-
PDF
- Split View
-
Views
-
Cite
Cite
Kai Shu, Xiaofeng Luo, Yongjie Meng, Wenyu Yang, Toward a Molecular Understanding of Abscisic Acid Actions in Floral Transition, Plant and Cell Physiology, Volume 59, Issue 2, February 2018, Pages 215–221, https://doi.org/10.1093/pcp/pcy007
- Share Icon Share
Abstract
The transition from the vegetative growth phase to flowering is a crucial checkpoint for plant reproduction and survival, especially under environmental stress conditions. Numerous factors regulate flowering time, including exogenous environmental cues such as day length and temperature, as well as salt and drought stresses, and endogenous phytohormone signaling cascades. Gibberellins and ABA are one classic combination of phytohormones which antagonistically regulate several biological processes, including seed dormancy and germination, primary root growth and seedling development. As regards control of flowering time, gibberellin exhibits a positive role, and represents an important pathway in the regulation of floral transition. However, over the past decades, numerous investigations have demonstrated that the contribution of the stress hormone ABA to floral transition is still controversial, as both positive and negative effects have been documented. It is important to determine why and how ABA shows this contradictory effect on flowering time. In this up to date review, primarily based on recent publications and emerging data, we summarize the distinct and contrasting roles of ABA on floral transition, while the detailed molecular mechanisms underlying these roles are discussed. Finally, the remaining challenges and open questions in this topic are presented.
Introduction
Flowering time is one of the most important plant traits, environmentally appropriate floral transition being central to both maximum seed yield and quality in crop plants (Hill and Li 2016, Okada et al. 2017) and to adaptation to stress conditions in wild plants (Park et al. 2016, Cho et al. 2017, Shu and Yang 2017). Research over the past decades has shown that floral transition and its timing are regulated by intricate networks involving exogenous environmental cues and endogenous phytohormone-based signals (Liu et al. 2013, Romera-Branchat et al. 2014, Conti 2017).
Of the phytohormone signaling pathways, gibberellins promote flowering and control an important pathway in the regulation of floral transition; these mechanisms have been extensively investigated and documented (Ding et al. 2013, Hyun et al. 2016, Zhu et al. 2016, Brambilla et al. 2017, Conti 2017, Gong et al. 2017, Sawettalake et al. 2017). ABA is another important phytohormone, which principally induces seed and bud dormancy, delays seed germination, represses seedling growth and enhances plant abiotic stress tolerance (Cutler et al. 2010, Sah et al. 2016, Shu et al. 2016c, Nonogaki 2017, Vishwakarma et al. 2017). ABA and gibberellin antagonistically regulate several biological processes, including flowering time control (Shu et al. 2018). However, earlier studies also showed that ABA is involved in the control of flowering time (El-Antably and Wareing 1966, Takeno and Maeda 1996, El-Antably et al. 1967), and more research in this area has emerged recently (Riboni et al. 2013, Wang et al. 2013, Riboni et al. 2016, Shu et al. 2016b, Conti 2017). In addition, it is noted that both positive and negative effects of ABA on floral transition have been reported (Takeno and Maeda 1996, Riboni et al. 2013, Wang et al. 2013, Riboni et al. 2016, Shu et al. 2016b). Consequently, key questions to be answered are how and why ABA shows such contradictory effects on flowering time and, in particular, what the underlying mechanisms are.
The regulation of flowering time is a heavily researched topic in the field of plant biology, and the literature in this area has been reviewed frequently in recent years, covering aspects such as the effect of nitrogen availability on flowering control (Lin and Tsay 2017), epigenetic regulation of the floral transition (Yaish et al. 2011, Campos-Rivero et al. 2017, Wang and Kohler 2017), transport regulation of florigen (Liu et al. 2013), the effect of environmental factors on the regulation of flowering (Cho et al. 2017), abiotic stress and flowering (Park et al. 2016, Takeno 2016), and the relationship between ambient temperature and floral transition (Jagadish et al. 2016, McClung et al. 2016). However, an overview primarily focused on the detailed mechanisms of ABA involved in floral transition has not been published since 2014 (Conti et al. 2014); given the fast pace of scientific progress in this exciting field, numerous new results have emerged since the publication of the previous review.
