-
PDF
- Split View
-
Views
-
Cite
Cite
Nidhi Kandhol, Vijay Pratap Singh, Jason C White, Lam-Son Phan Tran, Durgesh Kumar Tripathi, Plant Growth Hormones and Nanomaterial Interface: Exploring the connection from development to defense, Plant and Cell Physiology, Volume 63, Issue 12, December 2022, Pages 1840–1847, https://doi.org/10.1093/pcp/pcac147
- Share Icon Share
Abstract
The global increase in nanotechnology applications has been unprecedented and has now moved into the area of agriculture and food production. Applications with promising potential in sustainable agriculture include nanobiosensors, nanofertilizers, nanopesticides, nano-mediated remediation strategies for contaminated soils and nanoscale strategies to increase crop production and protection. Given this, the impact of nanomaterials/nanoparticles (NPs) on plant species needs to be thoroughly evaluated as this represents a critical interface between the biosphere and the environment. Importantly, phytohormones represent a critical class of biomolecules to plant health and productivity; however, the impact of NPs on these molecules is poorly understood. In addition, phytohormones, and associated pathways, are widely explored in agriculture to influence several biological processes for the improvement of plant growth and productivity under natural as well as stressed conditions. However, the impact of exogenous applications of phytohormones on NP-treated plants has not been explored. The importance of hormone signaling and cross-talk with other metabolic systems makes these biomolecules ideal candidates for a thorough assessment of NP impacts on plant species. This article presents a critical evaluation of the existing yet limited literature available on NP–phytohormone interactions in plants. In addition, the developing strategy of nano-enabled precision delivery of phytohormones via nanocarriers will be explored. Finally, directions for future research and critical knowledge gaps will be identified for this important aspect of nano-enabled agriculture.
Introduction
The colonization of land by plants created significant evolutionary pressure for the development of phytohormone signaling pathways, along with other regulatory networks, to support the adaptation to and survival under the significantly more uncertain and variable environmental conditions of terrestrial ecosystems (Lu and Xu 2015, Berens et al. 2017, Bowman et al. 2019). Phytohormones, also referred to as plant hormones, are active organic chemicals produced by intracellular metabolism and modulate an exceptionally broad range of biological activities (Fahad et al. 2015, Verma et al. 2016, Saini et al. 2021). These important biomolecules regulate physiological responses during plant growth, and their total content and overall activity also dictate the ability of plants to acclimatize to environmental challenges (Verma et al. 2016, Checker et al. 2018, Yu et al. 2020, Zhao et al. 2021). The phytohormone signaling pathways are quite intricate (Fahad et al. 2015, Blázquez et al. 2020) and include important molecules, such as auxins [e.g. indole-3 acetic acid (IAA)], cytokinin (CK), gibberellin (GA), abscisic acid (ABA), salicylic acid (SA), brassinosteriods (BRs), jasmonic acid (JA) and ethylene. All of these hormones have been reported to enable successful plant response to environmental stress and to effectively maintain plant growth under a broad range of conditions (Per et al. 2018, Chen et al. 2020, Emamverdian et al. 2020, Sharma et al. 2020, Chen et al. 2021, Jamil et al. 2021, Li et al. 2021a, b, Singh et al. 2021).
Research seeking to develop nano-enabled techniques to sustainably improve crop yield and increase stress tolerance in plants has increased significantly in recent years (Li and Yan 2020; Wu and Li 2021, Wang et al. 2022). Notably, the increased use of nanotechnological applications has continued and will continue to increase the release of engineered nanomaterials in the environment (Rastogi et al. 2017). As plants play critical roles in terrestrial ecosystems, this growing environmental release of nanoparticles (NPs) necessitates a significant and thorough assessment of the impact of these materials on plant species (Yang et al. 2017). Plants have continuous interactions with air, soil and water that may contain NPs and therefore may serve as entry points into human and ecological food chains (Li and Yan 2020). Furthermore, it is very important to recognize that these interactions will be highly dynamic and specific, potentially varying significantly with nanomaterial properties and plant species (Usman et al. 2020). There is a developing and fairly robust literature evaluating the ability of NPs to modulate different processes in plants (Tripathi et al. 2016, Khan and Upadhyaya 2019, Freitas et al. 2021, Hu and Xianyu 2021, Rizwan et al. 2021, Zhang et al. 2022). The binary nature of exposure of plants to a range of NPs is well reported for end points such as seed germination and seedling growth, as well as physiological, biochemical and molecular processes (Goswami and Mathur 2019, Agrahari and Dubey 2020, Landa 2021), with the difference between NP-induced toxicity and benefit often being a matter of dose, although clearly factors such as plant species and exposure scenario are important.
