by new high-temperature mafic melts. Processes of partial melting of previously injected sills and fractional crystallisation of newly injected sills are occurring concomitantly and are thus effectively indistinguishable when considering the deep crustal hot zone in its entirety. The intermediate to felsic differentiated hot zone melts are taken to be the residual melts from partially crystalline regions of the deep crust (Annen et al., 2006). This implies that the most felsic melts represent the lowest melt fractions in a crystal-rich supersolidus framework (i.e. a lower crustal mush), while the most mafic melts originate from regions of low or no crystallinity (sill-shaped melt reservoirs). In this model, at low melt fractions, i.e., for felsic differentiates, melt compositions will be buffered by the mineralogy of the rigid crystal sponge (Blundy, 2022).
One fundamental question is how felsic melts are separated from the lower crustal mush without carrying many (if any) crystals of the mushy framework, given that we have established above that much of the crystal cargo may in fact be sourced from subsolidus plutonic rocks. In subduction zone environments, where mafic melts generated by fluid-fluxing of the mantle are hydrous, low-degree residual felsic melts will be extremely H2O-rich (Gavrilenko et al., 2019), and it may be postulated that the viscosity of these melts is so low that they may be easily expelled to shallower crustal levels simply by compaction of the solid crystalline framework that hosts them. But what is the temperature–composition relationship of such hydrous interstitial hot zone melts, and are natural felsic samples reflecting this relationship? Following D'Mello et al. (2023), we have plotted silica content versus the temperature of residual melts from two experimental studies of crystallization of variably hydrous mafic arc magmas (Melekhova et al., 2013; Nandedkar et al., 2014) as a grey field in Figure 11. As can be seen, intermediate to felsic deep crustal hot zone differentiates are in fact not that hot, yielding temperatures of <1100°C to c. 950°C in andesitic and c. 1000°C to 900°C in dacitic compositions. Further, residual melts indeed reach very high water contents, carrying 6 to >16 wt % H2O (depending on the initial H2O content of the basaltic starting material), and they can reach 10 wt % H2O in andesites even at <1 wt % initial H2O in the parental basaltic melt (Melekhova et al., 2013).
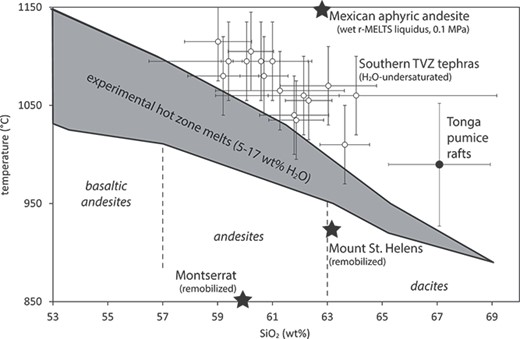
Temperature versus composition diagram showing the field of superhydrous experimental hot zone magmas (0.7–1.3 GPa, Melekhova et al., 2013, Nandedkar et al., 2014), compared to H2O-undersaturated hot southern Taupo Volcanic Zone andesitic tephras (Lormand et al., 2020); the Ventorillo aphyric dacite from Popocatepetl volcano (sample CH-09-18, Straub et al., 2014), with liquidus temperature estimated using r-MELTS (Gualda & Ghiorso, 2014; Gualda & Ghiorso, 2015); and Montserrat andesite and Mount St. Helens dacite averages (Gardner et al., 1995; Murphy et al., 1998). Also plotted are pumice rafts of unknown H2O content from the oceanic Tonga arc (Bryan et al., 2004).
When these intermediate to felsic deep crustal hot zone melts rise towards the surface, they will supersaturate in H2O at relatively deep crustal levels. We may, therefore, conclude that intermediate to felsic arc magmas crystallizing at shallow crustal levels will yield H2O-saturated hygrometric data and return modest thermometric temperature estimates. By contrast, hotter, H2O-undersaturated intermediate to felsic arc magmas cannot be sourced from a deep crustal hot zone environment. They will instead have to come from a hotter (i.e., subcrustal) source region, while also not being a product of extreme differentiation of hydrous basaltic melts to account for their H2O-undersaturation in the shallow crust.
Also plotted on Figure 11 are some natural intermediate eruptives. Crystal-rich andesites and dacites of Soufriere Hills, Montserrat, and Mount St. Helens, USA, respectively, plot at low temperature, below the experimental hot zone differentiates, permissive of their genesis in the deep crust. However, it may be argued that their high crystallinity in fact points to remobilization of plutonic protoliths, and thus that their low temperature is not sufficient evidence for their ultimate origin being crustal and not subcrustal. In contrast, the intermediate composition tephras erupted from the Central Plateau volcanoes of New Zealand (Ruapehu, Tongariro) yield too high pyroxene microlite-glass temperatures and too low plagioclase microlite-glass H2O contents (< 1.5 wt % at up to 500 MPa pressure, Lormand et al., 2020) to be generated in a deep crustal hot zone. Similar high-temperature intermediates (at somewhat higher H2O contents, but still << 4 wt %) are erupted at Taranaki volcano in the Hikurangi back-arc, based on phenocryst rim-groundmass hygrothermometry (D'Mello et al., 2023). For these eruptives, we must conclude sub-Moho genesis of intermediate- to high-silica melts prior to uptake of the crystal cargo. We note that the subducting crust at the Hikurangi margin in the western Pacific is old and cold, as opposed to the Mexican subduction zone, where subcrustal genesis has also been suggested and where aphyric eruptives suggest magma temperatures of c. 1150°C at the andesite-dacite transition based on determining their liquidus temperature through MELTS modelling (cf. Figure 11). Thus, subcrustal generation of intermediate to felsic arc melts appears to operate in several Circum-Pacific continental arc (and back-arc) volcanoes, irrespective of the age and temperature of the subducting slab. The plutonic systems displayed in Figure 2b thus do not require the presence of a deep crustal hot zone, and we have purposefully omitted it from the plutonic system architecture. We argue that the formation of deep crustal hot zones may be rare. Their formation requires sustained injection of hot mafic magmas at deep crustal levels, a scenario that may be easily modelled but may not reflect typical magma ascent processes in natural systems.