This updated review is based on the discovery of the ABA signaling cascade, with an emphasis on recent findings on the distinct roles (both positive and negative effects) of ABA in floral transition, and the underlying mechanisms, identifying questions which remain unanswered and pinpointing future directions for research in this field.
Discovery of ABA and its Signaling Cascade
In the 1950s, Van Steveninck first identified an unknown chemical from yellow lupin which induced the abscission of flowers and reproductive organs (Van Steveninck 1958, Van Steveninck 1959). Several years later, during an investigation of factors causing the abscission of the cotton boll, Liu and Carnsdagger (1961) isolated and purified this chemical, and named it abscisin. Soon after, Addicott and colleagues further purified chemicals from the abscission site of the cotton boll, and renamed the chemical purified in the laboratory of Liu and Carnsdagger as abscisin I (Ohkuma et al. 1963), and designated a second factor abscisin II.
At around the same time, the Wareing laboratory obtained a growth-inhibitory extract from mature birch leaves which was able to induce dormancy in growth buds (Eagles and Wareing 1963, Eagles and Wareing 1964). The Wareing lab designated this extract as dormin, as it appeared to act as an endogenous dormancy inducer (Eagles and Wareing 1963). In 1965, Cornforth et al. from the Wareing lab crystallized dormin, and confirmed that dormin and abscisin II were identical chemicals (Cornforth et al. 1965). In 1968, at the 6th Conference of the International Plant Growth Substances Association, the pioneering researchers Wareing and Addicott suggested the name abscisic acid (ABA) to replace dormin and abscisin II (Addicott et al. 1968).
Although ABA was discovered half a century ago, characterization of the ABA signaling transduction pathway was achieved only in recent years. Briefly, there are three groups of components, namely the PYRABACTIN RESISTANCE (PYR)/REGULATORY COMPONENT OF ABA RECEPTOR (RCAR), the PROTEIN PHOSPHATASE 2Cs (PP2Cs) and SNF1-RELATED PROTEIN KINASE 2s (SnRK2s), involved in the ABA signaling pathway (Cutler et al. 2010, Vishwakarma et al. 2017). ABA is first recognized by the receptor proteins PYR/PYL/RCARs, and this physical interaction further triggers the interaction between receptors and PP2Cs, subsequently releasing the protein kinase activity of SnRK2s (Cutler et al. 2010, Yoshida et al. 2015, Yang et al. 2017), with the PP2Cs and SnRK2s acting as negative and positive regulators, respectively (Yoshida et al. 2015). Finally, SnRK2s activate expression of a series of bZIP transcription factors, including ABI5 (ABSCISIC ACID-INSENSITIVE 5) (Yu et al. 2015), to co-ordinate ABA responses. Transcription factor ABI4 is another key positive mediator in ABA signaling (Shu et al. 2013, Shu et al. 2016c), and both ABI4 and ABI5 are involved in plant floral transition control (Wang et al. 2013, Shu et al. 2016b).
Negative Effect of ABA on Flowering Time Control: Focusing on FLC and FT
Numerous studies have demonstrated that ABA exhibits an inhibitory effect on diverse plant growth and development stages, including seed germination (Shu et al. 2013, Shu et al. 2016c), seedling development (Shu et al. 2016a), plant male sterility and sexual reproduction (Shu et al. 2014, McAdam et al. 2016), and root elongation (Luo et al. 2014). Consistently, ABA has also been considered to be a flowering suppressor, and several reports have reported the negative effect of ABA on floral transition (Wang et al. 2013, Shu et al. 2016b).
