-
PDF
- Split View
-
Views
-
Cite
Cite
Yan Xiao, Meng Yuan, Ben-Xun Su, Chen Chen, Yang Bai, Shan Ke, Yang Sun, Paul T Robinson, The Chromite Crisis in the Evolution of Continental Magmas and the Initial High δ26Mg Reservoir, Journal of Petrology, Volume 64, Issue 4, April 2023, egad019, https://doi.org/10.1093/petrology/egad019
- Share Icon Share
Abstract
Fractional crystallization of Fe–Ti oxides can induce detectable Mg isotopic changes during late-stage basalt differentiation. Because chromite and olivine are early crystallizing phases during basaltic melt differentiation, the effect of chromite crystallization on the fractionation of Mg isotopes during early-stage basalt differentiation is still poorly understood. Here, we examine the possibility of chromite induced Mg isotope fractionation with a Mg isotopic study of chromite–olivine pairs in dunites and chromitites collected from major types of basaltic intrusive rock suites formed by fractional crystallization in different tectonic settings. The chromite δ26Mg (= [(26Mg/24Mg)sample / (26Mg/24Mg)DSM3–1] × 1000) values range from −0.19‰ to 0.30‰ in the Luobusa ophiolite, −0.09‰ to 0.78‰ in the Kızıldağ ophiolite, −0.04 to 0.42‰ in the Gaositai Alaskan-type complex, similar to those previously reported from the Stillwater layered intrusion (−0.05 to 0.84‰; Bai et al., 2021). They are significantly higher than those of coexisting olivine (δ26Mg = −0.48 to −0.10‰). The Δ26MgChr-Ol (= δ26MgChr − δ26MgOl) values in the rock suites investigated here fall largely between equilibrium fractionation lines of spinel–olivine pairs and magnesioferrite–olivine pairs, indicating equilibrium Mg isotopic fractionation. Furthermore, the Δ26MgChr-Ol values increase with decreasing Cr content of chromite in the dunites and chromitites, showing that high 26Mg has a greater affinity to Al-rich chromite than Cr-rich chromite. Fractional crystallization of such isotopically high chromite is expected to progressively lower the Mg isotope values of the in the remaining magma. Furthermore, continental basaltic magmas typically experience early crystallization of olivine and Al-rich chromite. Their δ26Mg values correlate positively with MgO (FeO, Cr,) and CaO/Al2O3 ratios and negatively with total alkali contents (Na2O + K2O). This indicates that detectable Mg isotopic fractionation occurred in intra-continental basalt magmas, probably by fractional crystallization of olivine and chromite. The observed low-δ26Mg intra-continental basalts can be accurately modeled by olivine + chromite fractionation with fractionation factors (Δ26MgChr-Melt) of 0.20‰, 0.60‰, and 1.18‰ as observed in the chromitites investigated during this study. Therefore, the early-stage basaltic melt differentiation involving separation of olivine and chromite may induce resolvable Mg isotopic fractionation, and the δ26Mg values of continental basalts should be used with caution in petrogenetic studies.
INTRODUCTION
Most of Earth’s oceanic crust returns to the mantle by subduction, generating significant mantle heterogeneity (e.g. Dasgupta & Hirschmann, 2010; van Keken et al., 2011; Ryan & Chauvel, 2014). Magnesium (Mg) isotopes can be used as tracers of recycled crustal materials, particularly those associated with sedimentary carbonates (e.g. Li et al., 2017), which have an extremely low Mg isotopic signatures (−5.60 to −0.66‰; e.g. Beinlich et al., 2014; Saenger & Wang, 2014) compared to dominant silicate minerals in the mantle (e.g. olivine and pyroxene) that have homogeneous δ26Mg (= [(26Mg/24Mg)sample / (26Mg/24Mg)DSM3–1] × 1000) values of −0.25 ± 0.04‰ (Teng et al., 2010). Therefore, low δ26Mg values (as low as −0.60‰) observed in many intra-continental basalts have been interpreted as reflecting incorporation of carbonates in their sources (Yang et al., 2012; Huang et al., 2015; Liu et al., 2015; Tian et al., 2016, 2018; Wang et al., 2016; Li et al., 2017; Cheng et al., 2018).
Many alkaline basaltic magmas could have experienced protracted differentiation, involving a variety of Mg-bearing oxides with distinctly different δ26Mg values (chromite, ilmenite, and titanomagnetite; Jeffery & Gertisser, 2018). Crystallization of these oxides may cause the isotopic compositions of basaltic rocks to deviate from those of their mantle sources (e.g. Su et al., 2019; Wang et al., 2021). For example, late-stage differentiation of basaltic magmas involving separation of Fe–Ti oxides could induce resolvable Mg isotopic fractionation (Wang et al., 2021). Chromite is a common oxide mineral in which Mg2+ is present as a major element in its general formula, [(Mg, Fe)IV(Al, Cr)2VIO4]. In this early crystallizing phase, 4-coordinated Mg has stronger Mg–O bonds and thus higher δ26Mg values than olivine (and pyroxene) with 6-coordinated Mg (e.g. Δ26MgSpl-Ol = 0.59‰ at 1000°C), as predicted by theoretical calculations (Schauble, 2011) and observed in natural peridotites (Young et al., 2009; Liu et al., 2011; Xiao et al., 2013). It may form earlier than, concurrently with, or later than olivine, and prior to clinopyroxene and ilmenite during crystallization of basaltic magmas (Kamenetsky et al., 2001). In addition, it has been proposed that chromite fractionation may play an important role in creating the low-δ26Mg values of intra-continental basalts, as suggested by negative correlations between basalt δ26Mg and indices of chromite crystallization (e.g. Cr, V, FeO; Su et al., 2019). However, this proposal remains equivocal and needs to be confirmed by further.
Chromite occurs widely in mafic-ultramafic rocks both as discrete grains and as massive bodies of chromitite ore. Chromitites are typically associated with dunite and occur either as podiform bodies in ophiolites or as stratiform(-like) layers in Alaskan-type complexes and other layered intrusions. In intrusive bodies they are considered to be magmatic cumulate rocks formed during the early stages of basaltic melt differentiation (Irvine, 1977; Zhou et al., 1994). In ophiolites, they are also considered to be magmatic in origin but not necessarily directly related to their host rocks. To constrain the degree of Mg isotope fractionation between basaltic magmas and crystallized chromite, we conducted a systematic investigation of olivine–chromite pairs collected from cumulate rock suites in the ophiolites and Alaskan-type complexes, and compare them to published data from dunites and chromitites of the Stillwater layered intrusion (Bai et al., 2021). The objective of our study is to demonstrate that the fractionation of Mg isotopes during the early differentiation of chromite is not limited to layered intrusions and is, instead, a process that may operate during the early stages of fractional crystallization of intra-continental basalts in general.
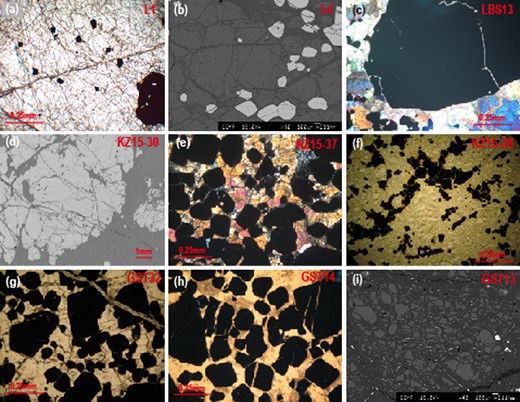
Photomicrographs and backscattered electron micrographs of representative dunites and chromitites in the Luobusa (a–c) and Kızıldağ ophiolites (d–f), and Gaositai Alaskan-type complex (g–i). (a), (f), (g), and (h) are plane-polarized light. (c) and (e) are cross-polarized light. (a, b, e, g, h), disseminated chromitite; (c, d) nodular chromitite; (f, i), dunite with cumulus texture.
SAMPLES
In this study, we analyzed chromite and olivine separates from three suites of cumulate mafic rock sequences that are geographically dispersed and chemically diverse, which included 14 chromitites from the Luobusa ophiolite in southern Tibet, 6 dunites and 16 chromitites from the Kızıldağ ophiolite in SE Turkey, and 2 dunites and 9 chromitites from the Gaositai Alaskan-type complex on the northern margin of the North China Craton. Brief descriptions of these different ultramafic rock suites are given below.
Luobusa and Kızıldağ ophiolites
The Luobusa ophiolite in southern Tibet is a fragment of Neo-Tethyan oceanic lithosphere that hosts the largest chromite deposit in China (Zhou et al., 1996, 2005). It consists of a harzburgitic mantle sequence, a paleo-Moho dunitic transition zone and a mélange zone (Zhou et al., 1996, 2005; Zhang et al., 2019). The chromite ore bodies are enveloped by dunites and distributed in the mantle sequence. They are crystal cumulates filling magma conduits in a suprasubduction zone setting (Lago et al., 1982; Zhou et al., 1996). The dunites consist of olivine (> 90%) with minor chromite and anhedral clinopyroxene. Chromitites from Luobusa analyzed in this study are composed of coarse-grained chromite (20–80 vol%) in an olivine matrix (Xiao et al., 2016). They have various textures from disseminated to nodular and massive (Fig. 1a–c).
The Kızıldağ ophiolite in SE Turkey has been interpreted as a remnant slice of Neo-Tethyan oceanic lithosphere emplaced onto the Arabian continental margin during the late Cretaceous (e.g. Parlak et al., 2009). It contains a complete sequence of mantle tectonite, ultramafic to mafic cumulate rocks, isotropic gabbro, sheeted dikes, plagiogranite, and a volcanic complex. Samples analyzed in this study include stratiform-like bodies of chromitite and cumulate dunite that originated in the Moho transition zone. The chromitite samples are mostly disseminated, banded, and massive (Fig. 1d–f). Their petrological characteristics have been reported in detail by Chen et al. (2015, 2019).
Gaositai Alaskan-type complex
The Gaositai intrusion, located on the northern margin of the North China Craton (Chen et al., 2009), is a typical Alaskan-type complex, concentrically zoned with dunite in the core, rimmed successively by wehrlite, clinopyroxenite, and hornblendite (Chen et al., 2009; Tian et al., 2011). The complex is believed to have formed by fractional crystallization of arc magmas above a subduction zone (Chen et al., 2009; Tian et al., 2011). Olivine in the central dunite core is coarse-grained, but becomes medium- to fine-grained with distance from the center. Subhedral to euhedral chromite grains are the dominant accessory mineral in the dunite. Platinum-bearing chromite schlieren, veinlets, and massive chromitites within the coarse-grained dunite are composed of chromite (20–80 vol%) and olivine (Wang et al., 1983). Samples analyzed in this study are coarse-grained dunites and disseminated and massive chromitites (Fig. 1g–i).
ANALYTICAL METHODS
Major element analysis of chromite and olivine, and temperature estimates
Major element compositions of chromite and olivine were determined using a JEOL JXA8100 electron probe micro-analyzer (EPMA) at the Institute of Geology and Geophysics, Chinese Academy of Sciences (IGGCAS). The analyses were carried out at an accelerating voltage of 15 kV, 10 nA beam current, 5 μm beam spot and 10–30 s peak counting time. Natural minerals and synthetic oxides used for calibration included diopside (Ca, Si, and Mg), albite (Na and Al), rutile (Ti), bustamite (Mn), K-feldspar (K), NiO (Ni), Fe2O3 (Fe), and Cr2O3 (Cr). Detection limits (1σ) were ~0.02 wt% for Mg, Al, Cr, K, Si, Mn, Ca, and Fe; and ~0.03 wt% for Ni and Ti. A ZAF-based program (Armstrong, 1995) was used for matrix corrections (Table 1). The Fe3+/∑Fe ratio of chromite was calculated based on microprobe analyses using stoichiometric criteria (Droop, 1985).
Major oxide (wt.%) compositions of chromite and olivine in the dunites and chromitites from Luobusa and Kızıldağ ophiolites, and Gaositai Alaskan-type complex
Locality | Luobusa | |||||||||||||||||||||
Sample | LBS09005–8 | LBS13–21 | LBS13–22 | LBS13–23 | LBS13–34 | LBS13–39 | L1 | L2 | L4 | L3 | L5 | L6 | L7 | L06a | ||||||||
Rock type | Chromitite | Chromitite | Chromitite | Chromitite | Chromitite | Chromitite | Chromitite | Chromitite | Chromitite | Chromitite | Chromitite | Chromitite | Chromitite | Chromitite | ||||||||
Mineral | Chra | Ola | Chr | Chr | Chr | Chr | Chr | Chr | Ol | Chr | Ol | Chr | Ol | Chr | Ol | Chr | Ol | Chr | Chr | Chr | ||
Point | 8 | 8 | 8 | 8 | 8 | 8 | 8 | 8 | 8 | 8 | 8 | 8 | 8 | 8 | 8 | 8 | 8 | 8 | 8 | 8 | ||
SiO2 | 0.00 | 41.81 | 0.05 | 0.00 | 0.03 | 0.01 | 0.01 | 0.02 | 42.0 | 0.00 | 42.0 | 0.03 | 42.1 | 0.00 | 41.8 | 0.02 | 42.1 | 0.00 | 0.00 | 0.00 | ||
TiO2 | 0.23 | 0.00 | 0.21 | 0.20 | 0.13 | 0.19 | 0.23 | 0.18 | 0.03 | 0.19 | 0.02 | 0.19 | 0.01 | 0.20 | 0.01 | 0.22 | 0.00 | 0.19 | 0.16 | 0.22 | ||
Al2O3 | 10.4 | 0.00 | 11.0 | 11.9 | 10.3 | 13.1 | 11.0 | 10.2 | 0.00 | 10.7 | 0.00 | 10.6 | 0.00 | 12.4 | 0.01 | 11.1 | 0.00 | 10.9 | 12.8 | 11.6 | ||
Cr2O3 | 61.5 | 0.02 | 60.0 | 58.8 | 60.5 | 58.7 | 60.8 | 59.4 | 0.01 | 59.3 | 0.02 | 59.2 | 0.02 | 57.9 | 0.01 | 59.8 | 0.02 | 60.1 | 57.5 | 61.2 | ||
FeO | 16.3 | 4.69 | 14.6 | 15.8 | 15.2 | 12.8 | 12.0 | 15.4 | 4.34 | 14.6 | 4.43 | 13.4 | 3.17 | 13.6 | 3.59 | 13.5 | 3.52 | 12.8 | 13.5 | 12.4 | ||
MnO | 0.15 | 0.08 | 0.22 | 0.23 | 0.22 | 0.20 | 0.20 | 0.13 | 0.07 | 0.11 | 0.03 | 0.09 | 0.02 | 0.11 | 0.04 | 0.10 | 0.04 | 0.10 | 0.08 | 0.05 | ||
MgO | 13.2 | 53.0 | 15.2 | 13.3 | 13.4 | 16.0 | 16.5 | 14.1 | 53.3 | 14.4 | 53.2 | 15.7 | 53.7 | 15.2 | 53.6 | 15.7 | 54.0 | 15.6 | 15.7 | 16.0 | ||
CaO | 0.01 | 0.04 | 0.00 | 0.00 | 0.00 | 0.01 | 0.00 | 0.00 | 0.00 | 0.01 | 0.01 | 0.01 | 0.05 | 0.00 | 0.02 | 0.01 | 0.05 | 0.01 | 0.00 | 0.02 | ||
Na2O | 0.02 | 0.01 | 0.01 | 0.00 | 0.02 | 0.00 | 0.01 | 0.05 | 0.00 | 0.01 | 0.01 | 0.04 | 0.01 | 0.01 | 0.00 | 0.01 | 0.00 | 0.01 | 0.01 | 0.00 | ||
K2O | 0.00 | 0.00 | 0.00 | 0.00 | 0.00 | 0.00 | 0.00 | 0.01 | 0.01 | 0.01 | 0.00 | 0.01 | 0.01 | 0.00 | 0.00 | 0.01 | 0.01 | 0.01 | 0.01 | 0.00 | ||
NiO | 0.09 | 0.53 | 0.10 | 0.12 | 0.04 | 0.15 | 0.20 | 0.10 | 0.49 | 0.10 | 0.53 | 0.14 | 0.58 | 0.12 | 0.67 | 0.13 | 0.61 | 0.17 | 0.15 | 0.17 | ||
Total | 102 | 100 | 101 | 100 | 99.8 | 101 | 101 | 99.4 | 100 | 99.3 | 100 | 99.3 | 100 | 99.5 | 100 | 100 | 100 | 99.7 | 99.7 | 101 | ||
Si | 0.00 | 1.00 | 0.00 | 0.00 | 0.00 | 0.00 | 0.00 | 0.00 | 1.00 | 0.00 | 1.00 | 0.00 | 1.00 | 0.00 | 1.00 | 0.00 | 1.00 | 0.00 | 0.00 | 0.00 | ||
Ti | 0.01 | 0.00 | 0.00 | 0.00 | 0.00 | 0.00 | 0.01 | 0.00 | 0.00 | 0.00 | 0.00 | 0.00 | 0.00 | 0.00 | 0.00 | 0.01 | 0.00 | 0.00 | 0.00 | 0.01 | ||
Al | 0.39 | 0.00 | 0.41 | 0.45 | 0.39 | 0.48 | 0.38 | 0.38 | 0.00 | 0.41 | 0.00 | 0.40 | 0.00 | 0.46 | 0.00 | 0.41 | 0.00 | 0.41 | 0.47 | 0.42 | ||
Cr | 1.54 | 0.00 | 1.49 | 1.48 | 1.54 | 1.44 | 1.55 | 1.51 | 0.00 | 1.50 | 0.00 | 1.49 | 0.00 | 1.45 | 0.00 | 1.49 | 0.00 | 1.51 | 1.43 | 1.50 | ||
Fe3+ | 0.06 | 0.09 | 0.06 | 0.06 | 0.07 | 0.06 | 0.10 | 0.08 | 0.11 | 0.08 | 0.09 | 0.08 | 0.09 | 0.06 | ||||||||
Fe2+ | 0.37 | 0.09 | 0.29 | 0.36 | 0.35 | 0.26 | 0.26 | 0.32 | 0.09 | 0.31 | 0.09 | 0.25 | 0.06 | 0.28 | 0.07 | 0.26 | 0.07 | 0.26 | 0.26 | 0.26 | ||
Mn | 0.00 | 0.00 | 0.01 | 0.01 | 0.01 | 0.01 | 0.00 | 0.00 | 0.00 | 0.00 | 0.00 | 0.00 | 0.00 | 0.00 | 0.00 | 0.00 | 0.00 | 0.00 | 0.00 | 0.00 | ||
Mg | 0.62 | 1.89 | 0.71 | 0.63 | 0.64 | 0.74 | 0.73 | 0.68 | 1.90 | 0.69 | 1.89 | 0.74 | 1.91 | 0.72 | 1.91 | 0.74 | 1.91 | 0.74 | 0.73 | 0.74 | ||