Transcrustal plutonic systems under scrutiny
In this section we address several arguments that may be taken to point to a supersolidus magmatic system architecture with polybaric magma storage regions in the overriding crust and discuss how the data that underly these arguments may instead be explained through a plutonic system architecture. Again, we emphasize that magmatic and plutonic systems are just hot and cold endmember scenarios, respectively, bounding a whole range of thermal conditions of the overriding crust that ultimately depend on magma flux rates. The potential feasibility of plutonic systems is here put under scrutiny. We conclude that the plutonic system architecture is a feasible solution if it is accepted that within them, magmatic differentiation processes may still operate on a local scale, allowing spatially restricted cumulate formation, particularly in the lower crust where temperature differences between injected melt pockets and surrounding rocks are relatively small so that solidification of the melts through cooling will take some time.
Apparent dominance of equilibrium mineral phases over xenocrysts
The crystal assemblages of some erupted arc magmas are frequently not random as would be expected by chance entrainment from unrelated sources. For example, the magma suite of the 1912 Valley of Ten Thousand Smokes eruption has ubiquitous plagioclase, orthopyroxene, Fe–Ti oxides, apatite, and pyrrhotite, with the rhyolite having quartz but no clinopyroxene, the dacite and andesite lacking quartz but yielding clinopyroxene, and some of the late andesites having olivine. In terms of mineral chemistry, plagioclase compositions are An25–29 in the rhyolite, An36–53 in the dacite, and An47–79 in the andesite. Fe–Ti oxide temperatures also vary systematically, with ranges of 805°C to 850°C, 855°C to 955°C, and 955°C to 990°C in rhyolite, dacite and andesite, respectively. Similar consistent variations are seen in the zoned climatic eruption of Mount Mazama (Bacon & Druitt, 1988; Druitt & Bacon, 1989).
Clearly, in these specific cases, uptake of crystals from plutonic precursor rocks cannot be called upon. However, we would argue that these examples come from zoned intermediate to felsic upper crustal magma chambers that fed large Plinian caldera-forming eruptions. Our work instead focuses on the mafic to intermediate regime and typically smaller eruptive volumes, which yield clear evidence of mineral-melt disequilibria (e.g. see Figure S1). We note that the plutonic system architecture does not preclude the short-term formation of shallow crustal intrusives that differentiate internally prior to eruption.
Apparent absence of exsolution textures in antecrystic mineral cargo
Gabbroic rocks often yield pyroxene crystals with exsolution lamellae, and if such cool plutonic precursors would typically be the source the crystals of volcanic rocks, exsolution lamellae may be expected to be commonly observed within them. Yet they appear to be rare, which ad hoc suggests that the involvement of subsolidus crystals in the genesis of volcanic eruptives is insignificant. However, we would argue that the uptake of crystals into hot carrier melts will result in re-solution and homogenization of such exsolution textures. There is little experimental data on the timescales of such re-solution processes, but early work on homogenization of magnetite-ulvospinel exsolution textures, for example, indicates that re-solution occurs within hours (Chevallier et al., 1957).
Further, there is in fact evidence of pyroxene exsolution textures in some intermediate composition volcanics, e.g. such textures are found in pyroxene microantecrysts in the Southern Taupo Volcanic Zone (Lormand et al., 2021). If some exsolution textures remain despite re-solution during ascent in hot carrier melts to the surface, perhaps they are frequently overseen.
Proportions of hydrothermally altered crystal cargo
Hard evidence for the subsolidus origin of some crystal cargo in the present study primarily comes from the presence of hydrothermal alteration signatures. What proportion of crystals show such alteration within any one sample, and what proportion of arc volcanic rocks carry such signatures? Are these regional exceptions or common globally? These questions will need to be addressed by future work.
It is evident from Figure 3a that in the case of the Abu volcanic field, southwest Japan, all olivine mesocrysts have alteration rims. For 234U–238U disequilibria, mineral separates are being analysed, so here the proportion of crystals that carry δ234U excesses cannot be exactly quantified. However, it appears that in New Zealand basalts, the initial δ234U excess is similar across all samples, as the signal decays with increasing eruption age as one would expect (Zellmer et al., 2020). Therefore, the proportion of hydrothermally altered minerals that show δ234U excess can be considered similar, irrespective of eruption location. The easiest explanation for this observation is that all mineral grains of the mineral phase displaying disequilibrium have been altered to a similar degree.
