-
PDF
- Split View
-
Views
-
Cite
Cite
Ha Thi Kim Nguyen, Soo Youn Kim, Kwang-Moon Cho, Jong Chan Hong, Jeong Sheop Shin, Hae Jin Kim, A Transcription Factor γMYB1 Binds to the P1BS cis -Element and Activates PLA 2 -γ Expression with its Co-Activator γMYB2 , Plant and Cell Physiology, Volume 57, Issue 4, April 2016, Pages 784–797, https://doi.org/10.1093/pcp/pcw024
- Share Icon Share
Abstract
Phospholipase A 2 (PLA 2 ) hydrolyzes phospholipid molecules to produce two products that are both precursors of second messengers of signaling pathways and signaling molecules per se. Arabidopsis thaliana PLA 2 paralogs ( -β , -γ and -δ ) play critical roles during pollen development, pollen germination and tube growth. In this study, analysis of the PLA 2 -γ promoter using a deletion series revealed that the promoter region –153 to –1 is crucial for its pollen specificity. Using a yeast one-hybrid screening assay with the PLA 2 -γ promoter and an Arabidopsis transcription factor (TF)-only library, we isolated two novel MYB-like TFs belonging to the MYB-CC family, denoted here as γMYB1 and γMYB2. By electrophoretic mobility shift assay, we found that these two TFs bind directly to the P1BS (phosphate starvation response 1-binding sequence) cis -element of the PLA 2 -γ promoter. γMYB1 alone functioned as a transcriptional activator for PLA 2 -γ expression, whereas γMYB2 directly interacted with γMYB1 and enhanced its activation. Overexpression of γMYB1 in the mature pollen grain led to increased expression of not only the PLA 2 -γ gene but also of several genes whose promoters contain the P1BS cis -element and which are involved in the Pi starvation response, phospholipid biosynthesis and sugar synthesis. Based on these results, we suggest that the TF γMYB1 binds to the P1BS cis -element, activates the expression of PLA 2 -γ with the assistance of its co-activator, γMYB2, and regulates the expression of several target genes involved in many plant metabolic reactions.
Introduction
Transcriptional regulation of gene expression plays a pivotal role in plant development and in plant responses to environmental stimuli. The specificity of a gene’s expression largely depends on cis -element(s) present in the gene promoter and the interaction of specific transcription factor(s) (TFs) with the cis -element(s). In many species of plants, a number of pollen-specific cis -elements have been identified, such as GTGA ( Rogers et al. 2001 ), the PB core motif (TGTGGTT) ( Twell et al. 1991 ), two novel co-dependent regulatory elements (AGAAA and TCCACCATA) in the Lat52 promoter ( Bate and Twell 1998 ), a novel pollen-specific cis -acting element (AAATGA) of tobacco ( Weterings et al. 1995 ) and the palindromic DNA sequence in the SBgLR promoter of tomato ( Zhou et al. 2010 ). Although the competence of most pollen-specific promoters has been verified in heterologous transgenic plants, the pollen-specific cis -elements listed above are not universally present in pollen-specific promoters. Also, the presence of known pollen-specific cis -elements in a promoter does not necessarily confer pollen specificity ( Hamilton et al. 1998 ). Thus, it is not simple to make generalizations regarding the true character of pollen-specific elements. Detailed studies on the precise function of cis -element motifs in promoters would improve our understanding of pollen-specific regulation of gene expression during pollen development in plants.
Several extensive transcriptomic data sets from pollen have provided valuable insight into the complexity of gene expression in pollen. In two separate studies of Arabidopsis pollen, 7,235 ( Honys and Twell 2004 ) and 6,587 ( Pina et al. 2005 ) genes were expressed in mature pollen grain. Of these genes, 1,594 belong to 34 gene families encoding putative TFs ( Honys and Twell 2004 ). In addition, of the 1,350 TF genes (85%) represented on the GeneChip Arabidopsis ATH1 Genome Array, 608 (45%) were expressed in the pollen, of which 54 genes (15.7%) were pollen specific. To date, several TFs, such as DUO1, MYB97, MYB101, MYB120 and MADS-box TFs of the MIKC* class, and their target genes are known to be involved in pollen development, maturation and tube growth ( Rotman et al. 2005 , Verelst et al. 2007 , Adamczyk and Fernandez 2009 , Brownfield et al. 2009 , Borg et al. 2011 , Leydon et al. 2013 ). Although several pollen-specific/related promoters and TFs have been reported, little is known about which TFs bind to which pollen-specific cis -elements, and whether those TFs induce or repress genes in a pollen-specific manner. The limited body of knowledge on these processes is one of the major factors contributing to a poor understanding of the association between TFs and pollen development.