Importantly and as noted previously, the interaction between NPs and phytohormones remains a topic in need of significantly more research. Given the important role of phytohormones in plant growth and stress management, it is important to understand the interaction of NPs with these important biomolecules, as well as the associated impacts on related biosynthetic pathways and signal transduction reactions. This review evaluates the available literature in this context and also discusses the impacts of exogenous supplementation of plant hormones to regulate the influence of NPs on plants. This evaluation will outline future work to improve NP-mediated beneficial impacts on plants, as well as mitigate the toxicity associated with certain NPs, and will advance efforts for sustainable agriculture for global food security.
NP-Induced Modulation of Hormone Contents in Plants
Despite being the critical regulators of plant growth and development, phytohormones still present a relatively unexplored area in plant–NP interaction studies (Sonkar et al. 2021), although some recent work has been published. Pociecha et al. (2021) reported that NPs can promote plant growth by modulating phytohormone levels. The authors showed that exposure of wheat (Triticum aestivum) plants to 10 mg l−1 silver NPs (AgNPs) accelerated the transition between vegetative and reproductive development by inducing changes in the content and activity of GAs and CKs. Mechanistically, the AgNP-treated wheat plants showed better growth and earlier flowering due to reduced cis-zeatin riboside (a less active CK type) and increased GA6 (important for flowering) content in comparison with controls (Pociecha et al. 2021). Vinković et al. (2017) conducted a hormone analysis of pepper (Capsicum annuum) plants treated with AgNPs (0.01–1 mg l−1) using ultra-high performance liquid chromatography–electrospray tandem mass spectrometry and demonstrated that total CKs in the leaves were significantly increased upon AgNP treatment, indicating that CKs (especially cis-zeatin type—a CK associated with stress responses) increase in AgNP-exposed plants and promoted growth (Vinković et al. 2017). Diploid watermelon (Citrullus lanatus) seedlings grown from iron (Fe) oxide NP (Fe-NPs)–primed seeds at 160 mg l−1 exhibited no toxic impact on their growth and showed a significant increase (approximately 70 µM g−1 fresh weight) in the JA content compared with unprimed control, suggesting that Fe-NPs at non-toxic concentrations can be employed to prime or induce JA-linked defense responses in watermelon seedlings (Kasote et al. 2019).