Regarding the proportion of arc rocks that carry such signatures, this question needs further work. We have identified δ234U excesses in mineral separates at many but not all locations. In the Andes, for example, they appear to occur at Callaqui, Lonquimay, and Calbuco, but not at Lascar in the Altiplano desert (Zellmer et al., 2014a). One may speculate that hydrothermal alteration by circulating meteoric waters is less effective in the dry desert environment. Irrespective of such speculations, that some minerals do not show hydrothermal alteration is insufficient evidence that their storage areas were hot, supersolidus mushy environments. Looking at the wide spatial separation of Circum-Pacific volcanic vents studied here that do show evidence of hydrothermal alteration, from Chile through Japan to New Zealand, we would argue that this is a fairly common feature that may have been overlooked, particularly because U-isotope studies are time-consuming and cannot be considered as standard analyses.
Evidence from exposed arc sections
Exposed arc sections provide key insights into the processes operating in transcrustal plumbing systems. We address here the evidence for cumulate formation and the geothermometric constraints that such arc sections yield. However, we also note that exposed arc sections do not provide a snapshot in time but instead yield an integrated history of tens of millions of years of magma flux from the mantle, and their ability to portrait active arc magmatic processes needs to be evaluated with this in mind.
Layered crustal cumulates. There is ample evidence for magmatic differentiation occurring in the overriding crust from the existence of layered cumulates in exposed arc crustal sections at all crustal levels, and specifically in the lowermost crust (e.g. Greene et al., 2006; Jagoutz, 2010; Dessimoz et al., 2012; Walker Jr. et al., 2015; Klein & Jagoutz, 2021). Cumulate formation implies concomitant melt differentiation to more silica-rich composition, which is a clear hallmark of magmatic rather than plutonic systems. Nevertheless, exposed arc crustal sections are typically not well enough preserved to allow quantification of the scale of individual magma reservoirs active at any one time during the evolution of the arc. For example, lower crustal cumulate piles of exposed arc sections do not necessarily imply the existence of a large-scale long-lived active deep crustal hot zone. Plutonic systems do allow for the formation of small magma chambers that during their crystallization differentiate and deposit cumulates, potentially releasing the evolved melt to mid- or upper crustal levels prior to complete solidification. From the perspective of a plutonic system, large cumulate piles are constructed over time by repeated injection of small-scale magma bodies that produce small, laterally discontinuous bodies of cumulates. These merge to larger piles during maturation of the arc crust.
Geothermometry. There is information on the temperature of the mid- to deep crust from mineral thermometry of exposed arc crustal sections. Pyroxenes from Talkeetna gabbronorites give temperatures of 700°C to 930°C (Greene et al., 2006), while those from the Kohistan arc give temperatures near 800°C (Jan & Howie, 1980). In the Kohistan arc, pyroxenes commonly contain exsolution lamellae (Khan et al., 1989), evidence of re-equilibration to low temperatures. These temperatures are clearly higher than 600°C, i.e. higher than the background temperature of the Moho (Whittington et al., 2009), but also clearly lower than 1000°C to 1100°C, which may be a temperature range typical of a mature deep crustal hot zone (Annen et al., 2006). Thus, the geothermometric data suggests that mature arcs do not reach deep crustal hot zone temperatures despite the existence of thick cumulate piles suggesting magmatic differentiation. Temperatures instead may indicate the evolution of a cool lower crust from perhaps 600°C at arc initiation to a warm but still subsolidus lower crust at <1000°C at maturation. This evolution occurs through small-scale magmatic intrusions that rapidly solidify or, if they deposit significant amount of cumulates, release their differentiated residual melts prior to solidification.
Evidence from xenoliths
Scarcity of deep crustal xenoliths. It is uncommon for arc rocks to carry deep crustal xenoliths with unambiguously high-pressure minerals such as garnet, epidote, or Al-rich spinel. Ad hoc, this argues against rapid magma ascent of most arc magmas from the mantle to the surface. However, as arcs mature, repeated injection of magmas into the deep crust will result in a dominance of plutonic rocks and cumulates over the original amphibolite- or granulite-facies ancient lower crustal lithologies that would be the source of such deep crustal xenoliths. Therefore, that such xenoliths are rare is an intrinsic feature of the plutonic system architecture, where magmas pick up crystals from the entire crustal column, including mid- to shallow-crustal lithologies. Further, while uncommon, high pressure mineral phases do exist in some arc rocks, including garnets in andesites and dacites of Miocene volcanics in Northland, New Zealand (Day et al., 1992), and garnets in high-silica arc eruptives from Japan (Kano & Yashima, 1976; Suzuki et al., 1992; Hirayama et al., 2022). Some of these garnets are xenocrystic and derived from xenoliths carried by these rocks, while others are thought to be formed from deep crystallization of silica-rich magmas, pointing to a diversity of deep melt compositions, see below.
Geothermometry. Coexisting pyroxenes from plutonic xenoliths of the Lesser Antilles arc yield temperatures of <800°C to ~1050°C (Arculus & Wills, 1980). Garnet-bearing gabbroic and clinopyroxenitic xenoliths that are thought to have been sourced from cumulates complementary to the Sierra Nevada batholith return garnet-clinopyroxene temperatures of 700°C to 950°C (Ducea & Saleeby, 1996; Ducea et al., 2021). Again, these temperatures are mostly lower than those of mature deep crustal hot zones, and as above we argue for a warm but still subsolidus lower crust in support of a plutonic system architecture.
