-
PDF
- Split View
-
Views
-
Cite
Cite
Zhenjun Li, Rihe Peng, Yongsheng Tian, Hongjuan Han, Jing Xu, Quanhong Yao, Genome-Wide Identification and Analysis of the MYB Transcription Factor Superfamily in Solanum lycopersicum, Plant and Cell Physiology, Volume 57, Issue 8, August 2016, Pages 1657–1677, https://doi.org/10.1093/pcp/pcw091
- Share Icon Share
Abstract
MYB proteins constitute one of the largest transcription factor families in the plant kingdom, members of which perform a variety of functions in plant biological processes. However, there are only very limited reports on the characterization of MYB transcription factors in tomato (Solanum lycopersicum). In our study, a total of 127 MYB genes have been identified in the tomato genome. A complete overview of these MYB genes is presented, including the phylogeny, gene structures, protein motifs, chromosome locations and expression patterns. The 127 SlMYB proteins could be classified into 18 subgroups based on domain similarity and phylogenetic topology. Phylogenetic analysis of SlMYBs along with MYBs from Arabidopsis (Arabidopsis thaliana) and rice (Oryza sativa) indicated 14 subfamilies. Conserved motifs outside the MYB domain may reflect their functional conservation. The identified tomato MYB genes were distributed on 12 chromosomes at various densities but mainly in chromosomes 6 and 10 (12.6% and 11.8%, respectively). Genome-wide segmental and tandem duplications were also found, which may contribute to the expansion of SlMYB genes. RNA-sequencing and microarray data revealed tissue-specific and stress-responsive expression patterns of SlMYB genes. The expression profiles of SlMYB genes in response to salicylic acid (SA) and jasmonic acid methyl ester (MeJA) were also investigated by real-time PCR. Moreover, ethylene-responsive element-binding factor-associated amphiphilic repression (EAR) motifs were found in 24 SlMYB proteins. Collectively, our comprehensive analysis of SlMYB genes will facilitate future functional studies of the tomato MYB gene family and probably other Solanaceae plants.
Introduction
Transcription factors (TFs) are essential for the regulation of gene expression and are, as a consequence, found in all living organisms. The number of TFs found within an organism increases generally with genome size, and larger genomes tend to have more TFs (Latchman 1997). In Arabidopsis and rice genomes, approximately 5.9% and 4% of the estimated total number of genes code for TFs, respectively (Riechmann et al. 2000, Goff et al. 2002, Pérez-Rodríguez et al. 2009). As for tomato, approximately 5.3% of the 34,727 protein-coding loci have been identified to encode 1,845 putative TFs (Tomato Genome Consortium 2012). A TF usually contains two different functional domains involved in DNA binding and transcriptional activation and/or repression (Riechmann et al. 2000). These two domains together with other signature motifs function to activate and/or repress transcription in response to endogenous and exogenous stimuli, and finally mediate many physiological and biochemical processes (Riechmann et al. 2000, Amoutzias et al. 2007).
TFs can be grouped into many different families based on the domains that specifically bind to DNA sequences in the regulatory regions of downstream target genes. In plants, one example of such domains is the MYB domain. The MYB superfamily has the largest number of members of any Arabidopsis gene family (Riechmann and Ratcliffe 2000). The MYB TF family is not plant specific and is present in all eukaryotes. The ‘oncogene’ v-MYB was the first MYB gene identified in avian myeloblastosis virus (Klempnauer et al. 1982). Three v-MYB-related genes namely c-MYB, A-MYB and B-MYB, were subsequently found in many vertebrates and were proved to participate in the regulation of cell differentiation, proliferation and apoptosis (Weston 1998). Homologous genes were also identified in other organisms such as insects, fungi and slime molds (Lipsick 1996). Zea mays C1, a homolog of the mammalian c-MYB gene and involved in regulation of anthocyanin biosynthesis, was the first MYB gene to be functionally characterized in plants (Paz-Ares et al. 1987). Moreover, there are a larger number of MYB genes in plants as compared with fungi and animals (Martin and Paz-Ares 1997, Kranz et al. 2000, Riechmann et al. 2000, Qu and Zhu 2006, Yanhui et al. 2006). Extensive functions of MYB genes have been reviewed in previous studies (Du et al. 2009, Dubos et al. 2010, Ambawat et al. 2013).
MYB proteins contain a MYB DNA-binding domain (DBD), which is approximately 50–53 amino acid residues in length, and forms a helix–turn–helix (HTH) fold with three regularly spaced tryptophan residues (Kanei-Ishii et al. 1990). The three-dimensional structure of the MYB domain revealed that the DNA recognition site α-helix interacts with the major groove of DNA during transcription (Ogata et al. 1994). However, amino acid sequences outside the conserved MYB domain are highly divergent in length and sequence. Depending on the number of adjacent MYB repeats, MYB TFs have been classified into four major groups, namely 1R-MYB, 2R-MYB, 3R-MYB and 4R-MYB, containing one, two, three and four MYB repeats, respectively (Dubos et al. 2010). 1R-MYBs were referred to as MYB-related proteins (Du et al. 2013). In animals, R1R2R3-type MYB domain proteins are predominant, while in plants, the R2R3-type MYB domain proteins are more prevalent (Paz-Ares et al. 1987, Lipsick 1996, Dubos et al. 2010). The plant R2R3-MYB genes probably evolved from an R1R2R3-MYB gene ancestor by the loss of an R1 repeat or from an R1-MYB gene through duplication of an R1 repeat (Rosinski and Atchley 1998, Jiang et al. 2004a). In addition, 2R-, 3R- and 4R-MYBs were taken as typical MYB proteins and comprehensively analyzed in our research.
An increasing number of plant MYB TF members have been identified and characterized in numerous plant species based on the highly conserved DBDs in Arabidopsis, Populus trichocarpa, Cucumis sativus, maize, apple and soybean (Yanhui et al. 2006, Wilkins et al. 2009, Du et al. 2012a, Du et al. 2012b, Li et al. 2012, Cao et al. 2013). Several MYB genes are involved in regulating responses to environmental stresses such as drought, salt and cold. A cotton MYB TF, GbMYB5, confers drought tolerance in cotton and transgenic tobacco plants (Chen et al. 2015). Arabidopsis AtMYB96, an R2R3-type MYB TF, regulates drought stress response by integrating ABA and auxin signals (Seo et al. 2009). Plants overexpressing OsMYB91 showed accumulation of endogenous ABA under control conditions and enhanced tolerance to salt stress (Zhu et al. 2015a). Transgenic rice overexpressing OsMYB3R-2 exhibited enhanced cold tolerance as well as increased cell mitotic index (Ma et al. 2009). A tremendous amount of data is available on the roles of MYB TFs in enhancing the resistance of plants to biotic stresses. The OsLTR1 gene from rice was found to regulate jasmonic acid (JA)-dependent defense whereas AtMYB15, AtMYB34, AtMYB51 and AtMYB75 were found to be associated with the mechanical wound response or resistance against insect herbivores (Cheong et al. 2002, Johnson and Dowd 2004). It has been shown that AtMYB102 contributes to basal resistance against the insect herbivore Pieris rapae (De Vos et al. 2006). Similarly, AtMYB44 has been reported to play a part in the plant defense response against aphids (Liu et al. 2010). Other functions of MYBs include control of cellular morphogenesis, regulation of secondary metabolism, secondary cell wall biosynthesis, meristem formation and cell cycle regulation (Ito et al. 2001, Araki et al. 2004, Dubos et al. 2010, Kobayashi et al. 2015, Sun et al. 2015). A rice R2R3-type MYB gene (OsCSA), whose expression could be promoted by brassinosteroids, directly triggers expression of sugar partitioning and metabolic genes during pollen and seed development (Zhu et al. 2015b). Transgenic tobacco plants overexpressing SbMYB2, SbMYB7 or SbMYB8 from Scutellaria baicalensis showed elevated phenylpropanoid content, enhanced expression of flavonoid biosynthesis-related genes and oxidative stress tolerance (Qi et al. 2015, Yuan et al. 2015). In contrast, the soybean GmMYB100 and grape VvMYB4-like gene negatively regulate plant flavonoid biosynthesis (Yan et al. 2015, Pérez-Díaz et al. 2016). PhMYB1, AmMYBML2 and AtMYB16 are able to induce changes in the epidermal cell shape (Baumann et al. 2007). The rice and maize MYB TFs, OsMYB46 and ZmMYB46, were reported to be able to activate the entire secondary wall biosynthetic program in Arabidopsis (Zhong et al. 2011).
Tomato, originating in South America, has spread around the world to become one of the most extensively used vegetable crops. Besides its economic value, it has interesting developmental features, such as compound leaves, fleshy fruits and sympodial shoot branching (Schmitz and Theres 1999, Manning et al. 2006, Townsley and Sinha 2012). Moreover, it has simple diploid genetics, a short generation time and routine transformation technology, and is easy to maintain. Together, these make tomato an ideal species for both basic and applied biological research (Ranjan et al. 2012).
Although quite a few MYB TFs have been functionally characterized in the model plant Arabidopsis and others, the roles of most members of the plant MYB family remain unknown. Especially in tomato, the typical commercial and model species, there are only very limited reports on the functional characterization of tomato MYB TFs (Lin et al. 1996, Schmitz et al. 2002, Mathews et al. 2003, AbuQamar et al. 2009, Ballester et al. 2010). The release of the whole tomato genomic sequence in 2012 contributed significantly to subsequent studies. Based on the published sequence data, comprehensive analysis of the tomato MYB gene family can be carried out to uncover its functions, evolution and expression profiles (Tomato Genome Consortium 2012).
In the present study, a genome-wide identification of MYB domain TFs in tomato was performed and revealed an expanded MYB family with 127 members, most of which remain to be functionally characterized. Phylogenetic analyses of 127 tomato MYB proteins were performed and, by analysis of the conserved motifs, we provide additional evidence for the subgroup definition. In addition, all the tomato MYB proteins were subjected to a phylogenetic analysis with their Arabidopsis and rice counterparts to identify gene orthologs and clusters of orthologous groups, enabling functional prediction. Chromosomal distribution analysis revealed that some of the duplication events are likely to have contributed to the expansion of this family. Real-time PCR was employed to investigate the responses of all tomato MYB genes to treatments with the plant hormone salicylic acid (SA) and methyl jasmonate (MeJA). We also performed a comprehensive expression analysis of all tomato MYB genes in various tissues and under different biotic and abiotic stresses using the wealth of available expression data, which were generated by RNA-sequencing (RNA-seq) and microarray. These data, in turn, provided important complementary information to assist in the elucidation of the functions of the tomato MYB genes. Moreover, full-length sequences of 127 tomato MYB proteins were used to search iteratively for EAR (ethylene-responsive element-binding factor-associated amphiphilic repression) motif-containing candidates. The EAR motif might be critical in determining whether a MYB protein will act as an activator or a repressor (Kagale and Rozwadowski 2011).
Taken together, this study provided a useful resource for future studies on the structure and function of tomato MYB proteins in the regulation of tomato growth and development. The results also provided an approach for identifying and characterizing MYB TFs in other species.
Results
Identification and conserved DBD analysis of MYB TFs in tomato
With the aim of defining the tomato MYB gene family, we searched the entire tomato genome sequence for genes that encode proteins containing the MYB DBD. Consensus-typical R2R3-MYB DBD sequences were used as queries for BLASTP searches. Approximately 160 protein sequences that might contain MYB repeats were identified. Initially, based on the identification numbers and chromosome locations, any redundant sequences were removed from the data set. Meanwhile, sequences including a single MYB domain (MYB-related) or somewhat different conserved domains were discarded from the data list. We also performed SMART (Simple Modular Architecture Research Tool) analysis to confirm the reliability of the candidates. Currently, 140 members of tomato MYB genes are presented in the Plant Transcription Factor Database (PlantTFDB; http://planttfdb.cbi.pku.edu.cn) (Jin et al. 2014). However, based on SMART analysis, several members do not possess a typical R2R3-MYB domain such as Solyc04g049740.1.1 and Solyc04g050090.1.1. Therefore, they were provionally excluded from our study. Finally, a primary data set of 122 typical R2R3-MYB proteins (2R-MYB), four R1R2R3-MYB proteins (3R-MYB) and one 4R-like MYB protein (4R-MYB) was successfully identified in tomato (Solanum lycopersicum MYB, SlMYB). The nucleotide and protein sequences of the SlMYB gene family are listed in Supplementary Table S1. In order to better reflect the appropriate orthologous relationship between SlMYBs and AtMYBs, we named each SlMYB based on their phylogenies and sequence similarity corresponding to individual AtMYBs. To achieve this, an unrooted phylogenetic tree, constructed depending on the full-length sequences of MYB proteins from tomato and Arabidopsis, was employed to identify possible orthologs between the two species, supported by bootstrap values (1,000 re-sampling) (Supplementary Fig. S1). Among the 127 SlMYBs, SlMYB4R-1 is the longest protein, with 1,70 amino acids, while the shortest is SlMYB11, with 125 amino acids
To investigate the homologous domain sequence features, and the frequency of the most prevalent amino acids at each position within each repeat of the 122 tomato R2R3-MYB domains, we performed WebLogo and multiple alignment (ClustalX 2.1) analysis using the amino acid sequences of R2 and R3 repeats (Fig. 1; Supplementary Fig. S2). The results showed that they all included highly conserved triplet tryptophan (Trp, W) residues in each repeat, and the characteristic W residues were located at positions 9, 29 and 49 of the R2 repeat and 62, 81 and 100 of the R3 repeat in tomato (Fig. 1). In the R3 repeat, the first tryptophan (Trp62) residue was generally replaced by phenylalanine or isoleucine (Phe, F or Ile, I). However, the second and the third tryptophan residues were highly conserved. In R2, a total of 24 (of 54) positions are occupied by a single residues in >70% of the proteins. For R3, the equivalent number is 27 (out of 54), indicating that R3 is slightly more conserved than R2. In general, the basic regions of MYB domains had 108 basic residues (including the linker region), with rare deletions or insertions (Supplementary Fig. S2). It was revealed that the linker region between R2 and R3 repeats was highly conserved, and that four amino acids in the first half of the linker (LNPE) formed a highly conserved motif (Hegvold and Gabrielsen 1996, van Aalten et al. 1998). It is noteworthy that a similar motif exists in the linker region in tomato MYB proteins as well (LRPD, Leu53 to Asn56 in Fig. 1). In addition, the evolution of the plant-specific R2R3-MYB domain involved the insertion of a leucine residue (L) between the second and third helices of the R2 repeat (Williams and Grotewold 1997). In the present study, we observed that up to 107 of the 122 R2R3-type SlMYBs (about 88%) also had a leucine (Leu38) insertion at the same site (Fig. 1; Supplementary Fig. S2). As shown in Fig. 1, the major conserved residues in the MYB domain were mainly distributed between the second and third conserved tryptophan residues in both repeats. Thus, the first part of each repeat in the MYB domain was apparently less conserved, and the third helix of the HTH domain in each repeat was the most conserved region among the 122 SlMYBs.