Spraying plants with exogenous ABA significantly delays Arabidopsis thaliana floral transition (Wang et al. 2013) and, in line with this, several loss-of-function mutants of the positive regulators in the ABA signaling pathway, abi3, abi4 and abi5, showed the early flowering phenotype, while transgenic plants overexpressing those genes (ABI3, ABI4 and ABI5) showed delayed floral transition (Kurup et al. 2000, Zhang et al. 2005, Shu et al. 2016b, Foyer et al. 2012, Wang et al. 2013). Recently, the detailed mechanisms underlying the effects of ABI4 and ABI5 on floral transition were uncovered (Wang et al. 2013, Shu et al. 2016b). Interestingly, the transcription factors ABI4 and ABI5 negatively mediate flowering by directly promoting the transcription of the same target gene, FLC (FLOWERING LOCUS C), which is a key repressor of flowering initiation (Wang et al. 2013, Shu et al. 2016b). Thus, it is noted that FLC is the target for both ABI4 and ABI5. Given that ABI4 could also directly promote ABI5 transcription (Bossi et al. 2009), it is proposed that the induction effect of ABI4 on FLC expression may be dependent on ABI5. In a word, these key transcription factors, ABIs, regulate floral transition though the central mediator FLC in Arabidopsis (Fig. 1). Given that ABI4 negatively controls gibberellin biogenesis, resulting in the ABI4–gibberellin flowering pathway being proposed (Shu et al. 2016b), similarly the ABI5–gibberellin flowering and ABI3–FLC–FLOWERING LOCUST (FT) flowering cascades are valuable hypotheses to test (Fig. 1).
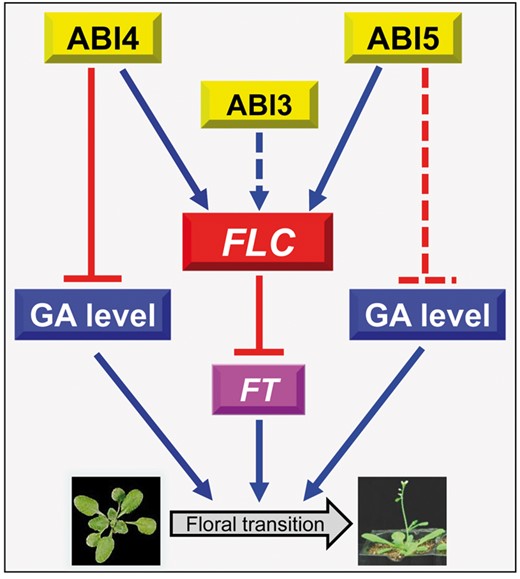
The proposed working network by which ABIs regulate floral transition though the central mediator FLC in Arabidopsis. ABI4 and ABI5 negatively regulate floral transition by directly mediating FLC transcription, while FLC further represses expression of the florigen gene FT in Arabidopsis. In addition, recent research (Shu et al. 2016b) also showed that ABI4 negatively controls gibberellin biogenesis, resulting in the ABI4– gibberellin flowering pathway being proposed. Similarly, the ABI5–gibberellin flowering and ABI3–FLC–FT flowering cascades are valuable hypotheses to test. The solid lines represent the confirmed pathway, and the dashed lines are proposed from current investigations and need further exploration. Blue lines ending with arrows denote positive regulation, while red lines ending with bars denote negative regulation.
In addition to the three key transcription factors, ABI3, ABI4 and ABI5, several other components involved in the ABA signaling transduction pathway are also involved in the regulation of flowering. Overexpression of MYB37, which encodes a R2R3 MYB transcription factor, conferred an ABA-hypersensitive phenotype, and showed the expected delayed floral transition (Yu et al. 2016). An AP2 domain-containing transcription factor, ERF96, positively regulated ABA signaling, while transgenic plants overexpressing ERF96 also exhibited hypersensitivity to ABA and delayed flowering phenotypes (Wang et al. 2015). Similar to ERF96, two more AP2 domain-containing transcription factors AtRAV1 (RELATED_TO_ABA-INSENSITIVE3/VIVIPAROUS1) and AtRAV2, involved in ABA signaling, also negatively regulated floral transition in transgenic cotton (Mittal et al. 2015). Gene expression analysis showed that transcription of the GhFT and GhFT-Like (FTL) genes in cotton was repressed in transgenic cotton plants overexpressing AtRAV1 or AtRAV2 (Mittal et al. 2015). In rice, ABA induced the expression of OsABF1 (ABA responsive element binding factor 1), which encodes a bZIP transcription factor, acting as a suppressor of the flowering initiation processes (Zhang et al. 2016). OsABF1 indirectly suppresses transcription of the Ehd1 (Early heading date 1) gene, which encodes a key activator of rice flowering (Zhang et al. 2016). Taken together, the transcription factors mentioned here enhance ABA signaling, and negatively impact upon floral transition by directly or indirectly affecting expression of floral initiation genes, including FT and FTL, suggesting an important negative role for ABA in regulation of the floral transition.