Ca | 0.00 | 0.00 | 0.00 | 0.00 | 0.00 | 0.00 | 0.00 | 0.00 | 0.00 | 0.00 | 0.00 | 0.00 | 0.00 | 0.00 | 0.00 | 0.00 | 0.00 | 0.00 | 0.00 | 0.00 | ||
Na | 0.00 | 0.00 | 0.00 | 0.00 | 0.00 | 0.00 | 0.00 | 0.00 | 0.00 | 0.00 | 0.00 | 0.00 | 0.00 | 0.00 | 0.00 | 0.00 | 0.00 | 0.00 | 0.00 | 0.00 | ||
K | 0.00 | 0.00 | 0.00 | 0.00 | 0.00 | 0.00 | 0.00 | 0.00 | 0.00 | 0.00 | 0.00 | 0.00 | 0.00 | 0.00 | 0.00 | 0.00 | 0.00 | 0.00 | 0.00 | 0.00 | ||
Ni | 0.00 | 0.01 | 0.00 | 0.00 | 0.00 | 0.00 | 0.01 | 0.00 | 0.01 | 0.00 | 0.01 | 0.00 | 0.01 | 0.00 | 0.01 | 0.00 | 0.01 | 0.00 | 0.00 | 0.00 | ||
Total | 3.00 | 3.00 | 3.00 | 3.00 | 3.00 | 3.00 | 3.00 | 3.00 | 3.00 | 3.00 | 3.00 | 3.00 | 3.00 | 3.00 | 3.00 | 3.00 | 3.00 | 3.00 | 3.00 | 3.00 | ||
Mg#b | 62.5 | 95.3 | 71.1 | 63.6 | 64.7 | 74.4 | 74.7 | 68.2 | 95.7 | 68.9 | 95.6 | 74.8 | 96.8 | 72.0 | 96.4 | 73.7 | 96.5 | 74.1 | 73.6 | 74.4 | ||
Cr#b | 79.8 | 78.6 | 76.9 | 79.7 | 75.0 | 78.8 | 79.7 | 78.8 | 78.9 | 75.8 | 78.3 | 78.8 | 75.1 | 78.0 | ||||||||
Fe3+/∑Feb | 0.13 | 0.25 | 0.14 | 0.14 | 0.23 | 0.18 | 0.23 | 0.21 | 0.30 | 0.22 | 0.26 | 0.24 | 0.26 | 0.20 | ||||||||
SiO2 | 0.05 | 41.6 | 0.02 | 42.0 | 0.03 | 41.4 | 0.02 | 41.6 | 0.05 | 40.8 | 0.00 | 41.5 | 0.03 | 41.6 | 0.00 | 41.6 | 0.02 | 42.1 | 0.10 | 41.4 | 0.06 | 41.5 |
TiO2 | 0.15 | 0.00 | 0.06 | 0.05 | 0.28 | 0.00 | 0.18 | 0.00 | 0.05 | 0.02 | 0.16 | 0.03 | 0.36 | 0.00 | 0.36 | 0.00 | 0.23 | 0.03 | 0.27 | 0.00 | 0.39 | 0.04 |
Al2O3 | 15.6 | 0.00 | 22.6 | 0.01 | 16.9 | 0.00 | 24.8 | 0.00 | 27.3 | 0.00 | 21.8 | 0.00 | 20.9 | 0.00 | 20.3 | 0.00 | 15.1 | 0.01 | 21.3 | 0.01 | 20.3 | 0.01 |
Cr2O3 | 50.2 | 0.00 | 41.9 | 0.00 | 51.3 | 0.02 | 42.5 | 0.04 | 37.6 | 0.06 | 46.0 | 0.14 | 44.9 | 0.00 | 44.5 | 0.00 | 53.4 | 0.04 | 44.3 | 0.01 | 46.4 | 0.00 |
FeO | 22.4 | 7.23 | 23.4 | 8.99 | 19.0 | 7.11 | 18.6 | 8.95 | 20.8 | 8.33 | 18.7 | 8.64 | 21.9 | 9.52 | 23.9 | 9.52 | 16.6 | 5.91 | 21.5 | 9.03 | 17.9 | 7.48 |
MnO | 0.22 | 0.12 | 0.17 | 0.10 | 0.16 | 0.12 | 0.13 | 0.14 | 0.00 | 0.09 | 0.00 | 0.14 | 0.21 | 0.13 | 0.18 | 0.13 | 0.13 | 0.06 | 0.22 | 0.11 | 0.16 | 0.11 |
MgO | 10.7 | 51.6 | 12.0 | 49.9 | 12.7 | 49.4 | 13.5 | 49.2 | 13.3 | 49.3 | 12.6 | 49.4 | 12.1 | 49.0 | 11.3 | 49.0 | 14.5 | 51.1 | 12.1 | 48.9 | 13.9 | 51.0 |
CaO | 0.03 | 0.11 | 0.00 | 0.11 | 0.00 | 0.21 | 0.00 | 0.04 | 0.00 | 0.03 | 0.03 | 0.12 | 0.00 | 0.07 | 0.02 | 0.07 | 0.00 | 0.15 | 0.04 | 0.07 | 0.03 | 0.02 |
Na2O | 0.00 | 0.00 | 0.01 | 0.00 | 0.00 | 0.01 | 0.00 | 0.00 | 0.00 | 0.00 | 0.00 | 0.00 | 0.04 | 0.00 | 0.00 | 0.00 | 0.00 | 0.00 | 0.03 | 0.00 | 0.03 | 0.01 |
K2O | 0.00 | 0.00 | 0.00 | 0.00 | 0.00 | 0.00 | 0.00 | 0.02 | 0.00 | 0.00 | 0.00 | 0.00 | 0.00 | 0.01 | 0.00 | 0.01 | 0.00 | 0.00 | 0.00 | 0.00 | 0.00 | 0.00 |
NiO | 0.07 | 0.39 | 0.13 | 0.38 | 0.08 | 0.42 | 0.16 | 0.34 | 0.13 | 0.39 | 0.11 | 0.37 | 0.11 | 0.36 | 0.12 | 0.36 | 0.13 | 0.43 | 0.10 | 0.33 | 0.12 | 0.38 |
Total | 99.5 | 101 | 100 | 101 | 100 | 99 | 99.9 | 100 | 99.2 | 99 | 99.4 | 100 | 100 | 101 | 101 | 101 | 100 | 100 | 100 | 100 | 99.3 | 101 |
Si | 0.00 | 1.00 | 0.00 | 1.01 | 0.00 | 1.02 | 0.00 | 1.01 | 0.00 | 1.00 | 0.00 | 1.01 | 0.00 | 1.01 | 0.00 | 1.01 | 0.00 | 1.01 | 0.00 | 1.01 | 0.00 | 1.00 |
Ti | 0.00 | 0.00 | 0.00 | 0.00 | 0.01 | 0.00 | 0.00 | 0.00 | 0.00 | 0.00 | 0.00 | 0.00 | 0.01 | 0.00 | 0.01 | 0.00 | 0.01 | 0.00 | 0.01 | 0.00 | 0.01 | 0.00 |
Al | 0.59 | 0.00 | 0.82 | 0.00 | 0.63 | 0.00 | 0.89 | 0.00 | 0.97 | 0.00 | 0.80 | 0.00 | 0.76 | 0.00 | 0.74 | 0.00 | 0.56 | 0.00 | 0.78 | 0.00 | 0.74 | 0.00 |
Cr | 1.28 | 0.00 | 1.02 | 0.00 | 1.27 | 0.00 | 1.02 | 0.00 | 0.90 | 0.00 | 1.13 | 0.00 | 1.10 | 0.00 | 1.09 | 0.00 | 1.32 | 0.00 | 1.08 | 0.00 | 1.13 | 0.00 |
Fe3+ | 0.12 | 0.16 | 0.09 | 0.09 | 0.12 | 0.07 | 0.13 | 0.15 | 0.11 | 0.12 | 0.11 | |||||||||||
Fe2+ | 0.48 | 0.14 | 0.44 | 0.18 | 0.41 | 0.15 | 0.39 | 0.18 | 0.40 | 0.17 | 0.42 | 0.18 | 0.44 | 0.19 | 0.48 | 0.19 | 0.32 | 0.12 | 0.44 | 0.18 | 0.36 | 0.15 |
Mn | 0.01 | 0.00 | 0.00 | 0.00 | 0.00 | 0.00 | 0.00 | 0.00 | 0.00 | 0.00 | 0.00 | 0.00 | 0.01 | 0.00 | 0.00 | 0.00 | 0.00 | 0.00 | 0.01 | 0.00 | 0.00 | 0.00 |
Mg | 0.51 | 1.85 | 0.55 | 1.79 | 0.59 | 1.81 | 0.61 | 1.79 | 0.60 | 1.81 | 0.58 | 1.79 | 0.56 | 1.77 | 0.52 | 1.77 | 0.67 | 1.84 | 0.56 | 1.78 | 0.64 | 1.83 |
Ca | 0.00 | 0.00 | 0.00 | 0.00 | 0.00 | 0.01 | 0.00 | 0.00 | 0.00 | 0.00 | 0.00 | 0.00 | 0.00 | 0.00 | 0.00 | 0.00 | 0.00 | 0.00 | 0.00 | 0.00 | 0.00 | 0.00 |
Na | 0.00 | 0.00 | 0.00 | 0.00 | 0.00 | 0.00 | 0.00 | 0.00 | 0.00 | 0.00 | 0.00 | 0.00 | 0.00 | 0.00 | 0.00 | 0.00 | 0.00 | 0.00 | 0.00 | 0.00 | 0.00 | 0.00 |
K | 0.00 | 0.00 | 0.00 | 0.00 | 0.00 | 0.00 | 0.00 | 0.00 | 0.00 | 0.00 | 0.00 | 0.00 | 0.00 | 0.00 | 0.00 | 0.00 | 0.00 | 0.00 | 0.00 | 0.00 | 0.00 | 0.00 |
Ni | 0.00 | 0.01 | 0.00 | 0.01 | 0.00 | 0.01 | 0.00 | 0.01 | 0.00 | 0.01 | 0.00 | 0.01 | 0.00 | 0.01 | 0.00 | 0.01 | 0.00 | 0.01 | 0.00 | 0.01 | 0.00 | 0.01 |
Total | 3.00 | 3.00 | 3.00 | 2.99 | 3.00 | 2.98 | 3.00 | 2.99 | 3.00 | 3.00 | 3.00 | 2.99 | 3.00 | 2.99 | 3.00 | 2.99 | 3.00 | 2.98 | 3.00 | 2.99 | 3.00 | 3.00 |
Mg# | 51.4 | 92.7 | 55.3 | 90.8 | 59.1 | 92.5 | 61.2 | 90.7 | 59.9 | 91.3 | 58.3 | 91.1 | 56.0 | 90.2 | 52.4 | 90.2 | 67.6 | 93.9 | 56.2 | 90.6 | 64.3 | 92.4 |
Cr# | 68.3 | 55.5 | 67.0 | 53.5 | 48.0 | 58.6 | 59.1 | 59.5 | 70.3 | 58.3 | 60.5 | |||||||||||
Fe3+/∑Fe | 0.20 | 0.26 | 0.18 | 0.18 | 0.24 | 0.14 | 0.22 | 0.23 | 0.25 | 0.22 | 0.23 | |||||||||||
SiO2 | 0.00 | 41.3 | 0.00 | 41.3 | 0.05 | 41.5 | 0.09 | 0.00 | 42.1 | 0.00 | 42.2 | 0.00 | 42.3 | 0.02 | 42.0 | 0.00 | 42.0 | 0.00 | 42.0 | 0.01 | 41.5 | |
TiO2 | 0.15 | 0.00 | 0.30 | 0.00 | 0.10 | 0.00 | 0.28 | 0.26 | 0.05 | 0.20 | 0.00 | 0.17 | 0.00 | 0.22 | 0.00 | 0.21 | 0.00 | 0.20 | 0.00 | 0.08 | 0.00 | |
Al2O3 | 20.9 | 0.00 | 20.2 | 0.00 | 33.1 | 0.00 | 18.7 | 20.6 | 0.00 | 20.4 | 0.01 | 12.4 | 0.00 | 15.6 | 0.00 | 15.3 | 0.00 | 12.7 | 0.00 | 21.5 | 0.01 | |
Cr2O3 | 46.8 | 0.01 | 47.0 | 0.00 | 34.1 | 0.01 | 49.3 | 48.2 | 0.06 | 46.9 | 0.05 | 55.6 | 0.05 | 53.1 | 0.03 | 53.2 | 0.04 | 56.9 | 0.00 | 46.7 | 0.08 | |
FeO | 18.4 | 8.95 | 20.0 | 7.68 | 17.5 | 8.54 | 17.3 | 15.7 | 5.10 | 16.9 | 5.94 | 16.5 | 3.67 | 15.2 | 5.07 | 16.0 | 5.19 | 14.5 | 4.90 | 15.2 | 7.55 | |
MnO | 0.12 | 0.14 | 0.15 | 0.10 | 0.00 | 0.11 | 0.00 | 0.00 | 0.05 | 0.00 | 0.06 | 0.00 | 0.06 | 0.00 | 0.04 | 0.00 | 0.08 | 0.00 | 0.09 | 0.00 | 0.09 | |
MgO | 13.4 | 48.7 | 12.6 | 49.4 | 14.8 | 49.3 | 13.7 | 15.1 | 52.1 | 14.0 | 52.0 | 13.6 | 52.8 | 14.9 | 52.2 | 14.4 | 51.8 | 14.6 | 51.7 | 15.1 | 49.6 | |
CaO | 0.00 | 0.06 | 0.01 | 0.03 | 0.00 | 0.11 | 0.02 | 0.05 | 0.02 | 0.03 | 0.02 | 0.03 | 0.05 | 0.00 | 0.08 | 0.00 | 0.07 | 0.02 | 0.07 | 0.00 | 0.01 | |
Na2O | 0.00 | 0.00 | 0.02 | 0.00 | 0.00 | 0.00 | 0.00 | 0.00 | 0.00 | 0.00 | 0.00 | 0.00 | 0.00 | 0.01 | 0.00 | 0.01 | 0.00 | 0.00 | 0.00 | 0.00 | 0.00 | |
K2O | 0.00 | 0.00 | 0.00 | 0.01 | 0.00 | 0.00 | 0.00 | 0.00 | 0.00 | 0.00 | 0.01 | 0.00 | 0.03 | 0.00 | 0.00 | 0.00 | 0.00 | 0.00 | 0.00 | 0.00 | 0.00 | |
NiO | 0.10 | 0.37 | 0.13 | 0.42 | 0.13 | 0.37 | 0.12 | 0.09 | 0.56 | 0.15 | 0.43 | 0.09 | 0.57 | 0.16 | 0.52 | 0.12 | 0.44 | 0.16 | 0.53 | 0.10 | 0.51 | |
Total | 99.9 | 100 | 100 | 99 | 99.8 | 100 | 99.5 | 100 | 100 | 98.6 | 101 | 98.4 | 100 | 99.2 | 100 | 99.2 | 100 | 99.1 | 99 | 98.7 | 99 | |
Si | 0.00 | 1.01 | 0.00 | 1.01 | 0.00 | 1.01 | 0.00 | 0.00 | 1.01 | 0.00 | 1.01 | 0.00 | 1.01 | 0.00 | 1.01 | 0.00 | 1.01 | 0.00 | 1.01 | 0.00 | 1.01 | |
Ti | 0.00 | 0.00 | 0.01 | 0.00 | 0.00 | 0.00 | 0.01 | 0.01 | 0.00 | 0.00 | 0.00 | 0.00 | 0.00 | 0.01 | 0.00 | 0.00 | 0.00 | 0.00 | 0.00 | 0.00 | 0.00 | |
Al | 0.57 | 0.00 | 0.74 | 0.00 | 1.14 | 0.00 | 0.69 | 0.74 | 0.00 | 0.75 | 0.00 | 0.47 | 0.00 | 0.58 | 0.00 | 0.76 | 0.00 | 0.48 | 0.00 | 0.78 | 0.00 | |
Cr | 1.33 | 0.00 | 1.15 | 0.00 | 0.79 | 0.00 | 1.21 | 1.16 | 0.00 | 1.15 | 0.00 | 1.42 | 0.00 | 1.32 | 0.00 | 1.14 | 0.00 | 1.43 | 0.00 | 1.13 | 0.00 | |
Fe3+ | 0.10 | 0.10 | 0.07 | 0.08 | 0.08 | 0.09 | 0.10 | 0.10 | 0.09 | 0.08 | 0.08 | |||||||||||
Fe2+ | 0.32 | 0.18 | 0.42 | 0.16 | 0.36 | 0.17 | 0.37 | 0.32 | 0.10 | 0.35 | 0.12 | 0.35 | 0.07 | 0.30 | 0.10 | 0.38 | 0.10 | 0.31 | 0.10 | 0.31 | 0.15 | |
Mn | 0.00 | 0.00 | 0.00 | 0.00 | 0.00 | 0.00 | 0.00 | 0.00 | 0.00 | 0.00 | 0.00 | 0.00 | 0.00 | 0.00 | 0.00 | 0.00 | 0.00 | 0.00 | 0.00 | 0.00 | 0.00 | |
Mg | 0.68 | 1.78 | 0.58 | 1.81 | 0.64 | 1.79 | 0.64 | 0.69 | 1.86 | 0.65 | 1.85 | 0.65 | 1.89 | 0.70 | 1.87 | 0.62 | 1.86 | 0.69 | 1.86 | 0.69 | 1.80 | |
Ca | 0.00 | 0.00 | 0.00 | 0.00 | 0.00 | 0.00 | 0.00 | 0.00 | 0.00 | 0.00 | 0.00 | 0.00 | 0.00 | 0.00 | 0.00 | 0.00 | 0.00 | 0.00 | 0.00 | 0.00 | 0.00 | |
Na | 0.00 | 0.00 | 0.00 | 0.00 | 0.00 | 0.00 | 0.00 | 0.00 | 0.00 | 0.00 | 0.00 | 0.00 | 0.00 | 0.00 | 0.00 | 0.00 | 0.00 | 0.00 | 0.00 | 0.00 | 0.00 | |
K | 0.00 | 0.00 | 0.00 | 0.00 | 0.00 | 0.00 | 0.00 | 0.00 | 0.00 | 0.00 | 0.00 | 0.00 | 0.00 | 0.00 | 0.00 | 0.00 | 0.00 | 0.00 | 0.00 | 0.00 | 0.00 | |
Ni | 0.00 | 0.01 | 0.00 | 0.01 | 0.00 | 0.01 | 0.00 | 0.00 | 0.01 | 0.00 | 0.01 | 0.00 | 0.01 | 0.00 | 0.01 | 0.00 | 0.01 | 0.00 | 0.01 | 0.00 | 0.01 | |
Total | 3.00 | 2.99 | 3.00 | 2.99 | 3.00 | 2.99 | 3.00 | 3.00 | 2.99 | 3.00 | 2.99 | 3.00 | 2.99 | 3.00 | 2.99 | 3.00 | 2.99 | 3.00 | 2.99 | 3.00 | 2.99 | |
Mg# | 61.9 | 90.7 | 58.3 | 92.0 | 64.3 | 91.1 | 63.2 | 68.5 | 94.8 | 64.9 | 94.0 | 65.4 | 96.2 | 69.6 | 94.8 | 67.6 | 94.7 | 69.3 | 95.0 | 69.2 | 92.1 | |
Cr# | 60.0 | 61.0 | 40.9 | 63.9 | 61.1 | 60.7 | 75.0 | 69.5 | 70.0 | 75.0 | 59.3 | |||||||||||
Fe3+/∑Fe | 0.20 | 0.21 | 0.16 | 0.18 | 0.21 | 0.20 | 0.22 | 0.24 | 0.20 | 0.21 | 0.21 |
Locality | Luobusa | |||||||||||||||||||||
Sample | LBS09005–8 | LBS13–21 | LBS13–22 | LBS13–23 | LBS13–34 | LBS13–39 | L1 | L2 | L4 | L3 | L5 | L6 | L7 | L06a | ||||||||
Rock type | Chromitite | Chromitite | Chromitite | Chromitite | Chromitite | Chromitite | Chromitite | Chromitite | Chromitite | Chromitite | Chromitite | Chromitite | Chromitite | Chromitite | ||||||||
Mineral | Chra | Ola | Chr | Chr | Chr | Chr | Chr | Chr | Ol | Chr | Ol | Chr | Ol | Chr | Ol | Chr | Ol | Chr | Chr | Chr | ||
Point | 8 | 8 | 8 | 8 | 8 | 8 | 8 | 8 | 8 | 8 | 8 | 8 | 8 | 8 | 8 | 8 | 8 | 8 | 8 | 8 | ||
SiO2 | 0.00 | 41.81 | 0.05 | 0.00 | 0.03 | 0.01 | 0.01 | 0.02 | 42.0 | 0.00 | 42.0 | 0.03 | 42.1 | 0.00 | 41.8 | 0.02 | 42.1 | 0.00 | 0.00 | 0.00 | ||
TiO2 | 0.23 | 0.00 | 0.21 | 0.20 | 0.13 | 0.19 | 0.23 | 0.18 | 0.03 | 0.19 | 0.02 | 0.19 | 0.01 | 0.20 | 0.01 | 0.22 | 0.00 | 0.19 | 0.16 | 0.22 | ||
Al2O3 | 10.4 | 0.00 | 11.0 | 11.9 | 10.3 | 13.1 | 11.0 | 10.2 | 0.00 | 10.7 | 0.00 | 10.6 | 0.00 | 12.4 | 0.01 | 11.1 | 0.00 | 10.9 | 12.8 | 11.6 | ||
Cr2O3 | 61.5 | 0.02 | 60.0 | 58.8 | 60.5 | 58.7 | 60.8 | 59.4 | 0.01 | 59.3 | 0.02 | 59.2 | 0.02 | 57.9 | 0.01 | 59.8 | 0.02 | 60.1 | 57.5 | 61.2 | ||
FeO | 16.3 | 4.69 | 14.6 | 15.8 | 15.2 | 12.8 | 12.0 | 15.4 | 4.34 | 14.6 | 4.43 | 13.4 | 3.17 | 13.6 | 3.59 | 13.5 | 3.52 | 12.8 | 13.5 | 12.4 | ||
MnO | 0.15 | 0.08 | 0.22 | 0.23 | 0.22 | 0.20 | 0.20 | 0.13 | 0.07 | 0.11 | 0.03 | 0.09 | 0.02 | 0.11 | 0.04 | 0.10 | 0.04 | 0.10 | 0.08 | 0.05 | ||
MgO | 13.2 | 53.0 | 15.2 | 13.3 | 13.4 | 16.0 | 16.5 | 14.1 | 53.3 | 14.4 | 53.2 | 15.7 | 53.7 | 15.2 | 53.6 | 15.7 | 54.0 | 15.6 | 15.7 | 16.0 | ||
CaO | 0.01 | 0.04 | 0.00 | 0.00 | 0.00 | 0.01 | 0.00 | 0.00 | 0.00 | 0.01 | 0.01 | 0.01 | 0.05 | 0.00 | 0.02 | 0.01 | 0.05 | 0.01 | 0.00 | 0.02 | ||
Na2O | 0.02 | 0.01 | 0.01 | 0.00 | 0.02 | 0.00 | 0.01 | 0.05 | 0.00 | 0.01 | 0.01 | 0.04 | 0.01 | 0.01 | 0.00 | 0.01 | 0.00 | 0.01 | 0.01 | 0.00 | ||
K2O | 0.00 | 0.00 | 0.00 | 0.00 | 0.00 | 0.00 | 0.00 | 0.01 | 0.01 | 0.01 | 0.00 | 0.01 | 0.01 | 0.00 | 0.00 | 0.01 | 0.01 | 0.01 | 0.01 | 0.00 | ||
NiO | 0.09 | 0.53 | 0.10 | 0.12 | 0.04 | 0.15 | 0.20 | 0.10 | 0.49 | 0.10 | 0.53 | 0.14 | 0.58 | 0.12 | 0.67 | 0.13 | 0.61 | 0.17 | 0.15 | 0.17 | ||
Total | 102 | 100 | 101 | 100 | 99.8 | 101 | 101 | 99.4 | 100 | 99.3 | 100 | 99.3 | 100 | 99.5 | 100 | 100 | 100 | 99.7 | 99.7 | 101 | ||
Si | 0.00 | 1.00 | 0.00 | 0.00 | 0.00 | 0.00 | 0.00 | 0.00 | 1.00 | 0.00 | 1.00 | 0.00 | 1.00 | 0.00 | 1.00 | 0.00 | 1.00 | 0.00 | 0.00 | 0.00 | ||
Ti | 0.01 | 0.00 | 0.00 | 0.00 | 0.00 | 0.00 | 0.01 | 0.00 | 0.00 | 0.00 | 0.00 | 0.00 | 0.00 | 0.00 | 0.00 | 0.01 | 0.00 | 0.00 | 0.00 | 0.01 | ||
Al | 0.39 | 0.00 | 0.41 | 0.45 | 0.39 | 0.48 | 0.38 | 0.38 | 0.00 | 0.41 | 0.00 | 0.40 | 0.00 | 0.46 | 0.00 | 0.41 | 0.00 | 0.41 | 0.47 | 0.42 | ||
Cr | 1.54 | 0.00 | 1.49 | 1.48 | 1.54 | 1.44 | 1.55 | 1.51 | 0.00 | 1.50 | 0.00 | 1.49 | 0.00 | 1.45 | 0.00 | 1.49 | 0.00 | 1.51 | 1.43 | 1.50 | ||
Fe3+ | 0.06 | 0.09 | 0.06 | 0.06 | 0.07 | 0.06 | 0.10 | 0.08 | 0.11 | 0.08 | 0.09 | 0.08 | 0.09 | 0.06 | ||||||||
Fe2+ | 0.37 | 0.09 | 0.29 | 0.36 | 0.35 | 0.26 | 0.26 | 0.32 | 0.09 | 0.31 | 0.09 | 0.25 | 0.06 | 0.28 | 0.07 | 0.26 | 0.07 | 0.26 | 0.26 | 0.26 | ||
Mn | 0.00 | 0.00 | 0.01 | 0.01 | 0.01 | 0.01 | 0.00 | 0.00 | 0.00 | 0.00 | 0.00 | 0.00 | 0.00 | 0.00 | 0.00 | 0.00 | 0.00 | 0.00 | 0.00 | 0.00 | ||
Mg | 0.62 | 1.89 | 0.71 | 0.63 | 0.64 | 0.74 | 0.73 | 0.68 | 1.90 | 0.69 | 1.89 | 0.74 | 1.91 | 0.72 | 1.91 | 0.74 | 1.91 | 0.74 | 0.73 | 0.74 | ||
Ca | 0.00 | 0.00 | 0.00 | 0.00 | 0.00 | 0.00 | 0.00 | 0.00 | 0.00 | 0.00 | 0.00 | 0.00 | 0.00 | 0.00 | 0.00 | 0.00 | 0.00 | 0.00 | 0.00 | 0.00 | ||
Na | 0.00 | 0.00 | 0.00 | 0.00 | 0.00 | 0.00 | 0.00 | 0.00 | 0.00 | 0.00 | 0.00 | 0.00 | 0.00 | 0.00 | 0.00 | 0.00 | 0.00 | 0.00 | 0.00 | 0.00 | ||
K | 0.00 | 0.00 | 0.00 | 0.00 | 0.00 | 0.00 | 0.00 | 0.00 | 0.00 | 0.00 | 0.00 | 0.00 | 0.00 | 0.00 | 0.00 | 0.00 | 0.00 | 0.00 | 0.00 | 0.00 | ||
Ni | 0.00 | 0.01 | 0.00 | 0.00 | 0.00 | 0.00 | 0.01 | 0.00 | 0.01 | 0.00 | 0.01 | 0.00 | 0.01 | 0.00 | 0.01 | 0.00 | 0.01 | 0.00 | 0.00 | 0.00 | ||
Total | 3.00 | 3.00 | 3.00 | 3.00 | 3.00 | 3.00 | 3.00 | 3.00 | 3.00 | 3.00 | 3.00 | 3.00 | 3.00 | 3.00 | 3.00 | 3.00 | 3.00 | 3.00 | 3.00 | 3.00 | ||
Mg#b | 62.5 | 95.3 | 71.1 | 63.6 | 64.7 | 74.4 | 74.7 | 68.2 | 95.7 | 68.9 | 95.6 | 74.8 | 96.8 | 72.0 | 96.4 | 73.7 | 96.5 | 74.1 | 73.6 | 74.4 | ||
Cr#b | 79.8 | 78.6 | 76.9 | 79.7 | 75.0 | 78.8 | 79.7 | 78.8 | 78.9 | 75.8 | 78.3 | 78.8 | 75.1 | 78.0 | ||||||||
Fe3+/∑Feb | 0.13 | 0.25 | 0.14 | 0.14 | 0.23 | 0.18 | 0.23 | 0.21 | 0.30 | 0.22 | 0.26 | 0.24 | 0.26 | 0.20 | ||||||||
SiO2 | 0.05 | 41.6 | 0.02 | 42.0 | 0.03 | 41.4 | 0.02 | 41.6 | 0.05 | 40.8 | 0.00 | 41.5 | 0.03 | 41.6 | 0.00 | 41.6 | 0.02 | 42.1 | 0.10 | 41.4 | 0.06 | 41.5 |
TiO2 | 0.15 | 0.00 | 0.06 | 0.05 | 0.28 | 0.00 | 0.18 | 0.00 | 0.05 | 0.02 | 0.16 | 0.03 | 0.36 | 0.00 | 0.36 | 0.00 | 0.23 | 0.03 | 0.27 | 0.00 | 0.39 | 0.04 |
Al2O3 | 15.6 | 0.00 | 22.6 | 0.01 | 16.9 | 0.00 | 24.8 | 0.00 | 27.3 | 0.00 | 21.8 | 0.00 | 20.9 | 0.00 | 20.3 | 0.00 | 15.1 | 0.01 | 21.3 | 0.01 | 20.3 | 0.01 |
Cr2O3 | 50.2 | 0.00 | 41.9 | 0.00 | 51.3 | 0.02 | 42.5 | 0.04 | 37.6 | 0.06 | 46.0 | 0.14 | 44.9 | 0.00 | 44.5 | 0.00 | 53.4 | 0.04 | 44.3 | 0.01 | 46.4 | 0.00 |