Origin, chemistry, and thermal properties of the carrier melts
A hallmark of arc magmatic systems is the differentiation of primary basaltic melts within the overriding crust, a paradigm that is over 40 years old (Gill, 1981; Thorpe, 1982). While the production of higher-silica melts through slab melting has been argued for on basis of experimental data (Nichols et al., 1994; Hermann & Spandler, 2007), evidence for the presence of such melts in natural samples is scarce (Eiler et al., 1998; Dallai et al., 2022). A relatively high proportion of such slab melts would be required to exhaust olivine in the hot part of the mantle wedge and result in the production of high-silica magmas through mantle melting, as suggested by more recent experiments (Rebaza et al., 2023). Thus, although slab fluids rather than melts may also carry major oxides including silica (Negulescu & Săbău, 2014), there is still skepticism that high-silica melts can be produced in large enough quantities to be considered a significant contributor melt-flux across the Moho. Their reaction with mantle wedge lithologies is thought to form metasomatised mantle domains (Prouteau et al., 2001; Mallik et al., 2015), but these are then typically not considered to contribute significant amounts of magma flux to the overriding crust. Nevertheless, if silica-rich melts are produced subcrustally from secondary olivine-free lithologies, they may mix with basaltic compositions to form subcrustal intermediates (Straub et al., 2011). While the melt inclusion evidence suggests that truly intermediate primary melts, which would require mixing of mafic and felsic melts in roughly equal proportions, may be the exception (Reubi & Blundy, 2009; Reubi & Müntener, 2022), subcrustal felsic melts may lead to some degree of diversity by mixing with mafic melts, resulting in mafic to intermediate melt compositions. We here discuss potential arguments against the plutonic system architecture, which indeed requires a diversity of melt compositions to be produced in the mantle wedge and to cross the Moho into the overriding crust.
Oxygen isotopes of subcrustally generated high-Si melts. It may be argued that subducting sediments have high δ18O isotope signatures (> 10) and that subcrustal high-silica melts involving subducted sediments would, therefore, also expected to have higher δ18O isotope signatures than typical for arc magmas, including arc rhyolites that typically yield values <8 (Sas et al., 2022). However, the oxygen isotope cycle through subduction zones is not well constrained to date. Firstly, the recent radiogenic isotope evidence suggests that many of the more evolved subcrustal compositions are generated by subduction erosion of lower fore-arc crust, which overwhelm due to their 8:2 or higher relative proportion the isotopic signature of subducting sediments (Straub et al., 2020; Corella Santa Cruz et al., 2023). Lower crustal felsic lithologies may have lower δ18O than subducting sediments. Secondly, any silica-rich material taken into the subduction channel will undergo O-isotope exchange with slab fluids driven off underlying lithologies, including low-δ18O serpentinized mantle lithosphere and the subducting basaltic crust. Recent work has shown that the resulting oxygen isotopic signature of subducted silica-rich rocks can be significantly lowered (Vho et al., 2020). And finally, subduction melanges involved in the genesis of subcrustal compositional magma diversity mix several lithologies, including those with δ18O isotope signatures less than those of subducting sediments. Clearly, more work is required on the oxygen isotope cycle through subduction zones to assess if this parameter can be used to better constrain the genesis of high-silica arc magmas, subcrustal or crustal.
Sustaining the temperature of superheated melts during ascent. Superheating of melts during rapid adiabatic ascent may also be caused by a steeper P/T slope of the melt adiabat than that of the melt liquidus. In the case of the plutonic system, subcrustal melts may also be initially superheated if their composition is rich enough in silica and the source temperature, i.e. the core of the mantle wedge, is hotter (~1250–1300°C, England & Katz, 2010) than their liquidus. A key problem is the sustainment of the high temperature of this melt in the presence of antecrysts that the melt picks up during ascent through the overriding crust. In thermodynamic equilibrium, the crystals carried by the melt will undergo resorption, and due to the latent heat required for silicate dissolution, the temperature of the carrier melt would decrease significantly. Therefore, it may be argued that crystal-bearing melts may not be able to carry significant excess heat or retain super-liquidus temperatures, and thus that such melts would start to crystallize autocrystic minerals. However, we argue that rapid magma ascent in the plutonic system is not an equilibrium process. Crystal resorption, just like crystal growth, are kinetic processes that need time. If magma ascent is rapid enough, there may not be enough time for significant crystal resorption to operate. We note again that resorption in natural silicate melts is typically operating at rates of <1 μm per hour (Cashman, 1990; Hammer & Rutherford, 2002; Couch et al., 2003; Mollard et al., 2012; Zellmer et al., 2016b; Zellmer et al., 2018; Lormand et al., 2020). At typical magma ascent rates of centimetres to tens of meters per second (Petrelli & Zellmer, 2020), antecrysts carried up rapidly from 10 km depth to the surface may see <1 μm resorption. This very small amount of melting will not significantly affect the temperature of the carrier melt. Instead, it will lead to the formation of melt films on grain boundaries of igneous xenoliths and glomerocryst (Holness et al., 2018) and, therefore, aid their mechanical disintegration into individual phenocrysts (sensu Iddings, 1892).