Positive Effect of ABA on Floral Transition: Focusing on FT and GIGANTEA
Although the negative effects of ABA on flowering date had been widely documented, there were still some reports that ABA promoted floral transition, especially under environmental stress conditions, such as drought (Riboni et al. 2013, Riboni et al. 2016). As early as the 1960s, soon after the discovery of ABA, it was reported that ABA induced or inhibited flowering initiation, with the effect of ABA on flowering being species and photoperiod dependent (El-Antably and Wareing 1966, El-Antably et al. 1967, Nakayama and Hashimoto 1973, Shinozaki and Takimoto 1983). In addition, some key genes involved in the ABA signaling cascade also showed the contradictory effects on floral transition. For example, although the abi3 mutant exhibited the early-flowering phenotype (Kurup et al. 2000), intriguingly it was reported that the physical interaction between ABI3 and the CONSTANS (CO) transcription factor might result in an increase in CO activity, and further promote florigen FT transcription (Tiwari et al. 2010). The mechanisms underlying both the positive and negative roles of ABI3 warrant further exploration.
The relationship between diverse abiotic stress responses and ABA and flowering time control is also mediated by microRNAs (miRNAs). In soybean (Glycine max L.), miRNA172c enhances ABA signaling through directly mediating cleavage of the mRNA of its target genes; overexpression of miRNA172c resulted in ABA hypersensitivity but exhibited the early-flowering phenotype, especially under drought stress conditions (Li et al. 2016). Interestingly, in Arabidopsis, it is also demonstrated that the elevated ABA level caused by drought further induces miRNA172 transcription and processing, and subsequently miRNA172 could suppress the levels of its targets including WRKY44 and TOE1, and finally promotes flowering and plant drought response (Han et al. 2013). A recent study also revealed that, in rice, numerous drought-responsive miRNAs during the heading stage were identified under drought conditions through next-generation sequencing assay, some of which were involved in flowering time control (Cheah et al. 2017). Similar to this, in apple, some miRNAs were also identified which were potentially involved in the regulation of flowering induction and stress responses (Xing et al. 2016). Consequently, those pioneering investigations provided the foundation and clues for subsequent studies, and further studies are needed to dissect the precise mechanisms underlying the effect of those miRNAs on floral transition under abiotic stresses.
Numerous studies showed that epigenetic modifications are also involved in the floral transition process (Yaish et al. 2011, Campos-Rivero et al. 2017, Wang and Kohler 2017), but only a few pieces of direct evidence about ABA and flowering and epigenetic regulation are available. An earlier investigation revealed that Arabidopsis ABO4, a negative regulator in the ABA signaling pathway, changed the histone modification in FLC and FT and finally negatively mediated flowering time (Yin et al. 2009). Consequently, the abo4-1 mutant showed the early-flowering phenotype (Yin et al. 2009). Moreover, a recent study identified several genetic loci and their association with functional mechanisms in 240 accessions of subtropical maize under water stress by genome-wide association mapping. It is also found that some genes are involved in the ABA signaling pathway by which they regulate floral transition by epigenetic changes in the stress-responsive genes (Thirunavukkarasu et al. 2014). However, the more detailed and direct mechanisms underlying the effect of epigenetic regulation on flowering mediated by the ABA cascade require further investigations.
It is noted that drought stress significantly damages plant growth, including both vegetative and reproductive phases, so that drought stress causes early flowering in various plant species, and this flowering response is also known as the drought escape (DE) response (Conti et al. 2014, Riboni et al. 2014). ABA biogenesis is up-regulated under drought stress (Budak et al. 2015, Munemasa et al. 2015), and the level of endogenous ABA may be a signal for flowering control and stress response. Finally, the relationship between drought-induced early floral initiation and the ABA level needs further dissection.