Phospholipase A 2 (PLA 2 ) hydrolyzes phospholipid molecules at the sn-2 position to produce lysophospholipid and a free fatty acid. These two products are precursors of second messengers of signaling pathways, and are signaling molecules themselves. Of four low molecular weight PLA 2 s in Arabidopsis, the genes encoding two of them, PLA 2 -γ and PLA 2 -δ, have pollen-specific expression, and, together with PLA 2 -β, they play critical roles in pollen development, germination and pollen tube growth ( Kim et al. 2011 ). Expression of PLA 2 -γ mRNA gradually increases during three different pollen development stages (microspore, bicellular and tricellular stage) and remains high in mature pollen, germinated pollen and the pollen tube. On the other hand, compared with PLA 2 -γ , PLA 2 -δ has lower expression and is detected later, from the tricellular stage to mature pollen, germinated pollen and the pollen tube ( Kim et al. 2011 ). To date, the mechanism regulating expression of PLA 2 -γ and PLA 2 -δ in pollen has not been characterized. Moreover, none of the TFs that bind to promoters of the PLA 2 genes to control their expression during pollen development has been identified. Because of the dominant expression of PLA 2 -γ in mature pollen, we characterized the features of the PLA 2 -γ promoter and binding TFs in this study.
We performed a deletion series analysis of the PLA 2 -γ promoter to identify the minimal region critical for its specific expression in pollen grains and to identify the regions containing cis -element(s) bound by activator(s). Two putative MYB-like TFs that bind to the PLA 2 -γ promoter were identified using a yeast one-hybrid screen with an Arabidopsis TF cDNA library; these were named γMYB1 (At2g20400) and γMYB2 (At3g13040). We then characterized their ability to bind to the PLA 2 -γ promoter, identified the specific cis -elements to which they bind and investigated how they co-operate to control the expression of the PLA 2 -γ gene in mature pollen. Additionally, we investigated whether γMYB1 induces other target genes with promoters containing the specific cis -element that we identified.
Results
Identification of cis -elements in the PLA 2 -γ promoter and its core sequences
To identify putative cis -elements that regulate gene expression and pollen specificity of the PLA 2 -γ promoter, we used PLACE ( https://sogo.dna.affrc.go.jp/cgi-bin/sogo.cgi ), a database of plant cis -acting regulatory elements ( Higo et al. 1999 ), to analyze the 660 bp PLA 2 -γ promoter region. The PLA 2 -γ promoter region contains the 3′-untranslated region (UTR) of the preceding PLA 2 -δ gene and the 5′-UTR of PLA 2 -γ ( Fig. 1 A). We identified a large number of putative cis -elements, as shown in Supplementary Fig. S1 .

Histochemical GUS staining and fluorometric assay of the PLA 2 -γ promoter. (A) Diagram of the PLA 2 -δ and PLA 2 -γ genomic region. The gray dotted lines indicate the untranslated regions, the black boxes indicate the exons, and black bold lines indicate the introns. (B) Diagram of the PLA 2 -γ promoter and its 5′-deletion series. Numbers indicate the distance in nucleotides from the translational start codon. (C) Histochemical GUS staining of flowers of three independent T 3 homozygous Arabidopsis lines transformed with ProPLA 2 -γ : GUS or its deletion constructs. Flowers in this figure display the representative phenotypes of each line. (D) Fluorometric GUS activity assay of flowers in three independent T 3 transgenic lines. Flowers of a wild-type plant were used as a negative control. Error bars show ± SD ( n = 3).
We analyzed a deletion series of the PLA 2 -γ promoter by making fusion constructs for β-glucuronidase (GUS) expression [ ProPLA 2 -γ:GUS (full length, 660 bp), ProPLA 2 -γA:GUS (421 bp), ProPLA 2 -γB:GUS (260 bp) and ProPLA 2 -γC:GUS (153 bp)]. We observed that GUS activity decreased with the first two deletions in the promoter ( Fig. 1 B–D). The substantial reduction of GUS activity in the T 3 line with the ProPLA 2 -γB construct implies that positive cis -regulatory elements are located in the region from –421 to –260. In this region, two MYBCOREs (CNGTTR; N = A/T/G/C, R = A/G) and one MYBGAHV were located at three positions, –413, –316 and –274, respectively ( Supplementary Fig. S1 ). The comparable GUS activity in T 3 lines with both ProPLA 2 -γB:GUS and ProPLA 2 -γC:GUS constructs indicates the absence of a cis -element between positions –260 and –153 ( Fig. 1 D), which is supported by the analysis with the PLACE database ( Supplementary Fig. S1 ). However, the pollen specificity of the PLA 2 -γ promoter was not altered by partial deletion of the promoter ( Fig. 1 C; Supplementary Fig. S2 ), demonstrating that the minimal promoter region of ProPLA 2 -γC is sufficient to determine the specific expression of PLA 2 -γ in pollen. Several cis -elements were identified in this PLA 2 -γC region. MYBPZM and MYBST1, binding sites for MYB family proteins and MybSt1, respectively, are positioned at –110 and –97. A P1BS motif (GNATATNC; dotted box in Supplementary Fig. S1 ), which is the PHR1 (phosphate starvation response 1)-binding sequence ( Rubio et al. 2001 ), is located at position –97. This motif is known to exist in the promoters of the phosphate starvation-responsive genes in many plant species and is a common cis -regulatory motif controlling genes that are highly induced by phosphate (Pi) starvation ( Bustos et al. 2010 , Sobkowiak et al. 2012 ). One CArG consensus sequence (CCW 6 GG), the binding site for the MADS domain proteins, is located at position –106. Moreover, at position–6 and –124, there are two POLLEN1LELAT52 cis -elements (AGAAA) that function in a co-dependent manner with the TCCACCAT element to regulate the pollen-specific activation of the tomato Lat52 gene ( Bate and Twell 1998 ). It remains to be determined whether those cis -elements located within the ProPLA 2 -γC region are responsible for the pollen-specific expression of PLA 2 -γ.