Importantly, separate from the growth impacts, NPs can mediate stress responses in plants by induction or suppression of stress-related hormones. NPs can also influence phytohormone levels in a fashion similar to that induced by mechanical stresses. Angelini et al. (2022) reported increases in the levels of derivatives of three major stress phytohormones JA, ABA and SA in Arabidopsis thaliana plants treated with 50 mg l−1 of AgNP and that this response resembled the physiological response of the plant to mechanical stress. Importantly, nanomaterials can have variable impacts on different phytohormones in the same plant. For instance, Hao et al. (2016) exposed rice (Oryza sativa) to hollow multiwalled carbon nanotubes (CNTs), Fe–Cobalt (Co)-filled CNTs and Fe-filled CNTs at 10, 50 and 300 mg l−1. The authors reported that this exposure decreased the concentrations of IAA, GA1, GA3 and isopentenyl adenosine (a type of CK). These CNT treatments also decreased the biomass of rice roots and shoots, root length and shoot height at higher doses, suggesting that CNTs inhibit plant growth through downregulation of these growth-promoting hormones. In addition, decreases in JA, BR and ABA contents were observed, likely resulting in an attenuation of plant tolerance to CNT-induced stress (Hao et al. 2016). It is important to highlight that the change in phytohormone content upon NP exposure can also be tissue-specific. Vankova et al. (2017) reported changes in the hormone pools of A. thaliana in response to zinc oxide NP (ZnO NP, 0.16–100 mg l−1) exposure. The authors evaluated the content of CKs, auxins, ABA, SA and JA in different plant tissues, including apices, leaves and roots (Vankova et al. 2017). The most rapid response to ZnO NPs was observed in apices, which was evident from downregulated growth and decreased CK and auxin content. However, cis-zeatin accumulated gradually in the roots, which may enhance stress tolerance (Vankova et al. 2017). ZnO NP–induced substantial phenotypic changes in a dose-dependent manner, for instance, reductions in root length and shoot growth. The upregulation of ABA in the apices and leaves and increases in the other stress hormone SA in the leaves and roots suggest that A. thaliana plants sense ZnO NP as a severe stress. Conversely, Vankova et al. reported that JA was suppressed in apices, leaves and roots at all concentrations (0.16–100 mg l−1) of ZnO NPs, except 0.16 mg l−1 ZnO NP–treated leaves, where upregulation in the JA content was observed (Vankova et al. 2017). Collectively, this brief survey of the existing literature presents significant evidence that NP exposure to plants can result in rapid changes in phytohormone levels that can in turn trigger a broad range of plant growth and stress responses. Besides phytohormonal regulation, NP treatment of plants also modulates the levels of reactive oxygen species (ROS) in a dose-dependent manner (Wu et al. 2017, Wang et al. 2020, Qi et al. 2021). Both phytohormones and ROS act as signaling molecules for the regulation of various cellular processes in plants (Singh et al. 2016, 2019, Foyer 2018, Roychoudhury and Aftab 2021); therefore, understanding their relationship in plants during NP exposure can help in delineating the regulatory mechanisms involved in NP-induced responses in plants.
Effects of Hormones on NP-Induced Impacts on Plants
There have been several recent studies in plant nanotechnology that report on the influence of exogenously supplied phytohormones on NP-induced impacts on plant species (Iftikhar et al. 2019, Alharby et al. 2021, Fig. 1). This hormone–NP interaction can be synergistic and promote overall growth. For example, Sharma et al. (2022) supplied IAA (1 µM) exogenously in combination with silicon NPs (SiNPs, 10 µM) to rice seedlings that were stressed by hexavalent chromium (100 μM) exposure. The authors found that IAA enhanced the protective effects of SiNPs against metal stress. More specifically, IAA activated both tolerance and defense pathways, leading to increases in biomass, as well as the content of photosynthetic pigments, endogenous nitric oxide and antioxidants (Sharma et al. 2022).
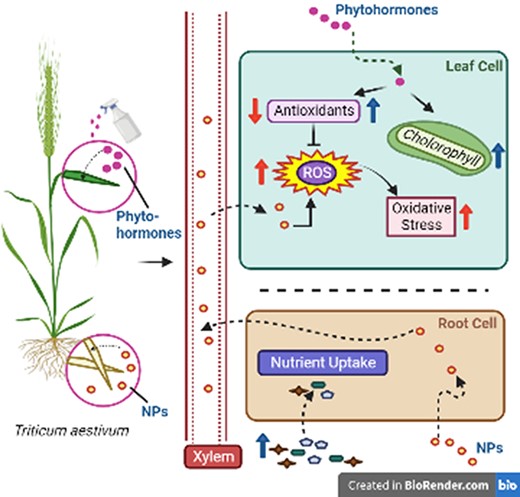
Proposed mechanism of NP-induced toxicity mitigation in plants (here, T. aestivum) by exogenously supplied phytohormones. Exposure of plants to toxic NPs through soil application and subsequent translocation through the plant tissues via xylem, leading to reduced growth. The mechanisms of toxicity include excess ROS generation and associated oxidative damage, along with downregulation of antioxidant activities. Foliar application of phytohormones improves plant growth by enhancing chlorophyll content, nutrient uptake and antioxidant activities, which in turn scavenge the NP-induced excess ROS and subsequently reduce oxidative stress in the plant. Figure created with BioRender.com.