FeO | 22.4 | 7.23 | 23.4 | 8.99 | 19.0 | 7.11 | 18.6 | 8.95 | 20.8 | 8.33 | 18.7 | 8.64 | 21.9 | 9.52 | 23.9 | 9.52 | 16.6 | 5.91 | 21.5 | 9.03 | 17.9 | 7.48 |
MnO | 0.22 | 0.12 | 0.17 | 0.10 | 0.16 | 0.12 | 0.13 | 0.14 | 0.00 | 0.09 | 0.00 | 0.14 | 0.21 | 0.13 | 0.18 | 0.13 | 0.13 | 0.06 | 0.22 | 0.11 | 0.16 | 0.11 |
MgO | 10.7 | 51.6 | 12.0 | 49.9 | 12.7 | 49.4 | 13.5 | 49.2 | 13.3 | 49.3 | 12.6 | 49.4 | 12.1 | 49.0 | 11.3 | 49.0 | 14.5 | 51.1 | 12.1 | 48.9 | 13.9 | 51.0 |
CaO | 0.03 | 0.11 | 0.00 | 0.11 | 0.00 | 0.21 | 0.00 | 0.04 | 0.00 | 0.03 | 0.03 | 0.12 | 0.00 | 0.07 | 0.02 | 0.07 | 0.00 | 0.15 | 0.04 | 0.07 | 0.03 | 0.02 |
Na2O | 0.00 | 0.00 | 0.01 | 0.00 | 0.00 | 0.01 | 0.00 | 0.00 | 0.00 | 0.00 | 0.00 | 0.00 | 0.04 | 0.00 | 0.00 | 0.00 | 0.00 | 0.00 | 0.03 | 0.00 | 0.03 | 0.01 |
K2O | 0.00 | 0.00 | 0.00 | 0.00 | 0.00 | 0.00 | 0.00 | 0.02 | 0.00 | 0.00 | 0.00 | 0.00 | 0.00 | 0.01 | 0.00 | 0.01 | 0.00 | 0.00 | 0.00 | 0.00 | 0.00 | 0.00 |
NiO | 0.07 | 0.39 | 0.13 | 0.38 | 0.08 | 0.42 | 0.16 | 0.34 | 0.13 | 0.39 | 0.11 | 0.37 | 0.11 | 0.36 | 0.12 | 0.36 | 0.13 | 0.43 | 0.10 | 0.33 | 0.12 | 0.38 |
Total | 99.5 | 101 | 100 | 101 | 100 | 99 | 99.9 | 100 | 99.2 | 99 | 99.4 | 100 | 100 | 101 | 101 | 101 | 100 | 100 | 100 | 100 | 99.3 | 101 |
Si | 0.00 | 1.00 | 0.00 | 1.01 | 0.00 | 1.02 | 0.00 | 1.01 | 0.00 | 1.00 | 0.00 | 1.01 | 0.00 | 1.01 | 0.00 | 1.01 | 0.00 | 1.01 | 0.00 | 1.01 | 0.00 | 1.00 |
Ti | 0.00 | 0.00 | 0.00 | 0.00 | 0.01 | 0.00 | 0.00 | 0.00 | 0.00 | 0.00 | 0.00 | 0.00 | 0.01 | 0.00 | 0.01 | 0.00 | 0.01 | 0.00 | 0.01 | 0.00 | 0.01 | 0.00 |
Al | 0.59 | 0.00 | 0.82 | 0.00 | 0.63 | 0.00 | 0.89 | 0.00 | 0.97 | 0.00 | 0.80 | 0.00 | 0.76 | 0.00 | 0.74 | 0.00 | 0.56 | 0.00 | 0.78 | 0.00 | 0.74 | 0.00 |
Cr | 1.28 | 0.00 | 1.02 | 0.00 | 1.27 | 0.00 | 1.02 | 0.00 | 0.90 | 0.00 | 1.13 | 0.00 | 1.10 | 0.00 | 1.09 | 0.00 | 1.32 | 0.00 | 1.08 | 0.00 | 1.13 | 0.00 |
Fe3+ | 0.12 | 0.16 | 0.09 | 0.09 | 0.12 | 0.07 | 0.13 | 0.15 | 0.11 | 0.12 | 0.11 | |||||||||||
Fe2+ | 0.48 | 0.14 | 0.44 | 0.18 | 0.41 | 0.15 | 0.39 | 0.18 | 0.40 | 0.17 | 0.42 | 0.18 | 0.44 | 0.19 | 0.48 | 0.19 | 0.32 | 0.12 | 0.44 | 0.18 | 0.36 | 0.15 |
Mn | 0.01 | 0.00 | 0.00 | 0.00 | 0.00 | 0.00 | 0.00 | 0.00 | 0.00 | 0.00 | 0.00 | 0.00 | 0.01 | 0.00 | 0.00 | 0.00 | 0.00 | 0.00 | 0.01 | 0.00 | 0.00 | 0.00 |
Mg | 0.51 | 1.85 | 0.55 | 1.79 | 0.59 | 1.81 | 0.61 | 1.79 | 0.60 | 1.81 | 0.58 | 1.79 | 0.56 | 1.77 | 0.52 | 1.77 | 0.67 | 1.84 | 0.56 | 1.78 | 0.64 | 1.83 |
Ca | 0.00 | 0.00 | 0.00 | 0.00 | 0.00 | 0.01 | 0.00 | 0.00 | 0.00 | 0.00 | 0.00 | 0.00 | 0.00 | 0.00 | 0.00 | 0.00 | 0.00 | 0.00 | 0.00 | 0.00 | 0.00 | 0.00 |
Na | 0.00 | 0.00 | 0.00 | 0.00 | 0.00 | 0.00 | 0.00 | 0.00 | 0.00 | 0.00 | 0.00 | 0.00 | 0.00 | 0.00 | 0.00 | 0.00 | 0.00 | 0.00 | 0.00 | 0.00 | 0.00 | 0.00 |
K | 0.00 | 0.00 | 0.00 | 0.00 | 0.00 | 0.00 | 0.00 | 0.00 | 0.00 | 0.00 | 0.00 | 0.00 | 0.00 | 0.00 | 0.00 | 0.00 | 0.00 | 0.00 | 0.00 | 0.00 | 0.00 | 0.00 |
Ni | 0.00 | 0.01 | 0.00 | 0.01 | 0.00 | 0.01 | 0.00 | 0.01 | 0.00 | 0.01 | 0.00 | 0.01 | 0.00 | 0.01 | 0.00 | 0.01 | 0.00 | 0.01 | 0.00 | 0.01 | 0.00 | 0.01 |
Total | 3.00 | 3.00 | 3.00 | 2.99 | 3.00 | 2.98 | 3.00 | 2.99 | 3.00 | 3.00 | 3.00 | 2.99 | 3.00 | 2.99 | 3.00 | 2.99 | 3.00 | 2.98 | 3.00 | 2.99 | 3.00 | 3.00 |
Mg# | 51.4 | 92.7 | 55.3 | 90.8 | 59.1 | 92.5 | 61.2 | 90.7 | 59.9 | 91.3 | 58.3 | 91.1 | 56.0 | 90.2 | 52.4 | 90.2 | 67.6 | 93.9 | 56.2 | 90.6 | 64.3 | 92.4 |
Cr# | 68.3 | 55.5 | 67.0 | 53.5 | 48.0 | 58.6 | 59.1 | 59.5 | 70.3 | 58.3 | 60.5 | |||||||||||
Fe3+/∑Fe | 0.20 | 0.26 | 0.18 | 0.18 | 0.24 | 0.14 | 0.22 | 0.23 | 0.25 | 0.22 | 0.23 | |||||||||||
SiO2 | 0.00 | 41.3 | 0.00 | 41.3 | 0.05 | 41.5 | 0.09 | 0.00 | 42.1 | 0.00 | 42.2 | 0.00 | 42.3 | 0.02 | 42.0 | 0.00 | 42.0 | 0.00 | 42.0 | 0.01 | 41.5 | |
TiO2 | 0.15 | 0.00 | 0.30 | 0.00 | 0.10 | 0.00 | 0.28 | 0.26 | 0.05 | 0.20 | 0.00 | 0.17 | 0.00 | 0.22 | 0.00 | 0.21 | 0.00 | 0.20 | 0.00 | 0.08 | 0.00 | |
Al2O3 | 20.9 | 0.00 | 20.2 | 0.00 | 33.1 | 0.00 | 18.7 | 20.6 | 0.00 | 20.4 | 0.01 | 12.4 | 0.00 | 15.6 | 0.00 | 15.3 | 0.00 | 12.7 | 0.00 | 21.5 | 0.01 | |
Cr2O3 | 46.8 | 0.01 | 47.0 | 0.00 | 34.1 | 0.01 | 49.3 | 48.2 | 0.06 | 46.9 | 0.05 | 55.6 | 0.05 | 53.1 | 0.03 | 53.2 | 0.04 | 56.9 | 0.00 | 46.7 | 0.08 | |
FeO | 18.4 | 8.95 | 20.0 | 7.68 | 17.5 | 8.54 | 17.3 | 15.7 | 5.10 | 16.9 | 5.94 | 16.5 | 3.67 | 15.2 | 5.07 | 16.0 | 5.19 | 14.5 | 4.90 | 15.2 | 7.55 | |
MnO | 0.12 | 0.14 | 0.15 | 0.10 | 0.00 | 0.11 | 0.00 | 0.00 | 0.05 | 0.00 | 0.06 | 0.00 | 0.06 | 0.00 | 0.04 | 0.00 | 0.08 | 0.00 | 0.09 | 0.00 | 0.09 | |
MgO | 13.4 | 48.7 | 12.6 | 49.4 | 14.8 | 49.3 | 13.7 | 15.1 | 52.1 | 14.0 | 52.0 | 13.6 | 52.8 | 14.9 | 52.2 | 14.4 | 51.8 | 14.6 | 51.7 | 15.1 | 49.6 | |
CaO | 0.00 | 0.06 | 0.01 | 0.03 | 0.00 | 0.11 | 0.02 | 0.05 | 0.02 | 0.03 | 0.02 | 0.03 | 0.05 | 0.00 | 0.08 | 0.00 | 0.07 | 0.02 | 0.07 | 0.00 | 0.01 | |
Na2O | 0.00 | 0.00 | 0.02 | 0.00 | 0.00 | 0.00 | 0.00 | 0.00 | 0.00 | 0.00 | 0.00 | 0.00 | 0.00 | 0.01 | 0.00 | 0.01 | 0.00 | 0.00 | 0.00 | 0.00 | 0.00 | |
K2O | 0.00 | 0.00 | 0.00 | 0.01 | 0.00 | 0.00 | 0.00 | 0.00 | 0.00 | 0.00 | 0.01 | 0.00 | 0.03 | 0.00 | 0.00 | 0.00 | 0.00 | 0.00 | 0.00 | 0.00 | 0.00 | |
NiO | 0.10 | 0.37 | 0.13 | 0.42 | 0.13 | 0.37 | 0.12 | 0.09 | 0.56 | 0.15 | 0.43 | 0.09 | 0.57 | 0.16 | 0.52 | 0.12 | 0.44 | 0.16 | 0.53 | 0.10 | 0.51 | |
Total | 99.9 | 100 | 100 | 99 | 99.8 | 100 | 99.5 | 100 | 100 | 98.6 | 101 | 98.4 | 100 | 99.2 | 100 | 99.2 | 100 | 99.1 | 99 | 98.7 | 99 | |
Si | 0.00 | 1.01 | 0.00 | 1.01 | 0.00 | 1.01 | 0.00 | 0.00 | 1.01 | 0.00 | 1.01 | 0.00 | 1.01 | 0.00 | 1.01 | 0.00 | 1.01 | 0.00 | 1.01 | 0.00 | 1.01 | |
Ti | 0.00 | 0.00 | 0.01 | 0.00 | 0.00 | 0.00 | 0.01 | 0.01 | 0.00 | 0.00 | 0.00 | 0.00 | 0.00 | 0.01 | 0.00 | 0.00 | 0.00 | 0.00 | 0.00 | 0.00 | 0.00 | |
Al | 0.57 | 0.00 | 0.74 | 0.00 | 1.14 | 0.00 | 0.69 | 0.74 | 0.00 | 0.75 | 0.00 | 0.47 | 0.00 | 0.58 | 0.00 | 0.76 | 0.00 | 0.48 | 0.00 | 0.78 | 0.00 | |
Cr | 1.33 | 0.00 | 1.15 | 0.00 | 0.79 | 0.00 | 1.21 | 1.16 | 0.00 | 1.15 | 0.00 | 1.42 | 0.00 | 1.32 | 0.00 | 1.14 | 0.00 | 1.43 | 0.00 | 1.13 | 0.00 | |
Fe3+ | 0.10 | 0.10 | 0.07 | 0.08 | 0.08 | 0.09 | 0.10 | 0.10 | 0.09 | 0.08 | 0.08 | |||||||||||
Fe2+ | 0.32 | 0.18 | 0.42 | 0.16 | 0.36 | 0.17 | 0.37 | 0.32 | 0.10 | 0.35 | 0.12 | 0.35 | 0.07 | 0.30 | 0.10 | 0.38 | 0.10 | 0.31 | 0.10 | 0.31 | 0.15 | |
Mn | 0.00 | 0.00 | 0.00 | 0.00 | 0.00 | 0.00 | 0.00 | 0.00 | 0.00 | 0.00 | 0.00 | 0.00 | 0.00 | 0.00 | 0.00 | 0.00 | 0.00 | 0.00 | 0.00 | 0.00 | 0.00 | |
Mg | 0.68 | 1.78 | 0.58 | 1.81 | 0.64 | 1.79 | 0.64 | 0.69 | 1.86 | 0.65 | 1.85 | 0.65 | 1.89 | 0.70 | 1.87 | 0.62 | 1.86 | 0.69 | 1.86 | 0.69 | 1.80 | |
Ca | 0.00 | 0.00 | 0.00 | 0.00 | 0.00 | 0.00 | 0.00 | 0.00 | 0.00 | 0.00 | 0.00 | 0.00 | 0.00 | 0.00 | 0.00 | 0.00 | 0.00 | 0.00 | 0.00 | 0.00 | 0.00 | |
Na | 0.00 | 0.00 | 0.00 | 0.00 | 0.00 | 0.00 | 0.00 | 0.00 | 0.00 | 0.00 | 0.00 | 0.00 | 0.00 | 0.00 | 0.00 | 0.00 | 0.00 | 0.00 | 0.00 | 0.00 | 0.00 | |
K | 0.00 | 0.00 | 0.00 | 0.00 | 0.00 | 0.00 | 0.00 | 0.00 | 0.00 | 0.00 | 0.00 | 0.00 | 0.00 | 0.00 | 0.00 | 0.00 | 0.00 | 0.00 | 0.00 | 0.00 | 0.00 | |
Ni | 0.00 | 0.01 | 0.00 | 0.01 | 0.00 | 0.01 | 0.00 | 0.00 | 0.01 | 0.00 | 0.01 | 0.00 | 0.01 | 0.00 | 0.01 | 0.00 | 0.01 | 0.00 | 0.01 | 0.00 | 0.01 | |
Total | 3.00 | 2.99 | 3.00 | 2.99 | 3.00 | 2.99 | 3.00 | 3.00 | 2.99 | 3.00 | 2.99 | 3.00 | 2.99 | 3.00 | 2.99 | 3.00 | 2.99 | 3.00 | 2.99 | 3.00 | 2.99 | |
Mg# | 61.9 | 90.7 | 58.3 | 92.0 | 64.3 | 91.1 | 63.2 | 68.5 | 94.8 | 64.9 | 94.0 | 65.4 | 96.2 | 69.6 | 94.8 | 67.6 | 94.7 | 69.3 | 95.0 | 69.2 | 92.1 | |
Cr# | 60.0 | 61.0 | 40.9 | 63.9 | 61.1 | 60.7 | 75.0 | 69.5 | 70.0 | 75.0 | 59.3 | |||||||||||
Fe3+/∑Fe | 0.20 | 0.21 | 0.16 | 0.18 | 0.21 | 0.20 | 0.22 | 0.24 | 0.20 | 0.21 | 0.21 |
Major oxide (wt.%) compositions of chromite and olivine in the dunites and chromitites from Luobusa and Kızıldağ ophiolites, and Gaositai Alaskan-type complex
Locality | Luobusa | |||||||||||||||||||||
Sample | LBS09005–8 | LBS13–21 | LBS13–22 | LBS13–23 | LBS13–34 | LBS13–39 | L1 | L2 | L4 | L3 | L5 | L6 | L7 | L06a | ||||||||
Rock type | Chromitite | Chromitite | Chromitite | Chromitite | Chromitite | Chromitite | Chromitite | Chromitite | Chromitite | Chromitite | Chromitite | Chromitite | Chromitite | Chromitite | ||||||||
Mineral | Chra | Ola | Chr | Chr | Chr | Chr | Chr | Chr | Ol | Chr | Ol | Chr | Ol | Chr | Ol | Chr | Ol | Chr | Chr | Chr | ||
Point | 8 | 8 | 8 | 8 | 8 | 8 | 8 | 8 | 8 | 8 | 8 | 8 | 8 | 8 | 8 | 8 | 8 | 8 | 8 | 8 | ||
SiO2 | 0.00 | 41.81 | 0.05 | 0.00 | 0.03 | 0.01 | 0.01 | 0.02 | 42.0 | 0.00 | 42.0 | 0.03 | 42.1 | 0.00 | 41.8 | 0.02 | 42.1 | 0.00 | 0.00 | 0.00 | ||
TiO2 | 0.23 | 0.00 | 0.21 | 0.20 | 0.13 | 0.19 | 0.23 | 0.18 | 0.03 | 0.19 | 0.02 | 0.19 | 0.01 | 0.20 | 0.01 | 0.22 | 0.00 | 0.19 | 0.16 | 0.22 | ||
Al2O3 | 10.4 | 0.00 | 11.0 | 11.9 | 10.3 | 13.1 | 11.0 | 10.2 | 0.00 | 10.7 | 0.00 | 10.6 | 0.00 | 12.4 | 0.01 | 11.1 | 0.00 | 10.9 | 12.8 | 11.6 | ||
Cr2O3 | 61.5 | 0.02 | 60.0 | 58.8 | 60.5 | 58.7 | 60.8 | 59.4 | 0.01 | 59.3 | 0.02 | 59.2 | 0.02 | 57.9 | 0.01 | 59.8 | 0.02 | 60.1 | 57.5 | 61.2 | ||
FeO | 16.3 | 4.69 | 14.6 | 15.8 | 15.2 | 12.8 | 12.0 | 15.4 | 4.34 | 14.6 | 4.43 | 13.4 | 3.17 | 13.6 | 3.59 | 13.5 | 3.52 | 12.8 | 13.5 | 12.4 | ||
MnO | 0.15 | 0.08 | 0.22 | 0.23 | 0.22 | 0.20 | 0.20 | 0.13 | 0.07 | 0.11 | 0.03 | 0.09 | 0.02 | 0.11 | 0.04 | 0.10 | 0.04 | 0.10 | 0.08 | 0.05 | ||
MgO | 13.2 | 53.0 | 15.2 | 13.3 | 13.4 | 16.0 | 16.5 | 14.1 | 53.3 | 14.4 | 53.2 | 15.7 | 53.7 | 15.2 | 53.6 | 15.7 | 54.0 | 15.6 | 15.7 | 16.0 | ||
CaO | 0.01 | 0.04 | 0.00 | 0.00 | 0.00 | 0.01 | 0.00 | 0.00 | 0.00 | 0.01 | 0.01 | 0.01 | 0.05 | 0.00 | 0.02 | 0.01 | 0.05 | 0.01 | 0.00 | 0.02 | ||
Na2O | 0.02 | 0.01 | 0.01 | 0.00 | 0.02 | 0.00 | 0.01 | 0.05 | 0.00 | 0.01 | 0.01 | 0.04 | 0.01 | 0.01 | 0.00 | 0.01 | 0.00 | 0.01 | 0.01 | 0.00 | ||
K2O | 0.00 | 0.00 | 0.00 | 0.00 | 0.00 | 0.00 | 0.00 | 0.01 | 0.01 | 0.01 | 0.00 | 0.01 | 0.01 | 0.00 | 0.00 | 0.01 | 0.01 | 0.01 | 0.01 | 0.00 | ||
NiO | 0.09 | 0.53 | 0.10 | 0.12 | 0.04 | 0.15 | 0.20 | 0.10 | 0.49 | 0.10 | 0.53 | 0.14 | 0.58 | 0.12 | 0.67 | 0.13 | 0.61 | 0.17 | 0.15 | 0.17 | ||
Total | 102 | 100 | 101 | 100 | 99.8 | 101 | 101 | 99.4 | 100 | 99.3 | 100 | 99.3 | 100 | 99.5 | 100 | 100 | 100 | 99.7 | 99.7 | 101 | ||
Si | 0.00 | 1.00 | 0.00 | 0.00 | 0.00 | 0.00 | 0.00 | 0.00 | 1.00 | 0.00 | 1.00 | 0.00 | 1.00 | 0.00 | 1.00 | 0.00 | 1.00 | 0.00 | 0.00 | 0.00 | ||
Ti | 0.01 | 0.00 | 0.00 | 0.00 | 0.00 | 0.00 | 0.01 | 0.00 | 0.00 | 0.00 | 0.00 | 0.00 | 0.00 | 0.00 | 0.00 | 0.01 | 0.00 | 0.00 | 0.00 | 0.01 | ||
Al | 0.39 | 0.00 | 0.41 | 0.45 | 0.39 | 0.48 | 0.38 | 0.38 | 0.00 | 0.41 | 0.00 | 0.40 | 0.00 | 0.46 | 0.00 | 0.41 | 0.00 | 0.41 | 0.47 | 0.42 | ||
Cr | 1.54 | 0.00 | 1.49 | 1.48 | 1.54 | 1.44 | 1.55 | 1.51 | 0.00 | 1.50 | 0.00 | 1.49 | 0.00 | 1.45 | 0.00 | 1.49 | 0.00 | 1.51 | 1.43 | 1.50 | ||
Fe3+ | 0.06 | 0.09 | 0.06 | 0.06 | 0.07 | 0.06 | 0.10 | 0.08 | 0.11 | 0.08 | 0.09 | 0.08 | 0.09 | 0.06 | ||||||||
Fe2+ | 0.37 | 0.09 | 0.29 | 0.36 | 0.35 | 0.26 | 0.26 | 0.32 | 0.09 | 0.31 | 0.09 | 0.25 | 0.06 | 0.28 | 0.07 | 0.26 | 0.07 | 0.26 | 0.26 | 0.26 | ||
Mn | 0.00 | 0.00 | 0.01 | 0.01 | 0.01 | 0.01 | 0.00 | 0.00 | 0.00 | 0.00 | 0.00 | 0.00 | 0.00 | 0.00 | 0.00 | 0.00 | 0.00 | 0.00 | 0.00 | 0.00 | ||
Mg | 0.62 | 1.89 | 0.71 | 0.63 | 0.64 | 0.74 | 0.73 | 0.68 | 1.90 | 0.69 | 1.89 | 0.74 | 1.91 | 0.72 | 1.91 | 0.74 | 1.91 | 0.74 | 0.73 | 0.74 | ||
Ca | 0.00 | 0.00 | 0.00 | 0.00 | 0.00 | 0.00 | 0.00 | 0.00 | 0.00 | 0.00 | 0.00 | 0.00 | 0.00 | 0.00 | 0.00 | 0.00 | 0.00 | 0.00 | 0.00 | 0.00 | ||
Na | 0.00 | 0.00 | 0.00 | 0.00 | 0.00 | 0.00 | 0.00 | 0.00 | 0.00 | 0.00 | 0.00 | 0.00 | 0.00 | 0.00 | 0.00 | 0.00 | 0.00 | 0.00 | 0.00 | 0.00 | ||
K | 0.00 | 0.00 | 0.00 | 0.00 | 0.00 | 0.00 | 0.00 | 0.00 | 0.00 | 0.00 | 0.00 | 0.00 | 0.00 | 0.00 | 0.00 | 0.00 | 0.00 | 0.00 | 0.00 | 0.00 | ||
Ni | 0.00 | 0.01 | 0.00 | 0.00 | 0.00 | 0.00 | 0.01 | 0.00 | 0.01 | 0.00 | 0.01 | 0.00 | 0.01 | 0.00 | 0.01 | 0.00 | 0.01 | 0.00 | 0.00 | 0.00 | ||
Total | 3.00 | 3.00 | 3.00 | 3.00 | 3.00 | 3.00 | 3.00 | 3.00 | 3.00 | 3.00 | 3.00 | 3.00 | 3.00 | 3.00 | 3.00 | 3.00 | 3.00 | 3.00 | 3.00 | 3.00 | ||
Mg#b | 62.5 | 95.3 | 71.1 | 63.6 | 64.7 | 74.4 | 74.7 | 68.2 | 95.7 | 68.9 | 95.6 | 74.8 | 96.8 | 72.0 | 96.4 | 73.7 | 96.5 | 74.1 | 73.6 | 74.4 | ||
Cr#b | 79.8 | 78.6 | 76.9 | 79.7 | 75.0 | 78.8 | 79.7 | 78.8 | 78.9 | 75.8 | 78.3 | 78.8 | 75.1 | 78.0 | ||||||||
Fe3+/∑Feb | 0.13 | 0.25 | 0.14 | 0.14 | 0.23 | 0.18 | 0.23 | 0.21 | 0.30 | 0.22 | 0.26 | 0.24 | 0.26 | 0.20 | ||||||||
SiO2 | 0.05 | 41.6 | 0.02 | 42.0 | 0.03 | 41.4 | 0.02 | 41.6 | 0.05 | 40.8 | 0.00 | 41.5 | 0.03 | 41.6 | 0.00 | 41.6 | 0.02 | 42.1 | 0.10 | 41.4 | 0.06 | 41.5 |
TiO2 | 0.15 | 0.00 | 0.06 | 0.05 | 0.28 | 0.00 | 0.18 | 0.00 | 0.05 | 0.02 | 0.16 | 0.03 | 0.36 | 0.00 | 0.36 | 0.00 | 0.23 | 0.03 | 0.27 | 0.00 | 0.39 | 0.04 |
Al2O3 | 15.6 | 0.00 | 22.6 | 0.01 | 16.9 | 0.00 | 24.8 | 0.00 | 27.3 | 0.00 | 21.8 | 0.00 | 20.9 | 0.00 | 20.3 | 0.00 | 15.1 | 0.01 | 21.3 | 0.01 | 20.3 | 0.01 |
Cr2O3 | 50.2 | 0.00 | 41.9 | 0.00 | 51.3 | 0.02 | 42.5 | 0.04 | 37.6 | 0.06 | 46.0 | 0.14 | 44.9 | 0.00 | 44.5 | 0.00 | 53.4 | 0.04 | 44.3 | 0.01 | 46.4 | 0.00 |
FeO | 22.4 | 7.23 | 23.4 | 8.99 | 19.0 | 7.11 | 18.6 | 8.95 | 20.8 | 8.33 | 18.7 | 8.64 | 21.9 | 9.52 | 23.9 | 9.52 | 16.6 | 5.91 | 21.5 | 9.03 | 17.9 | 7.48 |
MnO | 0.22 | 0.12 | 0.17 | 0.10 | 0.16 | 0.12 | 0.13 | 0.14 | 0.00 | 0.09 | 0.00 | 0.14 | 0.21 | 0.13 | 0.18 | 0.13 | 0.13 | 0.06 | 0.22 | 0.11 | 0.16 | 0.11 |
MgO | 10.7 | 51.6 | 12.0 | 49.9 | 12.7 | 49.4 | 13.5 | 49.2 | 13.3 | 49.3 | 12.6 | 49.4 | 12.1 | 49.0 | 11.3 | 49.0 | 14.5 | 51.1 | 12.1 | 48.9 | 13.9 | 51.0 |
CaO | 0.03 | 0.11 | 0.00 | 0.11 | 0.00 | 0.21 | 0.00 | 0.04 | 0.00 | 0.03 | 0.03 | 0.12 | 0.00 | 0.07 | 0.02 | 0.07 | 0.00 | 0.15 | 0.04 | 0.07 | 0.03 | 0.02 |
Na2O | 0.00 | 0.00 | 0.01 | 0.00 | 0.00 | 0.01 | 0.00 | 0.00 | 0.00 | 0.00 | 0.00 | 0.00 | 0.04 | 0.00 | 0.00 | 0.00 | 0.00 | 0.00 | 0.03 | 0.00 | 0.03 | 0.01 |
K2O | 0.00 | 0.00 | 0.00 | 0.00 | 0.00 | 0.00 | 0.00 | 0.02 | 0.00 | 0.00 | 0.00 | 0.00 | 0.00 | 0.01 | 0.00 | 0.01 | 0.00 | 0.00 | 0.00 | 0.00 | 0.00 | 0.00 |
NiO | 0.07 | 0.39 | 0.13 | 0.38 | 0.08 | 0.42 | 0.16 | 0.34 | 0.13 | 0.39 | 0.11 | 0.37 | 0.11 | 0.36 | 0.12 | 0.36 | 0.13 | 0.43 | 0.10 | 0.33 | 0.12 | 0.38 |
Total | 99.5 | 101 | 100 | 101 | 100 | 99 | 99.9 | 100 | 99.2 | 99 | 99.4 | 100 | 100 | 101 | 101 | 101 | 100 | 100 | 100 | 100 | 99.3 | 101 |
Si | 0.00 | 1.00 | 0.00 | 1.01 | 0.00 | 1.02 | 0.00 | 1.01 | 0.00 | 1.00 | 0.00 | 1.01 | 0.00 | 1.01 | 0.00 | 1.01 | 0.00 | 1.01 | 0.00 | 1.01 | 0.00 | 1.00 |
Ti | 0.00 | 0.00 | 0.00 | 0.00 | 0.01 | 0.00 | 0.00 | 0.00 | 0.00 | 0.00 | 0.00 | 0.00 | 0.01 | 0.00 | 0.01 | 0.00 | 0.01 | 0.00 | 0.01 | 0.00 | 0.01 | 0.00 |