Consensus sequence and the level of conservation of R2R3-type MYB domains from tomato. The sequence logos of the R2 and R3 MYB repeats are based on full-length alignments of all tomato R2R3-MYB domains. Multiple alignment analysis of 122 tomato typical R2R3-MYB domains was performed with ClustalX 2.1 (for full representation of the alignment, see Supplementary Fig. S2). The overall height of each stack indicates the conservation of the sequence at that position and the bit score indicates the relative frequency of the corresponding acid. The numbers below the amino acid residues (which are represented by the single-letter amino acid code) indicate the percentage of times the particular residue appears at the respective position. The conserved tryptophan residues (Trp, W) in the MYB domain are marked with black asterisks. The replaced residues in the R3 repeat are shown by blue asterisks.
Phylogenetic relationships, gene structures, motif composition and tissue-specific expression of MYB genes in tomato
To examine the phylogenetic relationships among the MYB domain proteins in tomato, an unrooted tree was constructed from alignments of the full-length amino acid sequences of all SlMYB proteins (Fig. 2A). The observed sequence similarity and phylogenetic tree topology allowed us to classify the tomato MYB gene family into 18 subgroups (subgroups 1–18). Each subgroup had 2–18 members and the high bootstrap value in each subgroup suggested a common origin for the MYB genes in each subgroup. Careful inspection of the phylogenetic tree topology revealed several pairs of MYB proteins with a high degree of homology in the terminal nodes of each subgroup, suggesting that they are putative paralogous pairs (Fig. 2A).
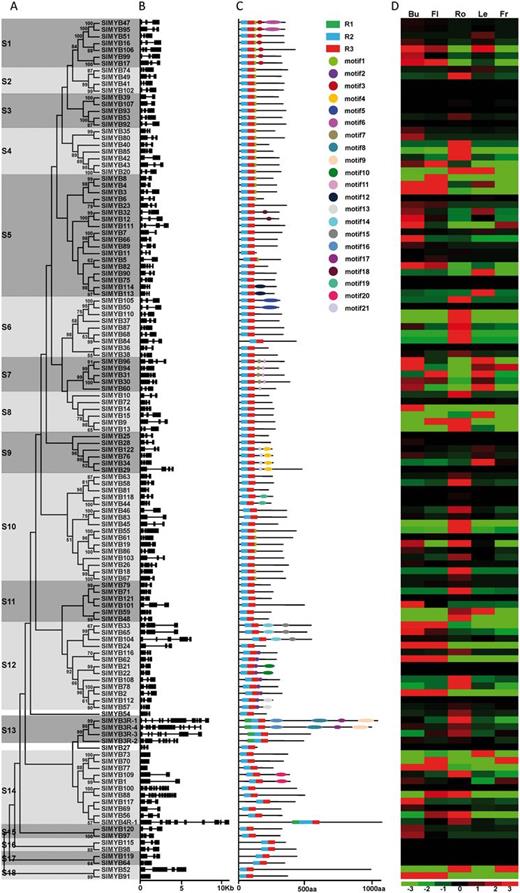
Phylogenetic relationships, gene structures, motif composition and tissue-specific expression of MYB genes in tomato. (A) Phylogenetic analysis of SlMYBs. The amino acid sequences of 127 SlMYB proteins were aligned using ClustalX 2.1, and the phylogenetic tree was constructed using MEGA 6.05 by the Neighbor–Joining (NJ) method with 1,000 bootstrap replicates. Bootstrap values >50 are indicated on the nodes. Different subgroups were marked with alternating tones of a gray background to make subgroup identification easier. (B) Exon/intron structures of the SlMYB genes. Black boxes represent exons and spaces between the black boxes correspond to introns. The sizes of exons and introns can be estimated using the horizontal lines. (C) Schematic representation of conserved motifs (obtained using MEME) in SlMYB proteins. Different motifs are represented by circles of different colors. Details of the individual motifs are shown in Supplementary Table S2. The black lines represent the non-conserved sequences. The three colored boxes represent the R1, R2 and R3 MYB repeat, respectively. The sizes of motifs can be estimated using the scale below. (D) Tissue-specific expression patterns of SlMYB genes. The RNA-seq data were generated from the TFGD database. The expression data were gene-wise normalized. The relative expression level of a particular gene in each row was normalized against the mean value. The color scale below represents expression values, green indicating low levels and red indicating high levels of transcript abundance. The sources of the samples were as follows: Bu (bud), Fl (flower), Ro (root), Le (leaf) and Fr (fruit).
It is well known that gene structural diversity might be an explanation for the evolution of multigene families. In order to gain further insights into the structural diversity of SlMYB genes, we analyzed the exon/intron organization in the coding sequence of each SlMYB gene (Fig. 2B). According to their predicted structures, four of the 127 SlMYB genes have no introns (SlMYB70, SlMYB73, SlMYB77 and SlMYB91). The most closely related members in the same subgroup generally showed the same exon/intron pattern in terms of intron number and position or exon length (Fig. 2B). For instance, pairs of SlMYB genes (SlMYB114/113, SlMYB33/65 and SlMYB21/22) showed very similar exon/intron patterns. In contrast, the gene structure appeared to be quite different in subgroup 14, which had a number of exon/intron structural variants with striking distinctions.
To reveal the diversification of MYB genes in tomato, the program MEME (Multiple Em for Motif Elicitation, version 4.11.0) was used to study further the diversification of these proteins. Twenty-one conserved motifs were identified (Fig. 2C; Table 1). The logos of the 21 motifs are presented in Supplementary Table S2. Most of the closely related members have a common motif composition, suggesting possible functional similarity among these SlMYB proteins (Fig. 2C). As expected, most of the closely related members in the phylogenetic tree had common motif compositions. For example, there were no conserved motifs outside the MYB domain in subgroups 8, 11 and 15–18, while motif 2 appeared in most members of subgroup 12. In other subgroups, motif 3 was specific to subgroup 1, and motif 11 was specific to subgroup 7 (Fig. 2C). These similarities in motif patterns might be related to similar functions of the MYB proteins within the same subgroup.
Motif . | Width . | Sites . | Multilevel consensus sequence . | e-value . |
---|---|---|---|---|
1 | 15 | 34 | [IL]KMGIDP[VN]TH[KR]P[LR]SD | 2.5e-182 |
2 | 29 | 9 | [KR]QL[KH]C[DE][VIS]NS[KQ]QF[KQR][DEH][TF] [LIM][RKL]YLW[IM]P[RS]L[LV][EA][RKQ][IQ][QEI] | 2.3e-98 |
3 | 41 | 7 | [SV]A[NS][LA][SR]HMA[QE]WESARLEAEARL[VS]R[EK]S[KQ]IL [FV][NP][NS][NS][NV][NG][NRS][HNS]N[NTY][ND][IY][NF] | 1.5e-81 |
4 | 57 | 4 | DYQQDIFQEELANLE[ED]ITSGSFWTQPFEVDTKIDFVAP [SP]IDYCGL[VI]CPPSPF[IM][PS]HEF | 1.5e-65 |
5 | 119 | 2 | Y[IV]EPIPLVQPNF[MT]YNNNNMMNFQL GTNNQ[HY]SYNFHDQSL[MV][NY]PMQ[AT]ISSCSSSDGLSC [KN]QISYGNEE[IM]MCQIPFEETQKFTLD [NS]YCTTWADHQK[AT]NGYFGNNFQSSQ[FL]QYDDHTNIEEIK | 3.2e-47 |
6 | 120 | 2 | YYQQLPCLD[IR]LKAWQ[IM][AT]STKLPTINDISHAILR [NS]N[LS]KNKKLDSSI[PQ]SS[ST]LNSSENIFA[KN][DN]APTTTK [FV]D[DV]DD[DH]QNL[HQ]NLSTINSCF EDDQLLQTELPSFMQEFSGVFPEY[AT]QNSTNGLQVD | 1.8e-32 |
7 | 26 | 4 | [KR]GQWER[RT]LQ[AT]DI[HN][MT]A[KR][KQ]AL[CHQ][NDE]ALSL[DE] | 5.2e-30 |
8 | 120 | 2 | GALCY[DE]PPRFPSLD[TV]PF[FL][SY]CDL[IK]QSG[ST]DTQQE[FY] SPLGIRQLM[MT]TS[TV][DN]C[LS]TP[FL]RLWDSP[KL]R[AD] [DG]SPDA[IV]L[KR]SAAKTFT[GS]TPSILKKR[HQ]R [DH]LV[ST]PLSEKR[CW][EG]KKLESDL[NR] [KR]ESFSN[LM][AV][KT][DE]F[PS]R | 1.3e-27 |
9 | 120 | 2 | TP[FY]K[IR]SIESPSAWKSPW[FI][FT][DT]P[FL]LSSPR[LY][DE] [KN]ELT[FY]E[DE][FL]A[FL][FL][LM]SPGDRSYDAIGLMKQLSEQTA [GP][AS][FI]ADA[QR][EQ][IV]LG[GS][DE]TPETIL[LR]GRNSK[EN] QKADEN[HR][ST]LL[AS][AS]NAMSERRTLDFSECG[ST]PGKG | 3.8e-23 |
10 | 79 | 2 | Y[LP]N[TV]NIISSTDSF[LV][KR]EDDSNIW[KN][KN]LS SFEDGINI[PS][HR]DFSTPS[DY]M[KT]LHNYSSH[DH][EV] DDQYYFHNYWTSAEMPSLYEMD[HL]WL | 1.2e-22 |
11 | 17 | 5 | YASS[AT]ENI[AS][RK]LL[QEK][GNQ]W[MIT][KRG] | 3.7e-18 |
12 | 82 | 2 | TKIVPREK[FI][KN][NS]K[CR]GEISTKIEIIKPQ[AR]RK[FY][FI]S [NS]T[EM]KN[IV]TNN[IN]VI[LV]D[EK]EE[EH] CKEIISEKQT[PR]DAS[IM]DN[GV]D[EP]WW[AI]NLLENCNDD | 1.7e-16 |
13 | 64 | 2 | TSVTLENSSVATSSENSNQDYNQVNQSDQLWYEDYQAM [DE]QQNNIELWMDNEDVFNNLCN[IN]EDIW | 3.5e-19 |
14 | 78 | 3 | [NM]N[FVY]EIP[AT][VA]E[FN][KR][KNR][LF]E[FLP][NST] [QH][HLQ]L[YC]PP[AQR]L[LA][DN][IN][PD][AFT][GRS][SG] [FIL]L[DGN][DIT]P[AGV][RST][SN][LF][LR][ADS]QG[LH][NR][SA] [APS][YW][NSY][ST][HRT][SY][FV][LP]ST[TV][HPY][PS] [AS]KR[IT][RQ]G[SL]ES[LVW]FS[GN][LRS]N[GV]D[LC] | 8.8e-14 |
15 | 51 | 3 | [LV]PS[LI][DE]S[VS][DS][TI][LF]IQSPP[ANT][EG][HY][TS][ED]S[CG] [SN]LSP[QRS][NS]S[GD]LL[DH]A[VI]L[HY][EG]SQT[MP]KAS[KNS][SD][NI][SL][YH]Q | 2.4e-12 |
16 | 93 | 2 | [KM]L[HQ]Q[ST]SED[DE]SV[HR]KEG[AT]E[LM]EEV [MS]ECSQGS[NT]LAGC[PS]QSTSD[LM]GNTF[AV]H[KT]RE[EN]G [GK][LM][LS][EM][DE][IS][NV][RY][ER]KD[PT]S[AS]S[AS]APC[PS] [ET]YYTPAFED[IV][GT][CF]S[MV][EQ]EVPSEL[AV] | 3.5e-11 |
17 | 57 | 2 | G[AT]N[AD]A[IM]GK[IV]KQP[PS]GVLVEL[NS][AT][NS]D[LM] [FL]FSPDRF[FG][AT][KN][AH]DRAT[NS]LS[IN]KALGNQYARR[IL]EAA | 5.9e-10 |