There have also been reports suggesting antagonistic interactions between hormones and NPs. This is generally observed with phytotoxic NPs that result in a decrease in plant growth parameters through stress response induction in exposed plants. The application of phytohormones has been shown to improve growth parameters, including biomass, nutrient uptake and chlorophyll content of the plants exposed to toxic NPs, as well as to reduce the oxidative damage induced by NPs. Iftikhar et al. (2019) reported that GA (0, 100 and 200 mg l−1) amendment reduced the toxicity of ZnO NPs (0–1,200 mg kg−1), particularly at high doses, in wheat. Specifically, the addition of GA increased plant biomass, photosynthesis, nutrient content and antioxidant activities to scavenge ZnO NP–induced ROS while decreasing Zn accumulation. These results collectively demonstrate that GA very effectively reduced ZnO NP–induced toxicity in wheat (Iftikhar et al. 2019). Separately, Iftikhar et al. (2020) demonstrated that foliar application of GA (0, 100 and 200 mg l−1) to wheat mitigated the toxicity induced by soil exposure of plants to cerium oxide NP (0–600 mg kg−1). This benefit was demonstrated by the increases in chlorophyll and nutrient content, as well as the overall growth and yield upon GA addition (Iftikhar et al. 2020). GA also alleviated the NP-induced oxidative stress in wheat by promoting overall intracellular antioxidant enzyme activity (Iftikhar et al. 2020).
Similarly, Alharby et al. (2021) demonstrated that in wheat plants exposed to titanium dioxide NPs (0–600 mg kg−1), foliar application of GA (0, 100 and 200 mg l−1) improved a number of plant growth parameters, such as nutrient uptake and chlorophyll content. Yadav et al. (2021) showed that in copper oxide NP (CuO NP)–stressed wheat seedlings, IAA supplementation repressed the elevating effect of 2,3,5-triiodobenzoic acid (TIBA, a polar auxin transport inhibitor) on CuO NP toxicity. More specifically, the added IAA assisted calcium-mediated alleviation of CuO NP stress and prevented TIBA from increasing CuO NP toxicity (Yadav et al. 2021). Although the literature on NP–phytohormone interactions is limited, the aforementioned analysis suggests significant benefits from externally applied phytohormones in plants under significant NP-induced stresses.
Hormone–NP Interaction in Genetically Modified and Conventional Crops
Most of the available literature related to phytohormone–NP interactions in plants is confined to conventional non-transgenic crop species. The exposure of genetically modified (GM) crops to NPs is expected to increase in the near future given the potential synergistic benefits of genetic engineering and nanotechnology. Therefore, studies assessing phytohormone–NP interactions in GM crops are needed. Along those lines, Gui et al. (2015) conducted a glasshouse study on transgenic 93–11 LRK1 (a high-production transgenic rice cultivar with LEUCINE-RICH REPEAT SERINE/THREONINE-PROTEIN KINASE 1 gene) and its parent non-transgenic rice (93–11) plants exposed to iron oxide NPs (Fe2O3 NPs, 0, 2, 20 and 200 mg kg−1). Along with measuring Fe2O3 NP uptake, nutrient concentration, plant biomass and enzymatic activities, the authors also evaluated the concentrations of ABA and IAA. Reductions in the levels of ABA and IAA were reported in the roots of transgenic and non-transgenic rice and were positively correlated with NP concentrations, suggesting that Fe2O3 NPs negatively affect ABA and IAA contents in both types of rice plants (Gui et al. 2015). However, the levels of IAA and ABA were higher in the roots of non-transgenic than transgenic rice plants treated with 200 mg kg−1 Fe2O3 NPs, suggesting that GM and conventional rice plants responded differently to Fe2O3 NP exposure. Le Van et al. (2016) assessed the impact of CuO NPs (0, 10, 200 and 1,000 mg l−1) on growth, nutrient content and hormone levels (IAA, ABA and GA) in two genotypes of cotton (Gossypium sp.), namely, Bacillus thuringiensis (Bt)-transgenic (Bt-29317, with CRY transgene derived from bacterium Bacillus thuringiensis and codes for Bt-toxin, an insecticidal protein that protects cotton plants from bollworms) and conventional cotton (Jihe 321). The leaf and root contents of IAA, ABA and GA were differentially impacted with respect to NP doses in the transgenic and non-transgenic cotton (Le Van et al. 2016). Van Nhan et al. conducted a similar study with Bt-transgenic