Al | 0.59 | 0.00 | 0.82 | 0.00 | 0.63 | 0.00 | 0.89 | 0.00 | 0.97 | 0.00 | 0.80 | 0.00 | 0.76 | 0.00 | 0.74 | 0.00 | 0.56 | 0.00 | 0.78 | 0.00 | 0.74 | 0.00 |
Cr | 1.28 | 0.00 | 1.02 | 0.00 | 1.27 | 0.00 | 1.02 | 0.00 | 0.90 | 0.00 | 1.13 | 0.00 | 1.10 | 0.00 | 1.09 | 0.00 | 1.32 | 0.00 | 1.08 | 0.00 | 1.13 | 0.00 |
Fe3+ | 0.12 | 0.16 | 0.09 | 0.09 | 0.12 | 0.07 | 0.13 | 0.15 | 0.11 | 0.12 | 0.11 | |||||||||||
Fe2+ | 0.48 | 0.14 | 0.44 | 0.18 | 0.41 | 0.15 | 0.39 | 0.18 | 0.40 | 0.17 | 0.42 | 0.18 | 0.44 | 0.19 | 0.48 | 0.19 | 0.32 | 0.12 | 0.44 | 0.18 | 0.36 | 0.15 |
Mn | 0.01 | 0.00 | 0.00 | 0.00 | 0.00 | 0.00 | 0.00 | 0.00 | 0.00 | 0.00 | 0.00 | 0.00 | 0.01 | 0.00 | 0.00 | 0.00 | 0.00 | 0.00 | 0.01 | 0.00 | 0.00 | 0.00 |
Mg | 0.51 | 1.85 | 0.55 | 1.79 | 0.59 | 1.81 | 0.61 | 1.79 | 0.60 | 1.81 | 0.58 | 1.79 | 0.56 | 1.77 | 0.52 | 1.77 | 0.67 | 1.84 | 0.56 | 1.78 | 0.64 | 1.83 |
Ca | 0.00 | 0.00 | 0.00 | 0.00 | 0.00 | 0.01 | 0.00 | 0.00 | 0.00 | 0.00 | 0.00 | 0.00 | 0.00 | 0.00 | 0.00 | 0.00 | 0.00 | 0.00 | 0.00 | 0.00 | 0.00 | 0.00 |
Na | 0.00 | 0.00 | 0.00 | 0.00 | 0.00 | 0.00 | 0.00 | 0.00 | 0.00 | 0.00 | 0.00 | 0.00 | 0.00 | 0.00 | 0.00 | 0.00 | 0.00 | 0.00 | 0.00 | 0.00 | 0.00 | 0.00 |
K | 0.00 | 0.00 | 0.00 | 0.00 | 0.00 | 0.00 | 0.00 | 0.00 | 0.00 | 0.00 | 0.00 | 0.00 | 0.00 | 0.00 | 0.00 | 0.00 | 0.00 | 0.00 | 0.00 | 0.00 | 0.00 | 0.00 |
Ni | 0.00 | 0.01 | 0.00 | 0.01 | 0.00 | 0.01 | 0.00 | 0.01 | 0.00 | 0.01 | 0.00 | 0.01 | 0.00 | 0.01 | 0.00 | 0.01 | 0.00 | 0.01 | 0.00 | 0.01 | 0.00 | 0.01 |
Total | 3.00 | 3.00 | 3.00 | 2.99 | 3.00 | 2.98 | 3.00 | 2.99 | 3.00 | 3.00 | 3.00 | 2.99 | 3.00 | 2.99 | 3.00 | 2.99 | 3.00 | 2.98 | 3.00 | 2.99 | 3.00 | 3.00 |
Mg# | 51.4 | 92.7 | 55.3 | 90.8 | 59.1 | 92.5 | 61.2 | 90.7 | 59.9 | 91.3 | 58.3 | 91.1 | 56.0 | 90.2 | 52.4 | 90.2 | 67.6 | 93.9 | 56.2 | 90.6 | 64.3 | 92.4 |
Cr# | 68.3 | 55.5 | 67.0 | 53.5 | 48.0 | 58.6 | 59.1 | 59.5 | 70.3 | 58.3 | 60.5 | |||||||||||
Fe3+/∑Fe | 0.20 | 0.26 | 0.18 | 0.18 | 0.24 | 0.14 | 0.22 | 0.23 | 0.25 | 0.22 | 0.23 | |||||||||||
SiO2 | 0.00 | 41.3 | 0.00 | 41.3 | 0.05 | 41.5 | 0.09 | 0.00 | 42.1 | 0.00 | 42.2 | 0.00 | 42.3 | 0.02 | 42.0 | 0.00 | 42.0 | 0.00 | 42.0 | 0.01 | 41.5 | |
TiO2 | 0.15 | 0.00 | 0.30 | 0.00 | 0.10 | 0.00 | 0.28 | 0.26 | 0.05 | 0.20 | 0.00 | 0.17 | 0.00 | 0.22 | 0.00 | 0.21 | 0.00 | 0.20 | 0.00 | 0.08 | 0.00 | |
Al2O3 | 20.9 | 0.00 | 20.2 | 0.00 | 33.1 | 0.00 | 18.7 | 20.6 | 0.00 | 20.4 | 0.01 | 12.4 | 0.00 | 15.6 | 0.00 | 15.3 | 0.00 | 12.7 | 0.00 | 21.5 | 0.01 | |
Cr2O3 | 46.8 | 0.01 | 47.0 | 0.00 | 34.1 | 0.01 | 49.3 | 48.2 | 0.06 | 46.9 | 0.05 | 55.6 | 0.05 | 53.1 | 0.03 | 53.2 | 0.04 | 56.9 | 0.00 | 46.7 | 0.08 | |
FeO | 18.4 | 8.95 | 20.0 | 7.68 | 17.5 | 8.54 | 17.3 | 15.7 | 5.10 | 16.9 | 5.94 | 16.5 | 3.67 | 15.2 | 5.07 | 16.0 | 5.19 | 14.5 | 4.90 | 15.2 | 7.55 | |
MnO | 0.12 | 0.14 | 0.15 | 0.10 | 0.00 | 0.11 | 0.00 | 0.00 | 0.05 | 0.00 | 0.06 | 0.00 | 0.06 | 0.00 | 0.04 | 0.00 | 0.08 | 0.00 | 0.09 | 0.00 | 0.09 | |
MgO | 13.4 | 48.7 | 12.6 | 49.4 | 14.8 | 49.3 | 13.7 | 15.1 | 52.1 | 14.0 | 52.0 | 13.6 | 52.8 | 14.9 | 52.2 | 14.4 | 51.8 | 14.6 | 51.7 | 15.1 | 49.6 | |
CaO | 0.00 | 0.06 | 0.01 | 0.03 | 0.00 | 0.11 | 0.02 | 0.05 | 0.02 | 0.03 | 0.02 | 0.03 | 0.05 | 0.00 | 0.08 | 0.00 | 0.07 | 0.02 | 0.07 | 0.00 | 0.01 | |
Na2O | 0.00 | 0.00 | 0.02 | 0.00 | 0.00 | 0.00 | 0.00 | 0.00 | 0.00 | 0.00 | 0.00 | 0.00 | 0.00 | 0.01 | 0.00 | 0.01 | 0.00 | 0.00 | 0.00 | 0.00 | 0.00 | |
K2O | 0.00 | 0.00 | 0.00 | 0.01 | 0.00 | 0.00 | 0.00 | 0.00 | 0.00 | 0.00 | 0.01 | 0.00 | 0.03 | 0.00 | 0.00 | 0.00 | 0.00 | 0.00 | 0.00 | 0.00 | 0.00 | |
NiO | 0.10 | 0.37 | 0.13 | 0.42 | 0.13 | 0.37 | 0.12 | 0.09 | 0.56 | 0.15 | 0.43 | 0.09 | 0.57 | 0.16 | 0.52 | 0.12 | 0.44 | 0.16 | 0.53 | 0.10 | 0.51 | |
Total | 99.9 | 100 | 100 | 99 | 99.8 | 100 | 99.5 | 100 | 100 | 98.6 | 101 | 98.4 | 100 | 99.2 | 100 | 99.2 | 100 | 99.1 | 99 | 98.7 | 99 | |
Si | 0.00 | 1.01 | 0.00 | 1.01 | 0.00 | 1.01 | 0.00 | 0.00 | 1.01 | 0.00 | 1.01 | 0.00 | 1.01 | 0.00 | 1.01 | 0.00 | 1.01 | 0.00 | 1.01 | 0.00 | 1.01 | |
Ti | 0.00 | 0.00 | 0.01 | 0.00 | 0.00 | 0.00 | 0.01 | 0.01 | 0.00 | 0.00 | 0.00 | 0.00 | 0.00 | 0.01 | 0.00 | 0.00 | 0.00 | 0.00 | 0.00 | 0.00 | 0.00 | |
Al | 0.57 | 0.00 | 0.74 | 0.00 | 1.14 | 0.00 | 0.69 | 0.74 | 0.00 | 0.75 | 0.00 | 0.47 | 0.00 | 0.58 | 0.00 | 0.76 | 0.00 | 0.48 | 0.00 | 0.78 | 0.00 | |
Cr | 1.33 | 0.00 | 1.15 | 0.00 | 0.79 | 0.00 | 1.21 | 1.16 | 0.00 | 1.15 | 0.00 | 1.42 | 0.00 | 1.32 | 0.00 | 1.14 | 0.00 | 1.43 | 0.00 | 1.13 | 0.00 | |
Fe3+ | 0.10 | 0.10 | 0.07 | 0.08 | 0.08 | 0.09 | 0.10 | 0.10 | 0.09 | 0.08 | 0.08 | |||||||||||
Fe2+ | 0.32 | 0.18 | 0.42 | 0.16 | 0.36 | 0.17 | 0.37 | 0.32 | 0.10 | 0.35 | 0.12 | 0.35 | 0.07 | 0.30 | 0.10 | 0.38 | 0.10 | 0.31 | 0.10 | 0.31 | 0.15 | |
Mn | 0.00 | 0.00 | 0.00 | 0.00 | 0.00 | 0.00 | 0.00 | 0.00 | 0.00 | 0.00 | 0.00 | 0.00 | 0.00 | 0.00 | 0.00 | 0.00 | 0.00 | 0.00 | 0.00 | 0.00 | 0.00 | |
Mg | 0.68 | 1.78 | 0.58 | 1.81 | 0.64 | 1.79 | 0.64 | 0.69 | 1.86 | 0.65 | 1.85 | 0.65 | 1.89 | 0.70 | 1.87 | 0.62 | 1.86 | 0.69 | 1.86 | 0.69 | 1.80 | |
Ca | 0.00 | 0.00 | 0.00 | 0.00 | 0.00 | 0.00 | 0.00 | 0.00 | 0.00 | 0.00 | 0.00 | 0.00 | 0.00 | 0.00 | 0.00 | 0.00 | 0.00 | 0.00 | 0.00 | 0.00 | 0.00 | |
Na | 0.00 | 0.00 | 0.00 | 0.00 | 0.00 | 0.00 | 0.00 | 0.00 | 0.00 | 0.00 | 0.00 | 0.00 | 0.00 | 0.00 | 0.00 | 0.00 | 0.00 | 0.00 | 0.00 | 0.00 | 0.00 | |
K | 0.00 | 0.00 | 0.00 | 0.00 | 0.00 | 0.00 | 0.00 | 0.00 | 0.00 | 0.00 | 0.00 | 0.00 | 0.00 | 0.00 | 0.00 | 0.00 | 0.00 | 0.00 | 0.00 | 0.00 | 0.00 | |
Ni | 0.00 | 0.01 | 0.00 | 0.01 | 0.00 | 0.01 | 0.00 | 0.00 | 0.01 | 0.00 | 0.01 | 0.00 | 0.01 | 0.00 | 0.01 | 0.00 | 0.01 | 0.00 | 0.01 | 0.00 | 0.01 | |
Total | 3.00 | 2.99 | 3.00 | 2.99 | 3.00 | 2.99 | 3.00 | 3.00 | 2.99 | 3.00 | 2.99 | 3.00 | 2.99 | 3.00 | 2.99 | 3.00 | 2.99 | 3.00 | 2.99 | 3.00 | 2.99 | |
Mg# | 61.9 | 90.7 | 58.3 | 92.0 | 64.3 | 91.1 | 63.2 | 68.5 | 94.8 | 64.9 | 94.0 | 65.4 | 96.2 | 69.6 | 94.8 | 67.6 | 94.7 | 69.3 | 95.0 | 69.2 | 92.1 | |
Cr# | 60.0 | 61.0 | 40.9 | 63.9 | 61.1 | 60.7 | 75.0 | 69.5 | 70.0 | 75.0 | 59.3 | |||||||||||
Fe3+/∑Fe | 0.20 | 0.21 | 0.16 | 0.18 | 0.21 | 0.20 | 0.22 | 0.24 | 0.20 | 0.21 | 0.21 |
Locality | Luobusa | |||||||||||||||||||||
Sample | LBS09005–8 | LBS13–21 | LBS13–22 | LBS13–23 | LBS13–34 | LBS13–39 | L1 | L2 | L4 | L3 | L5 | L6 | L7 | L06a | ||||||||
Rock type | Chromitite | Chromitite | Chromitite | Chromitite | Chromitite | Chromitite | Chromitite | Chromitite | Chromitite | Chromitite | Chromitite | Chromitite | Chromitite | Chromitite | ||||||||
Mineral | Chra | Ola | Chr | Chr | Chr | Chr | Chr | Chr | Ol | Chr | Ol | Chr | Ol | Chr | Ol | Chr | Ol | Chr | Chr | Chr | ||
Point | 8 | 8 | 8 | 8 | 8 | 8 | 8 | 8 | 8 | 8 | 8 | 8 | 8 | 8 | 8 | 8 | 8 | 8 | 8 | 8 | ||
SiO2 | 0.00 | 41.81 | 0.05 | 0.00 | 0.03 | 0.01 | 0.01 | 0.02 | 42.0 | 0.00 | 42.0 | 0.03 | 42.1 | 0.00 | 41.8 | 0.02 | 42.1 | 0.00 | 0.00 | 0.00 | ||
TiO2 | 0.23 | 0.00 | 0.21 | 0.20 | 0.13 | 0.19 | 0.23 | 0.18 | 0.03 | 0.19 | 0.02 | 0.19 | 0.01 | 0.20 | 0.01 | 0.22 | 0.00 | 0.19 | 0.16 | 0.22 | ||
Al2O3 | 10.4 | 0.00 | 11.0 | 11.9 | 10.3 | 13.1 | 11.0 | 10.2 | 0.00 | 10.7 | 0.00 | 10.6 | 0.00 | 12.4 | 0.01 | 11.1 | 0.00 | 10.9 | 12.8 | 11.6 | ||
Cr2O3 | 61.5 | 0.02 | 60.0 | 58.8 | 60.5 | 58.7 | 60.8 | 59.4 | 0.01 | 59.3 | 0.02 | 59.2 | 0.02 | 57.9 | 0.01 | 59.8 | 0.02 | 60.1 | 57.5 | 61.2 | ||
FeO | 16.3 | 4.69 | 14.6 | 15.8 | 15.2 | 12.8 | 12.0 | 15.4 | 4.34 | 14.6 | 4.43 | 13.4 | 3.17 | 13.6 | 3.59 | 13.5 | 3.52 | 12.8 | 13.5 | 12.4 | ||
MnO | 0.15 | 0.08 | 0.22 | 0.23 | 0.22 | 0.20 | 0.20 | 0.13 | 0.07 | 0.11 | 0.03 | 0.09 | 0.02 | 0.11 | 0.04 | 0.10 | 0.04 | 0.10 | 0.08 | 0.05 | ||
MgO | 13.2 | 53.0 | 15.2 | 13.3 | 13.4 | 16.0 | 16.5 | 14.1 | 53.3 | 14.4 | 53.2 | 15.7 | 53.7 | 15.2 | 53.6 | 15.7 | 54.0 | 15.6 | 15.7 | 16.0 | ||
CaO | 0.01 | 0.04 | 0.00 | 0.00 | 0.00 | 0.01 | 0.00 | 0.00 | 0.00 | 0.01 | 0.01 | 0.01 | 0.05 | 0.00 | 0.02 | 0.01 | 0.05 | 0.01 | 0.00 | 0.02 | ||
Na2O | 0.02 | 0.01 | 0.01 | 0.00 | 0.02 | 0.00 | 0.01 | 0.05 | 0.00 | 0.01 | 0.01 | 0.04 | 0.01 | 0.01 | 0.00 | 0.01 | 0.00 | 0.01 | 0.01 | 0.00 | ||
K2O | 0.00 | 0.00 | 0.00 | 0.00 | 0.00 | 0.00 | 0.00 | 0.01 | 0.01 | 0.01 | 0.00 | 0.01 | 0.01 | 0.00 | 0.00 | 0.01 | 0.01 | 0.01 | 0.01 | 0.00 | ||
NiO | 0.09 | 0.53 | 0.10 | 0.12 | 0.04 | 0.15 | 0.20 | 0.10 | 0.49 | 0.10 | 0.53 | 0.14 | 0.58 | 0.12 | 0.67 | 0.13 | 0.61 | 0.17 | 0.15 | 0.17 | ||
Total | 102 | 100 | 101 | 100 | 99.8 | 101 | 101 | 99.4 | 100 | 99.3 | 100 | 99.3 | 100 | 99.5 | 100 | 100 | 100 | 99.7 | 99.7 | 101 | ||
Si | 0.00 | 1.00 | 0.00 | 0.00 | 0.00 | 0.00 | 0.00 | 0.00 | 1.00 | 0.00 | 1.00 | 0.00 | 1.00 | 0.00 | 1.00 | 0.00 | 1.00 | 0.00 | 0.00 | 0.00 | ||
Ti | 0.01 | 0.00 | 0.00 | 0.00 | 0.00 | 0.00 | 0.01 | 0.00 | 0.00 | 0.00 | 0.00 | 0.00 | 0.00 | 0.00 | 0.00 | 0.01 | 0.00 | 0.00 | 0.00 | 0.01 | ||
Al | 0.39 | 0.00 | 0.41 | 0.45 | 0.39 | 0.48 | 0.38 | 0.38 | 0.00 | 0.41 | 0.00 | 0.40 | 0.00 | 0.46 | 0.00 | 0.41 | 0.00 | 0.41 | 0.47 | 0.42 | ||
Cr | 1.54 | 0.00 | 1.49 | 1.48 | 1.54 | 1.44 | 1.55 | 1.51 | 0.00 | 1.50 | 0.00 | 1.49 | 0.00 | 1.45 | 0.00 | 1.49 | 0.00 | 1.51 | 1.43 | 1.50 | ||
Fe3+ | 0.06 | 0.09 | 0.06 | 0.06 | 0.07 | 0.06 | 0.10 | 0.08 | 0.11 | 0.08 | 0.09 | 0.08 | 0.09 | 0.06 | ||||||||
Fe2+ | 0.37 | 0.09 | 0.29 | 0.36 | 0.35 | 0.26 | 0.26 | 0.32 | 0.09 | 0.31 | 0.09 | 0.25 | 0.06 | 0.28 | 0.07 | 0.26 | 0.07 | 0.26 | 0.26 | 0.26 | ||
Mn | 0.00 | 0.00 | 0.01 | 0.01 | 0.01 | 0.01 | 0.00 | 0.00 | 0.00 | 0.00 | 0.00 | 0.00 | 0.00 | 0.00 | 0.00 | 0.00 | 0.00 | 0.00 | 0.00 | 0.00 | ||
Mg | 0.62 | 1.89 | 0.71 | 0.63 | 0.64 | 0.74 | 0.73 | 0.68 | 1.90 | 0.69 | 1.89 | 0.74 | 1.91 | 0.72 | 1.91 | 0.74 | 1.91 | 0.74 | 0.73 | 0.74 | ||
Ca | 0.00 | 0.00 | 0.00 | 0.00 | 0.00 | 0.00 | 0.00 | 0.00 | 0.00 | 0.00 | 0.00 | 0.00 | 0.00 | 0.00 | 0.00 | 0.00 | 0.00 | 0.00 | 0.00 | 0.00 | ||
Na | 0.00 | 0.00 | 0.00 | 0.00 | 0.00 | 0.00 | 0.00 | 0.00 | 0.00 | 0.00 | 0.00 | 0.00 | 0.00 | 0.00 | 0.00 | 0.00 | 0.00 | 0.00 | 0.00 | 0.00 | ||
K | 0.00 | 0.00 | 0.00 | 0.00 | 0.00 | 0.00 | 0.00 | 0.00 | 0.00 | 0.00 | 0.00 | 0.00 | 0.00 | 0.00 | 0.00 | 0.00 | 0.00 | 0.00 | 0.00 | 0.00 | ||
Ni | 0.00 | 0.01 | 0.00 | 0.00 | 0.00 | 0.00 | 0.01 | 0.00 | 0.01 | 0.00 | 0.01 | 0.00 | 0.01 | 0.00 | 0.01 | 0.00 | 0.01 | 0.00 | 0.00 | 0.00 | ||
Total | 3.00 | 3.00 | 3.00 | 3.00 | 3.00 | 3.00 | 3.00 | 3.00 | 3.00 | 3.00 | 3.00 | 3.00 | 3.00 | 3.00 | 3.00 | 3.00 | 3.00 | 3.00 | 3.00 | 3.00 | ||
Mg#b | 62.5 | 95.3 | 71.1 | 63.6 | 64.7 | 74.4 | 74.7 | 68.2 | 95.7 | 68.9 | 95.6 | 74.8 | 96.8 | 72.0 | 96.4 | 73.7 | 96.5 | 74.1 | 73.6 | 74.4 | ||
Cr#b | 79.8 | 78.6 | 76.9 | 79.7 | 75.0 | 78.8 | 79.7 | 78.8 | 78.9 | 75.8 | 78.3 | 78.8 | 75.1 | 78.0 | ||||||||
Fe3+/∑Feb | 0.13 | 0.25 | 0.14 | 0.14 | 0.23 | 0.18 | 0.23 | 0.21 | 0.30 | 0.22 | 0.26 | 0.24 | 0.26 | 0.20 | ||||||||
SiO2 | 0.05 | 41.6 | 0.02 | 42.0 | 0.03 | 41.4 | 0.02 | 41.6 | 0.05 | 40.8 | 0.00 | 41.5 | 0.03 | 41.6 | 0.00 | 41.6 | 0.02 | 42.1 | 0.10 | 41.4 | 0.06 | 41.5 |
TiO2 | 0.15 | 0.00 | 0.06 | 0.05 | 0.28 | 0.00 | 0.18 | 0.00 | 0.05 | 0.02 | 0.16 | 0.03 | 0.36 | 0.00 | 0.36 | 0.00 | 0.23 | 0.03 | 0.27 | 0.00 | 0.39 | 0.04 |
Al2O3 | 15.6 | 0.00 | 22.6 | 0.01 | 16.9 | 0.00 | 24.8 | 0.00 | 27.3 | 0.00 | 21.8 | 0.00 | 20.9 | 0.00 | 20.3 | 0.00 | 15.1 | 0.01 | 21.3 | 0.01 | 20.3 | 0.01 |
Cr2O3 | 50.2 | 0.00 | 41.9 | 0.00 | 51.3 | 0.02 | 42.5 | 0.04 | 37.6 | 0.06 | 46.0 | 0.14 | 44.9 | 0.00 | 44.5 | 0.00 | 53.4 | 0.04 | 44.3 | 0.01 | 46.4 | 0.00 |
FeO | 22.4 | 7.23 | 23.4 | 8.99 | 19.0 | 7.11 | 18.6 | 8.95 | 20.8 | 8.33 | 18.7 | 8.64 | 21.9 | 9.52 | 23.9 | 9.52 | 16.6 | 5.91 | 21.5 | 9.03 | 17.9 | 7.48 |
MnO | 0.22 | 0.12 | 0.17 | 0.10 | 0.16 | 0.12 | 0.13 | 0.14 | 0.00 | 0.09 | 0.00 | 0.14 | 0.21 | 0.13 | 0.18 | 0.13 | 0.13 | 0.06 | 0.22 | 0.11 | 0.16 | 0.11 |
MgO | 10.7 | 51.6 | 12.0 | 49.9 | 12.7 | 49.4 | 13.5 | 49.2 | 13.3 | 49.3 | 12.6 | 49.4 | 12.1 | 49.0 | 11.3 | 49.0 | 14.5 | 51.1 | 12.1 | 48.9 | 13.9 | 51.0 |
CaO | 0.03 | 0.11 | 0.00 | 0.11 | 0.00 | 0.21 | 0.00 | 0.04 | 0.00 | 0.03 | 0.03 | 0.12 | 0.00 | 0.07 | 0.02 | 0.07 | 0.00 | 0.15 | 0.04 | 0.07 | 0.03 | 0.02 |
Na2O | 0.00 | 0.00 | 0.01 | 0.00 | 0.00 | 0.01 | 0.00 | 0.00 | 0.00 | 0.00 | 0.00 | 0.00 | 0.04 | 0.00 | 0.00 | 0.00 | 0.00 | 0.00 | 0.03 | 0.00 | 0.03 | 0.01 |
K2O | 0.00 | 0.00 | 0.00 | 0.00 | 0.00 | 0.00 | 0.00 | 0.02 | 0.00 | 0.00 | 0.00 | 0.00 | 0.00 | 0.01 | 0.00 | 0.01 | 0.00 | 0.00 | 0.00 | 0.00 | 0.00 | 0.00 |
NiO | 0.07 | 0.39 | 0.13 | 0.38 | 0.08 | 0.42 | 0.16 | 0.34 | 0.13 | 0.39 | 0.11 | 0.37 | 0.11 | 0.36 | 0.12 | 0.36 | 0.13 | 0.43 | 0.10 | 0.33 | 0.12 | 0.38 |
Total | 99.5 | 101 | 100 | 101 | 100 | 99 | 99.9 | 100 | 99.2 | 99 | 99.4 | 100 | 100 | 101 | 101 | 101 | 100 | 100 | 100 | 100 | 99.3 | 101 |
Si | 0.00 | 1.00 | 0.00 | 1.01 | 0.00 | 1.02 | 0.00 | 1.01 | 0.00 | 1.00 | 0.00 | 1.01 | 0.00 | 1.01 | 0.00 | 1.01 | 0.00 | 1.01 | 0.00 | 1.01 | 0.00 | 1.00 |
Ti | 0.00 | 0.00 | 0.00 | 0.00 | 0.01 | 0.00 | 0.00 | 0.00 | 0.00 | 0.00 | 0.00 | 0.00 | 0.01 | 0.00 | 0.01 | 0.00 | 0.01 | 0.00 | 0.01 | 0.00 | 0.01 | 0.00 |
Al | 0.59 | 0.00 | 0.82 | 0.00 | 0.63 | 0.00 | 0.89 | 0.00 | 0.97 | 0.00 | 0.80 | 0.00 | 0.76 | 0.00 | 0.74 | 0.00 | 0.56 | 0.00 | 0.78 | 0.00 | 0.74 | 0.00 |
Cr | 1.28 | 0.00 | 1.02 | 0.00 | 1.27 | 0.00 | 1.02 | 0.00 | 0.90 | 0.00 | 1.13 | 0.00 | 1.10 | 0.00 | 1.09 | 0.00 | 1.32 | 0.00 | 1.08 | 0.00 | 1.13 | 0.00 |
Fe3+ | 0.12 | 0.16 | 0.09 | 0.09 | 0.12 | 0.07 | 0.13 | 0.15 | 0.11 | 0.12 | 0.11 | |||||||||||
Fe2+ | 0.48 | 0.14 | 0.44 | 0.18 | 0.41 | 0.15 | 0.39 | 0.18 | 0.40 | 0.17 | 0.42 | 0.18 | 0.44 | 0.19 | 0.48 | 0.19 | 0.32 | 0.12 | 0.44 | 0.18 | 0.36 | 0.15 |
Mn | 0.01 | 0.00 | 0.00 | 0.00 | 0.00 | 0.00 | 0.00 | 0.00 | 0.00 | 0.00 | 0.00 | 0.00 | 0.01 | 0.00 | 0.00 | 0.00 | 0.00 | 0.00 | 0.01 | 0.00 | 0.00 | 0.00 |
Mg | 0.51 | 1.85 | 0.55 | 1.79 | 0.59 | 1.81 | 0.61 | 1.79 | 0.60 | 1.81 | 0.58 | 1.79 | 0.56 | 1.77 | 0.52 | 1.77 | 0.67 | 1.84 | 0.56 | 1.78 | 0.64 | 1.83 |
Ca | 0.00 | 0.00 | 0.00 | 0.00 | 0.00 | 0.01 | 0.00 | 0.00 | 0.00 | 0.00 | 0.00 | 0.00 | 0.00 | 0.00 | 0.00 | 0.00 | 0.00 | 0.00 | 0.00 | 0.00 | 0.00 | 0.00 |
Na | 0.00 | 0.00 | 0.00 | 0.00 | 0.00 | 0.00 | 0.00 | 0.00 | 0.00 | 0.00 | 0.00 | 0.00 | 0.00 | 0.00 | 0.00 | 0.00 | 0.00 | 0.00 | 0.00 | 0.00 | 0.00 | 0.00 |
K | 0.00 | 0.00 | 0.00 | 0.00 | 0.00 | 0.00 | 0.00 | 0.00 | 0.00 | 0.00 | 0.00 | 0.00 | 0.00 | 0.00 | 0.00 | 0.00 | 0.00 | 0.00 | 0.00 | 0.00 | 0.00 | 0.00 |
Ni | 0.00 | 0.01 | 0.00 | 0.01 | 0.00 | 0.01 | 0.00 | 0.01 | 0.00 | 0.01 | 0.00 | 0.01 | 0.00 | 0.01 | 0.00 | 0.01 | 0.00 | 0.01 | 0.00 | 0.01 | 0.00 | 0.01 |
Total | 3.00 | 3.00 | 3.00 | 2.99 | 3.00 | 2.98 | 3.00 | 2.99 | 3.00 | 3.00 | 3.00 | 2.99 | 3.00 | 2.99 | 3.00 | 2.99 | 3.00 | 2.98 | 3.00 | 2.99 | 3.00 | 3.00 |
Mg# | 51.4 | 92.7 | 55.3 | 90.8 | 59.1 | 92.5 | 61.2 | 90.7 | 59.9 | 91.3 | 58.3 | 91.1 | 56.0 | 90.2 | 52.4 | 90.2 | 67.6 | 93.9 | 56.2 | 90.6 | 64.3 | 92.4 |