Recently, the molecular mechanisms underlying the ABA-mediated DE response were analyzed. Riboni and colleagues identified the central roles of the flower-promoting gene GI (GIGANTEA) and the florigen genes FT and TSF (TWIN SISTER OF FT) in the DE response, in which ABA response is key (Riboni et al. 2013). Interestingly, this effect is largely dependent on photoperiod, because the early-flowering phenotype does not only occur under long-day conditions, in which GI and SOC1 (SUPPRESSOR OF OVEREXPRESSION OF CO 1) are key components (Riboni et al. 2013). More recently, these authors further dissected the relationship between ABA and photoperiod pathways. It was found that ABA modulates GI signaling and activity, and thus activates transcription of the florigen genes (Riboni et al. 2016). ABA induces the DE response by promoting FT expression, for which CO is required; inhibiting ABA signaling reduces FT expression markedly (Riboni et al. 2016).
In citrus species, water deficit promotes flowering, and the higher level of ABA was also detected during the floral inductive stage (Li et al. 2017). Furthermore, in litchi species, drought stress combined with low temperature remarkably promoted flowering; the transcriptome analysis showed that expression of a large number of genes was changed (Shen et al. 2016). However, the detailed relationship between ABA biosynthesis and/or signaling and flowering in litchi needs further investigation (Shen et al. 2016). Actually, those are similar to the DE response in Arabidopsis which promotes flowering under drought conditions (Riboni et al. 2013, Riboni et al. 2016). Taken together, all these studies demonstrated that variations in ABA levels and signaling direct plant development to achieve maximum plant survival under stress conditions such as drought.
Concluding Remarks and Open Questions: Why and How Does ABA Exhibit Contradictory Effects on Flowering?
As a well-known stress phytohormone, ABA is involved both in flowering time control and in response to diverse abiotic stresses such as drought. Numerous studies have also independently revealed the contradictory effects of ABA on floral transition. Although rapid progress has been achieved through forward and reverse genetic dissection of ABA signaling and its effects on flowering time, etc., primarily in the model plants Arabidopsis and rice, some key questions on this subject still remain.
The positive and negative roles of ABA in plant floral transition are puzzling phenomena, and key questions include why these opposite effects of ABA arise, and how they are achieved. Here, we attempt to address these questions from a new viewpoint, based on recent research progress. Interestingly, we found that ABA negatively impacts on flowering time when the increase in ABA resulted from the overexpression of some key genes such as ABI4 and ABI5 (Wang et al. 2013, Shu et al. 2016b), while the positive effect of ABA on flowering time was detected when the increased content of ABA was a response to drought (Riboni et al. 2013, Riboni et al. 2014). Interestingly, the downstream regulators in both circumstances, including FLC and FT, were the same (Riboni et al. 2013, Wang et al. 2013, Riboni et al. 2014, Shu et al. 2016b). Thus, it is proposed that plants can distinguish the origin of ABA increases between endogenous (e.g. caused by genetic change) and exogenous causes (e.g. drought) (Fig. 2). Further, we speculated that plants also can distinguish the level of drought conditions and maximally escape from this stress by regulating the balance between vegetative and reproducible growth. For this purpose, plant might have evolved one or more currently unidentified sensors to achieve these ends (Fig. 2). For example, under gentle and moderate levels of drought stress, sensor X is activated; while with long-term drought (serious), sensor Y is activated. The physiological effect of activation of X and Y is distinct with regard to the transition from vegetative to reproductive growth. It is believed that the identification and characterization of the unknown signals or sensors will deepen and widen our understanding of the mechanisms underlying the effects of ABA on plant floral transition.