Identification of TFs binding to the PLA 2 -γ promoter
To isolate TFs that bind to the promoter and possibly regulate PLA 2 -γ expression, we performed a yeast one-hybrid screen using the 660 bp full-length promoter of PLA 2 -γ as bait and an Arabidopsis TF cDNA expression library as prey ( Welchen et al. 2009 ). From a total of 6 × 10 5 transformed yeast clones, 162 colonies grew on the selection medium, indicating that the TF interacted with the PLA 2 -γ promoter. Approximately 50% of the plasmids from these colonies contained At2g20400 and At3g13040 genes as the prey. These two genes encode proteins that belong to the MYB-coiled-coil (MYB-CC) group of TFs ( Bustos et al. 2010 ) that contain a single MYB domain and a coiled-coil domain (CC-domain) ( Supplementary Fig. S3 ). This finding was not unexpected, as the PLA 2 -γ promoter includes several MYB-binding sites. We designated these two TFs as γMYB1 (At2g20400) and γMYB2 (At3g13040). Searching the NCBI protein database with their deduced amino acid sequences ( http://www.ncbi.nlm.nih.gov/BLAST/ ) revealed that γMYB1 and γMYB2 had the highest homology with PHR1 and PHR1-like (PHL1), which are key regulatory components of the Pi starvation stress response in Arabidopsis ( Rubio et al. 2001 , Bustos et al. 2010 ). The overall amino acid sequence identity between γMYB1 and PHR1 was 54%, and the sequence identity between γMYB1 and PHL1 was 38%, while the core MYB domain including the helix–turn–helix motif had amino acid sequence identities of 86% and 79%, respectively. Additionally, the overall amino acid sequence identity between γMYB2 and PHR1 was 38%, and between γMYB2 and PHL1 was 35%, while the core MYB domains both showed 77% sequence identity. The amino acid sequence identity between γMYB1 and γMYB2 was 35% overall and 75% in the MYB domain. Together with this single MYB DNA-binding domain, these four proteins had a second conserved domain predicted to form a coiled-coil, which is a fold usually involved in protein–protein interactions.
Spatial expression and nuclear localization of putative TFs
We examined the spatial and temporal expression of the γMYB1 and γMYB2 TF genes by analyzing GUS activity driven by their promoters. Using 1,405 bp of the γMYB1 promoter region, including the 5′-UTR ( ProγMYB1:GUS ; Supplementary Fig. S4B ), GUS expression was detected in all tissues ( Fig. 2 A–H; Supplementary Fig. S4C ), which is consistent with quantitative reverse transcription–PCR (qRT–PCR) results ( Fig. 2 R). In contrast, no GUS expression was observed in any tissue when GUS was driven by the γMYB1 promoter without the 5′-UTR ( ProγMYB1ΔUTR:GUS ; Supplementary Fig. S4D, E ). Notably, the expression of γMYB1 was the highest in the open flower and was detectable in pollen ( Fig. 2 R) where the PLA 2 -γ gene is specifically expressed ( Kim et al. 2011 ). Using the promoter of γMYB2 ( ProγMYB2:GUS ), GUS expression was observed in all tested tissue ( Fig. 2 I–Q; Supplementary Fig. S4H ). The transcript level of γMYB2 was investigated by qRT–PCR assay ( Fig. 2 S). Even though the level was very low in pollen, GUS was detected in mature pollen ( Fig. 2 P; Supplementary Fig. S4I ). Although γMYB1 and γMYB2 are expressed in pollen and may control the PLA 2 -γ expression there, the widespread expression of these TFs suggests that other factors contribute to pollen-specific expression of PLA 2 -γ.