Cr# | 68.3 | 55.5 | 67.0 | 53.5 | 48.0 | 58.6 | 59.1 | 59.5 | 70.3 | 58.3 | 60.5 | |||||||||||
Fe3+/∑Fe | 0.20 | 0.26 | 0.18 | 0.18 | 0.24 | 0.14 | 0.22 | 0.23 | 0.25 | 0.22 | 0.23 | |||||||||||
SiO2 | 0.00 | 41.3 | 0.00 | 41.3 | 0.05 | 41.5 | 0.09 | 0.00 | 42.1 | 0.00 | 42.2 | 0.00 | 42.3 | 0.02 | 42.0 | 0.00 | 42.0 | 0.00 | 42.0 | 0.01 | 41.5 | |
TiO2 | 0.15 | 0.00 | 0.30 | 0.00 | 0.10 | 0.00 | 0.28 | 0.26 | 0.05 | 0.20 | 0.00 | 0.17 | 0.00 | 0.22 | 0.00 | 0.21 | 0.00 | 0.20 | 0.00 | 0.08 | 0.00 | |
Al2O3 | 20.9 | 0.00 | 20.2 | 0.00 | 33.1 | 0.00 | 18.7 | 20.6 | 0.00 | 20.4 | 0.01 | 12.4 | 0.00 | 15.6 | 0.00 | 15.3 | 0.00 | 12.7 | 0.00 | 21.5 | 0.01 | |
Cr2O3 | 46.8 | 0.01 | 47.0 | 0.00 | 34.1 | 0.01 | 49.3 | 48.2 | 0.06 | 46.9 | 0.05 | 55.6 | 0.05 | 53.1 | 0.03 | 53.2 | 0.04 | 56.9 | 0.00 | 46.7 | 0.08 | |
FeO | 18.4 | 8.95 | 20.0 | 7.68 | 17.5 | 8.54 | 17.3 | 15.7 | 5.10 | 16.9 | 5.94 | 16.5 | 3.67 | 15.2 | 5.07 | 16.0 | 5.19 | 14.5 | 4.90 | 15.2 | 7.55 | |
MnO | 0.12 | 0.14 | 0.15 | 0.10 | 0.00 | 0.11 | 0.00 | 0.00 | 0.05 | 0.00 | 0.06 | 0.00 | 0.06 | 0.00 | 0.04 | 0.00 | 0.08 | 0.00 | 0.09 | 0.00 | 0.09 | |
MgO | 13.4 | 48.7 | 12.6 | 49.4 | 14.8 | 49.3 | 13.7 | 15.1 | 52.1 | 14.0 | 52.0 | 13.6 | 52.8 | 14.9 | 52.2 | 14.4 | 51.8 | 14.6 | 51.7 | 15.1 | 49.6 | |
CaO | 0.00 | 0.06 | 0.01 | 0.03 | 0.00 | 0.11 | 0.02 | 0.05 | 0.02 | 0.03 | 0.02 | 0.03 | 0.05 | 0.00 | 0.08 | 0.00 | 0.07 | 0.02 | 0.07 | 0.00 | 0.01 | |
Na2O | 0.00 | 0.00 | 0.02 | 0.00 | 0.00 | 0.00 | 0.00 | 0.00 | 0.00 | 0.00 | 0.00 | 0.00 | 0.00 | 0.01 | 0.00 | 0.01 | 0.00 | 0.00 | 0.00 | 0.00 | 0.00 | |
K2O | 0.00 | 0.00 | 0.00 | 0.01 | 0.00 | 0.00 | 0.00 | 0.00 | 0.00 | 0.00 | 0.01 | 0.00 | 0.03 | 0.00 | 0.00 | 0.00 | 0.00 | 0.00 | 0.00 | 0.00 | 0.00 | |
NiO | 0.10 | 0.37 | 0.13 | 0.42 | 0.13 | 0.37 | 0.12 | 0.09 | 0.56 | 0.15 | 0.43 | 0.09 | 0.57 | 0.16 | 0.52 | 0.12 | 0.44 | 0.16 | 0.53 | 0.10 | 0.51 | |
Total | 99.9 | 100 | 100 | 99 | 99.8 | 100 | 99.5 | 100 | 100 | 98.6 | 101 | 98.4 | 100 | 99.2 | 100 | 99.2 | 100 | 99.1 | 99 | 98.7 | 99 | |
Si | 0.00 | 1.01 | 0.00 | 1.01 | 0.00 | 1.01 | 0.00 | 0.00 | 1.01 | 0.00 | 1.01 | 0.00 | 1.01 | 0.00 | 1.01 | 0.00 | 1.01 | 0.00 | 1.01 | 0.00 | 1.01 | |
Ti | 0.00 | 0.00 | 0.01 | 0.00 | 0.00 | 0.00 | 0.01 | 0.01 | 0.00 | 0.00 | 0.00 | 0.00 | 0.00 | 0.01 | 0.00 | 0.00 | 0.00 | 0.00 | 0.00 | 0.00 | 0.00 | |
Al | 0.57 | 0.00 | 0.74 | 0.00 | 1.14 | 0.00 | 0.69 | 0.74 | 0.00 | 0.75 | 0.00 | 0.47 | 0.00 | 0.58 | 0.00 | 0.76 | 0.00 | 0.48 | 0.00 | 0.78 | 0.00 | |
Cr | 1.33 | 0.00 | 1.15 | 0.00 | 0.79 | 0.00 | 1.21 | 1.16 | 0.00 | 1.15 | 0.00 | 1.42 | 0.00 | 1.32 | 0.00 | 1.14 | 0.00 | 1.43 | 0.00 | 1.13 | 0.00 | |
Fe3+ | 0.10 | 0.10 | 0.07 | 0.08 | 0.08 | 0.09 | 0.10 | 0.10 | 0.09 | 0.08 | 0.08 | |||||||||||
Fe2+ | 0.32 | 0.18 | 0.42 | 0.16 | 0.36 | 0.17 | 0.37 | 0.32 | 0.10 | 0.35 | 0.12 | 0.35 | 0.07 | 0.30 | 0.10 | 0.38 | 0.10 | 0.31 | 0.10 | 0.31 | 0.15 | |
Mn | 0.00 | 0.00 | 0.00 | 0.00 | 0.00 | 0.00 | 0.00 | 0.00 | 0.00 | 0.00 | 0.00 | 0.00 | 0.00 | 0.00 | 0.00 | 0.00 | 0.00 | 0.00 | 0.00 | 0.00 | 0.00 | |
Mg | 0.68 | 1.78 | 0.58 | 1.81 | 0.64 | 1.79 | 0.64 | 0.69 | 1.86 | 0.65 | 1.85 | 0.65 | 1.89 | 0.70 | 1.87 | 0.62 | 1.86 | 0.69 | 1.86 | 0.69 | 1.80 | |
Ca | 0.00 | 0.00 | 0.00 | 0.00 | 0.00 | 0.00 | 0.00 | 0.00 | 0.00 | 0.00 | 0.00 | 0.00 | 0.00 | 0.00 | 0.00 | 0.00 | 0.00 | 0.00 | 0.00 | 0.00 | 0.00 | |
Na | 0.00 | 0.00 | 0.00 | 0.00 | 0.00 | 0.00 | 0.00 | 0.00 | 0.00 | 0.00 | 0.00 | 0.00 | 0.00 | 0.00 | 0.00 | 0.00 | 0.00 | 0.00 | 0.00 | 0.00 | 0.00 | |
K | 0.00 | 0.00 | 0.00 | 0.00 | 0.00 | 0.00 | 0.00 | 0.00 | 0.00 | 0.00 | 0.00 | 0.00 | 0.00 | 0.00 | 0.00 | 0.00 | 0.00 | 0.00 | 0.00 | 0.00 | 0.00 | |
Ni | 0.00 | 0.01 | 0.00 | 0.01 | 0.00 | 0.01 | 0.00 | 0.00 | 0.01 | 0.00 | 0.01 | 0.00 | 0.01 | 0.00 | 0.01 | 0.00 | 0.01 | 0.00 | 0.01 | 0.00 | 0.01 | |
Total | 3.00 | 2.99 | 3.00 | 2.99 | 3.00 | 2.99 | 3.00 | 3.00 | 2.99 | 3.00 | 2.99 | 3.00 | 2.99 | 3.00 | 2.99 | 3.00 | 2.99 | 3.00 | 2.99 | 3.00 | 2.99 | |
Mg# | 61.9 | 90.7 | 58.3 | 92.0 | 64.3 | 91.1 | 63.2 | 68.5 | 94.8 | 64.9 | 94.0 | 65.4 | 96.2 | 69.6 | 94.8 | 67.6 | 94.7 | 69.3 | 95.0 | 69.2 | 92.1 | |
Cr# | 60.0 | 61.0 | 40.9 | 63.9 | 61.1 | 60.7 | 75.0 | 69.5 | 70.0 | 75.0 | 59.3 | |||||||||||
Fe3+/∑Fe | 0.20 | 0.21 | 0.16 | 0.18 | 0.21 | 0.20 | 0.22 | 0.24 | 0.20 | 0.21 | 0.21 |
Magnesium isotopic analysis of chromite and olivine
Magnesium isotopes were analyzed at the University of Washington, Seattle and the China University of Geosciences, Beijing (CUGB), following previously established procedures (Teng et al., 2010, 2015; Ke et al., 2016). Chromites were dissolved in a mixture of concentrated HF-HNO3 in sealed Teflon vessels jacketed in steel acid digestion bombs at 220°C for 48 h, after which the solutions were transferred to Savillex screwtop beakers. Olivine grains were dissolved in a mixture of HF–HNO3–HCl in sealed 7 mL Teflon beakers and heated on a hot plate in a laminar flow exhaust hood. All the samples were refluxed with concentrated HNO3 until complete dissolution was achieved, and subsequently evaporated to dryness. Then, the powders were dissolved in 1N HNO3 for chromatographic separation. Separation of Mg was achieved by cation exchange chromatography with Bio-Rad 200–400 mesh AG50W-X8 pre-cleaned resin. The chromatographic procedures were repeated twice to separate Mg from matrix elements. Prior to Mg isotopic analyses, the abundances of Ti, Al, Cr, Fe, and Ca relative to Mg in each sample solution were measured. The recovery of Mg is up to 99.5% to 99.9%, and the whole procedural blank is less than 10 ng. Magnesium isotopic ratios were measured using a standard-sample bracketing method. Sample solutions containing 400 ng/g Mg were introduced into the plasma (~ 50 μL/min) through a standard H-skimmer cone, and an ESI PFA MicroFlow nebulizer with a quartz Scott-type spray chamber under low-resolution mode. Sample solution ratio measurements were made for ≥4 repeat runs during a session. The long-term external precision is ±0.06‰ (2SD) for δ26Mg (Teng et al., 2015; Ke et al., 2016). The standard materials San Carlos olivine, Hawaiian seawater, GSB, BHVO-2, and BCR-2 were processed during our sample analyses to assess the accuracy and reproducibility, both of which are listed in Table 2. All the measured Mg isotopic values of these standards show excellent agreement with the recommended values (BHVO-2: δ26Mg = −0.20 ± 0.07‰; seawater: δ26Mg = −0.83 ± 0.09‰; olivine: −0.26 ± 0.07‰; Teng et al., 2015) and previously reported values (GSB: δ26Mg = −2.04 ± 0.04‰; BCR-2: δ26Mg = −0.19 ± 0.05‰; Ke et al., 2016). In addition, replicate analyses were carried out for chromite (L4, L6, and KZ15–37) and olivine (KZ15–16). The results of chromite (−0.04 ± 0.06‰; −0.10 ± 0.06‰; 0.15 ± 0.04‰) agree well within analytical uncertainty (0.01 ± 0.05‰; −0.15 ± 0.05‰; 0.19 ± 0.06‰) and the olivine replicate (KZ15–16, −0.34 ± 0.03‰) is a bit higher (−0.26 ± 0.03‰), which demonstrates the accuracy of our data (Table 2).
Locality | Gaositai | ||||||||||||||||
Sample | GST-18 | GST-33-2 | GST-12 | GST-17 | GST-20 | GST-22 | GST-23 | GST-25 | GST-26 | GST-31 | GST-35-1 | ||||||
Rock type | Dunite | Dunite | Chromitite | Chromitite | Chromitite | Chromitite | Chromitite | Chromitite | Chromitite | Chromitite | Chromitite | ||||||
Mineral | Chr | Ol | Chr | Ol | Chr | Ol | Chr | Ol | Chr | Ol | Chr | Chr | Chr | Chr | Chr | Ol | Chr |
point | 8 | 8 | 8 | 8 | 8 | 8 | 8 | 8 | 8 | 8 | 8 | 8 | 8 | 8 | 8 | 8 | 8 |
SiO2 | 0.04 | 41.6 | 0.08 | 41.5 | 0.03 | 41.2 | 0.05 | 42.2 | 0.01 | 41.4 | 0.02 | 0.01 | 0.05 | 0.02 | 0.02 | 42.1 | 0.02 |
TiO2 | 0.49 | 0.01 | 0.49 | 0.01 | 0.50 | 0.01 | 0.52 | 0.01 | 0.47 | 0.00 | 0.49 | 0.51 | 0.50 | 0.56 | 0.51 | 0.02 | 0.50 |
Al2O3 | 7.75 | 0.00 | 7.40 | 0.00 | 7.19 | 0.00 | 7.46 | 0.00 | 7.65 | 0.00 | 7.26 | 7.62 | 7.32 | 7.14 | 7.39 | 0.00 | 7.36 |
Cr2O3 | 50.6 | 0.02 | 51.7 | 0.04 | 49.9 | 0.02 | 50.2 | 0.06 | 52.5 | 0.02 | 52.1 | 52.1 | 52.7 | 51.9 | 50.9 | 0.02 | 51.7 |
FeO | 29.9 | 7.73 | 30.2 | 7.13 | 31.5 | 7.63 | 31.8 | 6.66 | 25.4 | 7.28 | 26.2 | 26.1 | 26.4 | 27.9 | 29.5 | 7.47 | 28.3 |
MnO | 0.47 | 0.12 | 0.54 | 0.13 | 0.44 | 0.14 | 0.46 | 0.12 | 0.33 | 0.14 | 0.33 | 0.30 | 0.31 | 0.33 | 0.41 | 0.16 | 0.42 |
MgO | 9.37 | 49.11 | 9.44 | 50.94 | 9.44 | 50.18 | 9.01 | 51.35 | 12.4 | 50.47 | 12.4 | 12.7 | 11.5 | 11.4 | 10.6 | 50.74 | 11.3 |
CaO | 0.01 | 0.21 | 0.00 | 0.16 | 0.01 | 0.21 | 0.00 | 0.17 | 0.00 | 0.17 | 0.01 | 0.01 | 0.02 | 0.01 | 0.01 | 0.20 | 0.01 |
Na2O | 0.00 | 0.00 | 0.02 | 0.00 | 0.00 | 0.00 | 0.03 | 0.01 | 0.01 | 0.00 | 0.00 | 0.01 | 0.00 | 0.02 | 0.01 | 0.01 | 0.01 |
K2O | 0.01 | 0.01 | 0.00 | 0.01 | 0.00 | 0.00 | 0.00 | 0.00 | 0.00 | 0.00 | 0.00 | 0.00 | 0.00 | 0.00 | 0.00 | 0.01 | 0.00 |
NiO | 0.11 | 0.27 | 0.08 | 0.26 | 0.12 | 0.25 | 0.15 | 0.25 | 0.13 | 0.25 | 0.14 | 0.14 | 0.10 | 0.12 | 0.14 | 0.28 | 0.12 |
Total | 98.7 | 99.12 | 99.9 | 100 | 99.1 | 100 | 99.6 | 101 | 98.9 | 99.7 | 98.9 | 99.4 | 98.9 | 99.4 | 99.5 | 101 | 99.7 |
Si | 0.00 | 1.02 | 0.00 | 1.00 | 0.00 | 1.01 | 0.00 | 1.01 | 0.00 | 1.01 | 0.00 | 0.00 | 0.00 | 0.00 | 0.00 | 1.01 | 0.00 |
Ti | 0.01 | 0.00 | 0.01 | 0.00 | 0.01 | 0.00 | 0.01 | 0.00 | 0.01 | 0.00 | 0.01 | 0.01 | 0.01 | 0.01 | 0.01 | 0.00 | 0.01 |
Al | 0.31 | 0.00 | 0.29 | 0.00 | 0.29 | 0.00 | 0.29 | 0.00 | 0.30 | 0.00 | 0.28 | 0.29 | 0.29 | 0.28 | 0.29 | 0.00 | 0.29 |
Cr | 1.35 | 0.00 | 1.36 | 0.00 | 1.33 | 0.00 | 1.33 | 0.00 | 1.37 | 0.00 | 1.36 | 1.35 | 1.38 | 1.36 | 1.34 | 0.00 | 1.35 |
Fe3+ | 0.32 | 0.32 | 0.36 | 0.35 | 0.31 | 0.33 | 0.33 | 0.30 | 0.33 | 0.35 | 0.34 | ||||||
Fe2+ | 0.53 | 0.16 | 0.52 | 0.14 | 0.52 | 0.15 | 0.54 | 0.13 | 0.39 | 0.15 | 0.39 | 0.38 | 0.43 | 0.44 | 0.47 | 0.15 | 0.44 |
Mn | 0.01 | 0.00 | 0.02 | 0.00 | 0.01 | 0.00 | 0.01 | 0.00 | 0.01 | 0.00 | 0.01 | 0.01 | 0.01 | 0.01 | 0.01 | 0.00 | 0.01 |
Mg | 0.47 | 1.79 | 0.47 | 1.84 | 0.47 | 1.81 | 0.45 | 1.83 | 0.61 | 1.83 | 0.61 | 0.62 | 0.57 | 0.56 | 0.52 | 1.81 | 0.56 |
Ca | 0.00 | 0.01 | 0.00 | 0.00 | 0.00 | 0.01 | 0.00 | 0.00 | 0.00 | 0.00 | 0.00 | 0.00 | 0.00 | 0.00 | 0.00 | 0.01 | 0.00 |
Na | 0.00 | 0.00 | 0.00 | 0.00 | 0.00 | 0.00 | 0.00 | 0.00 | 0.00 | 0.00 | 0.00 | 0.00 | 0.00 | 0.00 | 0.00 | 0.00 | 0.00 |
K | 0.00 | 0.00 | 0.00 | 0.00 | 0.00 | 0.00 | 0.00 | 0.00 | 0.00 | 0.00 | 0.00 | 0.00 | 0.00 | 0.00 | 0.00 | 0.00 | 0.00 |
Ni | 0.00 | 0.01 | 0.00 | 0.01 | 0.00 | 0.00 | 0.00 | 0.00 | 0.00 | 0.00 | 0.00 | 0.00 | 0.00 | 0.00 | 0.00 | 0.01 | 0.00 |
Total | 3.00 | 2.98 | 3.00 | 3.00 | 3.00 | 2.99 | 3.00 | 2.99 | 3.00 | 2.99 | 3.00 | 3.00 | 3.00 | 3.00 | 3.00 | 2.99 | 3.00 |
Mg# | 47.5 | 91.9 | 47.2 | 92.7 | 47.4 | 92.1 | 45.3 | 93.2 | 61.2 | 92.5 | 61.9 | 61.2 | 56.8 | 56.1 | 52.6 | 92.4 | 55.9 |
Cr# | 81.4 | 82.0 | 82.3 | 81.9 | 82.1 | 82.8 | 82.1 | 82.9 | 83.0 | 82.2 | 82.5 | ||||||
Fe3+/∑Fe | 0.38 | 0.38 | 0.41 | 0.39 | 0.45 | 0.46 | 0.47 | 0.41 | 0.43 | 0.42 | 0.44 |
Locality | Gaositai | ||||||||||||||||
Sample | GST-18 | GST-33-2 | GST-12 | GST-17 | GST-20 | GST-22 | GST-23 | GST-25 | GST-26 | GST-31 | GST-35-1 | ||||||
Rock type | Dunite | Dunite | Chromitite | Chromitite | Chromitite | Chromitite | Chromitite | Chromitite | Chromitite | Chromitite | Chromitite | ||||||
Mineral | Chr | Ol | Chr | Ol | Chr | Ol | Chr | Ol | Chr | Ol | Chr | Chr | Chr | Chr | Chr | Ol | Chr |
point | 8 | 8 | 8 | 8 | 8 | 8 | 8 | 8 | 8 | 8 | 8 | 8 | 8 | 8 | 8 | 8 | 8 |
SiO2 | 0.04 | 41.6 | 0.08 | 41.5 | 0.03 | 41.2 | 0.05 | 42.2 | 0.01 | 41.4 | 0.02 | 0.01 | 0.05 | 0.02 | 0.02 | 42.1 | 0.02 |
TiO2 | 0.49 | 0.01 | 0.49 | 0.01 | 0.50 | 0.01 | 0.52 | 0.01 | 0.47 | 0.00 | 0.49 | 0.51 | 0.50 | 0.56 | 0.51 | 0.02 | 0.50 |
Al2O3 | 7.75 | 0.00 | 7.40 | 0.00 | 7.19 | 0.00 | 7.46 | 0.00 | 7.65 | 0.00 | 7.26 | 7.62 | 7.32 | 7.14 | 7.39 | 0.00 | 7.36 |
Cr2O3 | 50.6 | 0.02 | 51.7 | 0.04 | 49.9 | 0.02 | 50.2 | 0.06 | 52.5 | 0.02 | 52.1 | 52.1 | 52.7 | 51.9 | 50.9 | 0.02 | 51.7 |
FeO | 29.9 | 7.73 | 30.2 | 7.13 | 31.5 | 7.63 | 31.8 | 6.66 | 25.4 | 7.28 | 26.2 | 26.1 | 26.4 | 27.9 | 29.5 | 7.47 | 28.3 |
MnO | 0.47 | 0.12 | 0.54 | 0.13 | 0.44 | 0.14 | 0.46 | 0.12 | 0.33 | 0.14 | 0.33 | 0.30 | 0.31 | 0.33 | 0.41 | 0.16 | 0.42 |
MgO | 9.37 | 49.11 | 9.44 | 50.94 | 9.44 | 50.18 | 9.01 | 51.35 | 12.4 | 50.47 | 12.4 | 12.7 | 11.5 | 11.4 | 10.6 | 50.74 | 11.3 |
CaO | 0.01 | 0.21 | 0.00 | 0.16 | 0.01 | 0.21 | 0.00 | 0.17 | 0.00 | 0.17 | 0.01 | 0.01 | 0.02 | 0.01 | 0.01 | 0.20 | 0.01 |
Na2O | 0.00 | 0.00 | 0.02 | 0.00 | 0.00 | 0.00 | 0.03 | 0.01 | 0.01 | 0.00 | 0.00 | 0.01 | 0.00 | 0.02 | 0.01 | 0.01 | 0.01 |
K2O | 0.01 | 0.01 | 0.00 | 0.01 | 0.00 | 0.00 | 0.00 | 0.00 | 0.00 | 0.00 | 0.00 | 0.00 | 0.00 | 0.00 | 0.00 | 0.01 | 0.00 |
NiO | 0.11 | 0.27 | 0.08 | 0.26 | 0.12 | 0.25 | 0.15 | 0.25 | 0.13 | 0.25 | 0.14 | 0.14 | 0.10 | 0.12 | 0.14 | 0.28 | 0.12 |
Total | 98.7 | 99.12 | 99.9 | 100 | 99.1 | 100 | 99.6 | 101 | 98.9 | 99.7 | 98.9 | 99.4 | 98.9 | 99.4 | 99.5 | 101 | 99.7 |
Si | 0.00 | 1.02 | 0.00 | 1.00 | 0.00 | 1.01 | 0.00 | 1.01 | 0.00 | 1.01 | 0.00 | 0.00 | 0.00 | 0.00 | 0.00 | 1.01 | 0.00 |
Ti | 0.01 | 0.00 | 0.01 | 0.00 | 0.01 | 0.00 | 0.01 | 0.00 | 0.01 | 0.00 | 0.01 | 0.01 | 0.01 | 0.01 | 0.01 | 0.00 | 0.01 |
Al | 0.31 | 0.00 | 0.29 | 0.00 | 0.29 | 0.00 | 0.29 | 0.00 | 0.30 | 0.00 | 0.28 | 0.29 | 0.29 | 0.28 | 0.29 | 0.00 | 0.29 |
Cr | 1.35 | 0.00 | 1.36 | 0.00 | 1.33 | 0.00 | 1.33 | 0.00 | 1.37 | 0.00 | 1.36 | 1.35 | 1.38 | 1.36 | 1.34 | 0.00 | 1.35 |
Fe3+ | 0.32 | 0.32 | 0.36 | 0.35 | 0.31 | 0.33 | 0.33 | 0.30 | 0.33 | 0.35 | 0.34 | ||||||
Fe2+ | 0.53 | 0.16 | 0.52 | 0.14 | 0.52 | 0.15 | 0.54 | 0.13 | 0.39 | 0.15 | 0.39 | 0.38 | 0.43 | 0.44 | 0.47 | 0.15 | 0.44 |
Mn | 0.01 | 0.00 | 0.02 | 0.00 | 0.01 | 0.00 | 0.01 | 0.00 | 0.01 | 0.00 | 0.01 | 0.01 | 0.01 | 0.01 | 0.01 | 0.00 | 0.01 |
Mg | 0.47 | 1.79 | 0.47 | 1.84 | 0.47 | 1.81 | 0.45 | 1.83 | 0.61 | 1.83 | 0.61 | 0.62 | 0.57 | 0.56 | 0.52 | 1.81 | 0.56 |
Ca | 0.00 | 0.01 | 0.00 | 0.00 | 0.00 | 0.01 | 0.00 | 0.00 | 0.00 | 0.00 | 0.00 | 0.00 | 0.00 | 0.00 | 0.00 | 0.01 | 0.00 |
Na | 0.00 | 0.00 | 0.00 | 0.00 | 0.00 | 0.00 | 0.00 | 0.00 | 0.00 | 0.00 | 0.00 | 0.00 | 0.00 | 0.00 | 0.00 | 0.00 | 0.00 |
K | 0.00 | 0.00 | 0.00 | 0.00 | 0.00 | 0.00 | 0.00 | 0.00 | 0.00 | 0.00 | 0.00 | 0.00 | 0.00 | 0.00 | 0.00 | 0.00 | 0.00 |
Ni | 0.00 | 0.01 | 0.00 | 0.01 | 0.00 | 0.00 | 0.00 | 0.00 | 0.00 | 0.00 | 0.00 | 0.00 | 0.00 | 0.00 | 0.00 | 0.01 | 0.00 |
Total | 3.00 | 2.98 | 3.00 | 3.00 | 3.00 | 2.99 | 3.00 | 2.99 | 3.00 | 2.99 | 3.00 | 3.00 | 3.00 | 3.00 | 3.00 | 2.99 | 3.00 |
Mg# | 47.5 | 91.9 | 47.2 | 92.7 | 47.4 | 92.1 | 45.3 | 93.2 | 61.2 | 92.5 | 61.9 | 61.2 | 56.8 | 56.1 | 52.6 | 92.4 | 55.9 |
Cr# | 81.4 | 82.0 | 82.3 | 81.9 | 82.1 | 82.8 | 82.1 | 82.9 | 83.0 | 82.2 | 82.5 | ||||||
Fe3+/∑Fe | 0.38 | 0.38 | 0.41 | 0.39 | 0.45 | 0.46 | 0.47 | 0.41 | 0.43 | 0.42 | 0.44 |
Note: aChr, chromite; Ol, olivine.