Implications
Turning back to our observations, we summarize the features of magmatic and plutonic systems in Table 1. There are several implications of the occurrence of plutonic systems, and they are all based on a colder thermal structure of the overriding crust compared to traditional magmatic systems. These implications concern magma ascent, volcano monitoring, and economic geology, and the balance between continental crustal growth versus crustal recycling in modern continental subduction zones. The evidence against deep crustal hot zone differentiation of the samples highlighted here suggests that the deep arc crust is often in a subsolidus, cool state (as low as <600°C at the Moho in young arcs, Whittington et al., 2009), that the thermal gradient from deep crustal levels to the surface is less steep than typically presumed in active subduction zone settings, and that thermal spikes by magmatic intrusions are local features that decay rapidly.
Observational features of magmatic and plutonic systems in continental arcs.
Transcrustal System . | Hot magmatic Minor proportions of plutonic rocks . | Cold plutonic Small ephemeral melt or mush pockets . |
---|---|---|
Mineral proportions | Range in mineral proportions across several samples, intermediate compositions show multi-mineralic assemblages | Range of mineral proportions across several samples, some samples show aphyric and monomineralic assemblages at intermediate compositions |
Mineral textures | Each sample will yield fresh mineral cargo without hydrothermal alteration textures. Fe-rich rims on olivines adhere to olivine stoichometry and are free of aluminum and hydrogen. | In some samples, mineral cargo may show hydrothermal alteration textures. Fe-rich rims on olivines are non-stoichiometric, contain significant amounts of aluminum and hydrogen. TEM work may show amorphous material in olivine rims. |
234U/238U isotopes | Within each sample, whole rock, groundmass, and all mineral separates are in 234U–238U equilibrium. | Within some samples, separates are in 234U–238U disequilibrium, while the groundmass is in 234U–238U equilibrium |
Two-pyroxene thermobarometry | Each sample will yield temperatures and pressures that are within the uncertainties of these intensive variables, with roughly Gaussian distributions around a mean. | Some samples will yield invariant temperatures over large pressure ranges. These are pseudo-decompression paths, and neither temperature nor pressures are reliable indicators of the magmatic P–T conditions. |
Pyroxene-melt thermometry | Autocrystic microlites can only yield melt temperatures consistent with or lower than experimental deep crustal hot zone melts T ≤ 1980ºC – 15.6ºC/wt% × SiO2 | Autocrystic microlites can yield melt temperatures higher than experimental deep crustal hot zone melts T > 1980ºC – 15.6ºC/wt% × SiO2 |
Plagioclase-melt hygrometry | Intermediate composition magmas will all be H2O saturated or oversaturated at shallow crustal pressures. | Some intermediate composition magmas will be H2O undersaturated at shallow crustal pressures. |
Transcrustal System . | Hot magmatic Minor proportions of plutonic rocks . | Cold plutonic Small ephemeral melt or mush pockets . |
---|---|---|
Mineral proportions | Range in mineral proportions across several samples, intermediate compositions show multi-mineralic assemblages | Range of mineral proportions across several samples, some samples show aphyric and monomineralic assemblages at intermediate compositions |
Mineral textures | Each sample will yield fresh mineral cargo without hydrothermal alteration textures. Fe-rich rims on olivines adhere to olivine stoichometry and are free of aluminum and hydrogen. | In some samples, mineral cargo may show hydrothermal alteration textures. Fe-rich rims on olivines are non-stoichiometric, contain significant amounts of aluminum and hydrogen. TEM work may show amorphous material in olivine rims. |
234U/238U isotopes | Within each sample, whole rock, groundmass, and all mineral separates are in 234U–238U equilibrium. | Within some samples, separates are in 234U–238U disequilibrium, while the groundmass is in 234U–238U equilibrium |
Two-pyroxene thermobarometry | Each sample will yield temperatures and pressures that are within the uncertainties of these intensive variables, with roughly Gaussian distributions around a mean. | Some samples will yield invariant temperatures over large pressure ranges. These are pseudo-decompression paths, and neither temperature nor pressures are reliable indicators of the magmatic P–T conditions. |
Pyroxene-melt thermometry | Autocrystic microlites can only yield melt temperatures consistent with or lower than experimental deep crustal hot zone melts T ≤ 1980ºC – 15.6ºC/wt% × SiO2 | Autocrystic microlites can yield melt temperatures higher than experimental deep crustal hot zone melts T > 1980ºC – 15.6ºC/wt% × SiO2 |
Plagioclase-melt hygrometry | Intermediate composition magmas will all be H2O saturated or oversaturated at shallow crustal pressures. | Some intermediate composition magmas will be H2O undersaturated at shallow crustal pressures. |
Transcrustal System . | Hot magmatic Minor proportions of plutonic rocks . | Cold plutonic Small ephemeral melt or mush pockets . |
---|---|---|
Mineral proportions | Range in mineral proportions across several samples, intermediate compositions show multi-mineralic assemblages | Range of mineral proportions across several samples, some samples show aphyric and monomineralic assemblages at intermediate compositions |
Mineral textures | Each sample will yield fresh mineral cargo without hydrothermal alteration textures. Fe-rich rims on olivines adhere to olivine stoichometry and are free of aluminum and hydrogen. | In some samples, mineral cargo may show hydrothermal alteration textures. Fe-rich rims on olivines are non-stoichiometric, contain significant amounts of aluminum and hydrogen. TEM work may show amorphous material in olivine rims. |
234U/238U isotopes | Within each sample, whole rock, groundmass, and all mineral separates are in 234U–238U equilibrium. | Within some samples, separates are in 234U–238U disequilibrium, while the groundmass is in 234U–238U equilibrium |