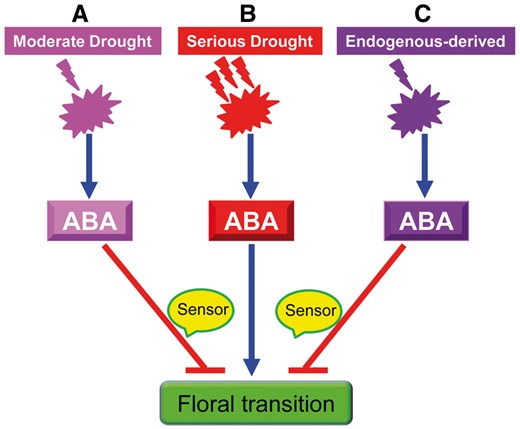
A proposed model in which the plant distinguishes the origin of ABA, in order to mediate the appropriate date of floral transition precisely. A moderate level of drought delays floral transition in order to escape the ongoing stress conditions, allowing for flowering to occur after the stress has abated (A), while a serious drought stress, causing very marked accumulation of ABA, promotes flowering and drought escape to maximize reproductive success (B). Endogenous ABA accumulation (e.g. caused by a transgene) also delayed floral transition (C). Finally, the ability with which a plant senses the environmental stress level (e.g. drought) is important for its survival. Moderate and serious drought stress-caused accumulation of ABA is highlighted in pink and red, respectively. Endogenous-derived ABA accumulation is marked with a purple background, and the predicted sensor is highlighted in yellow. Blue lines ending with arrows denote positive regulation, while red lines ending with bars denote negative regulation.
Similar to the contradictory effects of ABA on floral transition, drought stress can also trigger both early flowering (Riboni et al. 2013, Riboni et al. 2014, Riboni et al. 2016) and late flowering (Bocco et al. 2012, Galbiati et al. 2016). How can this apparent dichotomy be explained? Our proposal is that a moderate level of drought stress may delay floral transition in order to escape the ongoing stress conditions, and subsequently to delay flowering until suitable ambient conditions prevail (Fig. 2A), while more severe drought stress promotes floral transition to maximize reproductive success (Fig. 2B). Subsequently, the ability with which plants precisely sense the prevailing environmental stress conditions is important for their survival.
Last, but not least, it is noted that previous studies had revealed that NTL8, a member of the plant-specific transcription factor family NAC (NAM, ATAF1/2, CUC2), mediates the salt-responsive flowering date via FT in Arabidopsis, and FT expression was significantly repressed by high salt (Kim and Park 2007, Kim et al. 2007). Further studies showed that BFT (BROTHER OF FT AND TFL1) acts as a floral repressor under salt stress conditions (Ryu et al. 2011), with BFT delaying flowering under salt stress conditions by competing with FT for binding to the FD transcription factor (Ryu et al. 2014). Although it is suggested that salinity might negatively regulate flowering date, the mechanism by which salt stress affects the floral transition is still largely unknown, such as whether NTL8 directly binds to the FT promoter region. Given that salt stress also induces accumulation of ABA (Sah et al. 2016), questions raised include whether salt-regulated floral transition is mediated by ABA and whether there are also contradictory effects on flowering date under low and high stress conditions?
Funding
The work in our lab was supported by the Natural Science Foundation of China [31701064]; National Key Research and Development Program of China [2017YFD0201306]; and a China Postdoctoral Science Foundation funded project [2014M552377 and 2016T90868].
Acknowledgments
We would like to thank Dr. Qi Xie (Institute of Genetics and Developmental Biology, Chinese Academy of Sciences, Beijing) for critical reading and comments during the preparation of this manuscript. In addition, we also apologize to the colleagues who have contributed to this field but were not cited because of space limitations.
Disclosures
The authors have no conflicts of interest to declare.
References
Abbreviations
- ABI
ABSCISIC ACID-INSENSITIVE
- AtRAV
RELATED_TO_ABA-INSENSITIVE3/VIVIPAROUS1
- BFT
BROTHER OF FT AND TFL1
- CO
CONSTANS
- DE
drought escape
- Ehd1
Early heading date 1
- FLC
FLOWERING LOCUS C
- FLT
FLOWERING LOCUS T
- GI
GIGANTEA
- OsABF1
rice ABA responsive element-binding factor 1
- miRNA
microRNA
- PP2C
PROTEIN PHOSPHATASE 2C
- PYR
PYRABACTIN RESISTANCE
- RCAR
REGULATORY COMPONENT OF ABA RECEPTOR
- SnRK2
SNF1-RELATED PROTEIN KINASE 2