and non-transgenic cotton exposed to 0, 100 or 1,000 mg l−1 Fe2O3 NPs (Van Nhan et al. 2016). The authors also reported significant differences in the levels of IAA, ABA and GA across the two genotypes upon NP exposure. Fe2O3 NPs of 100 mg l−1 concentration increased the IAA level in leaves of both plant types, while no change was observed in the case of 1,000 mg l−1 Fe2O3 NP. Both Fe2O3 NPs treatments decreased the ABA content in conventional cotton but did not influence ABA levels significantly in the leaves of Bt-transgenic cotton. GA content was reduced in the conventional cotton leaves for both Fe2O3 NP treatments, whereas their levels were significantly increased in the leaves of Bt-transgenic cotton (Van Nhan et al. 2016). These findings do suggest that GM plants might respond differently to NPs at a hormonal level. As such, future work is needed to explore the implications for sustainable nano-enabled agriculture.
Nano-Enabled Delivery of Phytohormones to Plants
One recently developing area of nanotechnology is the use of nanoscale carrier platforms to encapsulate agrochemicals and biomolecules for precise and targeted active ingredient delivery for sustainable agriculture (Pereira et al. 2019). Such advanced material formulations, often denoted as nanofertilizers and nanopesticides, have shown controllable release and efficient targeted delivery of agrochemicals in plants (Chhipa 2017, Mendez et al. 2022, Xu et al. 2022). NPs of various constituents can be employed for biomolecule delivery, including cargo like microRNA, RNA interference and clustered regularly interspaced short palindromic repeats- CRISPR associated protein (CRISPR-Cas) in the plant tissues for improving the plant traits (Farooq et al. 2021, Mujtaba et al. 2021, Shidore et al. 2021). However, the number of studies investigating nanoscale delivery systems developed for phytohormones is very limited. Pereira et al. (2017a) described a nanocarrier system for GA3 that was based on poly-γ-glutamic acid (γ-PGA) and chitosan (CS) polymers. These encapsulated NPs (nano-γ-PGA/CS-GA3) were used with Phaseolus vulgaris seeds, and the results showed a 50–70% increase in germination rate in the first 24 h compared with free GA3 (10–16%) (Pereira et al. 2017a). The authors also reported a greater efficiency of encapsulated GA3 than the free hormone at increasing the leaf area and inducing root development, including lateral root formation. Importantly, these effects were not observed in seeds treated with the nano-γ-PGA/CS without GA3. Overall, their results indicate the significant potential of nano-γ-PGA/CS-GA3 in agriculture (Pereira et al. 2017a). In another study, Pereira et al. (2017b) developed nanocarriers composed of alginate/CS (ALG/CS) for GA3 and conducted bioactivity assays on P. vulgaris. The authors reported that ALG/CS-GA3 NPs increased the leaf area, as well as the chlorophyll and carotenoid contents. In a separate study, Pereira et al. (2019) evaluated the impact of seed priming with nano-ALG/CS containing GA3 on Solanum lycopersicum plants cultivated under field conditions. The authors reported that nano-ALG/CS-GA3 could enhance plant growth and fruit production (by nearly 4-fold) (Fig. 2). Korpayev et al. demonstrated the efficacy of CS and Ag as biopolymeric and metallic nanocarriers for promoting adventitious rooting potential of microcuttings in Malling Merton 106 apple rootstocks (Korpayev et al. 2021). Specifically, both Ag and CS NPs loaded with the auxins IAA and indole-butyric acid (IBA) were highly effective at promoting adventitious rooting of apple microcutting rootstocks compared with free IAA or IBA applications. However, IBA-loaded CS nanocarriers induced an undesirable development of large callus structures (Korpayev et al. 2021). Thangavelu et al. (2018) synthesized AgNPs using two kinds of rooting auxins, IAA and IBA, as reducing stabilizing agents. The authors demonstrated that in comparison with controls, these hormone-stabilized AgNPs improved root growth by 3-fold and enhanced resistance against root growth-inhibiting phytopathogens in the model plants Nicotiana tabacum and Hibiscus rosa-sinensis. These hormone-stabilized AgNPs interactions exerted no phytotoxicity as confirmed by analysis of random amplified polymorphic DNA molecular markers (Thangavelu et al. 2018). These findings highlight the significant potential of nanocarriers for phytohormone delivery to crop species.