18 | 38 | 2 | FDH[IN]DDQMLWHDDI[MV][LP]MD[AD][EN][DY][AE][KV]LDQDFI[FT][KN]CLW[DS]DQG | 9.1e-10 |
19 | 55 | 2 | TNCPNIQS[FL]DFTNSSSSSSYI[IT]FDENYDFLET[SY]NQ[EH]NDVTSIVNQVDDENIVILE | 1.1e-9 |
20 | 82 | 2 | LI[HP]T[FQ][AS]PD[FL][DG]I[CT]KLLEG[AV][FS] [GT][DE][RT][IL]VP[HQ][GQ]CGHGCC[AG][AN][TV][NS][KQ] [ES][IS][NS][GK]SSLLGPEFVDY[AE]E[HP]P[PS][FL][LS]SHELA [AS][LM]AT[DE][IL][NS]N[IV]AW[IY]KSGLE | 3.2e-9 |
21 | 21 | 4 | N[NI]N[NS][NT]MHEN[IV][VI]LE[SN]S[ED]WS[PS][NKS]E | 5.2e-9 |
Motif . | Width . | Sites . | Multilevel consensus sequence . | e-value . |
---|---|---|---|---|
1 | 15 | 34 | [IL]KMGIDP[VN]TH[KR]P[LR]SD | 2.5e-182 |
2 | 29 | 9 | [KR]QL[KH]C[DE][VIS]NS[KQ]QF[KQR][DEH][TF] [LIM][RKL]YLW[IM]P[RS]L[LV][EA][RKQ][IQ][QEI] | 2.3e-98 |
3 | 41 | 7 | [SV]A[NS][LA][SR]HMA[QE]WESARLEAEARL[VS]R[EK]S[KQ]IL [FV][NP][NS][NS][NV][NG][NRS][HNS]N[NTY][ND][IY][NF] | 1.5e-81 |
4 | 57 | 4 | DYQQDIFQEELANLE[ED]ITSGSFWTQPFEVDTKIDFVAP [SP]IDYCGL[VI]CPPSPF[IM][PS]HEF | 1.5e-65 |
5 | 119 | 2 | Y[IV]EPIPLVQPNF[MT]YNNNNMMNFQL GTNNQ[HY]SYNFHDQSL[MV][NY]PMQ[AT]ISSCSSSDGLSC [KN]QISYGNEE[IM]MCQIPFEETQKFTLD [NS]YCTTWADHQK[AT]NGYFGNNFQSSQ[FL]QYDDHTNIEEIK | 3.2e-47 |
6 | 120 | 2 | YYQQLPCLD[IR]LKAWQ[IM][AT]STKLPTINDISHAILR [NS]N[LS]KNKKLDSSI[PQ]SS[ST]LNSSENIFA[KN][DN]APTTTK [FV]D[DV]DD[DH]QNL[HQ]NLSTINSCF EDDQLLQTELPSFMQEFSGVFPEY[AT]QNSTNGLQVD | 1.8e-32 |
7 | 26 | 4 | [KR]GQWER[RT]LQ[AT]DI[HN][MT]A[KR][KQ]AL[CHQ][NDE]ALSL[DE] | 5.2e-30 |
8 | 120 | 2 | GALCY[DE]PPRFPSLD[TV]PF[FL][SY]CDL[IK]QSG[ST]DTQQE[FY] SPLGIRQLM[MT]TS[TV][DN]C[LS]TP[FL]RLWDSP[KL]R[AD] [DG]SPDA[IV]L[KR]SAAKTFT[GS]TPSILKKR[HQ]R [DH]LV[ST]PLSEKR[CW][EG]KKLESDL[NR] [KR]ESFSN[LM][AV][KT][DE]F[PS]R | 1.3e-27 |
9 | 120 | 2 | TP[FY]K[IR]SIESPSAWKSPW[FI][FT][DT]P[FL]LSSPR[LY][DE] [KN]ELT[FY]E[DE][FL]A[FL][FL][LM]SPGDRSYDAIGLMKQLSEQTA [GP][AS][FI]ADA[QR][EQ][IV]LG[GS][DE]TPETIL[LR]GRNSK[EN] QKADEN[HR][ST]LL[AS][AS]NAMSERRTLDFSECG[ST]PGKG | 3.8e-23 |
10 | 79 | 2 | Y[LP]N[TV]NIISSTDSF[LV][KR]EDDSNIW[KN][KN]LS SFEDGINI[PS][HR]DFSTPS[DY]M[KT]LHNYSSH[DH][EV] DDQYYFHNYWTSAEMPSLYEMD[HL]WL | 1.2e-22 |
11 | 17 | 5 | YASS[AT]ENI[AS][RK]LL[QEK][GNQ]W[MIT][KRG] | 3.7e-18 |
12 | 82 | 2 | TKIVPREK[FI][KN][NS]K[CR]GEISTKIEIIKPQ[AR]RK[FY][FI]S [NS]T[EM]KN[IV]TNN[IN]VI[LV]D[EK]EE[EH] CKEIISEKQT[PR]DAS[IM]DN[GV]D[EP]WW[AI]NLLENCNDD | 1.7e-16 |
13 | 64 | 2 | TSVTLENSSVATSSENSNQDYNQVNQSDQLWYEDYQAM [DE]QQNNIELWMDNEDVFNNLCN[IN]EDIW | 3.5e-19 |
14 | 78 | 3 | [NM]N[FVY]EIP[AT][VA]E[FN][KR][KNR][LF]E[FLP][NST] [QH][HLQ]L[YC]PP[AQR]L[LA][DN][IN][PD][AFT][GRS][SG] [FIL]L[DGN][DIT]P[AGV][RST][SN][LF][LR][ADS]QG[LH][NR][SA] [APS][YW][NSY][ST][HRT][SY][FV][LP]ST[TV][HPY][PS] [AS]KR[IT][RQ]G[SL]ES[LVW]FS[GN][LRS]N[GV]D[LC] | 8.8e-14 |
15 | 51 | 3 | [LV]PS[LI][DE]S[VS][DS][TI][LF]IQSPP[ANT][EG][HY][TS][ED]S[CG] [SN]LSP[QRS][NS]S[GD]LL[DH]A[VI]L[HY][EG]SQT[MP]KAS[KNS][SD][NI][SL][YH]Q | 2.4e-12 |
16 | 93 | 2 | [KM]L[HQ]Q[ST]SED[DE]SV[HR]KEG[AT]E[LM]EEV [MS]ECSQGS[NT]LAGC[PS]QSTSD[LM]GNTF[AV]H[KT]RE[EN]G [GK][LM][LS][EM][DE][IS][NV][RY][ER]KD[PT]S[AS]S[AS]APC[PS] [ET]YYTPAFED[IV][GT][CF]S[MV][EQ]EVPSEL[AV] | 3.5e-11 |
17 | 57 | 2 | G[AT]N[AD]A[IM]GK[IV]KQP[PS]GVLVEL[NS][AT][NS]D[LM] [FL]FSPDRF[FG][AT][KN][AH]DRAT[NS]LS[IN]KALGNQYARR[IL]EAA | 5.9e-10 |
18 | 38 | 2 | FDH[IN]DDQMLWHDDI[MV][LP]MD[AD][EN][DY][AE][KV]LDQDFI[FT][KN]CLW[DS]DQG | 9.1e-10 |
19 | 55 | 2 | TNCPNIQS[FL]DFTNSSSSSSYI[IT]FDENYDFLET[SY]NQ[EH]NDVTSIVNQVDDENIVILE | 1.1e-9 |
20 | 82 | 2 | LI[HP]T[FQ][AS]PD[FL][DG]I[CT]KLLEG[AV][FS] [GT][DE][RT][IL]VP[HQ][GQ]CGHGCC[AG][AN][TV][NS][KQ] [ES][IS][NS][GK]SSLLGPEFVDY[AE]E[HP]P[PS][FL][LS]SHELA [AS][LM]AT[DE][IL][NS]N[IV]AW[IY]KSGLE | 3.2e-9 |
21 | 21 | 4 | N[NI]N[NS][NT]MHEN[IV][VI]LE[SN]S[ED]WS[PS][NKS]E | 5.2e-9 |
Consensus amino acid sequences were obtained from analysis of the 127 tomato MYB proteins with MEME software. The motif numbers are equivalent to those described in Fig. 2C and Supplementary Table S2.
Motif . | Width . | Sites . | Multilevel consensus sequence . | e-value . |
---|---|---|---|---|
1 | 15 | 34 | [IL]KMGIDP[VN]TH[KR]P[LR]SD | 2.5e-182 |
2 | 29 | 9 | [KR]QL[KH]C[DE][VIS]NS[KQ]QF[KQR][DEH][TF] [LIM][RKL]YLW[IM]P[RS]L[LV][EA][RKQ][IQ][QEI] | 2.3e-98 |
3 | 41 | 7 | [SV]A[NS][LA][SR]HMA[QE]WESARLEAEARL[VS]R[EK]S[KQ]IL [FV][NP][NS][NS][NV][NG][NRS][HNS]N[NTY][ND][IY][NF] | 1.5e-81 |
4 | 57 | 4 | DYQQDIFQEELANLE[ED]ITSGSFWTQPFEVDTKIDFVAP [SP]IDYCGL[VI]CPPSPF[IM][PS]HEF | 1.5e-65 |
5 | 119 | 2 | Y[IV]EPIPLVQPNF[MT]YNNNNMMNFQL GTNNQ[HY]SYNFHDQSL[MV][NY]PMQ[AT]ISSCSSSDGLSC [KN]QISYGNEE[IM]MCQIPFEETQKFTLD [NS]YCTTWADHQK[AT]NGYFGNNFQSSQ[FL]QYDDHTNIEEIK | 3.2e-47 |
6 | 120 | 2 | YYQQLPCLD[IR]LKAWQ[IM][AT]STKLPTINDISHAILR [NS]N[LS]KNKKLDSSI[PQ]SS[ST]LNSSENIFA[KN][DN]APTTTK [FV]D[DV]DD[DH]QNL[HQ]NLSTINSCF EDDQLLQTELPSFMQEFSGVFPEY[AT]QNSTNGLQVD | 1.8e-32 |
7 | 26 | 4 | [KR]GQWER[RT]LQ[AT]DI[HN][MT]A[KR][KQ]AL[CHQ][NDE]ALSL[DE] | 5.2e-30 |
8 | 120 | 2 | GALCY[DE]PPRFPSLD[TV]PF[FL][SY]CDL[IK]QSG[ST]DTQQE[FY] SPLGIRQLM[MT]TS[TV][DN]C[LS]TP[FL]RLWDSP[KL]R[AD] [DG]SPDA[IV]L[KR]SAAKTFT[GS]TPSILKKR[HQ]R [DH]LV[ST]PLSEKR[CW][EG]KKLESDL[NR] [KR]ESFSN[LM][AV][KT][DE]F[PS]R | 1.3e-27 |
9 | 120 | 2 | TP[FY]K[IR]SIESPSAWKSPW[FI][FT][DT]P[FL]LSSPR[LY][DE] [KN]ELT[FY]E[DE][FL]A[FL][FL][LM]SPGDRSYDAIGLMKQLSEQTA [GP][AS][FI]ADA[QR][EQ][IV]LG[GS][DE]TPETIL[LR]GRNSK[EN] QKADEN[HR][ST]LL[AS][AS]NAMSERRTLDFSECG[ST]PGKG | 3.8e-23 |
10 | 79 | 2 | Y[LP]N[TV]NIISSTDSF[LV][KR]EDDSNIW[KN][KN]LS SFEDGINI[PS][HR]DFSTPS[DY]M[KT]LHNYSSH[DH][EV] DDQYYFHNYWTSAEMPSLYEMD[HL]WL | 1.2e-22 |
11 | 17 | 5 | YASS[AT]ENI[AS][RK]LL[QEK][GNQ]W[MIT][KRG] | 3.7e-18 |
12 | 82 | 2 | TKIVPREK[FI][KN][NS]K[CR]GEISTKIEIIKPQ[AR]RK[FY][FI]S [NS]T[EM]KN[IV]TNN[IN]VI[LV]D[EK]EE[EH] CKEIISEKQT[PR]DAS[IM]DN[GV]D[EP]WW[AI]NLLENCNDD | 1.7e-16 |
13 | 64 | 2 | TSVTLENSSVATSSENSNQDYNQVNQSDQLWYEDYQAM [DE]QQNNIELWMDNEDVFNNLCN[IN]EDIW | 3.5e-19 |
14 | 78 | 3 | [NM]N[FVY]EIP[AT][VA]E[FN][KR][KNR][LF]E[FLP][NST] [QH][HLQ]L[YC]PP[AQR]L[LA][DN][IN][PD][AFT][GRS][SG] [FIL]L[DGN][DIT]P[AGV][RST][SN][LF][LR][ADS]QG[LH][NR][SA] [APS][YW][NSY][ST][HRT][SY][FV][LP]ST[TV][HPY][PS] [AS]KR[IT][RQ]G[SL]ES[LVW]FS[GN][LRS]N[GV]D[LC] | 8.8e-14 |
15 | 51 | 3 | [LV]PS[LI][DE]S[VS][DS][TI][LF]IQSPP[ANT][EG][HY][TS][ED]S[CG] [SN]LSP[QRS][NS]S[GD]LL[DH]A[VI]L[HY][EG]SQT[MP]KAS[KNS][SD][NI][SL][YH]Q | 2.4e-12 |
16 | 93 | 2 | [KM]L[HQ]Q[ST]SED[DE]SV[HR]KEG[AT]E[LM]EEV [MS]ECSQGS[NT]LAGC[PS]QSTSD[LM]GNTF[AV]H[KT]RE[EN]G [GK][LM][LS][EM][DE][IS][NV][RY][ER]KD[PT]S[AS]S[AS]APC[PS] [ET]YYTPAFED[IV][GT][CF]S[MV][EQ]EVPSEL[AV] | 3.5e-11 |
17 | 57 | 2 | G[AT]N[AD]A[IM]GK[IV]KQP[PS]GVLVEL[NS][AT][NS]D[LM] [FL]FSPDRF[FG][AT][KN][AH]DRAT[NS]LS[IN]KALGNQYARR[IL]EAA | 5.9e-10 |
18 | 38 | 2 | FDH[IN]DDQMLWHDDI[MV][LP]MD[AD][EN][DY][AE][KV]LDQDFI[FT][KN]CLW[DS]DQG | 9.1e-10 |
19 | 55 | 2 | TNCPNIQS[FL]DFTNSSSSSSYI[IT]FDENYDFLET[SY]NQ[EH]NDVTSIVNQVDDENIVILE | 1.1e-9 |
20 | 82 | 2 | LI[HP]T[FQ][AS]PD[FL][DG]I[CT]KLLEG[AV][FS] [GT][DE][RT][IL]VP[HQ][GQ]CGHGCC[AG][AN][TV][NS][KQ] [ES][IS][NS][GK]SSLLGPEFVDY[AE]E[HP]P[PS][FL][LS]SHELA [AS][LM]AT[DE][IL][NS]N[IV]AW[IY]KSGLE | 3.2e-9 |
21 | 21 | 4 | N[NI]N[NS][NT]MHEN[IV][VI]LE[SN]S[ED]WS[PS][NKS]E | 5.2e-9 |
Motif . | Width . | Sites . | Multilevel consensus sequence . | e-value . |
---|---|---|---|---|
1 | 15 | 34 | [IL]KMGIDP[VN]TH[KR]P[LR]SD | 2.5e-182 |
2 | 29 | 9 | [KR]QL[KH]C[DE][VIS]NS[KQ]QF[KQR][DEH][TF] [LIM][RKL]YLW[IM]P[RS]L[LV][EA][RKQ][IQ][QEI] | 2.3e-98 |
3 | 41 | 7 | [SV]A[NS][LA][SR]HMA[QE]WESARLEAEARL[VS]R[EK]S[KQ]IL [FV][NP][NS][NS][NV][NG][NRS][HNS]N[NTY][ND][IY][NF] | 1.5e-81 |
4 | 57 | 4 | DYQQDIFQEELANLE[ED]ITSGSFWTQPFEVDTKIDFVAP [SP]IDYCGL[VI]CPPSPF[IM][PS]HEF | 1.5e-65 |
5 | 119 | 2 | Y[IV]EPIPLVQPNF[MT]YNNNNMMNFQL GTNNQ[HY]SYNFHDQSL[MV][NY]PMQ[AT]ISSCSSSDGLSC [KN]QISYGNEE[IM]MCQIPFEETQKFTLD [NS]YCTTWADHQK[AT]NGYFGNNFQSSQ[FL]QYDDHTNIEEIK | 3.2e-47 |
6 | 120 | 2 | YYQQLPCLD[IR]LKAWQ[IM][AT]STKLPTINDISHAILR [NS]N[LS]KNKKLDSSI[PQ]SS[ST]LNSSENIFA[KN][DN]APTTTK [FV]D[DV]DD[DH]QNL[HQ]NLSTINSCF EDDQLLQTELPSFMQEFSGVFPEY[AT]QNSTNGLQVD | 1.8e-32 |