Mg# = 100 * molar Mg2+/(Mg2+ + Fe2+); Cr# = 100 * molar Cr3+/(Cr3+ + Al3+).
Fe3+/∑Fe ratio of chromite are calculated based on microprobe analyses assuming stoichiometry (Droop, 1985).
Locality | Gaositai | ||||||||||||||||
Sample | GST-18 | GST-33-2 | GST-12 | GST-17 | GST-20 | GST-22 | GST-23 | GST-25 | GST-26 | GST-31 | GST-35-1 | ||||||
Rock type | Dunite | Dunite | Chromitite | Chromitite | Chromitite | Chromitite | Chromitite | Chromitite | Chromitite | Chromitite | Chromitite | ||||||
Mineral | Chr | Ol | Chr | Ol | Chr | Ol | Chr | Ol | Chr | Ol | Chr | Chr | Chr | Chr | Chr | Ol | Chr |
point | 8 | 8 | 8 | 8 | 8 | 8 | 8 | 8 | 8 | 8 | 8 | 8 | 8 | 8 | 8 | 8 | 8 |
SiO2 | 0.04 | 41.6 | 0.08 | 41.5 | 0.03 | 41.2 | 0.05 | 42.2 | 0.01 | 41.4 | 0.02 | 0.01 | 0.05 | 0.02 | 0.02 | 42.1 | 0.02 |
TiO2 | 0.49 | 0.01 | 0.49 | 0.01 | 0.50 | 0.01 | 0.52 | 0.01 | 0.47 | 0.00 | 0.49 | 0.51 | 0.50 | 0.56 | 0.51 | 0.02 | 0.50 |
Al2O3 | 7.75 | 0.00 | 7.40 | 0.00 | 7.19 | 0.00 | 7.46 | 0.00 | 7.65 | 0.00 | 7.26 | 7.62 | 7.32 | 7.14 | 7.39 | 0.00 | 7.36 |
Cr2O3 | 50.6 | 0.02 | 51.7 | 0.04 | 49.9 | 0.02 | 50.2 | 0.06 | 52.5 | 0.02 | 52.1 | 52.1 | 52.7 | 51.9 | 50.9 | 0.02 | 51.7 |
FeO | 29.9 | 7.73 | 30.2 | 7.13 | 31.5 | 7.63 | 31.8 | 6.66 | 25.4 | 7.28 | 26.2 | 26.1 | 26.4 | 27.9 | 29.5 | 7.47 | 28.3 |
MnO | 0.47 | 0.12 | 0.54 | 0.13 | 0.44 | 0.14 | 0.46 | 0.12 | 0.33 | 0.14 | 0.33 | 0.30 | 0.31 | 0.33 | 0.41 | 0.16 | 0.42 |
MgO | 9.37 | 49.11 | 9.44 | 50.94 | 9.44 | 50.18 | 9.01 | 51.35 | 12.4 | 50.47 | 12.4 | 12.7 | 11.5 | 11.4 | 10.6 | 50.74 | 11.3 |
CaO | 0.01 | 0.21 | 0.00 | 0.16 | 0.01 | 0.21 | 0.00 | 0.17 | 0.00 | 0.17 | 0.01 | 0.01 | 0.02 | 0.01 | 0.01 | 0.20 | 0.01 |
Na2O | 0.00 | 0.00 | 0.02 | 0.00 | 0.00 | 0.00 | 0.03 | 0.01 | 0.01 | 0.00 | 0.00 | 0.01 | 0.00 | 0.02 | 0.01 | 0.01 | 0.01 |
K2O | 0.01 | 0.01 | 0.00 | 0.01 | 0.00 | 0.00 | 0.00 | 0.00 | 0.00 | 0.00 | 0.00 | 0.00 | 0.00 | 0.00 | 0.00 | 0.01 | 0.00 |
NiO | 0.11 | 0.27 | 0.08 | 0.26 | 0.12 | 0.25 | 0.15 | 0.25 | 0.13 | 0.25 | 0.14 | 0.14 | 0.10 | 0.12 | 0.14 | 0.28 | 0.12 |
Total | 98.7 | 99.12 | 99.9 | 100 | 99.1 | 100 | 99.6 | 101 | 98.9 | 99.7 | 98.9 | 99.4 | 98.9 | 99.4 | 99.5 | 101 | 99.7 |
Si | 0.00 | 1.02 | 0.00 | 1.00 | 0.00 | 1.01 | 0.00 | 1.01 | 0.00 | 1.01 | 0.00 | 0.00 | 0.00 | 0.00 | 0.00 | 1.01 | 0.00 |
Ti | 0.01 | 0.00 | 0.01 | 0.00 | 0.01 | 0.00 | 0.01 | 0.00 | 0.01 | 0.00 | 0.01 | 0.01 | 0.01 | 0.01 | 0.01 | 0.00 | 0.01 |
Al | 0.31 | 0.00 | 0.29 | 0.00 | 0.29 | 0.00 | 0.29 | 0.00 | 0.30 | 0.00 | 0.28 | 0.29 | 0.29 | 0.28 | 0.29 | 0.00 | 0.29 |
Cr | 1.35 | 0.00 | 1.36 | 0.00 | 1.33 | 0.00 | 1.33 | 0.00 | 1.37 | 0.00 | 1.36 | 1.35 | 1.38 | 1.36 | 1.34 | 0.00 | 1.35 |
Fe3+ | 0.32 | 0.32 | 0.36 | 0.35 | 0.31 | 0.33 | 0.33 | 0.30 | 0.33 | 0.35 | 0.34 | ||||||
Fe2+ | 0.53 | 0.16 | 0.52 | 0.14 | 0.52 | 0.15 | 0.54 | 0.13 | 0.39 | 0.15 | 0.39 | 0.38 | 0.43 | 0.44 | 0.47 | 0.15 | 0.44 |
Mn | 0.01 | 0.00 | 0.02 | 0.00 | 0.01 | 0.00 | 0.01 | 0.00 | 0.01 | 0.00 | 0.01 | 0.01 | 0.01 | 0.01 | 0.01 | 0.00 | 0.01 |
Mg | 0.47 | 1.79 | 0.47 | 1.84 | 0.47 | 1.81 | 0.45 | 1.83 | 0.61 | 1.83 | 0.61 | 0.62 | 0.57 | 0.56 | 0.52 | 1.81 | 0.56 |
Ca | 0.00 | 0.01 | 0.00 | 0.00 | 0.00 | 0.01 | 0.00 | 0.00 | 0.00 | 0.00 | 0.00 | 0.00 | 0.00 | 0.00 | 0.00 | 0.01 | 0.00 |
Na | 0.00 | 0.00 | 0.00 | 0.00 | 0.00 | 0.00 | 0.00 | 0.00 | 0.00 | 0.00 | 0.00 | 0.00 | 0.00 | 0.00 | 0.00 | 0.00 | 0.00 |
K | 0.00 | 0.00 | 0.00 | 0.00 | 0.00 | 0.00 | 0.00 | 0.00 | 0.00 | 0.00 | 0.00 | 0.00 | 0.00 | 0.00 | 0.00 | 0.00 | 0.00 |
Ni | 0.00 | 0.01 | 0.00 | 0.01 | 0.00 | 0.00 | 0.00 | 0.00 | 0.00 | 0.00 | 0.00 | 0.00 | 0.00 | 0.00 | 0.00 | 0.01 | 0.00 |
Total | 3.00 | 2.98 | 3.00 | 3.00 | 3.00 | 2.99 | 3.00 | 2.99 | 3.00 | 2.99 | 3.00 | 3.00 | 3.00 | 3.00 | 3.00 | 2.99 | 3.00 |
Mg# | 47.5 | 91.9 | 47.2 | 92.7 | 47.4 | 92.1 | 45.3 | 93.2 | 61.2 | 92.5 | 61.9 | 61.2 | 56.8 | 56.1 | 52.6 | 92.4 | 55.9 |
Cr# | 81.4 | 82.0 | 82.3 | 81.9 | 82.1 | 82.8 | 82.1 | 82.9 | 83.0 | 82.2 | 82.5 | ||||||
Fe3+/∑Fe | 0.38 | 0.38 | 0.41 | 0.39 | 0.45 | 0.46 | 0.47 | 0.41 | 0.43 | 0.42 | 0.44 |
Locality | Gaositai | ||||||||||||||||
Sample | GST-18 | GST-33-2 | GST-12 | GST-17 | GST-20 | GST-22 | GST-23 | GST-25 | GST-26 | GST-31 | GST-35-1 | ||||||
Rock type | Dunite | Dunite | Chromitite | Chromitite | Chromitite | Chromitite | Chromitite | Chromitite | Chromitite | Chromitite | Chromitite | ||||||
Mineral | Chr | Ol | Chr | Ol | Chr | Ol | Chr | Ol | Chr | Ol | Chr | Chr | Chr | Chr | Chr | Ol | Chr |
point | 8 | 8 | 8 | 8 | 8 | 8 | 8 | 8 | 8 | 8 | 8 | 8 | 8 | 8 | 8 | 8 | 8 |
SiO2 | 0.04 | 41.6 | 0.08 | 41.5 | 0.03 | 41.2 | 0.05 | 42.2 | 0.01 | 41.4 | 0.02 | 0.01 | 0.05 | 0.02 | 0.02 | 42.1 | 0.02 |
TiO2 | 0.49 | 0.01 | 0.49 | 0.01 | 0.50 | 0.01 | 0.52 | 0.01 | 0.47 | 0.00 | 0.49 | 0.51 | 0.50 | 0.56 | 0.51 | 0.02 | 0.50 |
Al2O3 | 7.75 | 0.00 | 7.40 | 0.00 | 7.19 | 0.00 | 7.46 | 0.00 | 7.65 | 0.00 | 7.26 | 7.62 | 7.32 | 7.14 | 7.39 | 0.00 | 7.36 |
Cr2O3 | 50.6 | 0.02 | 51.7 | 0.04 | 49.9 | 0.02 | 50.2 | 0.06 | 52.5 | 0.02 | 52.1 | 52.1 | 52.7 | 51.9 | 50.9 | 0.02 | 51.7 |
FeO | 29.9 | 7.73 | 30.2 | 7.13 | 31.5 | 7.63 | 31.8 | 6.66 | 25.4 | 7.28 | 26.2 | 26.1 | 26.4 | 27.9 | 29.5 | 7.47 | 28.3 |
MnO | 0.47 | 0.12 | 0.54 | 0.13 | 0.44 | 0.14 | 0.46 | 0.12 | 0.33 | 0.14 | 0.33 | 0.30 | 0.31 | 0.33 | 0.41 | 0.16 | 0.42 |
MgO | 9.37 | 49.11 | 9.44 | 50.94 | 9.44 | 50.18 | 9.01 | 51.35 | 12.4 | 50.47 | 12.4 | 12.7 | 11.5 | 11.4 | 10.6 | 50.74 | 11.3 |
CaO | 0.01 | 0.21 | 0.00 | 0.16 | 0.01 | 0.21 | 0.00 | 0.17 | 0.00 | 0.17 | 0.01 | 0.01 | 0.02 | 0.01 | 0.01 | 0.20 | 0.01 |
Na2O | 0.00 | 0.00 | 0.02 | 0.00 | 0.00 | 0.00 | 0.03 | 0.01 | 0.01 | 0.00 | 0.00 | 0.01 | 0.00 | 0.02 | 0.01 | 0.01 | 0.01 |
K2O | 0.01 | 0.01 | 0.00 | 0.01 | 0.00 | 0.00 | 0.00 | 0.00 | 0.00 | 0.00 | 0.00 | 0.00 | 0.00 | 0.00 | 0.00 | 0.01 | 0.00 |
NiO | 0.11 | 0.27 | 0.08 | 0.26 | 0.12 | 0.25 | 0.15 | 0.25 | 0.13 | 0.25 | 0.14 | 0.14 | 0.10 | 0.12 | 0.14 | 0.28 | 0.12 |
Total | 98.7 | 99.12 | 99.9 | 100 | 99.1 | 100 | 99.6 | 101 | 98.9 | 99.7 | 98.9 | 99.4 | 98.9 | 99.4 | 99.5 | 101 | 99.7 |
Si | 0.00 | 1.02 | 0.00 | 1.00 | 0.00 | 1.01 | 0.00 | 1.01 | 0.00 | 1.01 | 0.00 | 0.00 | 0.00 | 0.00 | 0.00 | 1.01 | 0.00 |
Ti | 0.01 | 0.00 | 0.01 | 0.00 | 0.01 | 0.00 | 0.01 | 0.00 | 0.01 | 0.00 | 0.01 | 0.01 | 0.01 | 0.01 | 0.01 | 0.00 | 0.01 |
Al | 0.31 | 0.00 | 0.29 | 0.00 | 0.29 | 0.00 | 0.29 | 0.00 | 0.30 | 0.00 | 0.28 | 0.29 | 0.29 | 0.28 | 0.29 | 0.00 | 0.29 |
Cr | 1.35 | 0.00 | 1.36 | 0.00 | 1.33 | 0.00 | 1.33 | 0.00 | 1.37 | 0.00 | 1.36 | 1.35 | 1.38 | 1.36 | 1.34 | 0.00 | 1.35 |
Fe3+ | 0.32 | 0.32 | 0.36 | 0.35 | 0.31 | 0.33 | 0.33 | 0.30 | 0.33 | 0.35 | 0.34 | ||||||
Fe2+ | 0.53 | 0.16 | 0.52 | 0.14 | 0.52 | 0.15 | 0.54 | 0.13 | 0.39 | 0.15 | 0.39 | 0.38 | 0.43 | 0.44 | 0.47 | 0.15 | 0.44 |
Mn | 0.01 | 0.00 | 0.02 | 0.00 | 0.01 | 0.00 | 0.01 | 0.00 | 0.01 | 0.00 | 0.01 | 0.01 | 0.01 | 0.01 | 0.01 | 0.00 | 0.01 |
Mg | 0.47 | 1.79 | 0.47 | 1.84 | 0.47 | 1.81 | 0.45 | 1.83 | 0.61 | 1.83 | 0.61 | 0.62 | 0.57 | 0.56 | 0.52 | 1.81 | 0.56 |
Ca | 0.00 | 0.01 | 0.00 | 0.00 | 0.00 | 0.01 | 0.00 | 0.00 | 0.00 | 0.00 | 0.00 | 0.00 | 0.00 | 0.00 | 0.00 | 0.01 | 0.00 |
Na | 0.00 | 0.00 | 0.00 | 0.00 | 0.00 | 0.00 | 0.00 | 0.00 | 0.00 | 0.00 | 0.00 | 0.00 | 0.00 | 0.00 | 0.00 | 0.00 | 0.00 |
K | 0.00 | 0.00 | 0.00 | 0.00 | 0.00 | 0.00 | 0.00 | 0.00 | 0.00 | 0.00 | 0.00 | 0.00 | 0.00 | 0.00 | 0.00 | 0.00 | 0.00 |
Ni | 0.00 | 0.01 | 0.00 | 0.01 | 0.00 | 0.00 | 0.00 | 0.00 | 0.00 | 0.00 | 0.00 | 0.00 | 0.00 | 0.00 | 0.00 | 0.01 | 0.00 |
Total | 3.00 | 2.98 | 3.00 | 3.00 | 3.00 | 2.99 | 3.00 | 2.99 | 3.00 | 2.99 | 3.00 | 3.00 | 3.00 | 3.00 | 3.00 | 2.99 | 3.00 |
Mg# | 47.5 | 91.9 | 47.2 | 92.7 | 47.4 | 92.1 | 45.3 | 93.2 | 61.2 | 92.5 | 61.9 | 61.2 | 56.8 | 56.1 | 52.6 | 92.4 | 55.9 |
Cr# | 81.4 | 82.0 | 82.3 | 81.9 | 82.1 | 82.8 | 82.1 | 82.9 | 83.0 | 82.2 | 82.5 | ||||||
Fe3+/∑Fe | 0.38 | 0.38 | 0.41 | 0.39 | 0.45 | 0.46 | 0.47 | 0.41 | 0.43 | 0.42 | 0.44 |
Note: aChr, chromite; Ol, olivine.
Mg# = 100 * molar Mg2+/(Mg2+ + Fe2+); Cr# = 100 * molar Cr3+/(Cr3+ + Al3+).
Fe3+/∑Fe ratio of chromite are calculated based on microprobe analyses assuming stoichiometry (Droop, 1985).