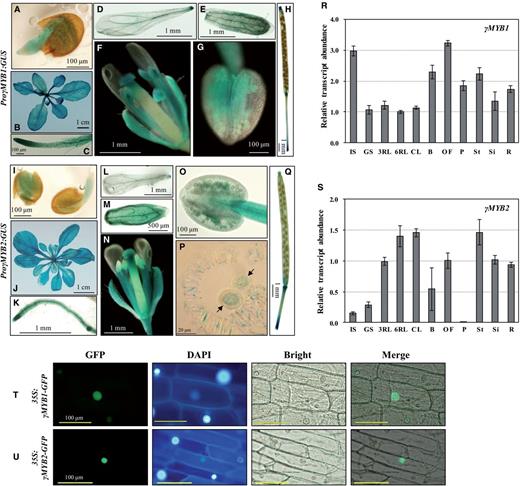
Spatial expression patterns and subcellular localization of γMYB1 and γMYB2. (A–H) GUS expression of the ProγMYB1:GUS lines. (A) One-day germinated seed, (B) 4-week-old plant, (C) root, (D) petal, (E) sepal, (F) flower at stage 14, (G) anther and (H) silique. (I–Q) GUS expression of the ProγMYB2:GUS lines. (I) One-day germinated seeds, (J) 6-week-old plant, (K) root, (L) petal, (M) sepal, (N) flower at stage 14, (O) anther, (P) mature pollen (black arrows) in the anther sac and (Q) silique. (R and S) Expression levels of γ MYB1 (R) and γ MYB2 (S) in diverse organs analyzed by qRT–PCR. Relative amounts of transcripts were calculated by the comparative CT (threshold cycle number at the cross-point between amplification plot and threshold) method, and values were normalized to the internal control. Bars show means ± SD ( n = 3). IS, 1-day-imbibed seed; GS, 1-day-germinated seeds; 3RL, 3-week-old rosette leaf; 6RL, 6-week-old rosette leaf; CL, 6-week-old cauline leaf; B, bud; OF, open flower; P, pollen; St, stem; Si, silique; R, root. (T) Nuclear localization of 35S:γMYB1-GFP in onion epidermal cells. (U) Nuclear localization of 35S:γMYB2-GFP in onion epidermal cells. GFP, GFP fluorescence; DAPI, 4′,6-diamidino-2-phenylindole staining of the nucleus; Bright, bright-field image; Merge, merged GFP and bright-field image.
To demonstrate the subcellular localizations of γMYB1 and γMYB2, 35S:γMYB1-GFP and 35S:γMYB2-GFP constructs were introduced into onion epidermal cells for fluorescence microscopy. We identified both γMYB1 and γMYB2 localized to the nuclei, as expected ( Fig. 2 T, U).
γMYB1 and γMYB2 directly bind to the P1BS cis -element in the PLA 2 -γ promoter
To verify that γMYB1 and γMYB2 bind to the PLA 2 -γ promoter, we performed an elctrophoretic mobility shift assay (EMSA) using the truncated forms of γMYB1 and γMYB2 that only contain the MYB-binding domain and CC-domain (note: the truncated MYB protein containing the intact MYB domain does not lose its specificity; Ramsay et al. 1992 , Li and Parish 1995 , Phan et al. 2011 ). As several types of MYB recognition sequences are present in the PLA 2 -γ promoter, the probes were designed by splitting the promoter region into six approximately equal sized fragments (100–120 bp) without interrupting putative MYB-binding sites, and named ProPLA 2 -γP1 to ProPLA 2 -γP6 ( Fig. 3 A; referred to as P1–P6 in the text).

EMSA of γMYB1 and γMYB2 binding to PLA 2 -γ? promoter fragments. (A) Diagram of P1–P6 fragments of the PLA 2 -γ promoter used for the EMSA. ProPLA 2 -γP6 is divided into five (a–e) fragments. The positions of individual fragments from the translational start codon are shown as numbers above or below the line. (B) EMSA with γMYB1 performed with 32 P-end-labeled promoter fragments P4 and P6 . The free probe of P4 was run out of the gel (C) EMSA of γMYB1 or γMYB2 with segments of P6 . The biotin-labeled probes were mixed with MYB and CC-domain proteins of either γMYB1 or γMYB2. (D) EMSA of γMYB1 or γMYB2 with segment P6_b with a specific probe competitor (non-labeled probe). (E) EMSA of γMYB1 or γMYB2 with mutated P6_b ( P6_mb ). Black arrows indicate the shifted bands; white arrows indicate reduced intensity of the shifted bands; white triangles indicate non-specific bands; a white star indicates the free probe of P6 .