7 | 26 | 4 | [KR]GQWER[RT]LQ[AT]DI[HN][MT]A[KR][KQ]AL[CHQ][NDE]ALSL[DE] | 5.2e-30 |
8 | 120 | 2 | GALCY[DE]PPRFPSLD[TV]PF[FL][SY]CDL[IK]QSG[ST]DTQQE[FY] SPLGIRQLM[MT]TS[TV][DN]C[LS]TP[FL]RLWDSP[KL]R[AD] [DG]SPDA[IV]L[KR]SAAKTFT[GS]TPSILKKR[HQ]R [DH]LV[ST]PLSEKR[CW][EG]KKLESDL[NR] [KR]ESFSN[LM][AV][KT][DE]F[PS]R | 1.3e-27 |
9 | 120 | 2 | TP[FY]K[IR]SIESPSAWKSPW[FI][FT][DT]P[FL]LSSPR[LY][DE] [KN]ELT[FY]E[DE][FL]A[FL][FL][LM]SPGDRSYDAIGLMKQLSEQTA [GP][AS][FI]ADA[QR][EQ][IV]LG[GS][DE]TPETIL[LR]GRNSK[EN] QKADEN[HR][ST]LL[AS][AS]NAMSERRTLDFSECG[ST]PGKG | 3.8e-23 |
10 | 79 | 2 | Y[LP]N[TV]NIISSTDSF[LV][KR]EDDSNIW[KN][KN]LS SFEDGINI[PS][HR]DFSTPS[DY]M[KT]LHNYSSH[DH][EV] DDQYYFHNYWTSAEMPSLYEMD[HL]WL | 1.2e-22 |
11 | 17 | 5 | YASS[AT]ENI[AS][RK]LL[QEK][GNQ]W[MIT][KRG] | 3.7e-18 |
12 | 82 | 2 | TKIVPREK[FI][KN][NS]K[CR]GEISTKIEIIKPQ[AR]RK[FY][FI]S [NS]T[EM]KN[IV]TNN[IN]VI[LV]D[EK]EE[EH] CKEIISEKQT[PR]DAS[IM]DN[GV]D[EP]WW[AI]NLLENCNDD | 1.7e-16 |
13 | 64 | 2 | TSVTLENSSVATSSENSNQDYNQVNQSDQLWYEDYQAM [DE]QQNNIELWMDNEDVFNNLCN[IN]EDIW | 3.5e-19 |
14 | 78 | 3 | [NM]N[FVY]EIP[AT][VA]E[FN][KR][KNR][LF]E[FLP][NST] [QH][HLQ]L[YC]PP[AQR]L[LA][DN][IN][PD][AFT][GRS][SG] [FIL]L[DGN][DIT]P[AGV][RST][SN][LF][LR][ADS]QG[LH][NR][SA] [APS][YW][NSY][ST][HRT][SY][FV][LP]ST[TV][HPY][PS] [AS]KR[IT][RQ]G[SL]ES[LVW]FS[GN][LRS]N[GV]D[LC] | 8.8e-14 |
15 | 51 | 3 | [LV]PS[LI][DE]S[VS][DS][TI][LF]IQSPP[ANT][EG][HY][TS][ED]S[CG] [SN]LSP[QRS][NS]S[GD]LL[DH]A[VI]L[HY][EG]SQT[MP]KAS[KNS][SD][NI][SL][YH]Q | 2.4e-12 |
16 | 93 | 2 | [KM]L[HQ]Q[ST]SED[DE]SV[HR]KEG[AT]E[LM]EEV [MS]ECSQGS[NT]LAGC[PS]QSTSD[LM]GNTF[AV]H[KT]RE[EN]G [GK][LM][LS][EM][DE][IS][NV][RY][ER]KD[PT]S[AS]S[AS]APC[PS] [ET]YYTPAFED[IV][GT][CF]S[MV][EQ]EVPSEL[AV] | 3.5e-11 |
17 | 57 | 2 | G[AT]N[AD]A[IM]GK[IV]KQP[PS]GVLVEL[NS][AT][NS]D[LM] [FL]FSPDRF[FG][AT][KN][AH]DRAT[NS]LS[IN]KALGNQYARR[IL]EAA | 5.9e-10 |
18 | 38 | 2 | FDH[IN]DDQMLWHDDI[MV][LP]MD[AD][EN][DY][AE][KV]LDQDFI[FT][KN]CLW[DS]DQG | 9.1e-10 |
19 | 55 | 2 | TNCPNIQS[FL]DFTNSSSSSSYI[IT]FDENYDFLET[SY]NQ[EH]NDVTSIVNQVDDENIVILE | 1.1e-9 |
20 | 82 | 2 | LI[HP]T[FQ][AS]PD[FL][DG]I[CT]KLLEG[AV][FS] [GT][DE][RT][IL]VP[HQ][GQ]CGHGCC[AG][AN][TV][NS][KQ] [ES][IS][NS][GK]SSLLGPEFVDY[AE]E[HP]P[PS][FL][LS]SHELA [AS][LM]AT[DE][IL][NS]N[IV]AW[IY]KSGLE | 3.2e-9 |
21 | 21 | 4 | N[NI]N[NS][NT]MHEN[IV][VI]LE[SN]S[ED]WS[PS][NKS]E | 5.2e-9 |
Consensus amino acid sequences were obtained from analysis of the 127 tomato MYB proteins with MEME software. The motif numbers are equivalent to those described in Fig. 2C and Supplementary Table S2.
Since high-throughput sequencing and gene expression analyses have been performed on many tomato tissues at various developmental stages, publicly available RNA-seq data are thought to be a useful resources for studying gene expression profiles. Distinct transcript abundance patterns were readily identifiable in the RNA-seq data set at the Tomato Functional Genomics Database (TFGD; http://ted.bti.cornell.edu/). Nearly all SlMYB genes (except for nine: SlMYB39, SlMYB75, SlMYB25, SlMYB29, SlMYB21, SlMYB27, SlMYB98, SlMYB119 and SlMYB64) have sequence reads in at least one tissue, their universal expression also indicating the importance of MYB TFs. The expression profiles of the 127 SlMYB genes were analyzed as shown in Fig. 2D. Most of the SlMYB genes showed distinct tissue-specific expression patterns across the five tissues examined. Detailed analysis of the expression patterns of the SlMYB genes showed that some of the genes clustered in the same subgroup of the phylogenetic tree (Fig. 2A) had similar expression patterns, also suggesting the existence of redundancy among the MYB genes in these subgroups. For example, most of the SlMYB genes in subgroup 6 had dominant expression patterns in the root and relatively low expression in the other four tissues tested. However, SlMYB52 and SlMYB91 from the same subgroup 18 had totally different expression patterns (Fig. 2D). The paralogs with different expression patterns may share similar functions at different stages in plant development.
Phylogenetic analysis of the MYB gene family in tomato, Arabidopsis and rice
To investigate the phylogenic relationship among the MYB genes in tomato, Arabidopsis and rice, and to generate an evolutionary framework, the 127 predicted SlMYB proteins were subjected to multiple sequence alignment along with 133 Arabidopsis and 117 rice MYB proteins (Supplementary Table S3), and an unrooted phylogenetic tree was constructed using the Neignbor–Joining (NJ) method, based on the alignment of all the MYB amino acid sequences (Fig. 3). The NJ tree showed that all the MYB family proteins from the three higher plants were divided into 14 subgroups (Fig. 3). Among these, subgroup IV constituted the largest clade, containing 45 members and accounting for 11.9% of the total MYB genes. As illustrated in Fig. 3, the MYB proteins from the three species demonstrated an interspersed distribution in all the subgroups, indicating that the expansions of MYB genes occurred before the divergence of tomato, Arabidopsis and rice. Interestingly, large-scale expansion of subgroup XI members seems to occur in rice and Arabidopsis after the speciation/divergence of the three species, evidenced by a number of rice and Arabidopsis MYB proteins exclusively clustering together in subgroup XI (Fig. 3), suggesting that the expansion events of subgroup XI in rice and Arabidopsis might be much more active than those in tomato. Moreover, in subgroup I, three tomato MYB genes (SlMYB9, SlMYB15 and SlMYB13) clustered with the Arabidopsis AtMYB15 gene, which has been identified to be involved in drought and freezing tolerance (Agarwal et al. 2006, Ding et al. 2009). SlMYB101 in subgroup V showed maximum similarity with AtMYB33, AtMYB65 and AtMYB101, which are targeted by the miR159 family and involved in anther or pollen development (Allen et al. 2007, Addo-Quaye et al. 2008). Since some of the Arabidopsis MYBs with similar functions showed a tendency to fall into one subgroup, there is a possibility that the SlMYBs clustered with already characterized AtMYBs have similar functions. This would help for future study, but it still needs further investigation and more evidence.
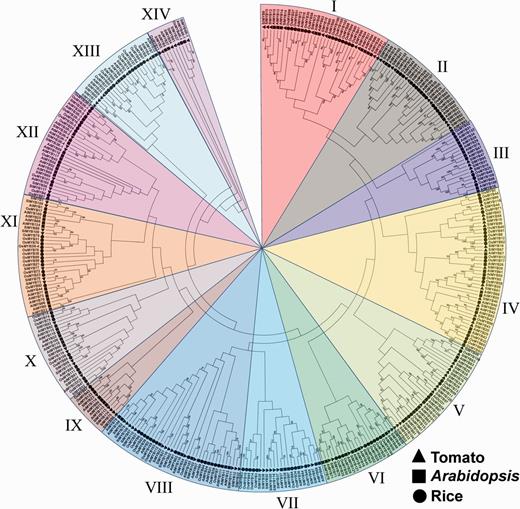
Phylogenetic tree of MYB genes among tomato, Arabidopsis and rice. Multiple sequence alignment of full-length MYB proteins was done using ClustalX 2.1, and the phylogenetic tree was constructed using MEGA 6.05 by the Neighbor–Joining method with 1,000 bootstrap replicates. The tree was divided into 14 phylogenetic subgroups marked with different colors. Bootstrap values >50 are indicated on the nodes. The leaf labels of members from tomato (SlMYBs), Arabidopsis (AtMYBs) and rice (OsMYBs) are denoted by a filled triangle, square and circle, respectively.
Chromosomal distribution and duplication events among tomato MYB genes
To determine the genomic distribution of the SlMYB genes, we searched the tomato genome database (Sol Genomics Network; SGN) using the identification number of each tomato MYB gene. Genome chromosomal location analyses revealed that tomato MYB genes were distributed on all chromosomes. Although each of the 12 tomato chromosomes contained MYB genes, the distribution appeared to be uneven (Fig. 4). In total, six SlMYB genes were present on chromosome 11; eight on each of chromosomes 9 and 12; nine on chromosome 7; 10 on each of chromosomes 1, 2, 4 and 8; 12 on chromosome 3; 13 on chromosome 5; 15 on chromosome 10; and 16 on chromosome 6 (Fig. 4). On average, one SlMYB gene was presented every 8.1 Mb. Relatively high densities of MYB genes were observed in some chromosomal regions, including the top and bottom of chromosomes 4, 5, 6 and 10; the top of chromosomes 9; and the bottom of chromosomes 1, 2, 3, 7, 8, 11 and 12. In contrast, almost all central chromosomal regions lacked MYB genes, including the centromere and the pericentromere regions (Fig. 4).