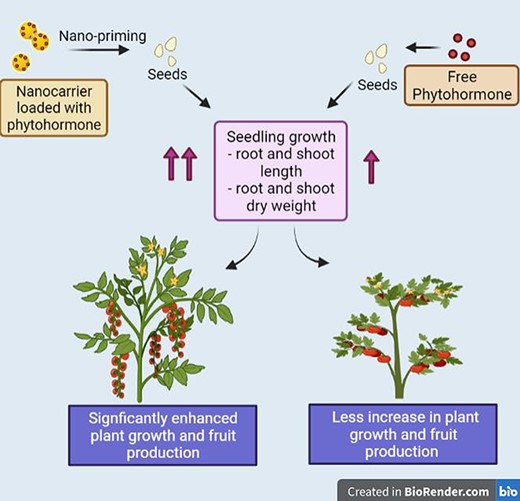
Comparative analysis of the impacts of free phytohormones and phytohormones loaded in nanoscale carriers on the growth and development of plants. Seed priming with a phytohormone-loaded nanocarrier leads to enhanced seedling growth compared with seeds treated with free phytohormone as measured by length and dry weights of roots and shoots. The overall growth of the plant is more significantly increased with the phytohormone-loaded nanocarriers, leading to a greater leaf area and fruit production. Figure drawn based on results explained by Pereira et al. (2019) and created with BioRender.com.
Concluding Remarks
Nanotechnology has the potential to revolutionize the agriculture sector with the wide-ranging applications that can improve crop health, growth and productivity, thereby simultaneously enabling us to combat food insecurity. However, the effects of NPs, both positive and negative ones, cannot be generalized as species, exposure time and/or dose dependence, and exposure scenario details impact their overall impacts. There has been significant research investigating the morphological, physiological, biochemical and molecular responses of plants to NP exposure. But as noted previously, there are only limited studies investigating the interactions of NPs and hormones in plants and their effect on plant growth and performance under environmentally relevant conditions. Given the critical role of growth-promoting and defense-related roles of phytohormones, obtaining a thorough understanding of NP–phytohormone interactions is highly recommended. In addition, cross-talk of phytohormones with other signaling cascades in plants during the NP interaction requires further research. Modern-day molecular techniques can be employed to discover the molecular basis of such complex relationships. The research in this area holds immense potential to provide effective ways for improving plant productivity and stress tolerance.
In addition, the following are some major points that need to be delineated through future research regarding this important research topic:
Assess the effects of growth conditions and NP exposure duration on phytohormone–NP interactions in plants.
Comparative evaluation of efficacy of NP-encapsulated phytohormones relative to free hormones in different plant species.
NP-induced modulation of phytohormone signaling and cross-talk with other signaling molecules.
Molecular mechanisms involved in the NP–phytohormone interaction for positive growth responses in plants.
Data Availability
No new data were generated or analyzed in this study.
Acknowledgements
D.K.T. is highly thankful to the Department of Science and Technology, Science and Engineering Research Board, New Delhi, for providing the research grant (SRG/2020/002309). D.K.T. and N.K. are also thankful to Amity University Uttar Pradesh for providing the research facilities.
Author Contributions
D.K.T. has conceptualized the review idea, and N.K. and D.K.T. have written the first draft of the manuscript. D.K.T., V.P.S., J.C.W. and L.-S.T.P. have critically edited the manuscript.
Disclosures
The authors have no conflicts of interest to declare.