Two-pyroxene thermobarometry | Each sample will yield temperatures and pressures that are within the uncertainties of these intensive variables, with roughly Gaussian distributions around a mean. | Some samples will yield invariant temperatures over large pressure ranges. These are pseudo-decompression paths, and neither temperature nor pressures are reliable indicators of the magmatic P–T conditions. |
Pyroxene-melt thermometry | Autocrystic microlites can only yield melt temperatures consistent with or lower than experimental deep crustal hot zone melts T ≤ 1980ºC – 15.6ºC/wt% × SiO2 | Autocrystic microlites can yield melt temperatures higher than experimental deep crustal hot zone melts T > 1980ºC – 15.6ºC/wt% × SiO2 |
Plagioclase-melt hygrometry | Intermediate composition magmas will all be H2O saturated or oversaturated at shallow crustal pressures. | Some intermediate composition magmas will be H2O undersaturated at shallow crustal pressures. |
Transcrustal System . | Hot magmatic Minor proportions of plutonic rocks . | Cold plutonic Small ephemeral melt or mush pockets . |
---|---|---|
Mineral proportions | Range in mineral proportions across several samples, intermediate compositions show multi-mineralic assemblages | Range of mineral proportions across several samples, some samples show aphyric and monomineralic assemblages at intermediate compositions |
Mineral textures | Each sample will yield fresh mineral cargo without hydrothermal alteration textures. Fe-rich rims on olivines adhere to olivine stoichometry and are free of aluminum and hydrogen. | In some samples, mineral cargo may show hydrothermal alteration textures. Fe-rich rims on olivines are non-stoichiometric, contain significant amounts of aluminum and hydrogen. TEM work may show amorphous material in olivine rims. |
234U/238U isotopes | Within each sample, whole rock, groundmass, and all mineral separates are in 234U–238U equilibrium. | Within some samples, separates are in 234U–238U disequilibrium, while the groundmass is in 234U–238U equilibrium |
Two-pyroxene thermobarometry | Each sample will yield temperatures and pressures that are within the uncertainties of these intensive variables, with roughly Gaussian distributions around a mean. | Some samples will yield invariant temperatures over large pressure ranges. These are pseudo-decompression paths, and neither temperature nor pressures are reliable indicators of the magmatic P–T conditions. |
Pyroxene-melt thermometry | Autocrystic microlites can only yield melt temperatures consistent with or lower than experimental deep crustal hot zone melts T ≤ 1980ºC – 15.6ºC/wt% × SiO2 | Autocrystic microlites can yield melt temperatures higher than experimental deep crustal hot zone melts T > 1980ºC – 15.6ºC/wt% × SiO2 |
Plagioclase-melt hygrometry | Intermediate composition magmas will all be H2O saturated or oversaturated at shallow crustal pressures. | Some intermediate composition magmas will be H2O undersaturated at shallow crustal pressures. |
The brittle-ductile transition of plutonic systems is thus deeper in the crust than in the case of magmatic systems, potentially as deep as the Moho itself, or perhaps even deeper if mantle lithosphere is not entirely removed through asthenospheric corner flow in the mantle wedge. This brittle regime facilitates magma ascent through fracture propagation. Magma batches may thus propagate through the crust without requiring large buoyancy contrasts to crustal basement rocks. Magma batches that traverse the crust are small in volume, and their intrusion level or eruption at the surface is controlled by the propagation of crustal fractures, i.e., ultimately by tectonism.
In terms of volcanic hazard mitigation, plutonic systems are a more challenging environment to monitor than magmatic systems. The latter provide opportunities to monitoring shallow crustal unrest with local small-aperture seismic and geodetic networks, as volcanism will be controlled mostly by magmatic processes in shallow crustal mush zones that are ductile and prevent deep magmas from directly breaching the surface. In contrast, brittle plutonic systems may feed volcanism through rapid direct melt ascent from the mantle through the crust, with shallow geophysical monitoring signals detectable only at the onset of eruption, without significant warning times. To increase the evacuation time windows between geophysical unrest and volcanism, the location of deep melt movement needs to be monitored, requiring wide aperture and highly sensitive monitoring networks. It may be possible that data from broadband seismic networks typically used to monitor tectonic crustal fracturing can be used by volcano seismologists to monitor and track deep crustal movements of liquids (fluids or melts). This should at least be attempted.
The cooler thermal structure of plutonic systems also has significant implications for the formation of economic mineral deposits (the exploration of which is required to power future low-carbon economies), because it determines where and how metals are concentrated and remobilized (Candela & Holland, 1986; de Ronde et al., 2011; Jego et al., 2016; Brandl et al., 2020; Blundy et al., 2021). For example, metal solubility in sub-volcanic brines is highly temperature-dependent, and metal precipitation will, therefore, not only depend on brine salinity but also on the prevalent geothermal gradient in the subvolcanic crust. In hot magmatic systems, precipitation of metals will be restricted to shallow crustal levels, while cooler, plutonic systems will allow brine exsolution deeper. Thus, gaining an understanding of the thermal structure of the upper crust will have direct implications for the prospecting of economic mineral resources. Further, the reduced need for crustal level differentiation processes in the envisaged plutonic systems removes the necessity of the removal of ultramafic cumulates from the base of a deep crustal hot zone back into the mantle. Such cumulates would be expected to also remove significant amounts of metal sulfides, a process not operating in the envisaged plutonic systems, where crustal level-concentration of metal sulfides may form high-grade deposits.