Chromosomal locations and gene duplications for tomato MYB genes. Chromosomal positions of the MYB genes in tomato are mapped on the basis of the tomato genome database SGN (Release 2.4). The chromosome number is indicated above each chromosome. The scale is in megabases (Mb). The number below the chromosome name indicates the number of SlMYB genes in this chromosome. The phylogenetic category of each gene (Fig. 2A) is indicated by the subgroup number. The plus (+) or minus (–) sign in parentheses indicates the gene direction. Each pair of duplicated MYB genes is connected with an orange line. Connecting lines mark the specific cases in which there is a strong correlation between duplicated genomic regions and the presence of MYB genes with closely related predicted amino acid sequences. Green boxes indicate tandemly duplicated gene clusters on the chromosomes. Red bars on the chromosomes and blue numbers beside the bars indicate the seven predicted duplication regions. The black dots on the chromosomes indicate the centromeres.
The origin of multigene families could be attributed to gene duplication that arose from region-specific duplication or genome-wide polyploidization. This mechanism has been proved to be a prominent feature of plant genome evolution. To detect a potential relationship between SlMYBs and genome duplications, we identified approximately 15 pairs of SlMYBs that were highly similar paralogs in the same subclades (Fig. 4, linked with orange lines) and shared a high degree of identity through their protein sequences. These genes represent approximately 23.6% (30 of 127) of the tomato MYBs, which supported the hypothesis that they might evolve from putative tomato genome duplication events. In addition, multiple pairs linked each of at least seven potential chromosomal/segmental duplications (Fig. 4, pairs of red bars with numbers 1–7). Our results showed that many predicted SlMYBs had paralogous counterparts in syntenic regions of related chromosomes (Fig. 4), which indicated that segmental duplication might play a role in the expansion of the tomato MYB gene family.
In the present study, intrachromosomal duplication was also observed in the tomato genome. A series of tandem gene duplications was also indicated to contribute to increasing the number of tomato MYB family genes, as evidenced by the fact that some closely related SlMYBs in a single cluster were physically located near to each other on a given chromosome with no more than one intervening annotated gene: for example, SlMYB63/SlMYB81 (subgroup 10, Fig. 2) on chromosome 3, and SlMYB105/SlMYB50 (subgroup 6, Fig. 2) on chromosome 6 (Fig. 4, marked with green boxes). In total, about 15.7% (20 of 127) of SlMYB genes were involved in tandem duplication. Together, our findings indicate that the segmental duplication and tandem gene duplication all contributed to MYB gene expansion in tomato.
Expression analysis of SlMYB genes in response to plant hormones SA and MeJA
Real-time PCR was used to analyze the expression profiles of MYB family genes under SA and MeJA treatments in tomato. A number of SlMYB family genes were related to these two plant hormones. Genes with fold changes >3 at the time points of 2 h and/or 6 h were presented in our study (Supplementary Figs. S3, S4). In addition, we did not obtain acceptable real-time PCR results for SlMYB5, SlMYB6, SlMYB11, SlMYB21, SlMYB35, SlMYB57, SlMYB80 and SlMYB118, which might be due to their low transcript abundance and/or weak treatment effect. In SA treatment, 18 SlMYB genes were significantly regulated (14 up-regulated and four down-regulated) (Supplementary Fig. S3). In MeJA treatment, the mRNA levels of 21 SlMYB genes were significantly changed (17 up-regulated and four down-regulated) (Supplementary Fig. S4). Interestingly, we found that 10 SlMYB genes showed response to both SA and MeJA treatments (Fig. 5). Of the 10 genes, seven (SlMYB14, SlMYB28, SlMYB65, SlMYB66, SlMYB77, SlMYB116 and SlMYB122) were up-regulated in response to both SA and MeJA, while SlMYB17 was down-regulated by both SA and MeJA. Noticeably, the expression level of SlMYB62 was enhanced by SA but repressed by MeJA. In contrast, the expression level of SlMYB113 was repressed by SA but enhanced by MeJA.
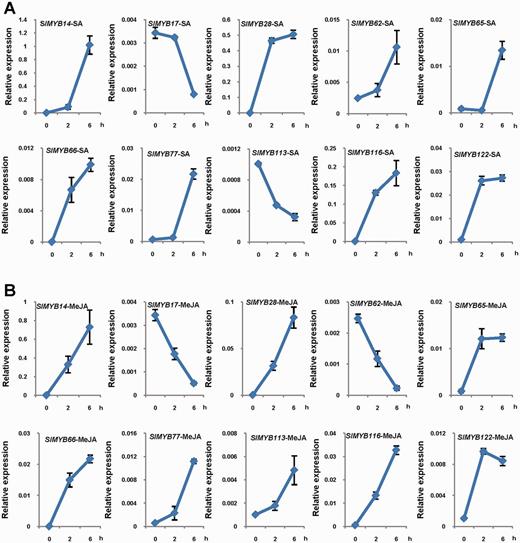
Expression patterns of 10 SlMYB genes in response to SA and MeJA treatments. (A) SA-induced expression patterns of 10 SlMYB genes. Three-week-old tomato seedlings, grown under standard conditions, were subjected to SA treatment. The aerial parts were harvested at the indicated times for RNA extraction and qRT-PCR analysis. Zero time samples were taken prior to treatment. Transcript levels of SlMYB genes were normalized to ACTIN2 expression. (B) MeJA-induced expression patterns of 10 SlMYB genes. Three-week-old tomato seedlings, grown under standard conditions, were subjected to MeJA treatment. The SlMYB mRNA levels were analyzed as in (A). All hormone treatments were initiated at the beginning of the 16 h light period (28°C) to ensure identical environmental conditions throughout the time course. Data shown are the average and SD of three biological replicates.
In order to validate our real-time results, histochemical β-glucuronidase (GUS) staining of transgenic Arabidopsis plants carrying a SlMYB promoter–GUS fusion construct was conducted. Promoters of four selected SlMYB genes that markedly respond to both SA and MeJA treatments (SlMYB14, SlMYB17, SlMYB62 and SlMYB66) were amplified from tomato genomic DNA by PCR and used to drive the expression of the GUS reporter gene. The SlMYBpro:GUS cassette was stably transformed into Arabidopsis. Seven-day old transgenic seedlings were subjected to SA and MeJA treatments and subsequently to histochemical staining (Fig. 6). Increased GUS staining was observed in plants harboring SlMYB14pro:GUS and SlMYB66pro:GUS vectors that were previously treated with SA or MeJA, mirroring the expression patterns of the two endogenous genes in tomato. This was also true for SlMYB17. Moreover, the plants harboring SlMYB62pro:GUS presented an enhanced GUS signal in response to SA; however, we did not observe an apparent change of GUS activity when the plants were treated with MeJA (Fig. 6).
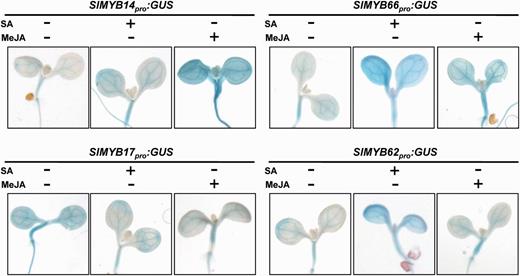
Histochemical analysis of GUS expression driven by four SlMYB promoters in transgenic Arabidopsis. Seven-day-old seedlings grown on 1/2 MS medium were subjected to SA or MeJA treatment. GUS activity was detected according to the method described by Jefferson et al. (1987).
Expression analysis of SlMYB genes under different stresses.
To investigate the SlMYB gene expression patterns in response to biotic and abiotic stresses, systematic analyses of RNA-seq and microarray data were carried out. One RNA-seq data set (series accession No. D007 and SRA accession No. 096750) and one microarray data set (Affymetrix microarray platform, series accession No, E051 and GEO accession No. GSE16401) were downloaded from the TFGD (http://ted.bti.cornell.edu). Through BLAST analysis, a total of 48 probes corresponding to SlMYB genes were identified, accounting for about 40% of the gene family. The expression of a number of SlMYB genes was significantly altered (fold change > 2) upon different biotic and abiotic stress treatments, including invasion of the bacterial pathogen Pst DC3000, Pseudomonas putida and Pseudomonas fluorescens and salt stress (Fig. 7A–D). Under the stresses of bacterial elicitors, the expression level was dramatically changed for a number of SlMYB genes (Fig. 7A–C). Twelve SlMYB genes displayed significantly elevated expression in response to Pst DC3000, while two (SlMYB31 and SlMYB33) were repressed (Fig. 7A). Thirteen SlMYB genes were regulated by both P. putida and P. fluorescens, and 10 SlMYB genes were specifically regulated by P. putida (Fig. 7B, C).The expression of six SlMYB genes was significantly increased under salt stress (Fig. 7D), while the expression of SlMYB91 and SlMYB96 was significantly down-regulated (Fig. 7D).
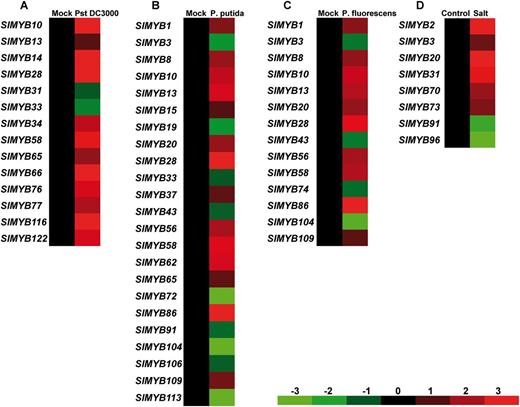
Expression profiles of SlMYB genes in response to different bacteria and salt stress. Expression was visualized using heat maps (see the Materials and Methods). Blocks with colors indicate decreased (green) or increased (red) transcript accumulation relative to the respective control. The bar at the bottom of the heat map represents relative expression values. The numbers above the bar indicate the log-transformed fold change. (A) Expression profiles of SlMYB genes in tomato infected by Pst DC3000. (B) Expression profiles of SlMYB genes in tomato infected by Pseudomonas putida. (C) Expression profiles of SlMYB genes in tomato infected by Pseudomonas fluorescens. (D) Expression profiles of SlMYB genes under salt stress.
In our study, the 127 SlMYB genes were also subjected to Gene Ontology (GO) analyses. Using updated GO terms maintained by the agriGO project (http://bioinfo.cau.edu.cn/agriGO/) and a Singular Enrichment Analysis (SEA) with both minimal P-value (Fisher) and false discovery rate (FDR) (Hochberg) q-value set at 0.05, we were able to map 33 significant GO terms to the tomato MYB gene family. GO analysis showed significant enrichment of SlMYB genes involved in metabolic and biosynthetic processes (Supplementary Fig. S5). It is tempting to speculate that substance biosynthesis and/or metabolism promoted by MYB proteins might be the basic mechanism for the involvement of MYB genes in plant response to various environmental stresses.
Identification of EAR motif-containing MYB proteins in tomato
EAR motif-mediated transcriptional repression is emerging as one of the principal mechanisms for plant gene regulation. The EAR motif, defined by the consensus sequence patterns of either LxLxL or DLNxxP (where x can be one of the 20 common amino acids), is the most predominant form of transcriptional repression motif so far identified in plants (Ohta et al. 2001, Kagale and Rozwadowski 2011).
MYB proteins are characterized by a highly conserved DBD and a variable C-terminal domain that is thought to be responsible for the activation or repression of specific target genes (Jin and Martin 1999). In our study, manual search has identified 24 members of the tomato MYB family containing at least one LxLxL or DLNxxP type of EAR motif (Table 2). SlMYB73 and SlMYB3R-2 have two EAR motifs. The 26 EAR motif sites were mainly found in the N-terminal region (16 out of 26) and at lower frequency in the C-terminal (seven out of 26) and middle (3 out of 26) regions (Table 2).