Magnesium isotopic (‰) compositions of chromite and olivine in the dunites and chromitites from Luobusa and Kızıldağ ophiolites, and Gaositai Alaskan-type complex
Locality | Sample | Rock type | Mineral | δ26Mg | 2SD | δ25Mg | 2SD | Mineral | δ26Mg | 2SD | δ25Mg | 2SD | Δ26MgChr-Ola | T(°C)b |
Luobusa | LBS09005–8 | Chromitite | Chr | 0.17 | 0.04 | 0.09 | 0.05 | Ol | −0.20 | 0.07 | −0.11 | 0.05 | 0.37 | 722 |
LBS13–21 | Chromitite | Chr | 0.30 | 0.04 | 0.17 | 0.05 | ||||||||
LBS13–22 | Chromitite | Chr | 0.29 | 0.04 | 0.17 | 0.05 | ||||||||
LBS13–23 | Chromitite | Chr | 0.20 | 0.04 | 0.09 | 0.05 | ||||||||
LBS13–34 | Chromitite | Chr | −0.19 | 0.04 | −0.11 | 0.05 | ||||||||
LBS13–39 | Chromitite | Chr | −0.11 | 0.04 | −0.06 | 0.05 | ||||||||
L1 | Chromitite | Chr | 0.08 | 0.05 | 0.04 | 0.04 | ||||||||
L2 | Chromitite | Chr | 0.12 | 0.05 | 0.07 | 0.04 | ||||||||
L4 | Chromitite | Chr | 0.01 | 0.05 | 0.00 | 0.04 | ||||||||
Chrc | −0.04 | 0.06 | −0.01 | 0.05 | ||||||||||
L3 | Chromitite | Chr | 0.09 | 0.05 | 0.05 | 0.04 | ||||||||
L5 | Chromitite | Chr | 0.06 | 0.05 | 0.02 | 0.04 | ||||||||
L6 | Chromitite | Chr | −0.15 | 0.05 | −0.09 | 0.04 | ||||||||
Chrc | −0.10 | 0.06 | −0.05 | 0.05 | ||||||||||
L7 | Chromitite | Chr | −0.05 | 0.05 | −0.04 | 0.04 | ||||||||
L06a | Chromitite | Chr | −0.06 | 0.04 | −0.02 | 0.05 | ||||||||
Kızıldağ | KZ14–15 | Dunite | Chr | 0.23 | 0.03 | 0.12 | 0.03 | Ol | −0.32 | 0.06 | −0.16 | 0.05 | 0.55 | 699 |
KZ14–33 | Dunite | Chr | 0.19 | 0.03 | 0.10 | 0.03 | Ol | −0.30 | 0.06 | −0.15 | 0.05 | 0.50 | 754 | |
KZ14–31 | Dunite | Chr | 0.17 | 0.04 | 0.09 | 0.03 | ||||||||
KZ14–40 | Dunite | Chr | 0.05 | 0.01 | 0.04 | 0.02 | Ol | −0.30 | 0.04 | −0.16 | 0.02 | 0.35 | 807 | |
KZ15–09 | Dunite | Chr | 0.34 | 0.06 | 0.19 | 0.04 | Ol | −0.32 | 0.06 | −0.17 | 0.05 | 0.66 | 732 | |
KZ15–16 | Dunite | Chr | 0.07 | 0.03 | 0.02 | 0.05 | Ol | −0.26 | 0.03 | −0.12 | 0.06 | 0.33 | 777 | |
Olc | −0.34 | 0.03 | −0.17 | 0.04 | 0.41 | |||||||||
KZ14–27-1 | Chromitite | Chr | 0.21 | 0.06 | 0.11 | 0.04 | ||||||||
KZ14–27-3 | Chromitite | Chr | 0.03 | 0.03 | 0.02 | 0.02 | Ol | −0.43 | 0.02 | −0.22 | 0.02 | 0.47 | 769 | |
KZ14–28-1 | Chromitite | Chr | 0.04 | 0.07 | 0.03 | 0.03 | Ol | −0.33 | 0.04 | −0.16 | 0.02 | 0.37 | 875 | |
KZ14–29 | Chromitite | Chr | 0.18 | 0.04 | 0.10 | 0.04 | Ol | −0.36 | 0.06 | −0.19 | 0.04 | 0.54 | 787 | |
KZ14–32-2 | Chromitite | Chr | 0.04 | 0.06 | 0.02 | 0.04 | Ol | −0.42 | 0.05 | −0.20 | 0.04 | 0.46 | 844 | |
KZ14–36 | Chromitite | Chr | 0.19 | 0.07 | 0.10 | 0.04 | ||||||||
KZ14–38 | Chromitite | Chr | 0.21 | 0.03 | 0.11 | 0.04 | Ol | −0.32 | 0.04 | −0.16 | 0.02 | 0.53 | 762 | |
KZ15–10 | Chromitite | Chr | 0.78 | 0.04 | 0.41 | 0.03 | Ol | −0.40 | 0.03 | −0.21 | 0.03 | 1.18 | 731 | |
KZ15–20 | Chromitite | Chr | 0.14 | 0.04 | 0.07 | 0.03 | ||||||||
KZ15–21 | Chromitite | Chr | −0.03 | 0.06 | −0.01 | 0.04 | Ol | −0.10 | 0.05 | −0.04 | 0.04 | 0.07 | 756 | |
KZ15–23 | Chromitite | Chr | 0.09 | 0.05 | 0.06 | 0.04 | Ol | −0.48 | 0.03 | −0.26 | 0.04 | 0.57 | 751 | |
KZ15–30 | Chromitite | Chr | −0.10 | 0.02 | −0.05 | 0.02 | Ol | −0.27 | 0.04 | −0.16 | 0.04 | 0.17 | 695 | |
KZ15–34 | Ol | −0.39 | 0.06 | −0.19 | 0.06 | |||||||||
KZ15–36 | Chromitite | Chr | −0.09 | 0.04 | −0.03 | 0.02 | Ol | −0.43 | 0.03 | −0.22 | 0.03 | 0.34 | 813 | |
KZ15–37 | Chromitite | Chr | 0.15 | 0.04 | 0.09 | 0.04 | Ol | −0.41 | 0.06 | −0.21 | 0.06 | 0.57 | 851 | |
Chrc | 0.19 | 0.06 | 0.10 | 0.04 | ||||||||||
KZ15–38 | Chromitite | Chr | 0.04 | 0.06 | 0.03 | 0.03 |
Locality | Sample | Rock type | Mineral | δ26Mg | 2SD | δ25Mg | 2SD | Mineral | δ26Mg | 2SD | δ25Mg | 2SD | Δ26MgChr-Ola | T(°C)b |
Luobusa | LBS09005–8 | Chromitite | Chr | 0.17 | 0.04 | 0.09 | 0.05 | Ol | −0.20 | 0.07 | −0.11 | 0.05 | 0.37 | 722 |
LBS13–21 | Chromitite | Chr | 0.30 | 0.04 | 0.17 | 0.05 | ||||||||
LBS13–22 | Chromitite | Chr | 0.29 | 0.04 | 0.17 | 0.05 | ||||||||
LBS13–23 | Chromitite | Chr | 0.20 | 0.04 | 0.09 | 0.05 | ||||||||
LBS13–34 | Chromitite | Chr | −0.19 | 0.04 | −0.11 | 0.05 | ||||||||
LBS13–39 | Chromitite | Chr | −0.11 | 0.04 | −0.06 | 0.05 | ||||||||
L1 | Chromitite | Chr | 0.08 | 0.05 | 0.04 | 0.04 | ||||||||
L2 | Chromitite | Chr | 0.12 | 0.05 | 0.07 | 0.04 | ||||||||
L4 | Chromitite | Chr | 0.01 | 0.05 | 0.00 | 0.04 | ||||||||
Chrc | −0.04 | 0.06 | −0.01 | 0.05 | ||||||||||
L3 | Chromitite | Chr | 0.09 | 0.05 | 0.05 | 0.04 | ||||||||
L5 | Chromitite | Chr | 0.06 | 0.05 | 0.02 | 0.04 | ||||||||
L6 | Chromitite | Chr | −0.15 | 0.05 | −0.09 | 0.04 | ||||||||
Chrc | −0.10 | 0.06 | −0.05 | 0.05 | ||||||||||
L7 | Chromitite | Chr | −0.05 | 0.05 | −0.04 | 0.04 | ||||||||
L06a | Chromitite | Chr | −0.06 | 0.04 | −0.02 | 0.05 | ||||||||
Kızıldağ | KZ14–15 | Dunite | Chr | 0.23 | 0.03 | 0.12 | 0.03 | Ol | −0.32 | 0.06 | −0.16 | 0.05 | 0.55 | 699 |
KZ14–33 | Dunite | Chr | 0.19 | 0.03 | 0.10 | 0.03 | Ol | −0.30 | 0.06 | −0.15 | 0.05 | 0.50 | 754 | |
KZ14–31 | Dunite | Chr | 0.17 | 0.04 | 0.09 | 0.03 | ||||||||
KZ14–40 | Dunite | Chr | 0.05 | 0.01 | 0.04 | 0.02 | Ol | −0.30 | 0.04 | −0.16 | 0.02 | 0.35 | 807 | |
KZ15–09 | Dunite | Chr | 0.34 | 0.06 | 0.19 | 0.04 | Ol | −0.32 | 0.06 | −0.17 | 0.05 | 0.66 | 732 | |
KZ15–16 | Dunite | Chr | 0.07 | 0.03 | 0.02 | 0.05 | Ol | −0.26 | 0.03 | −0.12 | 0.06 | 0.33 | 777 | |
Olc | −0.34 | 0.03 | −0.17 | 0.04 | 0.41 | |||||||||
KZ14–27-1 | Chromitite | Chr | 0.21 | 0.06 | 0.11 | 0.04 | ||||||||
KZ14–27-3 | Chromitite | Chr | 0.03 | 0.03 | 0.02 | 0.02 | Ol | −0.43 | 0.02 | −0.22 | 0.02 | 0.47 | 769 | |
KZ14–28-1 | Chromitite | Chr | 0.04 | 0.07 | 0.03 | 0.03 | Ol | −0.33 | 0.04 | −0.16 | 0.02 | 0.37 | 875 | |
KZ14–29 | Chromitite | Chr | 0.18 | 0.04 | 0.10 | 0.04 | Ol | −0.36 | 0.06 | −0.19 | 0.04 | 0.54 | 787 | |
KZ14–32-2 | Chromitite | Chr | 0.04 | 0.06 | 0.02 | 0.04 | Ol | −0.42 | 0.05 | −0.20 | 0.04 | 0.46 | 844 | |
KZ14–36 | Chromitite | Chr | 0.19 | 0.07 | 0.10 | 0.04 | ||||||||
KZ14–38 | Chromitite | Chr | 0.21 | 0.03 | 0.11 | 0.04 | Ol | −0.32 | 0.04 | −0.16 | 0.02 | 0.53 | 762 | |
KZ15–10 | Chromitite | Chr | 0.78 | 0.04 | 0.41 | 0.03 | Ol | −0.40 | 0.03 | −0.21 | 0.03 | 1.18 | 731 | |
KZ15–20 | Chromitite | Chr | 0.14 | 0.04 | 0.07 | 0.03 | ||||||||
KZ15–21 | Chromitite | Chr | −0.03 | 0.06 | −0.01 | 0.04 | Ol | −0.10 | 0.05 | −0.04 | 0.04 | 0.07 | 756 | |
KZ15–23 | Chromitite | Chr | 0.09 | 0.05 | 0.06 | 0.04 | Ol | −0.48 | 0.03 | −0.26 | 0.04 | 0.57 | 751 | |
KZ15–30 | Chromitite | Chr | −0.10 | 0.02 | −0.05 | 0.02 | Ol | −0.27 | 0.04 | −0.16 | 0.04 | 0.17 | 695 | |
KZ15–34 | Ol | −0.39 | 0.06 | −0.19 | 0.06 | |||||||||
KZ15–36 | Chromitite | Chr | −0.09 | 0.04 | −0.03 | 0.02 | Ol | −0.43 | 0.03 | −0.22 | 0.03 | 0.34 | 813 | |
KZ15–37 | Chromitite | Chr | 0.15 | 0.04 | 0.09 | 0.04 | Ol | −0.41 | 0.06 | −0.21 | 0.06 | 0.57 | 851 | |
Chrc | 0.19 | 0.06 | 0.10 | 0.04 | ||||||||||
KZ15–38 | Chromitite | Chr | 0.04 | 0.06 | 0.03 | 0.03 |
(Continued)
Magnesium isotopic (‰) compositions of chromite and olivine in the dunites and chromitites from Luobusa and Kızıldağ ophiolites, and Gaositai Alaskan-type complex
Locality | Sample | Rock type | Mineral | δ26Mg | 2SD | δ25Mg | 2SD | Mineral | δ26Mg | 2SD | δ25Mg | 2SD | Δ26MgChr-Ola | T(°C)b |
Luobusa | LBS09005–8 | Chromitite | Chr | 0.17 | 0.04 | 0.09 | 0.05 | Ol | −0.20 | 0.07 | −0.11 | 0.05 | 0.37 | 722 |
LBS13–21 | Chromitite | Chr | 0.30 | 0.04 | 0.17 | 0.05 | ||||||||
LBS13–22 | Chromitite | Chr | 0.29 | 0.04 | 0.17 | 0.05 | ||||||||
LBS13–23 | Chromitite | Chr | 0.20 | 0.04 | 0.09 | 0.05 | ||||||||
LBS13–34 | Chromitite | Chr | −0.19 | 0.04 | −0.11 | 0.05 | ||||||||
LBS13–39 | Chromitite | Chr | −0.11 | 0.04 | −0.06 | 0.05 | ||||||||
L1 | Chromitite | Chr | 0.08 | 0.05 | 0.04 | 0.04 | ||||||||
L2 | Chromitite | Chr | 0.12 | 0.05 | 0.07 | 0.04 | ||||||||
L4 | Chromitite | Chr | 0.01 | 0.05 | 0.00 | 0.04 | ||||||||
Chrc | −0.04 | 0.06 | −0.01 | 0.05 | ||||||||||
L3 | Chromitite | Chr | 0.09 | 0.05 | 0.05 | 0.04 | ||||||||
L5 | Chromitite | Chr | 0.06 | 0.05 | 0.02 | 0.04 | ||||||||
L6 | Chromitite | Chr | −0.15 | 0.05 | −0.09 | 0.04 | ||||||||
Chrc | −0.10 | 0.06 | −0.05 | 0.05 | ||||||||||
L7 | Chromitite | Chr | −0.05 | 0.05 | −0.04 | 0.04 | ||||||||
L06a | Chromitite | Chr | −0.06 | 0.04 | −0.02 | 0.05 | ||||||||
Kızıldağ | KZ14–15 | Dunite | Chr | 0.23 | 0.03 | 0.12 | 0.03 | Ol | −0.32 | 0.06 | −0.16 | 0.05 | 0.55 | 699 |
KZ14–33 | Dunite | Chr | 0.19 | 0.03 | 0.10 | 0.03 | Ol | −0.30 | 0.06 | −0.15 | 0.05 | 0.50 | 754 | |
KZ14–31 | Dunite | Chr | 0.17 | 0.04 | 0.09 | 0.03 | ||||||||
KZ14–40 | Dunite | Chr | 0.05 | 0.01 | 0.04 | 0.02 | Ol | −0.30 | 0.04 | −0.16 | 0.02 | 0.35 | 807 | |
KZ15–09 | Dunite | Chr | 0.34 | 0.06 | 0.19 | 0.04 | Ol | −0.32 | 0.06 | −0.17 | 0.05 | 0.66 | 732 | |
KZ15–16 | Dunite | Chr | 0.07 | 0.03 | 0.02 | 0.05 | Ol | −0.26 | 0.03 | −0.12 | 0.06 | 0.33 | 777 | |
Olc | −0.34 | 0.03 | −0.17 | 0.04 | 0.41 | |||||||||
KZ14–27-1 | Chromitite | Chr | 0.21 | 0.06 | 0.11 | 0.04 | ||||||||
KZ14–27-3 | Chromitite | Chr | 0.03 | 0.03 | 0.02 | 0.02 | Ol | −0.43 | 0.02 | −0.22 | 0.02 | 0.47 | 769 | |
KZ14–28-1 | Chromitite | Chr | 0.04 | 0.07 | 0.03 | 0.03 | Ol | −0.33 | 0.04 | −0.16 | 0.02 | 0.37 | 875 | |
KZ14–29 | Chromitite | Chr | 0.18 | 0.04 | 0.10 | 0.04 | Ol | −0.36 | 0.06 | −0.19 | 0.04 | 0.54 | 787 | |
KZ14–32-2 | Chromitite | Chr | 0.04 | 0.06 | 0.02 | 0.04 | Ol | −0.42 | 0.05 | −0.20 | 0.04 | 0.46 | 844 | |
KZ14–36 | Chromitite | Chr | 0.19 | 0.07 | 0.10 | 0.04 | ||||||||
KZ14–38 | Chromitite | Chr | 0.21 | 0.03 | 0.11 | 0.04 | Ol | −0.32 | 0.04 | −0.16 | 0.02 | 0.53 | 762 | |
KZ15–10 | Chromitite | Chr | 0.78 | 0.04 | 0.41 | 0.03 | Ol | −0.40 | 0.03 | −0.21 | 0.03 | 1.18 | 731 | |
KZ15–20 | Chromitite | Chr | 0.14 | 0.04 | 0.07 | 0.03 | ||||||||
KZ15–21 | Chromitite | Chr | −0.03 | 0.06 | −0.01 | 0.04 | Ol | −0.10 | 0.05 | −0.04 | 0.04 | 0.07 | 756 | |
KZ15–23 | Chromitite | Chr | 0.09 | 0.05 | 0.06 | 0.04 | Ol | −0.48 | 0.03 | −0.26 | 0.04 | 0.57 | 751 | |
KZ15–30 | Chromitite | Chr | −0.10 | 0.02 | −0.05 | 0.02 | Ol | −0.27 | 0.04 | −0.16 | 0.04 | 0.17 | 695 | |
KZ15–34 | Ol | −0.39 | 0.06 | −0.19 | 0.06 | |||||||||
KZ15–36 | Chromitite | Chr | −0.09 | 0.04 | −0.03 | 0.02 | Ol | −0.43 | 0.03 | −0.22 | 0.03 | 0.34 | 813 | |
KZ15–37 | Chromitite | Chr | 0.15 | 0.04 | 0.09 | 0.04 | Ol | −0.41 | 0.06 | −0.21 | 0.06 | 0.57 | 851 | |
Chrc | 0.19 | 0.06 | 0.10 | 0.04 | ||||||||||
KZ15–38 | Chromitite | Chr | 0.04 | 0.06 | 0.03 | 0.03 |
Locality | Sample | Rock type | Mineral | δ26Mg | 2SD | δ25Mg | 2SD | Mineral | δ26Mg | 2SD | δ25Mg | 2SD | Δ26MgChr-Ola | T(°C)b |
Luobusa | LBS09005–8 | Chromitite | Chr | 0.17 | 0.04 | 0.09 | 0.05 | Ol | −0.20 | 0.07 | −0.11 | 0.05 | 0.37 | 722 |
LBS13–21 | Chromitite | Chr | 0.30 | 0.04 | 0.17 | 0.05 | ||||||||
LBS13–22 | Chromitite | Chr | 0.29 | 0.04 | 0.17 | 0.05 | ||||||||
LBS13–23 | Chromitite | Chr | 0.20 | 0.04 | 0.09 | 0.05 | ||||||||
LBS13–34 | Chromitite | Chr | −0.19 | 0.04 | −0.11 | 0.05 | ||||||||
LBS13–39 | Chromitite | Chr | −0.11 | 0.04 | −0.06 | 0.05 | ||||||||
L1 | Chromitite | Chr | 0.08 | 0.05 | 0.04 | 0.04 | ||||||||
L2 | Chromitite | Chr | 0.12 | 0.05 | 0.07 | 0.04 | ||||||||
L4 | Chromitite | Chr | 0.01 | 0.05 | 0.00 | 0.04 | ||||||||
Chrc | −0.04 | 0.06 | −0.01 | 0.05 | ||||||||||
L3 | Chromitite | Chr | 0.09 | 0.05 | 0.05 | 0.04 | ||||||||
L5 | Chromitite | Chr | 0.06 | 0.05 | 0.02 | 0.04 | ||||||||
L6 | Chromitite | Chr | −0.15 | 0.05 | −0.09 | 0.04 | ||||||||
Chrc | −0.10 | 0.06 | −0.05 | 0.05 | ||||||||||
L7 | Chromitite | Chr | −0.05 | 0.05 | −0.04 | 0.04 | ||||||||
L06a | Chromitite | Chr | −0.06 | 0.04 | −0.02 | 0.05 | ||||||||
Kızıldağ | KZ14–15 | Dunite | Chr | 0.23 | 0.03 | 0.12 | 0.03 | Ol | −0.32 | 0.06 | −0.16 | 0.05 | 0.55 | 699 |
KZ14–33 | Dunite | Chr | 0.19 | 0.03 | 0.10 | 0.03 | Ol | −0.30 | 0.06 | −0.15 | 0.05 | 0.50 | 754 | |
KZ14–31 | Dunite | Chr | 0.17 | 0.04 | 0.09 | 0.03 | ||||||||
KZ14–40 | Dunite | Chr | 0.05 | 0.01 | 0.04 | 0.02 | Ol | −0.30 | 0.04 | −0.16 | 0.02 | 0.35 | 807 | |
KZ15–09 | Dunite | Chr | 0.34 | 0.06 | 0.19 | 0.04 | Ol | −0.32 | 0.06 | −0.17 | 0.05 | 0.66 | 732 | |
KZ15–16 | Dunite | Chr | 0.07 | 0.03 | 0.02 | 0.05 | Ol | −0.26 | 0.03 | −0.12 | 0.06 | 0.33 | 777 | |
Olc | −0.34 | 0.03 | −0.17 | 0.04 | 0.41 | |||||||||
KZ14–27-1 | Chromitite | Chr | 0.21 | 0.06 | 0.11 | 0.04 | ||||||||
KZ14–27-3 | Chromitite | Chr | 0.03 | 0.03 | 0.02 | 0.02 | Ol | −0.43 | 0.02 | −0.22 | 0.02 | 0.47 | 769 | |
KZ14–28-1 | Chromitite | Chr | 0.04 | 0.07 | 0.03 | 0.03 | Ol | −0.33 | 0.04 | −0.16 | 0.02 | 0.37 | 875 | |
KZ14–29 | Chromitite | Chr | 0.18 | 0.04 | 0.10 | 0.04 | Ol | −0.36 | 0.06 | −0.19 | 0.04 | 0.54 | 787 | |
KZ14–32-2 | Chromitite | Chr | 0.04 | 0.06 | 0.02 | 0.04 | Ol | −0.42 | 0.05 | −0.20 | 0.04 | 0.46 | 844 | |
KZ14–36 | Chromitite | Chr | 0.19 | 0.07 | 0.10 | 0.04 | ||||||||
KZ14–38 | Chromitite | Chr | 0.21 | 0.03 | 0.11 | 0.04 | Ol | −0.32 | 0.04 | −0.16 | 0.02 | 0.53 | 762 | |
KZ15–10 | Chromitite | Chr | 0.78 | 0.04 | 0.41 | 0.03 | Ol | −0.40 | 0.03 | −0.21 | 0.03 | 1.18 | 731 | |
KZ15–20 | Chromitite | Chr | 0.14 | 0.04 | 0.07 | 0.03 | ||||||||
KZ15–21 | Chromitite | Chr | −0.03 | 0.06 | −0.01 | 0.04 | Ol | −0.10 | 0.05 | −0.04 | 0.04 | 0.07 | 756 | |
KZ15–23 | Chromitite | Chr | 0.09 | 0.05 | 0.06 | 0.04 | Ol | −0.48 | 0.03 | −0.26 | 0.04 | 0.57 | 751 | |
KZ15–30 | Chromitite | Chr | −0.10 | 0.02 | −0.05 | 0.02 | Ol | −0.27 | 0.04 | −0.16 | 0.04 | 0.17 | 695 | |
KZ15–34 | Ol | −0.39 | 0.06 | −0.19 | 0.06 | |||||||||
KZ15–36 | Chromitite | Chr | −0.09 | 0.04 | −0.03 | 0.02 | Ol | −0.43 | 0.03 | −0.22 | 0.03 | 0.34 | 813 | |
KZ15–37 | Chromitite | Chr | 0.15 | 0.04 | 0.09 | 0.04 | Ol | −0.41 | 0.06 | −0.21 | 0.06 | 0.57 | 851 | |
Chrc | 0.19 | 0.06 | 0.10 | 0.04 | ||||||||||
KZ15–38 | Chromitite | Chr | 0.04 | 0.06 | 0.03 | 0.03 |
(Continued)
Locality | Sample | Rock type | Mineral | δ26Mg | 2SD | δ25Mg | 2SD | Mineral | δ26Mg | 2SD | δ25Mg | 2SD | Δ26MgChr-Ola | T(°C)b |
Gaositaid | GST-18 | Dunite | Chr | 0.38 | 0.04 | 0.18 | 0.05 | Ol | −0.34 | 0.04 | −0.17 | 0.02 | 0.72 | 822 |
GST-33-2 | Dunite | Chr | 0.28 | 0.04 | 0.15 | 0.05 | Ol | −0.34 | 0.04 | −0.16 | 0.02 | 0.62 | 792 | |
GST-12 | Chromitite | Chr | 0.19 | 0.04 | 0.07 | 0.05 | Ol | −0.30 | 0.04 | −0.15 | 0.02 | 0.49 | 829 | |
GST-17 | Chromitite | Chr | 0.12 | 0.04 | 0.06 | 0.05 | Ol | −0.31 | 0.04 | −0.17 | 0.02 | 0.43 | 753 | |
GST-20 | Chromitite | Chr | 0.08 | 0.04 | 0.06 | 0.05 | ||||||||
GST-22 | Chromitite | Chr | 0.04 | 0.04 | 0.00 | 0.05 | ||||||||
GST-23 | Chromitite | Chr | 0.42 | 0.04 | 0.23 | 0.05 | ||||||||
GST-25 | Chromitite | Chr | 0.34 | 0.04 | 0.18 | 0.05 | ||||||||
GST-26 | Chromitite | Chr | 0.30 | 0.04 | 0.13 | 0.05 | ||||||||
GST-31 | Chromitite | Chr | 0.21 | 0.04 | 0.09 | 0.05 | Ol | −0.38 | 0.04 | −0.18 | 0.02 | 0.59 | 883 | |
GST-35-1 | Chromitite | Chr | 0.16 | 0.04 | 0.07 | 0.05 | Ol | −0.34 | 0.04 | −0.19 | 0.02 | 0.50 | 1004 | |
Standard | CUGB | GSB | −2.02 | 0.04 | −1.04 | 0.01 | ||||||||
CUGB | GSB | −2.04 | 0.01 | −1.05 | 0.01 | |||||||||
CUGB | BHVO-2 | −0.22 | 0.03 | −0.11 | 0.05 | |||||||||
CUGB | BCR-2 | −0.19 | 0.06 | −0.09 | 0.05 | |||||||||
UW | San Carlos olivine | −0.30 | 0.04 | −0.15 | 0.05 | |||||||||
UW | SEA-1 | −0.82 | 0.04 | −0.43 | 0.05 |
Locality | Sample | Rock type | Mineral | δ26Mg | 2SD | δ25Mg | 2SD | Mineral | δ26Mg | 2SD | δ25Mg | 2SD | Δ26MgChr-Ola | T(°C)b |
Gaositaid | GST-18 | Dunite | Chr | 0.38 | 0.04 | 0.18 | 0.05 | Ol | −0.34 | 0.04 | −0.17 | 0.02 | 0.72 | 822 |
GST-33-2 | Dunite | Chr | 0.28 | 0.04 | 0.15 | 0.05 | Ol | −0.34 | 0.04 | −0.16 | 0.02 | 0.62 | 792 | |
GST-12 | Chromitite | Chr | 0.19 | 0.04 | 0.07 | 0.05 | Ol | −0.30 | 0.04 | −0.15 | 0.02 | 0.49 | 829 | |
GST-17 | Chromitite | Chr | 0.12 | 0.04 | 0.06 | 0.05 | Ol | −0.31 | 0.04 | −0.17 | 0.02 | 0.43 | 753 | |
GST-20 | Chromitite | Chr | 0.08 | 0.04 | 0.06 | 0.05 | ||||||||
GST-22 | Chromitite | Chr | 0.04 | 0.04 | 0.00 | 0.05 | ||||||||
GST-23 | Chromitite | Chr | 0.42 | 0.04 | 0.23 | 0.05 | ||||||||
GST-25 | Chromitite | Chr | 0.34 | 0.04 | 0.18 | 0.05 | ||||||||
GST-26 | Chromitite | Chr | 0.30 | 0.04 | 0.13 | 0.05 | ||||||||
GST-31 | Chromitite | Chr | 0.21 | 0.04 | 0.09 | 0.05 | Ol | −0.38 | 0.04 | −0.18 | 0.02 | 0.59 | 883 | |
GST-35-1 | Chromitite | Chr | 0.16 | 0.04 | 0.07 | 0.05 | Ol | −0.34 | 0.04 | −0.19 | 0.02 | 0.50 | 1004 | |
Standard | CUGB | GSB | −2.02 | 0.04 | −1.04 | 0.01 | ||||||||
CUGB | GSB | −2.04 | 0.01 | −1.05 | 0.01 | |||||||||
CUGB | BHVO-2 | −0.22 | 0.03 | −0.11 | 0.05 | |||||||||
CUGB | BCR-2 | −0.19 | 0.06 | −0.09 | 0.05 | |||||||||
UW | San Carlos olivine | −0.30 | 0.04 | −0.15 | 0.05 | |||||||||
UW | SEA-1 | −0.82 | 0.04 | −0.43 | 0.05 |
Note: aTemperature (T) is estimated using the olivine-spinel geothermometer defined by Ballhaus et al. (1991);
Δ26MgChr-Ol = δ26MgChr - δ26MgOl; c Replicate: repeat sample dissolution, column chemistry and instrumental analysis;
Samples from Gaositai Alaskan-type complex were determined at the University of Washington (Seattle) and the others were determined at CUGB.
Locality | Sample | Rock type | Mineral | δ26Mg | 2SD | δ25Mg | 2SD | Mineral | δ26Mg | 2SD | δ25Mg | 2SD | Δ26MgChr-Ola | T(°C)b |
Gaositaid | GST-18 | Dunite | Chr | 0.38 | 0.04 | 0.18 | 0.05 | Ol | −0.34 | 0.04 | −0.17 | 0.02 | 0.72 | 822 |
GST-33-2 | Dunite | Chr | 0.28 | 0.04 | 0.15 | 0.05 | Ol | −0.34 | 0.04 | −0.16 | 0.02 | 0.62 | 792 | |
GST-12 | Chromitite | Chr | 0.19 | 0.04 | 0.07 | 0.05 | Ol | −0.30 | 0.04 | −0.15 | 0.02 | 0.49 | 829 | |
GST-17 | Chromitite | Chr | 0.12 | 0.04 | 0.06 | 0.05 | Ol | −0.31 | 0.04 | −0.17 | 0.02 | 0.43 | 753 | |
GST-20 | Chromitite | Chr | 0.08 | 0.04 | 0.06 | 0.05 | ||||||||
GST-22 | Chromitite | Chr | 0.04 | 0.04 | 0.00 | 0.05 | ||||||||
GST-23 | Chromitite | Chr | 0.42 | 0.04 | 0.23 | 0.05 | ||||||||
GST-25 | Chromitite | Chr | 0.34 | 0.04 | 0.18 | 0.05 | ||||||||
GST-26 | Chromitite | Chr | 0.30 | 0.04 | 0.13 | 0.05 | ||||||||
GST-31 | Chromitite | Chr | 0.21 | 0.04 | 0.09 | 0.05 | Ol | −0.38 | 0.04 | −0.18 | 0.02 | 0.59 | 883 | |
GST-35-1 | Chromitite | Chr | 0.16 | 0.04 | 0.07 | 0.05 | Ol | −0.34 | 0.04 | −0.19 | 0.02 | 0.50 | 1004 | |
Standard | CUGB | GSB | −2.02 | 0.04 | −1.04 | 0.01 | ||||||||
CUGB | GSB | −2.04 | 0.01 | −1.05 | 0.01 | |||||||||
CUGB | BHVO-2 | −0.22 | 0.03 | −0.11 | 0.05 | |||||||||
CUGB | BCR-2 | −0.19 | 0.06 | −0.09 | 0.05 | |||||||||
UW | San Carlos olivine | −0.30 | 0.04 | −0.15 | 0.05 | |||||||||
UW | SEA-1 | −0.82 | 0.04 | −0.43 | 0.05 |
Locality | Sample | Rock type | Mineral | δ26Mg | 2SD | δ25Mg | 2SD | Mineral | δ26Mg | 2SD | δ25Mg | 2SD | Δ26MgChr-Ola | T(°C)b |
Gaositaid | GST-18 | Dunite | Chr | 0.38 | 0.04 | 0.18 | 0.05 | Ol | −0.34 | 0.04 | −0.17 | 0.02 | 0.72 | 822 |
GST-33-2 | Dunite | Chr | 0.28 | 0.04 | 0.15 | 0.05 | Ol | −0.34 | 0.04 | −0.16 | 0.02 | 0.62 | 792 | |
GST-12 | Chromitite | Chr | 0.19 | 0.04 | 0.07 | 0.05 | Ol | −0.30 | 0.04 | −0.15 | 0.02 | 0.49 | 829 | |
GST-17 | Chromitite | Chr | 0.12 | 0.04 | 0.06 | 0.05 | Ol | −0.31 | 0.04 | −0.17 | 0.02 | 0.43 | 753 | |
GST-20 | Chromitite | Chr | 0.08 | 0.04 | 0.06 | 0.05 | ||||||||
GST-22 | Chromitite | Chr | 0.04 | 0.04 | 0.00 | 0.05 | ||||||||
GST-23 | Chromitite | Chr | 0.42 | 0.04 | 0.23 | 0.05 | ||||||||
GST-25 | Chromitite | Chr | 0.34 | 0.04 | 0.18 | 0.05 | ||||||||
GST-26 | Chromitite | Chr | 0.30 | 0.04 | 0.13 | 0.05 | ||||||||
GST-31 | Chromitite | Chr | 0.21 | 0.04 | 0.09 | 0.05 | Ol | −0.38 | 0.04 | −0.18 | 0.02 | 0.59 | 883 | |
GST-35-1 | Chromitite | Chr | 0.16 | 0.04 | 0.07 | 0.05 | Ol | −0.34 | 0.04 | −0.19 | 0.02 | 0.50 | 1004 | |
Standard | CUGB | GSB | −2.02 | 0.04 | −1.04 | 0.01 | ||||||||
CUGB | GSB | −2.04 | 0.01 | −1.05 | 0.01 | |||||||||
CUGB | BHVO-2 | −0.22 | 0.03 | −0.11 | 0.05 | |||||||||
CUGB | BCR-2 | −0.19 | 0.06 | −0.09 | 0.05 | |||||||||
UW | San Carlos olivine | −0.30 | 0.04 | −0.15 | 0.05 | |||||||||
UW | SEA-1 | −0.82 | 0.04 | −0.43 | 0.05 |
Note: aTemperature (T) is estimated using the olivine-spinel geothermometer defined by Ballhaus et al. (1991);
Δ26MgChr-Ol = δ26MgChr - δ26MgOl; c Replicate: repeat sample dissolution, column chemistry and instrumental analysis;
Samples from Gaositai Alaskan-type complex were determined at the University of Washington (Seattle) and the others were determined at CUGB.