Beyond these considerations of immediate relevance to society, the prevalence of plutonic systems has fundamental implications regarding the balance between crustal growth and crustal recycling on Earth through the evolution of this planet. The presence of subduction zones in Hadean times is debated, but subduction and the juxtaposition of fragments of continental crust through plate tectonics likely operated since about 3 Ga ago (Hawkesworth et al., 2020). Initially, the subduction of mafic sediments would have dominated the material overlying the subducting mafic crust into the mantle, and the separation and ascent through the overriding crust of primary melts other than basaltic to basaltic-andesitic may have been rare. Then, deep crustal hot zone processes such as fractional crystallization and partial melting of previous deep crustal intrusives may have been a key process of the generation of more felsic melts in early subduction zone environments. As the continental crust became more evolved through time, sediments eroded from the crust also did. Further, subduction erosion of forearc crust, which has been shown to be a major contributor to melange formation subduction zones (Straub et al., 2020), would have increasingly provided more silica-rich materials to these melanges. As a consequence, relamination of felsic melts to the lower crust (Castro & Gerya, 2008) would have become more prevalent. Concomitant crustal cooling would have deepened the brittle-ductile transition, ultimately allowing ascent of aphyric melts of a range of compositions from mantle depths to the surface to feed modern arc volcanism in continental subduction zones. The scenario described here sees continental crust of bulk intermediate composition initially generated through crustal level differentiation mechanisms in early subduction zones, transitioning with time to a setting where crustal recycling dominates, though erosion of fore-arc crustal material and its transfer to the arc crust. The ratio of crustal generation to crustal recycling would thus have decreased over time, starting in the Archean. At present, crustal recycling appears to dominate by far in continental subduction zones, and it is questionable if new continental crust is still generated in these settings. Oceanic subduction zone settings might perhaps be more important in generating new continental crust at present.
Future work
This study has addressed the genesis of mafic to intermediate magmas in continental subduction zone settings, indicating that in many cases, we are dealing with cool plutonic systems. Supersolidus magmatic mushes in these systems are spatially restricted and ephemeral, representing the rapid transition of small melt pockets to solid plutonic rocks. In contrast, canonical magmatic systems are large scale, dominated by supersolidus mushes and melt lenses, perhaps with a few interspersed regions of solid plutonic wall rocks. Clearly, these are endmember concepts governed by the crustal thermal structure, and ultimately by the magma supply rate into the overriding crust (Karakas et al., 2017; Glazner, 2021).
Future work on crystal-scale evidence is required to distinguish between magmatic and plutonic systems, rather than assuming one or the other. It should address at what rates of magma supply a plutonic system will transition to a magmatic system and vice versa. The existing thermal models suggest that magmatic systems cannot be sustained over long time periods through mafic intrusions into the upper crust. Future numerical models might consider the injection of larger volumes of more evolved melts over short time periods. Some may argue that the existence of large rhyolitic caldera centres implies that magmatic systems are prevalent in continental arc environments, but it may be questioned if the existence of large-scale magmatic mush zones in the shallow upper crust are long-lived (long repose times for high-volume rhyolite eruptions and the thermal constraints argue against this) and whether their existence automatically implies that the mid- to lower crust must also be largely supersolidus and mushy in nature. In fact, some rhyolitic magmas may be generated in the mantle wedge (Dallai et al., 2022) and may traverse the mafic deep to middle crust rapidly, to be emplaced at shallow level without significant differentiation occurring prior to eruption. Recent high-precision isotope work of Corella Santa Cruz et al. (2023) suggests that dacites and some rhyolites erupted from the Taupo Volcanic Zone may be partial melts of fore-arc crust recycled through the mantle wedge (and overriding crust) by subduction erosion and melange formation. TVZ rhyolite samples demonstrate again a great variety in mineralogy and mineral content. In terms of crystallinity, they range from aphyric to sub-aphyric post-caldera obsidian domes near Taupo (Moore, 2011), through sparsely phyric (2–5%) Taupo ignimbrites erupted during the last c. 15 kyr (Smith et al., 2005), to typically more crystal-rich (up to 40%) older Taupo and Okataina ignimbrites (Smith et al., 2005). The mineralogy of phyric rhyolites is equally varied, with quartz, plagioclase, orthopyroxene, clinopyroxene, hornblende, cummingtonite and biotite variably present or absent (while oxides appear to be present always; Smith et al., 2005). Further work should be done on the mineralogy of such rhyolites and the origin of their crystal cargo, without an ad hoc assumption that they are erupted from the top of canonical magmatic systems and that their crystal cargo was stored in mush zones at supersolidus temperatures for long time periods. Perhaps canonical magmatic systems are in fact very uncommon, only existent during very short (in comparison to the lifetime of subduction zones) periods of ignimbrite flare-up (Gravley et al., 2016)? Future work should investigate this matter.