Name . | GeneID . | Size . | EAR motif . | |
---|---|---|---|---|
. | . | . | Sequence . | Location . |
SlMYB5 | Solyc01g005660.1.1 | 308 | LILRL | 93 |
SlMYB8 | Solyc01g111500.2.1 | 252 | LNLEL | 189 |
SlMYB73 | Solyc02g092930.1.1 | 361 | LSLSL | 198 |
LGLGL | 334 | |||
SlMYB116 | Solyc03g119370.1.1 | 280 | LILEL | 88 |
SlMYB70 | Solyc04g078420.1.1 | 328 | LCLSL | 205 |
SlMYB6 | Solyc05g008250.1.1 | 178 | LDLNLIP | 172 |
SlMYB62 | Solyc05g009230.1.1 | 274 | LILEL | 83 |
SlMYB17 | Solyc05g048830.2.1 | 319 | LQLLL | 274 |
SlMYB2 | Solyc05g053330.2.1 | 318 | LILEL | 101 |
SlMYB59 | Solyc06g005330.2.1 | 236 | LILEL | 71 |
SlMYB21 | Solyc06g009410.1.1 | 260 | LILLL | 73 |
SlMYB22 | Solyc06g009480.1.1 | 247 | LILLL | 73 |
SlMYB14 | Solyc06g083900.2.1 | 255 | LMLEL | 248 |
SlMYB29 | Solyc07g054980.1.1 | 468 | LILNL | 77 |
SlMYB3R-2 | Solyc08g080580.2.1 | 481 | LNLNL | 207 |
LSLSL | 390 | |||
SlMYB4 | Solyc10g055410.1.1 | 273 | LNLEL | 190 |
SlMYB90 | Solyc10g086250.1.1 | 275 | LILRL | 76 |
SlMYB114 | Solyc10g086260.1.1 | 274 | LILRL | 76 |
SlMYB113 | Solyc10g086270.1.1 | 259 | LILRL | 76 |
SlMYB75 | Solyc10g086290.1.1 | 265 | LILRL | 74 |
SlMYB48 | Solyc11g073120.1.1 | 217 | LVLEL | 72 |
SlMYB112 | Solyc12g099120.1.1 | 247 | LILQL | 83 |
SlMYB57 | Solyc12g099130.1.1 | 235 | LILQL | 83 |
SlMYB108 | Solyc12g099140.1.1 | 300 | LILQL | 100 |
Name . | GeneID . | Size . | EAR motif . | |
---|---|---|---|---|
. | . | . | Sequence . | Location . |
SlMYB5 | Solyc01g005660.1.1 | 308 | LILRL | 93 |
SlMYB8 | Solyc01g111500.2.1 | 252 | LNLEL | 189 |
SlMYB73 | Solyc02g092930.1.1 | 361 | LSLSL | 198 |
LGLGL | 334 | |||
SlMYB116 | Solyc03g119370.1.1 | 280 | LILEL | 88 |
SlMYB70 | Solyc04g078420.1.1 | 328 | LCLSL | 205 |
SlMYB6 | Solyc05g008250.1.1 | 178 | LDLNLIP | 172 |
SlMYB62 | Solyc05g009230.1.1 | 274 | LILEL | 83 |
SlMYB17 | Solyc05g048830.2.1 | 319 | LQLLL | 274 |
SlMYB2 | Solyc05g053330.2.1 | 318 | LILEL | 101 |
SlMYB59 | Solyc06g005330.2.1 | 236 | LILEL | 71 |
SlMYB21 | Solyc06g009410.1.1 | 260 | LILLL | 73 |
SlMYB22 | Solyc06g009480.1.1 | 247 | LILLL | 73 |
SlMYB14 | Solyc06g083900.2.1 | 255 | LMLEL | 248 |
SlMYB29 | Solyc07g054980.1.1 | 468 | LILNL | 77 |
SlMYB3R-2 | Solyc08g080580.2.1 | 481 | LNLNL | 207 |
LSLSL | 390 | |||
SlMYB4 | Solyc10g055410.1.1 | 273 | LNLEL | 190 |
SlMYB90 | Solyc10g086250.1.1 | 275 | LILRL | 76 |
SlMYB114 | Solyc10g086260.1.1 | 274 | LILRL | 76 |
SlMYB113 | Solyc10g086270.1.1 | 259 | LILRL | 76 |
SlMYB75 | Solyc10g086290.1.1 | 265 | LILRL | 74 |
SlMYB48 | Solyc11g073120.1.1 | 217 | LVLEL | 72 |
SlMYB112 | Solyc12g099120.1.1 | 247 | LILQL | 83 |
SlMYB57 | Solyc12g099130.1.1 | 235 | LILQL | 83 |
SlMYB108 | Solyc12g099140.1.1 | 300 | LILQL | 100 |
The transcriptional activation activities of five genes marked in bold in this table were investigated in the yeast system (Fig. 9).
Name . | GeneID . | Size . | EAR motif . | |
---|---|---|---|---|
. | . | . | Sequence . | Location . |
SlMYB5 | Solyc01g005660.1.1 | 308 | LILRL | 93 |
SlMYB8 | Solyc01g111500.2.1 | 252 | LNLEL | 189 |
SlMYB73 | Solyc02g092930.1.1 | 361 | LSLSL | 198 |
LGLGL | 334 | |||
SlMYB116 | Solyc03g119370.1.1 | 280 | LILEL | 88 |
SlMYB70 | Solyc04g078420.1.1 | 328 | LCLSL | 205 |
SlMYB6 | Solyc05g008250.1.1 | 178 | LDLNLIP | 172 |
SlMYB62 | Solyc05g009230.1.1 | 274 | LILEL | 83 |
SlMYB17 | Solyc05g048830.2.1 | 319 | LQLLL | 274 |
SlMYB2 | Solyc05g053330.2.1 | 318 | LILEL | 101 |
SlMYB59 | Solyc06g005330.2.1 | 236 | LILEL | 71 |
SlMYB21 | Solyc06g009410.1.1 | 260 | LILLL | 73 |
SlMYB22 | Solyc06g009480.1.1 | 247 | LILLL | 73 |
SlMYB14 | Solyc06g083900.2.1 | 255 | LMLEL | 248 |
SlMYB29 | Solyc07g054980.1.1 | 468 | LILNL | 77 |
SlMYB3R-2 | Solyc08g080580.2.1 | 481 | LNLNL | 207 |
LSLSL | 390 | |||
SlMYB4 | Solyc10g055410.1.1 | 273 | LNLEL | 190 |
SlMYB90 | Solyc10g086250.1.1 | 275 | LILRL | 76 |
SlMYB114 | Solyc10g086260.1.1 | 274 | LILRL | 76 |
SlMYB113 | Solyc10g086270.1.1 | 259 | LILRL | 76 |
SlMYB75 | Solyc10g086290.1.1 | 265 | LILRL | 74 |
SlMYB48 | Solyc11g073120.1.1 | 217 | LVLEL | 72 |
SlMYB112 | Solyc12g099120.1.1 | 247 | LILQL | 83 |
SlMYB57 | Solyc12g099130.1.1 | 235 | LILQL | 83 |
SlMYB108 | Solyc12g099140.1.1 | 300 | LILQL | 100 |
Name . | GeneID . | Size . | EAR motif . | |
---|---|---|---|---|
. | . | . | Sequence . | Location . |
SlMYB5 | Solyc01g005660.1.1 | 308 | LILRL | 93 |
SlMYB8 | Solyc01g111500.2.1 | 252 | LNLEL | 189 |
SlMYB73 | Solyc02g092930.1.1 | 361 | LSLSL | 198 |
LGLGL | 334 | |||
SlMYB116 | Solyc03g119370.1.1 | 280 | LILEL | 88 |
SlMYB70 | Solyc04g078420.1.1 | 328 | LCLSL | 205 |
SlMYB6 | Solyc05g008250.1.1 | 178 | LDLNLIP | 172 |
SlMYB62 | Solyc05g009230.1.1 | 274 | LILEL | 83 |
SlMYB17 | Solyc05g048830.2.1 | 319 | LQLLL | 274 |
SlMYB2 | Solyc05g053330.2.1 | 318 | LILEL | 101 |
SlMYB59 | Solyc06g005330.2.1 | 236 | LILEL | 71 |
SlMYB21 | Solyc06g009410.1.1 | 260 | LILLL | 73 |
SlMYB22 | Solyc06g009480.1.1 | 247 | LILLL | 73 |
SlMYB14 | Solyc06g083900.2.1 | 255 | LMLEL | 248 |
SlMYB29 | Solyc07g054980.1.1 | 468 | LILNL | 77 |
SlMYB3R-2 | Solyc08g080580.2.1 | 481 | LNLNL | 207 |
LSLSL | 390 | |||
SlMYB4 | Solyc10g055410.1.1 | 273 | LNLEL | 190 |
SlMYB90 | Solyc10g086250.1.1 | 275 | LILRL | 76 |
SlMYB114 | Solyc10g086260.1.1 | 274 | LILRL | 76 |
SlMYB113 | Solyc10g086270.1.1 | 259 | LILRL | 76 |
SlMYB75 | Solyc10g086290.1.1 | 265 | LILRL | 74 |
SlMYB48 | Solyc11g073120.1.1 | 217 | LVLEL | 72 |
SlMYB112 | Solyc12g099120.1.1 | 247 | LILQL | 83 |
SlMYB57 | Solyc12g099130.1.1 | 235 | LILQL | 83 |
SlMYB108 | Solyc12g099140.1.1 | 300 | LILQL | 100 |
The transcriptional activation activities of five genes marked in bold in this table were investigated in the yeast system (Fig. 9).
From the current literature, it is evident that the EAR motif functions as a ‘potential repressor’ by physically interacting with other co-repressors (Hill et al. 2008, Szemenyei et al. 2008). The protein sequence context in which the EAR motif appears may influence its ability to interact with potential interacting proteins. We analyzed the local sequence context within and around the EAR motif sites by comparing a protein sequence region of approximately nine amino acids, comprising the core EAR motif sites ±2 amino acids. Among the 26 EAR motifs, histidine is a preferred residue at position 8. Position 2 is occupied more frequently by leucine and aspartate. Glutamine and serine residues are more abundant in positions 1 and 9, respectively. Isoleucine and glutamate residues appear more frequently at position 4 and 6, respectively (Fig. 8A).
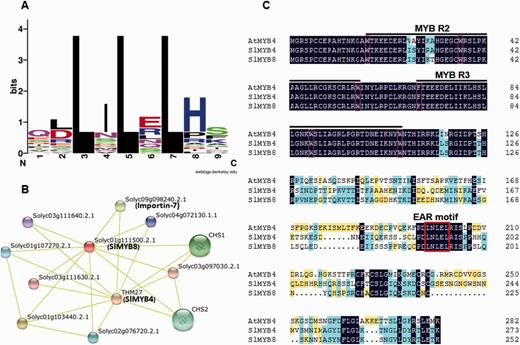
Protein sequence context of 26 EAR motifs and protein interaction network predicted for SlMYB4 and SlMYB8. (A) Amino acid sequence context of EAR motif-containing SlMYB proteins. The core EAR motif site and flanking ±2 amino acid sequences of 25 LxLxL and one overlapping LxLxL and DLNxxP types of EAR motif-containing proteins were used for generating WebLogos using the WebLogo program (Crooks et al. 2004). The height of the letter representing an amino acid in each position reflects the difference in the frequency of its occurrence in the experimental sets. (B) Interaction network of SlMYB4 and SlMYB8 in tomato. Colored balls (protein nodes) in the network were used as a visual aid to indicate different input proteins and predicted interactors. Protein nodes which are enlarged indicate the availability of 3D protein structure information. Yellow lines connect proteins which are associated by recurring textmining evidence. (C) Amino acid sequence alignment between SlMYB4, SlMYB8 and AtMYB4. The alignment was performed using ClustalX 2.1 and DNAMAN software. A black background indicates conserved residues among all the proteins selected, and non-identical residues are shaded in light blue and yellow. The dashes indicate gaps introduced for better alignment. The conserved W residues in R2 and R3 repeats are indicated by pink boxes. The MYB domain is indicated by black lines and the EAR motifs are marked with a red box.
Subsequently, the protein interactions were examined using STRING (Search Tool for the Retrieval of Interacting Genes/Proteins) software and the corresponding database to retrieve the interaction network. The 24 EAR motif-containing SlMYB proteins were involved in at least six interaction possibilities (Supplementary Fig. S6). Interestingly, THM27 (SlMYB4) and SlMYB8 (Solyc01g111500.2.1) were found to interact with CHS1, CHS2 and importin-7 (Solyc09g098240.2.1) (Fig. 8B). Previous research showed that AtMYB4 has to interact with SAD2, an importin β-like protein, to finish its nuclear trafficking (Zhao et al. 2007). The sequence alignment also showed that SlMYB4 and SlMYB8 shared relatively high homology with AtMYB4. Therefore, it is tempting to speculate that SlMYB4 and SlMYB8 might have functions similar to AtMYB4 (Fig. 8C).
As described above, the EAR motif is highly conserved in TFs known to function as negative regulators. The transcriptional repression activity of EAR motif-containing proteins has to be achieved by the interaction between the EAR motif and other co-repressors (Kagale et al. 2010). However, whether the EAR motif-containing SlMYB proteins have transcriptional activation activities is still unknown. To make a preliminary investigation of this possibility, five selected genes representing different EAR location categories in Table 2 were ligated into the pGBKT7 vector and introduced into the Y2HGold yeast strain. OsMYB103L, also known as OsMYB83 in our study, and its truncated version were used as the positive and negative control, respectively (Yang et al. 2014). The result showed that the five SlMYB genes displayed strong transcriptional activation activities in the yeast system (Fig. 9).
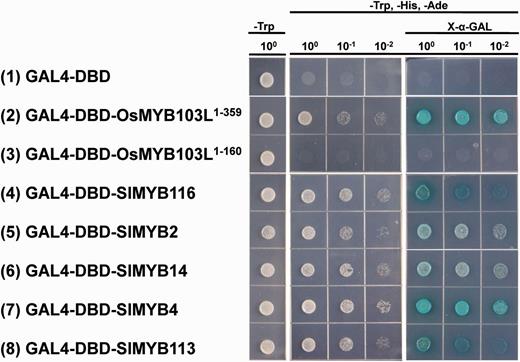
The investigation of transcriptional activation activities of five EAR motif-containing SlMYB proteins in yeast. Full-length selected SlMYB proteins were tested for the transactivation activity (see the Materials and Methods for details). The empty pGBKT7 vector was used as a blank control. Survival of yeast cells on the selective media needs the presence of functional activator peptides. X-α-Gal was used to test the expression of α-galactosidase. The indicated concentration shows the dilution of yeast cell culture.
Discussion
The MYB family is one of the largest transcription factor families in plants and has been identified in various species such as Arabidopsis, rice, maize and soybean (Stracke et al. 2001, Yanhui et al. 2006, Du et al. 2012a, Du et al. 2012b). In the present study, we performed a genome-wide survey of the MYB gene family in tomato. A total of 127 open reading frames (ORFs) encoding MYBs were identified, most of which remain to be functionally characterized. Subsequently, the deduced amino acid sequences encoded by the ORFs were subjected to an overview analysis. As a part of this, a phylogenetic tree was constructed to evaluate the evolutionary relationships of MYB genes in tomato. Detailed analyses including gene structures, conserved motif composition and chromosome distribution were performed to explore potential mechanisms leading to SlMYB family expansion. To identify the probable functions and evolution of SlMYB genes, we compared them with the MYBs of Arabidopsis and rice. In addition, we also analyzed the expression profiles of SlMYB genes in different tissues as well in response to plant hormones and different biotic and abiotic stresses. Finally, we reported the identification and structural analysis of 24 tomato EAR motif-containing proteins which may shed light on the transcriptional regulatory mechanisms of the tomato MYB gene family.