RESULTS
The Mg isotopic compositions of mineral separates, including olivine and chromite in the dunites and chromitites of the Luobusa and Kızıldağ ophiolites, and the Gaositai Alaskan-type complex, are presented in Tables 1 and 2 along with their major oxide compositions and equilibration temperatures. Olivine from only one Luobusa sample (LBS09005-8) was measured. Olivine in the other Luobusa samples was considered to be too altered to return reliable results.
Mineral chemistry
Chromites from the upper mantle-derived Luobusa chromitites are Cr-rich and have relatively low MgO contents (13.2–16.5 wt.%) and Cr# values (100 × Cr/(Cr + Al); 75.0–79.8). These values are slightly higher than those in the Cr- and Al-rich Kızıldağ dunites and chromitites (MgO = 10.7–15.1 wt.%; Cr# = 40.9–75.0) (Fig. 2a–c; Table 1). Coexisting olivines from both ophiolites are forsteritic, with Fo values (100 × Mg/(Mg + Fe)) varying from 90.7 to 96.2 (Fig. 2d; Table 1).
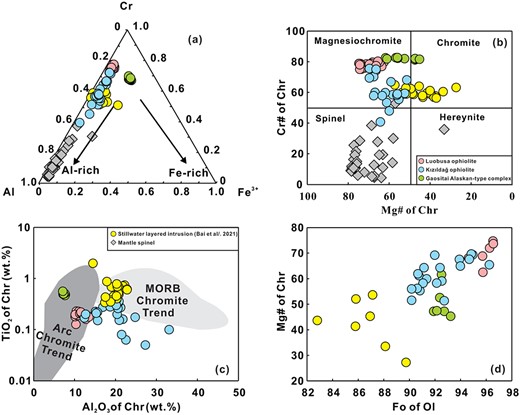
Chromite and olivine chemical compositions of dunites and chromitites from the Luobusa and Kızıldağ ophiolites and Gaositai Alaskan-type complex. The previously reported data of Luobusa ophiolite and Stillwater layered intrusion are from Xiao et al. (2016) and Bai et al. (2021). Grey filled diamonds represent chromite data in the mantle peridotites (Young et al., 2009; Liu et al., 2011; Xiao et al., 2013; Stracke et al., 2018). The fields of arc and MORB chromites in Figure 1c are after Kamenetsky et al. (2001). For simplification, both spinel and chromite are used synonymously with chromite in the main text.
Chromites from the Gaositai Alaskan-type complex are Fe- and Cr-rich. They have similar MgO contents (9.40–15.1 wt.%) but higher Cr# values (81.4–83.0) than those in the ophiolites (Fig. 2a–c; Table 1). Olivines have Fo values of 92.0 to 93.3 (Fig. 2d; Table 1).
Magnesium isotopic compositions of chromite and olivine
The Mg isotopic compositions of chromite in the dunites and chromitites display a total variation of more than 1‰, and extend to higher values than mantle chromites reported previously (Young et al., 2009; Liu et al., 2011; Xiao et al., 2013; Fig. 3). In detail, δ26Mg values of chromite vary from −0.19‰ to 0.30‰ in the Luobusa ophiolite, −0.09‰ to 0.78‰ in the Kızıldağ ophiolite, −0.04‰ to 0.42‰ in the Gaositai Alaskan-type complex (Table 2 and Fig. 3). The coexisting olivines always have lower Mg isotopic compositions, and their δ26Mg values show much smaller variations ranging from −0.48‰ to −0.10‰ in the Kızıldağ ophiolite, −0.38‰ to −0.30‰ in the Gaositai Alaskan-type complex, similar to those previously reported from Stillwater layered intrusion (−0.40‰ to −0.27‰; Bai et al., 2021). Most olivines also have slightly lower δ26Mg values than those of upper mantle rocks (−0.25 ± 0.04‰, Teng et al., 2010). The Δ26MgChr-Ol values mostly vary from 0.07‰ to 1.18‰ in the Kızıldağ ophiolite, 0.43 to 0.72‰ in the Gaositai Alaskan-type complex, slightly lower than those in the Stillwater intrusion (Table 2 and Fig. 4a; Bai et al., 2021).
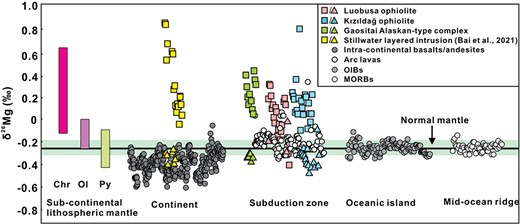
Magnesium isotopic compositions of chromite and olivine in the dunites and chromitites from the Luobusa and Kızıldağ ophiolites and Gaositai Alaskan-type complex, along with mantle-derived lava data in literature. The data of Stillwater layered intrusion are from Bai et al. (2021). The filled square and triangle symbols represent chromite and olivine, respectively. The Mg isotopic composition of intra-continental basalts, MORBs, OIBs, arc lavas and mantle minerals (chromite, olivine, and pyroxene) were reported by Handler et al. (2009), Yang et al. (2009, 2012), Bourdon et al. (2010), Teng et al. (2010, 2016), Liu et al. (2011), Macris et al. (2013), Xiao et al. (2013, 2016), Huang et al. (2015), Young et al. (2009), Liu et al. (2015), Tian et al. (2016, 2018), Wang et al. (2016), Li et al. (2017), Su et al. (2019), Sun et al. (2017), Wang et al. (2017, 2018), Zhong et al. (2017), Brewer et al. (2018), Cheng et al. (2018), Stracke et al. (2018) and Pang et al. (2020). The solid line and green bar represent the average δ26Mg value of normal mantle and its 2SD value, respectively, as sampled by global peridotite xenoliths (−0.25 ± 0.04‰; Teng et al., 2010). Chr, chromite; Ol, olivine; Py, pyroxene.
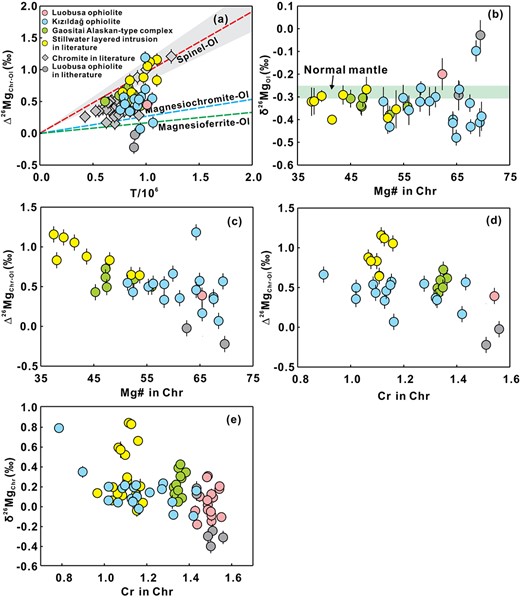
Comparison of measured chromite-olivine Mg isotope fractionation with theoretical calculation as a function of temperature (a); δ26MgOl vs. Mg# in chromite (b); variations of Δ26MgChr-Ol with Mg# (c) and Cr (d) in chromite, δ26MgChr vs. Cr in chromite (e). The Cr of chromite was calculated based on microprobe analyses using stoichiometric criteria (Droop, 1985). Theoretical calculations for pure spinel, magnesiochromite and magnesioferrite end members are from Schauble (2011). The previously reported data of Stillwater layered intrusion are from Bai et al. (2021). Grey filled diamonds represent chromite Mg isotopic composition in literature (Young et al., 2009; Liu et al., 2011; Xiao et al., 2013; Stracke et al., 2018; Su et al., 2019). Chr = chromite, Ol = olivine. The green bar in the Fig. 3a represent the average δ26Mg of normal mantle, as sampled by global peridotite xenoliths (−0.25 ± 0.04‰; Teng et al., 2010).
DISCUSSION
The data presented here demonstrate that chromite in magmatic dunites and chromitites has variable and significantly higher Mg isotopic compositions than coexisting olivine, or previously published values for mantle-derived magmas (Su et al., 2019; Bai et al., 2021). In this section, we first discuss the tectonic control on chromite fractionation and Mg isotopic variations of mantle-derived magmas. Then, we investigate the impact of olivine and chromite crystallization on Mg isotopic compositions of intra-continental basalts.
Magnesium isotope fractionation between chromite and olivine in magmatic dunites and chromitites
Previous experimental studies have shown that the Mg isotopic composition of a specific spinel species depends strongly on the cation species occupying the octahedral site (i.e. the identity of B3+ in the general formula of Macris et al., 2013). Enrichment of 26Mg in common spinel endmembers follows the sequence of Al-spinel [MgAl2O4] > magnesiochromite [MgCr2O4] > magnesioferrite [MgFe2O4] (Schauble, 2011; Macris et al., 2013; Tang et al., 2021). Thus, natural spinel group minerals with varying amounts of Al, Cr, and Fe3+ in the octahedral site should possess Δ26MgSpl/Chr-Ol values intermediate between these endmember compositions.
Chromite [FeCr2O4] forms a continuous solid solution series with magnesiochromite [MgCr2O4], whereby Mg2+ substitutes for Fe2+. Additional mixing with Al-spinel hercynite [FeAl2O4] occurs when Cr is replaced by Fe3+ or Al3+. The Δ26MgChr-Ol values in the rock suites investigated here fall largely between the equilibrium fractionation lines of spinel–olivine pairs and magnesioferrite–olivine pairs, suggesting that chromite is in isotopic equilibrium with coexisting olivine (Fig. 4a). Olivines in all our samples have Mg isotopic ranges from −0.48 to −0.10‰ and do not vary with increasing degrees of differentiation as indicated by decreasing Mg# in chromite (Fig. 4b). Therefore, the variation in Δ26MgChr-Ol mainly reflects a change in chromite δ26Mg driven by its compositional variation during evolution of the host magma (Fig. 4c–e). Diffusion of Fe–Mg between chromite and olivine is known to occur at subsolidus temperatures during which Mg diffuses from chromite to olivine and Fe from olivine to chromite. This process would result in higher Fo and lower δ26Mg values in olivine with a corresponding increase in δ26Mg of chromite (Xiao et al., 2016). However, most olivines in the studied complexes have narrow Mg isotopic compositions at intra- and inter-complex scales and do not correlate with the Mg# of chromite, suggesting that Mg isotope compositions in these rocks have been little changed by Fe–Mg exchange (Fig. 4b). Kamenetsky et al. (2001) demonstrated that Fe–Mg exchange between olivine and chromite is considered to be minimal (< 5 mol% Mg#), and that the decrease in chromite Mg# is mainly due to fractional crystallization (Fig. 2d). In addition, the observed decrease in Mg# (and increase in Fe2+) in chromite as Fo contents decrease is most likely controlled by spinel-liquid equilibrium (Fig. 2d). Our study shows that within a given complex, the Δ26MgChr-Ol values increase with decreasing Mg# and Cr content of chromite in the dunites and chromitites, reflecting the effect of compositional variations on equilibrium chromite–olivine fractionation during magma evolution (Fig. 4d).
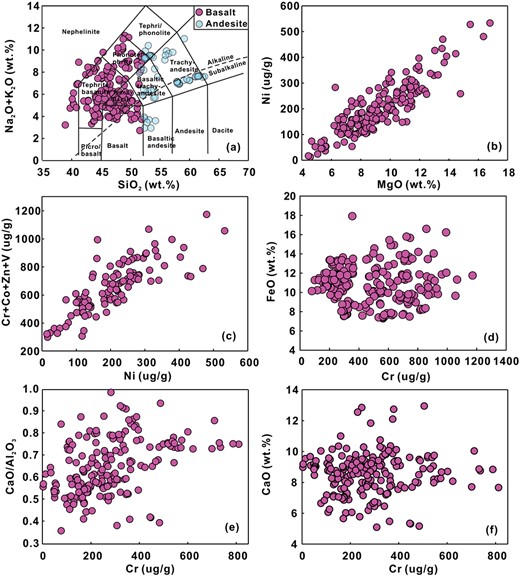
Na2O + K2O vs. SiO2 for intra-continental mantle-derived magmas (a). Plots of Ni vs. MgO (b), Cr + Co + Zn + V vs. Ni (c), FeO (d), CaO/Al2O3 (e) and CaO vs. Cr (f) in intra-continental basalts with SiO2 < 52 wt.% and MgO > 4%. The data of intra-continental basaltic magmas are from Yang et al. (2012), Huang et al. (2015), Tian et al. (2016, 2018), Liu et al. (2015), Wang et al. (2016), Li et al. (2017), Su et al. (2017b), Sun et al. (2017), Wang et al. (2017), Cheng et al. (2018).
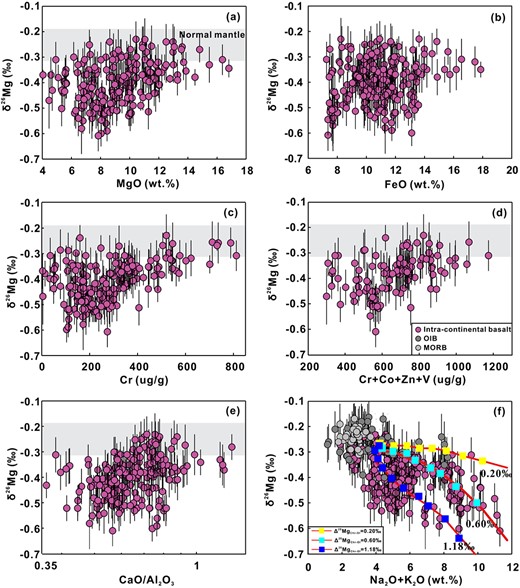
δ26Mg vs. MgO (a), FeO (b), Cr + Co + Zn + V (c), CaO/Al2O3 ratio (d) and Na2O + K2O (e) in intra-continental basalts with SiO2 < 52 wt.% and MgO > 4%. The data of intra-continental basalts are from Yang et al. (2012), Huang et al. (2015), Tian et al. (2016, 2018), Liu et al. (2015), Wang et al. (2016), Li et al. (2017), Su et al. (2017b), Sun et al. (2017), Wang et al. (2017), Cheng et al. (2018). The Mg isotopic composition of MORBs and OIBs were reported by Teng et al. (2010), Zhong et al. (2017) and Wang et al. (2018). The solid lines in the Fig 6f represent calculated Mg isotopic compositions of residual melts during fractional crystallization of olivine and chromite by assuming a Rayleigh distillation process with average crystal-melt fractionation factors (Δ26Mgcrystal-melt) of 0.20‰, 0.60‰ and 1.18‰.
Control of tectonic setting on chromite fractionation and mg isotopic variations of mantle-derived magmas
Chromite is a common solidus phase in basaltic magmas during the early stage of fractionation in a variety of tectonic settings. Mutch et al. (2020) demonstrated that primary magma can be stored near the Moho for thousands of years after initial mixing and then be rapidly transported over days to the mid- or shallow-level crust. Thus, cooling of magma bodies situated in cold country rock will result in crystallization and crystal segregation. Crystal segregation inevitably results in a progressive decrease in the mass of the residual liquid and a change in its composition (Lee et al., 2014). Crystallization of chromite with different chemical compositions during basaltic melt differentiation may therefore induce isotopic differences between mineral cumulates and an evolved magma.
The previously reported chromitites from the Stillwater layered intrusion in a continental setting (Bai et al., 2021) have lower Cr and MgO contents than those from the Luobusa and Kızıldağ ophiolites and the Gaositai Alaskan-type intrusion formed in subduction zone environments (Fig. 2). Experimental results also show that an increase in chromite Cr# can be produced by a pressure drop from 15 to 2 kbar (Roeder & Reynolds, 1991). We show here that the Δ26MgChr-Ol values in both dunites and chromitites decrease from the Stillwater complex to subduction-related chromitites from the Luobusa and Kızıldağ ophiolites and the Gaositai Alaskan-type intrusion with increasing Mg# and Cr content (Figs. 4c and d). These findings suggest that large Mg isotopic variations in magmatic chromites from different complexes primarily reflect inter-mineral fractionation between Al-rich chromite and olivine. Such variations would be always larger than those between Cr-rich chromite and olivine under equilibrium conditions.
On the other hand, alkaline basaltic lavas erupted on continents have typically experienced olivine and Al-chromite fractionation after low degrees of partial melting (Kamenetsky et al., 2001; Kumar & Rathna, 2014). These lavas have δ26Mg values as low as −0.6‰ (Fig. 3). As discussed above, chromite formed in continental settings may have lower Cr2O3 contents and δ26Mg values than those in mid-ocean ridges and subduction zone settings. Thus, crystallization and removal of chromite appears to be the dominant process in generating low δ26Mg intra-continental basalts (see next section for details).
In subduction zones, arc lavas have slightly higher Mg isotopic compositions than normal mantle (Fig. 3; Teng et al., 2016; Brewer et al., 2018; Pang et al., 2020), because the lavas are relatively oxidized and typically crystalize titanomagnetite or ilmenite rather than chromite. Chen et al. (2018) and Tian et al. (2020) reported that the ilmenite in mafic intrusions shows extremely large Mg isotopic variations (δ26Mg = −0.80‰ to 23.1‰). The low δ26Mg values of ilmenite are considered to be due to equilibration with coexisting silicate phases whereas the high values point to kinetic isotope fractionation during Mg–Fe exchange (Chen et al., 2018). In addition, Wang et al. (2021) showed that Fe–Ti oxide phenocrysts (titanomagnetite) in evolved samples have higher δ26Mg values (0.15 to 0.52‰) than the residual magma. These distinct Mg isotopic fractionation paths in highly evolved alkaline lavas have been considered to reflect fractional crystallization of different Fe–Ti oxides with contrasting Mg isotopic compositions (Wang et al., 2021). Therefore, fractional crystallization of titanomagnetite and ilmenite can potentially have a significant effect on the Mg isotopic composition of arc lavas.
At mid-ocean ridges, MORB lavas have homogeneous and normal mantle-like Mg isotopic compositions (Fig. 3; Teng et al., 2010), a finding that can be attributed to one or more of the following reasons: (1) Most MORBs are tholeiitic and the product of extensive melting. In addition, variations in lithosphere thickness also affect the composition of basalts (Guo et al., 2019). For example, the relatively thin lithosphere beneath mid-ocean ridges may result in limited fractional crystallization (Guo et al., 2019). (2) Very little chromitite is present in mid-ocean ridge sequences (Rollinson & Adetunji, 2013). (3) Continuous or episodic replenishment of magma storage sites situated below mid-ocean ridges may experience relatively continuous magmatic fluxing, particularly at fast-spreading ridges, which would decrease cooling and crystallization rates. Thus, the melt composition in the magma storage sites will initially be that of the parental magma and could have mantle-like δ26Mg values.
Ocean island basalts (OIB) possess slightly lower δ26Mg values than MORBs (Zhong et al., 2017; Wang et al., 2018), because they are generated from heterogeneous mantle sources that contain recycled crustal and/or lithospheric mantle materials and the magmas also undergo significant crystal fractionation. In addition, no chromitite pods have yet been reported in oceanic island sequences. Thus, melting of lithologically heterogeneous mantle sources, large overall degrees of partial melting, and later chromite crystallization may combine to make it more difficult to resolve small differences in δ26Mg values (−0.35‰ to −0.18‰; Fig. 3; Teng et al., 2010; Zhong et al., 2017; Stracke et al., 2018; Wang et al., 2018).
Impact of olivine and chromite crystallization on Mg isotopic compositions of intra-continental basalts
Low δ26Mg signatures have been documented in some Late Cretaceous and Cenozoic continental basalts from eastern China and the New Zealand (Yang et al., 2012; Huang et al., 2015; Liu et al., 2015; Tian et al., 2016, 2018; Wang et al., 2016; Li et al., 2017; Sun et al., 2017; Wang et al., 2017; Su et al., 2017; Cheng et al., 2018). These volcanic rocks include basanites, trachy-basalts, and normal basalts (SiO2 < 52 wt.%) along with minor basaltic trachyandesite and trachyandesite (SiO2 > 52 wt.%), most of which possess high total alkalis (2.72–11.3 wt.%; Fig. 5a). These rocks often have experienced oxide (chromite and Fe–Ti oxides) fractional crystallization during their ascent. The Fe–Ti oxides are commonly crystallized during the late stages of magma solidification and hence contain low MgO contents (mostly <4%). Thus, in our current study, we focused on samples with SiO2 contents <52 wt.% and MgO > 4 wt.% to minimize the effects of late-stage fractional crystallization. The MgO contents of these lavas correlated positively with Ni abundances, which are mainly controlled by the degree of olivine crystallization during magma differentiation (Fig. 5b). The elements Cr, Co, Zn, and V are highly compatible in chromite, with partition coefficients of 26 to 751, 4 to 8, 4 to 8, and 4 to 8, respectively (Pagé & Barnes, 2009; Liu et al., 2019). As shown in Fig. 5c, the Ni abundances in these lavas correlate positively with Cr and Cr + Co + Zn + V abundances, which is consistent with fractionation of olivine and chromite. Because olivine and chromite fractionation will increase Al2O3 contents in the evolving magmas (Righter et al., 2006), there is a positive correlation between Cr and FeO (or CaO/Al2O3; Fig. 5d and e). In addition, these basalts scatter widely in the plot of Cr vs CaO, indicating limited clinopyroxene fractionation (Fig. 5f). As a result, these intra-continental basalts appear to have experienced extensive polybaric crystallization of olivine and chromite.
Basalt δ26Mg values also decrease with the decrease of MgO, FeO, Cr, and Cr + Co + Zn + V abundances (Fig. 6a–d). In particular, there is a concomitant decrease in δ26Mg and CaO/Al2O3 (Fig. 6e), which is opposite to the expected negative correlation if carbonate had been incorporated in the mantle sources, where a higher contribution of isotopically low carbonates should lead to higher CaO/Al2O3 ratios and lower δ26Mg values in the basalts. (Huang et al., 2015; Li et al., 2017). Empirical and experimental studies predict that fractional crystallization of olivine from mafic melts induces very limited (< 0.07‰ for δ26Mg) Mg isotope fractionation (Teng et al., 2010; Schauble, 2011). Thus, the observed positive correlations between basalt δ26Mg values and indices of chromite crystallization strongly suggest that chromite is the major phase responsible for changes in δ26Mg value during igneous differentiation (Fig. 6). Removal of chromite is also consistent with the progressive increase in the Na2O + K2O with increasing degrees of differentiation (Fig. 6f).
CONCLUSIONS
This study presents high-precision Mg isotope data of chromite and olivine separates from cumulate rock suites formed by basaltic melt differentiation, including ophiolites and Alaskan-type complexes. Furthermore, it compares the data to published results from the Stillwater layered intrusion (Bai et al., 2021). The following conclusions can be drawn:
- (1)
The δ26Mg values of chromite (−0.19 to 0.84‰) are markedly higher than coexisting olivine (−0.48 to −0.10‰), although the coexisting minerals are in isotopic equilibrium.
- (2)
The positive correlations between Δ26MgChr-Ol values and Cr contents of chromite in dunites and chromitites are due to compositional variations in the chromite during magma evolution with equilibrium chromite–olivine fractionation.
- (3)
Alkaline basaltic magmas erupted on continents have undergone olivine and Al-chromite fractionation after low degrees of partial melting. Crystallization of isotopically high chromite lowers the δ26Mg of the basaltic magma and, along with olivine crystallization, reduces its MgO, Ni, and Cr contents; therefore, chromite crystallization plays an important role in generating the observed isotopically low-δ26Mg continental basalts.
FUNDING
This study was supported by the Second Tibetan Plateau Scientific Expedition and Research Program (STEP; 2019QZKK0801), the National Natural Science Foundation of China (41973012, 91755205 and 41772055), and project from the Ministry of Science and Technology National Key R&D Program of China (No. 2022YFC2903501).
DATA AVAILABILITY
All data presented in this study are given in tables.
CONFLICT OF INTEREST
We declare that no conflict of interest exists.
ACKNOWLEDGMENTS
The authors thank Fang-Zhen Teng and Yan Hu for their constructive comments on the original manuscript. We also thank Qian Mao and Li-Hui Jia for their assistance with the electronic microprobe analyses.Constructive comments and suggestions from the associate editor Jason Harvey and three anonymous reviewers are also highly appreciated.