Finally, here we have only addressed volcanoes from continental subduction zones, yet many arcs of the western-Pacific are of oceanic nature. One may hypothesise that subduction melanges may play less of a role in terms of the genesis of rhyolitic rocks in these oceanic settings. Perhaps canonical magmatic systems are required to produce rhyolitic eruptives generated by crustal AFC processing and repeated partial melting of previous intrusives, e.g. in deep crustal hot zones or MASH reservoirs. Yet again, this is merely a hypothesis, which will need to be tested. One should investigate the petrology and mineral chemistry of volcanic products of oceanic arcs carefully before jumping to conclusions. This study has provided some avenues of potential investigation, and it is still too early to judge whether modern oceanic arcs are not also dominated by non-canonical plutonic systems, perhaps transitioning into supersolidus magmatic systems only intermittently, during ignimbrite flare-ups occurring over short time periods (< 100kyr, cf. Gravley et al., 2016). In fact, the magmatic temperatures inferred from dacitic Tongan pumice rafts, plotted on Figure 11, suggests that they may be too hot for lower crustal genesis, indicating that subcrustal genesis of evolved rocks may be occurring in modern subduction zone settings irrespective of the composition and thickness of the overriding crust. We note that on the basis of isotopic data, Timm et al. (2014) suggested subduction melange diapirism to operate in the oceanic Kermadec arc, in line with the notion that subcrustal genesis of felsic melts may extent to the oceanic arc environment.
CONCLUSIONS
This study has adopted the concept of transcrustal plutonic systems, recently proposed for the southern Taupo Volcanic Zone, for circum-Pacific continental arcs in general. Here, small magma batches rapidly freeze, magmatic mushes are spatially restricted and ephemeral, and the crystal cargo of volcanic eruption products is dominantly remobilized from solid plutonic rocks. This contrasts with the canonical supersolidus transcrustal magmatic systems, characterized by large-scale magmatic mush zones, where crystal frameworks host interstitial melts, and where the crystal cargo of volcanic eruption products is dominated by crystals grown and remobilized from mushy reservoirs that feed the volcanism.
We argued that mafic to intermediate Circum-Pacific continental arc magmas support the prevalence of transcrustal plutonic systems in many cases, based on: (i) their variable mineral assemblages and mineral proportions, some inconsistent with representing mush frameworks in equilibrium with erupted melts; (ii) hydrous rim formation on nominally anhydrous minerals, indicating subsolidus hydrothermal alteration; (iii) uranium isotope disequilibria between minerals and matrix, again indicating hydrothermal alteration of the mineral cargo; and (iv) two-pyroxene thermobarometry yielding pseudo-decompression paths (PDPs) that cannot be explained through mixing of magmas carrying minerals but instead indicate the disintegration of solid plutonic precursors to form the crystal cargo of arc eruptives.
We showed that many mafic to intermediate continental arc magmas cannot be generated in deep crustal and mushy hot zones, because mineral thermometric and hygrometric constraints suggest that natural magmas are too hot and not hydrous enough compared to experimental run products that simulate the deep crustal hot zone evolution of hydrous arc basalts. We propose instead that felsic magmas are generated subcrustally.
We outlined that the prevalence of transcrustal plutonic systems has profound implications through their cooler crustal structure on crustal magma ascent, volcanic hazard monitoring, economic geology, and more fundamentally the balance between continental crustal growth and recycling, and how this balance may have shifted through deep time.
We provided avenues for future research that should elucidate if transcrustal plutonic systems may also govern silicic magmatism and volcanism, as well as the magmatism and volcanism in oceanic subduction zones.
ACKNOWLEDGEMENTS
We thank José Cembrano, Jorge Clavero, Andres Veloso, and Gerd Sielfeld for assistance with fieldwork at Andean arc volcanoes, and Magdalena Blum-Oeste, André Stechern, and Gerhard Wörner for useful insights into intrinsic parameters governing Andean magmatism at the eruptive centres discussed here. Sasha Belousov provided the sample from Mt. Hunglu in the Tatun Volcano Group of northern Taiwan. We thank Leslie Hale for assistance with access to the Smithsonian sample collection of rocks from the Cascades. Ya-Ting Hsu and Rong-Yi Yan helped with sample processing, and Hui-Ho Hsieh with EPMA analysis. Penny Wieser is acknowledged for making the Python3 tool Thermobar (v.0.07) available. We thank Silvio Mollo for discussions around clinopyroxene-melt (dis)equilibria, and Marian Holness for insights regarding the melting of xenoliths. We would also like to thank many of our colleagues in Japan, Taiwan, and New Zealand for the technical assistance during laboratory analytical work and the scientific discussions over the last decade, which ultimately led to the concepts presented in this study. Finally, we thank Tom Sisson and three anonymous reviewers for their very insightful and constructive feedback that has significantly improved this perspective, and Jörg Hermann for thorough editorial handling of this paper.
SUPPLEMENTARY DATA
Supplementary data are available at Journal of Petrology online.
FUNDING
GFZ acknowledges funding through a Catalyst Seeding Grant (CSG-MAU1901) and a Smart Ideas Grant from the Ministry of Business, Innovation and Employment (MAUX2202).
DATA AVAILABILITY
The data in this article are available in the article and in its online supplementary material.