In general, MYB proteins feature a highly conserved DBD in the N-terminus (Kranz et al. 1998, Jiang et al. 2004a, Dubos et al. 2010, Du et al. 2012b). This domain is mainly composed of up to four imperfect repeats (R) and contains three helices of each repeat, the second and third of which form a HTH structure when bound to a cis-element (Kranz et al. 1998, Dubos et al. 2010). Three highly conserved tryptophan residues were generally present in the R2 repeat, whereas the first tryptophan residue in the R3 repeat exhibits variability (Fig. 1). The highly conserved residues were generally distributed at the latter half of each repeat, especially in the third helix. Our results indicate that during evolution, the third helix is more conserved than the first two helixes. It was previously demonstrated that the residues in the third helix are important for DNA binding activity, by interaction with the major groove of the DNA bases when bound to DNA (Ogata et al. 1994). Therefore, the highly conserved characteristic of the third helix may reflect the functional conservation among different plant species during MYB evolution, while species-specific genes may be derived from key residue variations in this region. Accordingly, the substitutions in the third helix may result in recognition of novel target genes and/or may seriously impair the DNA-binding activities. This may dictate the transcriptional regulatory role in most biological processes. In addition, it has been reported that substitution of residues within the linker region between R2 and R3 repeats gave rise to reduced stability of protein–DNA complexes, and even loss of DNA-binding ability (Dias et al. 2003, Heine et al. 2004). Surprisingly, in our study, five SlMYBs (SlMYB12, SlMYB32, SlMYB88, SlMYB100 and SlMYB111) have a substitution at Pro55 (P) by serine (S) in the linker region (Supplementary Fig. S2), and are consequently predicted to affect their binding ability.
MYB TFs play significant roles in the regulation of secondary metabolism, the control of cell shape, the response to various stress conditions, and hormone responses in higher plants. In spite of their large number and significance, very few of these genes have been functionally characterized in Solanaceae, such as tomato. The current investigations unveiled the MYB TF family with 127 members in tomato. Compared with MYB proteins in various plant species, the MYBs that tended to cluster together were usually from the same lineage, which indicates recent common evolutionary origins. In general, the orthologs clustered in a subgroup or subclade (functional clade) shared similar gene structure and functions, indicating recent common evolutionary origins. In other words, knowledge of the functions of certain members should facilitate the confirmation of paralogous and orthologous functional relationships. Phylogenetic comparative analysis of MYBs in different plant species also revealed considerable diversification and conservation of the MYB gene family in plants. The major groups/subgroups contained members of orthologous genes belonging to tomato, Arabidopsis and rice, suggesting that the appearance of many of these genes pre-dates monocot/eudicot divergence (Fig. 3). Though the roles of most SlMYBs remain to be elucidated, it is likely that members of a given group/subgroup may have recent common evolutionary origins and also a conserved function.
According to our analysis, most SlMYBs are clustered with orthologs of plant MYBs in different subgroups (Fig. 3), suggesting the functional conservation of plant MYBs. Many outstanding examples of functional conservation are demonstrated in our analysis. For example, subgroup VIII consists of SlMYB12, AtMYB11, AtMYB12 and AtMYB111, implicated in the control of flavonoid accumulation (Adato et al. 2009, Ballester et al. 2010, Stracke et al. 2010, Pandey et al. 2014, Pandey et al. 2015a, Pandey et al. 2015b). In subgroup VII, SlMYB50, SlMYB105, SlMYB37 and SlMYB110 (blind gene) clustered together with a group of blind-like MYB genes (AtMYB37, AtMYB38 and AtMYB87) that are involved in plant development, such as axillary meristem regulation and lateral organ formation, etc (Schmitz et al. 2002, Keller et al. 2006, Müller et al. 2006, Chahtane et al. 2013). Although only one blind-related gene has been reported in tomato to date (SlMYB110), the other SlMYBs clustered in this subgroup share high sequence similarity with SlMYB10, suggesting a similar functional feature of these SlMYBs in plant development. An interesting question for future research will be to investigate whether these tomato MYB orthologs within this subgroup have retained the ancestral function or have evolved new functions. The existing knowledge of the functions of the plant MYB family and the phylogenetic analysis of the identified MYB genes among different species will facilitate the characterization of each subgroup of the tomato MYB gene family.
Remarkably, the genes in the same group generally exhibit the same intron pattern, and the position of the intron is almost completely conserved within most subgroups (Du et al. 2012b). Additionally, the first two exons of the modal lengths are very similar and highly conserved (Matus et al. 2008). This finding tests the reliability of our phylogenetic analysis and constitutes an independent criterion. In our study, the number of introns in the SlMYB genes appeared to be limited; among the 127 typical SlMYB genes, a majority (87.4%) had no more than two introns. These results validate our classification of SlMYB genes, and indicate that the intron patterns are not random, but highly conserved. Moreover, the members of SlMYB genes within the same subgroup did not always show similar intron/exon structures and formed a complex exon/intron organization in the entire ORF, such as subgroup 14 and 18 (Fig. 2B).
In contrast, the other regions, especially the C-terminus, of the MYB proteins are highly variable (Dubos et al. 2010, Li et al. 2012). However, the conserved C-terminal motifs of MYB proteins were identified in soybean, Arabidopsis and rice (Kranz et al. 199, Jiang et al. 2004b, Du et al. 2012b). Some of them have been proved to be involved in controlling transcriptional activity. For example, mutation of an amino acid in the conserved GY/FDFLGL motif abolishes the transcriptional repressive activity of AtMYB4, AtMYB7 and AtMYB32 (Zhou et al. 2015). In this study, it was found that some tomato MYB proteins have various conserved C-terminal motifs, suggesting that they may have similar functions (Fig. 2C; Supplementary Table S2). Members of the same subgroups generally shared one or more identical motifs outside the MYB DBDs. The schemes of protein motifs of individual members of the MYB gene family indicated structural similarities within subgroups, further supporting the subgroup definition in phylogenetic analysis. This may also indicate that the highly conserved protein motifs are protein domain combinations, often lineage specific. Although most of these conserved motifs remain to be functionally clarified, it is quite reasonable to expect that some play important roles in the transcriptional regulation of target genes and may promote further functional diversification in specific lineages.
The gene duplication events throughout plant evolution have long been recognized and contribute to the establishment of new gene functions and underline the origins of evolutionary novelty (Kent et al. 2003, Cannon et al. 2004). In this study, it was found that seven potential chromosomal/segmental duplications of multiple pairs are associated with genome-wide duplication in the tomato genome (Fig. 4). Furthermore, tandem duplication events of SlMYB genes were also found in the tomato genome (Fig. 4). Interestingly, the DBDs between tandemly duplicated SlMYB genes were virtually identical. Except for a few differences in the C-terminus, their corresponding whole sequences were very similar (>90%). Thus, it is tempting to infer that new genes initially resulted from the duplication, and thereafter from a series of synonymous and/or non-synonymous mutations in the whole sequence (especially in the MYB DBD), to perform new functions. In addition, the presence of one tandem array of SlMYB47/SlMYB95 (located on chromosome 5) suggests tandem duplication of the ancestor of these two genes posterior to the most recent segmental duplication (Fig. 4).
The activation of inducible immune responses in the plant is tightly regulated, ensuring an effective and cost-efficient response to pathogenic microbes and herbivorous insects (Vos et al. 2013). Recognition of an attacker leads to accumulation of signaling molecules such as the plant hormones SA, and JA and its derivatives, which play major roles in the activation of downstream defense responses (Pieterse et al. 2012). Cross-talk between SA and JA signaling pathways plays an important role in the regulation and fine-tuning of induced defenses that are activated upon pathogen and/or insect attack. SA- and JA-dependent pathways regulate defense responses that are differentially effective against specific types of attackers. Pathogens with a biotrophic lifestyle are generally more sensitive to SA-dependent responses, whereas necrotrophic pathogens and herbivorous insects are commonly deterred by JA-dependent defenses (Thomma et al. 2001, Glazebrook 2005). There is ample evidence that SA and JA signaling pathways are mutually antagonistic (Koornneef and Pieterse 2008). In fact, signaling interactions can be either mutually antagonistic or synergistic, resulting in negative or positive functional outcomes (Reymond and Farmer 1998). In our study, 10 SlMYB genes showed a significant response to both SA and MeJA (Fig. 5), which might be involved in the cross-talk between these two plant hormones and contribute to a regulatory potential that helps plants in fine-tuning the defense response to the invaders encountered.
As autotrophic sessile organisms, plants have developed various strategies to deal with unfavorable environmental conditions. In fact, many MYB genes in a wide range of plant species, such as Arabidopsis, rice and wheat, are involved in the response to biotic and abiotic stresses (Dubos et al. 2010). AtMYB60 and AtMYB96 act through the ABA signaling pathway to regulate stomatal movement (Cominelli et al. 2005), and drought stress and disease resistance (Seo et al. 2009, Seo and Park 2010), respectively. AtMYB102 and AtMYB41 contribute to plant resistance against insects (De Vos et al. 2006). In Arabidopsis, the overexpression of AtMYB44 and OsMYB4 confers tolerance to abiotic stresses (Vannini et al. 2004, Jung et al. 2008). Several Arabidopsis MYB genes are targets of S-nitrosoglutathione which can regulate protein function by a post-translational modification designated S-nitrosylation. This process may contribute to the modulation of gene expression in response to environmental stimuli (Begara-Morales et al. 2014). In rice, nine OsMYB genes were reported to be repressed by heat stress. This may be due to a self-protection strategy against environmental stress (González-Schain et al. 2015). In wheat (Triticum aestivum), the transcription of TaMYB33 is induced by abiotic stresses, and its overexpression increases the tolerance of the plant to abiotic stress (Qin et al. 2012). In grape, VvMYB60, identified as an ortholog of AtMYB60, was characterized to be involved in stomatal regulation and thus in abiotic stress responses (Galbiati et al. 2011). However, little is known about the specific roles of MYB genes in tomato in response to environmental stresses.
Based on our RNA-seq and microarray analyses, SlMYB genes displayed two distinct expression patterns, i.e. tissue-specific and induced expression patterns. A number of SlMYB genes showed significant responses under stresses of pathogens and high salinity. Our genome-wide analysis and expression profiles of tomato MYB TFs in response to various stresses provide a basis for their further functional characterization with stress tolerance.
A TF can act as an activator or repressor in transcriptional regulation (Latchman 1997). A number of MYB family members may serve as transcriptional repressors in a number of biological processes, such as AtMYB4, a negative regulator of hydroxycinnamic acid metabolism. Interestingly, the repression effect of AtMYB4 is attributed to the C-terminal region, where we detected an EAR motif, thus supporting the possibility that the repressor activity of AtMYB4 might be due to the EAR motif (Jin et al. 2000). In our study, 24 SlMYB proteins containing EAR motifs were identified (Table 2). It is tempting to test their basal transcriptional activities using a yeast system. However, only 14 ORFs of 24 EAR motif-containing SlMYBs were PCR-amplified successfully from the tomato cDNA pool. The difficulties in isolating the remaining full-length genes might be due to the low-abundance cDNA. Therefore, we decided to show the transcriptional activities of five selected genes representing different EAR location categories in Table 2 in our study. The results showed that they all have basal transcriptional activation activities (Fig. 9). We can assume that these proteins might have negative roles in promoting gene transcription in plants where their co-repressors exist. Of course, future biochemical and genetic studies are required to determine the exact functions of the EAR motifs in the 24 SlMYB proteins. Identification of potential interacting partners of the EAR motif and structural characterization of their physical interaction are also essential in understanding their functions. The investigation of EAR motif-containing proteins will not only provide new insight into the link between gene regulation and plant biology but will also provide a novel framework for genetic improvements of crops.
The MYB gene family has been demonstrated to be involved in multiple regulations of plant development and responses to various biotic and abiotic stresses. The information generated here about the structure of SlMYB proteins will shed light on their functional analysis. The comparative, phylogenetic and expression analyses of tomato MYB members will provide in depth insight into comprehensive functional characterization of the MYB gene family for tomato and various other Solanaceae species. The functional exploration of tomato MYBs will also facilitate better understanding of gene regulation in plants by the MYB-type TFs and the development of new varieties and other commercially important plants with metabolic engineering approaches.
Materials and Methods
Database searching and sequence retrieval
To identify potential members of the tomato MYB gene family, we performed multiple database searches. The amino acid sequences of three known tomato R2R3-MYB DBDs (about 108 amino acids; Supplementary Table S1) were used as queries in BLASTP searches against the tomato genome database [http://solgenomics.net/, International Tomato Annotation Group (ITAG) release 2.31]. The maximum number of hits to show was set to 200. The substitution matrix BLOSUM62 was used. All tentative consensus sequences and singlet sequences with an e-value of <1.0 were selected after the homology search. About 160 hits were obtained. Any redundant sequences and sequences including single MYB domain (MYB-related) or somewhat different conserved domains were discarded from the data list. In total, 127 tomato MYB genes (122 R2R3, four 3R and one 4R) were identified. Arabidopsis and rice MYB gene sequences were downloaded from the Arabidopsis genome TAIR (The Arabidopsis Information Resource) release 10.0 (http://www.arabidopsis.org/) and the rice genome annotation database (http://rice.plantbiology.msu.edu/index.shtml, release 7), respectively. All of the sequences retrieved were manually analyzed to confirm the presence of MYB domains using the SMART program (http://smart.embl-heidelberg.de/) (Letunic et al. 2015). In addition, to confirm the authenticity of the identified sequences, they were compared with the cDNA sequences of MYB genes in PlantTFDB (http://planttfdb.cbi.pku.edu.cn) (Jin et al. 2014). In total, 133 (127 R2R3, five 3R and one 4R) and 117 (111 R2R3, five 3R and one 4R) typical MYB genes were identified in Arabidopsis and rice, respectively. The Arabidopsis MYB genes were named according to the previous study and TAIR (Stracke et al. 2001). In order to better reflect the appropriate orthologous relationship between SlMYBs and AtMYBs, each SlMYB was assigned a name based on their phylogenies and sequence similarities corresponding to individual AtMYBs. The 122 R2R3 SlMYBs and 127 R2R3 AtMYBs were first used to contruct a phylogenetic tree (Supplementary Fig. S1). The terminal nodes containing one tomato MYB and one Arabidopsis MYB were used for naming some tomato MYBs which showed a high degree of homology between them. In order to facilitate the name assignment, the newly named SlMYBs and their homologs in Arabidopsis were removed from the tree. Then, earlier nodes containing both SlMYBs and AtMYBs were employed for naming the rest of the SlMYBs based on protein sequence similarities until all the SlMYBs were finally assigned a name (Supplementary Table S3). As regards the rice MYB genes, we provisionally named them OsMYB1 through OsMYB111, OsMYB3R-1 through OsMYB3R-5 and OsMYB4R-1 based on their location on the chromosome (Supplementary Table S3).