First, we tested whether γMYB1 protein could bind to PLA 2 -γ promoter segments. In this EMSA, γMYB1 bound to probes P4 and P6 (black arrows in Fig. 3 B; Supplementary Fig. S5A ), and the addition of an unlabeled competitor probe to the reaction reduced or abolished the binding of γMYB1 to these probes (white arrows in Fig. 3 B). Secondly, to identify the specific cis -elements to which γMYB1 and γMYB2 bind, we made smaller biotin-labeled fragments of P4 and P6 as probes ( Fig. 3 A; Supplementary Fig. S5B ). Both γMYB1 and γMYB2 bound to the P6_b region ( Fig. 3 A, C, D), as evidenced by the reduced target bands (black arrows) following the addition of competitors to the reaction ( Fig. 3 D). The P4_a region contained MYBCORE (CCGTTG), the P4_b region contained the calcium response cis -element ABRERATCAL, and the P4_c region included MYBGAHV (AACAAA) ( Supplementary Fig. S5B ). We observed very weak binding activity of γMYB1 to all three probes of P4 (white arrow in Supplementary Fig. S5C ), while γMYB2 did not bind P4 at all. Since the binding between γMYB1 and P6 regions showed non-specific bands (white triangles in Fig. 3 C), it is suspected that the weak binding between P4 regions and γMYB1 might also be an artifact. γMYB1 and γMYB2 share the highest homology with PHR1 and PHL1, and the P6_b region contains a P1BS cis -element ( Fig. 3 A, bold dotted box: GGATATTC); thus, we further analyzed the binding of γMYB1 and γMYB2 to P6_b by mutating the P1BS sequence to TTCGCGGA (designated as ProPLA 2 -γP6_mb ). The EMSA results revealed that these two TFs cannot bind to the mutated P6 _ b , thereby confirming that γMYB1 and γMYB2 bind directly to the P1BS cis -element ( Fig. 3 E).
Activity of the activator γMYB1 is enhanced by the co-activator γMYB2
To determine whether γMYB1 and γMYB2 can function as activators of transcription, we used a transactivation assay ( Fig. 4 ). GAL4(3×):LUC was used as a reporter with a triplicate yeast GAL4-binding site fused to a minimal TATA box and a luciferase gene from the firefly Photinus pyralis ( Gould and Subramani 1988 ). The GAL4 DNA-binding domain (GAL4DB) fused to all TFs (γMYB1 γMYB2, and VP16), which were under the control of the 35S promoter. These TFs bind to GAL4(3×):LUC via the interaction between the GAL4DB and the GAL4 site, and control the expression of the LUC gene. The Pro35S:GAL4DB-γMYB1 construct resulted in high luciferase activity, which was even stronger than the positive control containing the VP16 domain. In contrast, Pro35S:GAL4DB-γMYB2 did not show an activation effect on luciferase activity ( Fig. 4 A). This result indicates that γMYB1 functions as a strong transcriptional activator, whereas γMYB2 does not.
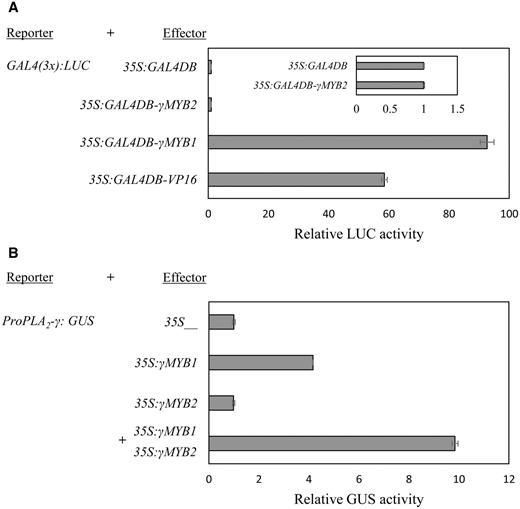
Transactivation by γMYB1 and γMYB2. (A) Transactivation by GAL4–γMYB1 and GAL4–γMYB2 fusion protein in Arabidopsis protoplasts. GAL4(3×)-LUC is the reporter. 35S:GUS was used as the internal control, and the relative luciferase (LUC) activity was normalized to GUS activity. The luciferase activity by Pro35S:GAL4DB was set at 1.0. Pro35S:GAL4DB-VP16 is used as a positive control. Bars show means ± SD ( n = 3). (B) Transactivation of PLA 2 -γ by γMYB1 and γMYB2 in tobacco protoplasts. ProPLA 2 -γ:GUS is the reporter. 35SγMYB1 and 35S:γMYB2 are effectors. 35S:LUC was used as the internal control. The relative GUS activity was normalized to the activity of LUC. The GUS activity by the vector containing only the 35S promoter was set as 1.0. Bars show means ± SD ( n = 3).
The observations that γMYB1 and γMYB2 bind to the PLA 2 -γ p romoter and that γMYB1 was a transcriptional activator led to the question of whether γMYB1 binding can activate GUS expression driven by the PLA 2 -γ promoter. By the GUS activity assay shown in Fig. 4 B, we confirmed that γMYB1 alone, but not γMYB2 alone, was able to activate the PLA 2 -γ promoter and that co-expression of γMYB1 and γMYB2 increased activation by 2.5-fold compared with that with only γMYB1. Therefore, we conclude that γMYB2 alone does not act as either a repressor or an activator, but rather it enhances the activity of γMYB1, which is a transcription activator. It is therefore possible that the two TFs interact, a notion supported by the presence of the CC-domain in both TFs.