WebLogo and gene structure analysis
The distribution of amino acid residues at the corresponding positions in domain profiles for the conserved MYB domains of R2R3-type SlMYBs were generated using the WebLogo program with default parameters (http://weblogo.berkeley.edu/logo.cgi) (Crooks et al. 2004).
For gene structure analysis, the exon and intron structures of individual SlMYB genes were illustrated using the Gene Structure Display Server (GSDS; http://gsds.cbi.pku.edu.cn/) (Hu et al. 2014) by aligning the cDNA sequences with the corresponding genomic DNA sequences from the SGN (http://solgenomics.net/).
Conserved motif identification and gene ontology analysis
The deduced amino acid sequences of the 127 SlMYBs were analyzed by MEME (http://meme-suite.org/tools/meme, version 4.11.0) for motif analysis (Bailey et al. 2015). To identify conserved motifs in these sequences, selection of the maximum number of motifs was set to 40 with a minimum width of six and a maximum width of 120 amino acids, and e-value <1e-8, while other factors were set at default values.
GO enrichment analysis was performed using the agriGO gene ontology enrichment analysis tool (http://bioinfo.cau.edu.cn/agriGO/) (Du et al. 2010). The loci of 127 SlMYBs were used as queries, and S. lycopersicum ITAG2.4 was used as the database for the singular enrichment analysis. The GO term enrichment was calculated using hypergeometric distribution with a P-value cut-off of 0.05 and a term-mapping count cut-off of 5. P-values obtained by Fisher’s exact test were adjusted with the FDR for multiple comparisons to detect over-represented GO terms. GO terms were considered as significantly enriched with both P-values and the FDR at <0.05.
Phylogenetic analysis
The alignments of full-length amino acid sequences of MYBs from tomato, Arabidopsis and rice were performed using ClustalX 2.1 with default settings (Aiyar 1999). Then, an unrooted phylogenetic tree was constructed based on the alignments using MEGA 6.05 with the NJ method (Tamura et al. 2013). The parameters used in the tree construction were JTT model plus gamma-distributed rates determined by ProtTest 3.0 (Darriba et al. 2011) and 1,000 bootstraps. The pairwise gap deletion mode was used to ensure that the more divergent C-terminal domains could contribute to the topology of the NJ tree.
The chromosomal location and duplication of SlMYB genes
SlMYB genes were located on tomato chromosomes according to their positions given in the ITAG release 2.4 in the SGN (http://solgenomics.net). The tandem gene duplications in SlMYB genes were identified based on the investigations described in Arabidopsis (Cannon et al. 2004). The tandemly duplicated genes were defined as an array of two or more SlMYB genes falling within 50 kb of one another. To identify the segmental duplicates, we defined synteny blocks to include conservation of gene content, order and direction between different chromosomes (Du et al. 2012b).
The RNA-seq and microarray data analysis
The tomato RNA-seq and microarray data sets were downloaded from the TFGD (http://ted.bti.cornell.edu) (Fei et al. 2011). For tissue-specific expression analysis of SlMYB genes, the RNA-seq data set D004 was downloaded. The total RNA samples of leaves, roots, flower buds, fully opened flowers and 1, 2 and 3 cm, mature green, breaker and breaker + 10 fruits of tomato cultivar Heinz, and leaves, immature green, breaker and breaker + 5 fruits of Solanum pimpinellifolium were subjected to Illumina RNA-seq analysis. In our study, the sequencing data for leaves, roots, flower buds, fully opened flowers and breaker + 10 fruits of tomato cultivar Heinz were used and the expression results of 127 SlMYB genes were extracted according to their ID numbers. The relative expression level of a particular SlMYB gene was normalized against the mean value among the five selected tissues. The heatmap was constructed using the Gene Cluster 3.0 and Java Treeview software (Page 2002). For the expression analysis of SlMYB genes in response to different bacteria, the file ‘D007_ratio_pvalue’ under RNA-seq data set D007 was downloaded. Treatments with three bacteria, Pst DC3000, P. putida, and P. fluorescens, were analyzed in our study. The 4- to 6-week-old tomato leaves (cultivar Rio Grande prf3) were syringe-infiltrated with different bacteria for 6 h and then subjected to total RNA extraction and RNA-seq analysis. The control sample was syringe-infiltrated with 10 mM MgCl2 for 6 h. An expression ratio >2 (adjusted P-value < 0.05) was considered statistically significant. For salt treatment, Affymetrix microarray data available in the Microarray data warehouse under the series accession number E051 were analyzed. The raw data ‘normalized intensity file’ were downloaded. The salt response of tomato cultivar ‘Moneymaker’ was used in our expression analysis. Six-leaf-stage tomato seedlings were treated with 200 mM NaCl for 5 h. The whole plants were subjected to hybridization. The normalized intensity values from unstressed and stressed Moneymaker were represented by the hybridization ID H0257 and H0258, respectively. The related probe sets of SlMYB genes were identified using the the Probe Match Tool in NetAffx Analysis Center (http://www.affymetrix.com). For probe sets matching several SlMYB gene models, only those exhibiting consistently high hybridization signals across multiple samples were considered. Expression was shown as fold change in stressed samples relative to unstressed samples. An adjusted P-value < 0.05 was used as a cut-off for statistical significance. Tab-delimited files for the average log signal intensity values were imported into Gene Cluster (v3.0) and the heat map was viewed in Java TreeView (Page 2002).
Treatment of tomato and Arabidopsis plants with SA and MeJA
The tomato (cultivar zhongshu 4) seedlings were grown in the greenhouse with long-day conditions (16 h light, 8 h dark) under a temperature of 28°C in light and 18°C in darkness. Before application of the chemicals, the plants were watered as needed. Chemical induction of 3-week-old tomato plants was performed by dipping the leaves in a solution containing 0.015% (v/v) Silwet L-77 and either 1 mM SA or 50 µM MeJA. Control plants were treated with 0.015% Silwet L-77 only (Spoel et al. 2003). Leaves from four individual plants were harvested at three selected time points during SA and MeJA treatment, frozen immediately in liquid nitrogen, and stored at –80°C for further analysis.
Arabidopsis seeds were sterilized in 70% ethanol for 1 min followed by 10% bleach for 15 min and were then rinsed five times with double-distilled water. Sterilized seeds were sown and monitored on half-strength Murashige and Skoog (1/2 MS) solid medium. Chemical treatment of Arabidopsis plants was performed by transferring 2 ml of 1/2 MS liquid medium containing 200 µM SA or 10 µM MeJA to 7-day-old seedlings grown on 1/2 MS solid medium and then incubated at 22°C for 4 h.
Real-time PCR analysis
Total RNA was extracted using the RNeasy Plant Mini Kit (Qiagen) according to the manufacturer’s instructions. RNA integrity was verified by 1.5% agar gel electrophoresis. First-strand cDNA synthesis was carried out with approximately 2 µg of RNA using M-MLV reverse transcriptase (Promega) and random primers according to the suggested procedure. All primer sequences are listed in Supplementary Table S4. Real-time PCR was conducted on a cycler apparatus (Bio-Rad) using a SYBR Premix Ex Taq™ Kit (TAKARA). Reactions were prepared in a total volume of 20 µl containing: 10 µl of 2 × SYBR Premix, 2 µl of cDNA template and 0.5 µl of each specific primer to a final concentration of 200 nM. The reactions were performed under the following conditions: initial denaturation step of 94°C for 30 s followed by a two-step thermal cycling profile of denaturation at 94°C for 10 s, and combined primer annealing/extension at 59°C for 30 s for 40 cycles. The ACTIN2 gene was used as an internal control. The 2–ΔΔCt method was used for the analysis and visualization of our real-time PCR data (Li et al. 2013). At the same time, the SEMs among three replicates were calculated.
GUS histochemical analysis
The promoter regions of SlMYB14, SlMYB17, SlMYB62 and SlMYB66 were PCR amplified from tomato genomic DNA. The PCR products were cloned into the HindIII–BamHI sites of the binary vector pCAMBIA1391s to generate the SlMYBpro:GUS construct. The constructs were sequenced and then transformed into the Agrobacterium tumefaciens strain LA4404, which were used to transform the flower buds of Arabidopsis wild-type Col-0 (Clough and Bent 1998). Transgenic lines were selected on 1/2 MS plates containing 50 µg ml–1 hygromycin. Primers used in the vector construction are listed in Supplementary Table S5.
Plants from independent transgenic Arabidopsis lines, all containing a single copy of the SlMYBpro:GUS construct, were used for histochemical staining of GUS, which was detected according to a previously described method (Jefferson et al. 1987). Whole chemically treated seedlings were soaked in the GUS staining buffer (1 mM X-glucuronide in 100 mM sodium phosphate, pH 7.2, 0.5 mM ferricyanide, 0.5 mM ferrocyanide and 0.1% Triton X-100), subjected briefly to a vacuum and incubated at 37°C in the dark for about 4 h. After being washed with 75% ethanol several times, plants were photographed using a Leica DFC 490 stereomicroscope. Images were processed with Adobe Photoshop CS 8.0.
Transcriptional activation assay
To test the transcriptional activation activities of five SlMYB genes, pGBKT7 vector and yeast strain Y2HGold (Clontech) were used. The full-length coding sequences of SlMYB genes were PCR-amplified from the tomato cDNA pool. The full-length and truncated version of OsMYB103L were PCR-amplified from the rice cDNA pool. The tomato (variety zhongshu 4) and rice (variety zhonghua 11) cDNA pools were prepared from the total RNA isolated from plant materials with Trizol reagent (Invitrogen). First-strand cDNA synthesis was carried using M-MLV reverse transcriptase (Promega) according to the suggested procedure. Enzyme-digested PCR products were cloned into the corresponding sites of the pGBKT7 vector. The methods for yeast assays were based on the Matchmaker two-hybrid system (Clontech). In yeast strain Y2HGold, the four reporter genes His, Ade, AUR1 and MEL1 are under the control of the GAL4-responsive promoter. If the protein used has transactivation potential, the expression of reporter genes could be detected. Empty pGBKT7 vector was used as a blank control. The yeast cells grown on SD/-Trp medium were considered as positive transformants. The positive yeast cells were transferred to liquid SD/-Trp, and then cultured until the OD600 reached 0.5. The suspensions were diluted to the indicated concentrations. A 5 µl aliquot of each yeast cell suspension was inoculated onto the SD plates (SD/-Trp and SD/-Trp/-His/-Ade) and incubated at 30°C for about 28 h. The presence of transactivation activity was confirmed by yeast growth on the SD/-Trp/-His/-Ade plate. The reporter gene MEL-1 encodes α-galactosidase. Yeast colonies that express MEL1 turn blue in the presence of the chromogenic substrate X-α-Gal (4 mg ml–1) (Matchmaker two-hybrid system, Clontech). The colonies for X-α-Gal staining were set up to grow on a different SD medium pre-containing X-α-Gal and incubated in the same manner as described above. Primers used to amplify the five SlMYB genes and OsMYB103L are listed in Supplementary Table S6.
Identification of EAR motif-containing SlMYB proteins
The 127 SlMYB proteins were manually searched to identify candidates with sequences similar to motif DLNxxP or LxLxL (Kagale and Rozwadowski 2011). Specific protein interactions were constructed applying STRING software (http://string-db.org/) (Szklarczyk et al. 2014). Textmining was used for active prediction of protein interactions with medium required confidence 0.400.
Funding
This work was supported by Shanghai Academy of Agricultural Sciences [the Youth Talents Growth Plan (Grant No. 2016-1-17)]; the Shanghai Municipal Committee of Agriculture [Key Project Fund (zhongzi2014-2)]; Agriculture science Technology Achievement Transformation Fund [143919N0300]. The funders had no role in study design, data collection and analysis, decision to publish, or preparation of the manuscript.
Abbreviations
- DBD
DNA-binding domain
- EAR
ethylene-responsive element-binding factor-associated amphiphilic repression
- FDR
false discovery rate
- GO
Gene Ontology
- GUS
β-glucuronidase
- HTH
helix–turn–helix
- ITAG
International Tomato Annotation Group
- JA
jasmonic acid
- MeJA
methyl jasmonate
- MEME
Multiple Em for Motif Elicitation
- MS
Murashige and Skoog
- NJ
Neighbor–Joining
- ORF
open reading frame
- PlantTFDB
Plant Transcription Factor Database
- RNA-seq
RNA-sequencing
- SA
salicylic acid
- SGN
Sol Genomics Network
- SMART
Simple Modular Architecture Research Tool
- STRING
Search Tool for the Retrieval of Interacting Genes/Proteins
- TAIR
The Arabidopsis Information Resource
- TF
transcription factor
- TFGD
Tomato Functional Genomics Database
Acknowledgements
We thank C. Yu and L. Chen for technical support in plant hormone treatment and the use of the stereomicroscope.
Disclosures
The authors have no conflicts of interest to declare.
References