To assess whether γMYB1 and γMYB2 physically interact, we performed a bimolecular fluorescence complementation (BiFC) assay ( Fig. 5 A). For this analysis, γMYB1 and γMYB2 were fused with sequences encoding the N-terminal fragment of yellow fluorescent protein (YFP; YN) and the C-terminal fragment of YFP (YC). After co-transforming coupled constructs carrying γMYB1-YN and γMYB2-YC or γMYB2-YN and γMYB1-YC into Arabidopsis leaf mesophyll protoplasts, we detected the YFP signal throughout the nucleus, indicating that γMYB1 and γMYB2 interacted with each other. Moreover, BiFC assays also revealed that γMYB2 formed a homodimer ( γMYB2-YN and γMYB2-YC ) but γMYB1 did not ( γMYB1-YN and γMYB1-YC ).
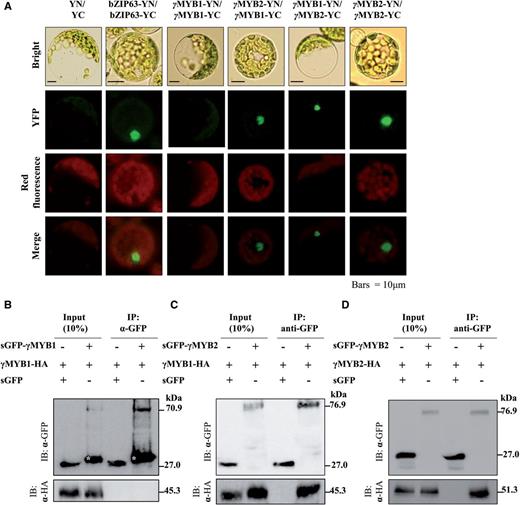
The homomeric and heteromeric interaction of γMYB1 and γMYB2. (A) BiFC assay of γMYB1 and γMYB2 in Arabidopsis mesophyll protoplasts. The bZIP63-YN and bZIP63-YC plasmids are the positive control. The empty vectors pSPYNE and pSPYCE are the negative control (YN/YC). YFP, yellow fluorescent protein; red fluorescence, Chl autofluorescence; Bright, bright-field image; Merge, merged YFP and red fluorescence image. Scale bar = 10 µm. (B–D) Immunoprecipitation assay of γMYB1 and γMYB. sGFP (synthetic green fluorescent protein) and HA (hemagglutinin) fused γMYB1 and γMYB from Arabidopsis mesophyll protoplasts are precipitated with sGFP antibody for immunoprecipitation. Western blots were detected with α-HA and α-sGFP antibody. White stars indicate bands of truncated size of sGFP–γMYB1.
To confirm the BiFC results, we employed a protein complex immunoprecipitation assay ( Fig. 5 B–D). We transformed constructs for synthetic green fluorescent protein (sGFP)–γMYB1 and γMYB1-hemagglutinin (HA) ( Fig. 5 B), sGFP–γMYB2 and γMYB1-HA ( Fig. 5 C) or sGFP–γMYB2 and γMYB2-HA ( Fig. 5 D) into Arabidopsis leaf mesophyll protoplasts. The protein extracts were precipitated with α-GFP antibody and then blotted with α-GFP antibody or α-HA antibody. We detected γMYB1-HA and γMYB2-HA by blotting with α-HA antibody in α-GFP antibody-precipitated protein complexes sGFP–γMYB2/γMYB1-HA ( Fig. 5 C) and sGFP–γMYB2/γMYB2-HA ( Fig. 5 D), but not in complex sGFP–γMYB1/γMYB1-HA ( Fig. 5 B). Taken together, we confirmed that γMYB1 interacts with γMYB2 in vivo and we demonstrate that γMYB1 interacts with its co-activator γMYB2 and this interaction enhances the activation of PLA 2 -γ expression by γMYB1 alone.
Overexpression of γMYB1 induces the expression of genes driven by the P1BS element-containing promoter
Three γMYB1 -overexpression and two γMYB2 -overexpression lines, driven by a pollen-specific promoter, ProLat52 ( Twell et al. 1989 ), were generated to study the roles of γMYB1 and γMYB2 in gene expression ( Fig. 6 ). Analysis of the expression levels of γMYB1 , γMYB2 and PLA 2 -γ using total RNA from mature pollen grains of γMYB1 -overexpression lines and γMYB2 -overexpression lines revealed that the expression of PLA 2 -γ increased in the γMYB1 -overexpression lines ( Fig. 6 A, C). However, γMYB2 overexpression did not affect the expression of PLA 2 -γ ( Fig. 6 B, D). These results are consistent with those of the transient assay showing that γMYB1 activated the expression of the PLA 2 -γ promoter but γMYB2 did not. To test if γMYB1 and γMYB2 activate expression of the other PLA 2 genes, we measured their expression ( PLA 2 -α , -β and -δ ) in the pollen grains of the γMYB1 - and γ MYB2 -overexpression lines, but expression of these genes was not consistently increased or decreased in the individual overexpression lines ( Supplementary Fig. S6 ). This result indicate that γMYB1 and γMYB2 might not activate the expression of PLA 2 -α , -β and -δ whose promoters do not contain the P1BS cis -element.

Expression of genes with P1BS cis -element-containing promoters in mature pollen from plants overexpressing γMYB1 or γMYB2. qRT–PCR was performed using cDNAs isolated from mature pollen grains of wild-type, Lat52:γMYB1 and Lat52:γMYB2. eIF4a1 is used as an internal control. Relative amounts of transcripts were calculated by the comparative CT (threshold cycle number at the cross-point between amplification plot and threshold) method, and values were normalized to the internal control. Bars show means ± SD ( n = 3, biological replicates). Statistical analysis was determined using one-way analysis of variance followed by a Dunnett test. Asterisks indicate significant differences from the wild type (* P < 0.05, ** P < 0.01, *** P < 0.001). γMYB1 , At2g20400; γMYB2 , At3g13040; PLA 2 -γ , At4g29460; CCT1 , phosphorylcholine cytidylyltransferase 1 (At2g32260); SPX1 , protein containing SPX domain 1 (At5g20150), SPX2 , protein containing SPX domain 2 (At2g26660), SPS2F , sucrose phosphate synthase 2F (At5g11110); ISA2 , isoamylase 2, (At1g03310); EMF1 , embryonic flower 1 (At5g11530).
Because γMYB1 binds to the region of the PLA 2 -γ promoter that contains the P1BS cis -element (GGATATTC), we used the RSAT tool ( http://rsat.ulb.ac.be/ ) ( van Helden 2003 ) to find other gene promoters with this element. Of 132 genes that had GGATATTC within 400 bp upstream of the start codon, we selected 10 genes known to be related to pollen development, Pi starvation, sugar synthesis or phospholipid synthesis and metabolism ( Supplementary Table S1 ). We use qRT–PCR to measure expression levels of these 10 genes in mature pollen grains of ProLat52:γMYB1 overexpression lines and the wild type. Six genes ( SPX1 , SPX2 , CCT1 , SPS2F , ISA2 and EMF1 ) had higher expression in the γMYB1 -overexpression lines than in the wild type ( Fig. 6 E–J). Taken together, our findings imply that γMYB1 directly binds to the P1BS cis -element in the promoters of these target genes and thereby regulates their expression.
We generated knock-down lines of γMYB1 by artificial microRNA (amiRNA), driven by the Lat52 promoter. Although the transcript level of γMYB1 decreased in pollen by 60–80%, the PLA 2 -γ transcript level only slightly decreased in pollen relative to the wild type ( Supplementary Fig. S7 ). The phenotype of multiple γMYB1 amiRNA lines was normal. This result suggests that the remaining ?γMYB1 transcripts provided enough protein for near-normal function.
Discussion
A short promoter region controls pollen specificity of PLA 2 -γ expression
By analyzing GUS activity using a deletion series of the PLA 2 -γ promoter ( Fig. 1 ), we found that the promoter C region (from –153 to –1) is crucial for pollen-specific expression of PLA 2 -γ. In other studies, cis -acting elements that control pollen-expressed genes are quite close to the transcription start site, within a 50 bp region upstream ( Carpenter et al. 1992 , Hamilton et al. 1992 , Dzelzkalns et al. 1993 , Eyal et al. 1995 , Tebbutt and Lonsdale 1995 ). Many different putative cis -acting elements, such as MYBPZM, MYBST, P1BS, CArG consensus and POLLEN1LELAT52, are present in the PLA 2 -γ promoter –153 to –1 region. Two TFs, γMYB1 and γMYB2, can bind to the P1BS element in the region between positions –97 and –90, and the expression of PLA 2 -γ was activated by these TFs ( Figs. 3 , 4 , 6 ). These results implied that P1BS contributes to PLA 2 -γ expression in pollen. P1BS is the only known cis -element for the Pi starvation response and can interact with two TFs, PHR1 and PHL1 ( Bustos et al. 2010 ). P1BS appears in promoters of a large number of Pi starvation response genes including genes involved in phospholipid degradation, which is controlled by PHR1 ( Pant et al. 2015 ). In Arabidopsis, only one Patatin-like PLA 2 A involved in lipid remodeling is known to be controlled by PHR1 under Pi starvation ( Ryu 2004 , Pant et al. 2015 ). PLA 2 is involved in phospholipid degradation ( Bahn et al. 2003 , Lee et al. 2003 , Lee et al. 2010 , Kim et al. 2011 ). However, further study is needed to explore whether or not P1BS is crucial for PLA 2 -γ expression during pollen development or under Pi starvation. Moreover, the widespread expression of γMYB1 and γMYB2 in Arabidopsis suggests that other factors might contribute to pollen-specific expression of PLA 2 -γ. We do not rule out the possibility that other MYB-binding sites, such as MYBPZM, MYBST and CArG, could be responsible for forming the pollen-specific cis -regulatory element region, and that this region can be occupied by other, as yet unknown, regulator(s) of pollen-specific expression of PLA 2 -γ.
γMYB1–γMYB2 heterodimer enhances PLA 2 -γ expression activated by γMYB1 alone
In this study, by performing in vivo assays, we showed that γMYB1 and γMYB2 also interact with each other ( Fig. 5 A, C), γMYB2 forms a homodimer ( Fig. 5 A, D) whereas γMYB1 does not ( Fig 5 A, B). sGFP–γMYB2 was more stable than sGFP–γMYB1, since the sGFP–γMYB1 fusion protein always had bands of truncated size (white stars in Fig. 5 B) under the bands with the expected size. The γMYB2 protein contains a sumoylation site to interact with SUMO (small ubiquitin-like modifier) proteins ( Nilsson et al. 2010 ). This interaction might increase protein stability, modify transcriptional activity and change the protein interaction ability ( Hay 2005 , Nilsson et al. 2010 ). Two questions were raised by the presence of a sumoylation site in γMYB2 and the formation of a heterodimer with γMYB1 and a homodimer itself: (i) whether those attributes can affect the stability of γMYB2 or not, and (ii) whether γMYB2 can stabilize γMYB1 by interacting with γMYB1. We are further investigating these questions.
Of 15 proteins of the MYB-CC group, only PHR1 and PHL1 form a dimer to bind to the P1BS cis -element found in the promoters of Pi starvation-responsive genes ( Bustos et al. 2010 ). In this study, EMSA and immunoprecipitation provided evidence for a direct and specific binding of a heterodimer of γMYB1–γMYB2 to the P1BS of the PLA 2 - γ promoter ( Figs. 3 D, 4, 5). γMYB1 alone, but not γMYB2 alone, acts as an activator on the PLA 2 - γ promoter, while the γMYB1–γMYB2 complex increased the GUS expression by 2.5-fold compared with that with only γMYB1 ( Fig. 4 B). This suggests that the γMYB1–γMYB2 heterodimer might play a role to stabilize γMYB1, which then enhances the activity of γMYB1 in the regulation of PLA 2 -γ expression. EMSA also indicated binding of γMYB1 to the P4 fragment (–329 to –214), although we could not find any specific cis -elements in P4 that are bound by γMYB1. The binding of γMYB1 to the P4 fragment possibly explains the large decrease in GUS activity with ProPLA 2 -γB (–260 to –1) compared with ProPLA 2 -γA (–421 to –1) ( Fig. 1 B, D). We conclude that γMYB1, together with its co-activator γMYB2, directly activates the expression of PLA 2 -γ in mature pollen.
To provide more supporting data for a role for γMYB1 in activating PLA 2 -γ expression, we tried to suppress? γMYB1 in pollen in order to reduce the PLA 2 -γ expression with amiRNA. Because the γMYB1 transcripts were still detected in amiRNA lines, γMYB1 protein was possibly still present in pollen. Also, we cannot exclude the possibility that γMYB1 interacts with other TFs in addition to γMYB2 to regulate the expression of PLA 2 -γ. We used an Arabidopsis TF-only library harboring 1,050 TF genes for the yeast one-hybrid assay because it was the only TF gene library available at the time of our initial screening. Given the 1,922 loci for TFs in the Arabidopsis thaliana TF database ( http://datf.cbi.pku.edu.cn/ ), the existence of other protein(s) associated with γMYB1 and γMYB2 and/or other TFs regulating PLA 2 -γ expression should not be ruled out. The PLA 2 -γ? knockout shows normal pollen development because the PLA 2 activity of PLA 2 -β and -δ can compensate ( Kim et al. 2011 ). The abnormal pollen phenotype as described in Kim et al. (2011) occurs only when all three genes, PLA 2 -α , -β and -δ , are suppressed together in pollen. We showed that γMYB1 controls the expression of PLA 2 -γ but no other PLA 2 paralogs ( Supplementary Fig. S6 ). Therefore, the γMYB1 knock-down or knock-out lines could not have any abnormal phenotypes related directly to PLA 2 -γ expression. Although, in this study, results with γMYB1 do not explain the pollen-specific expression of PLA 2 -γ , they shed light on the mechanism of controlling the general expression of PLA 2 -γ in mature pollen.
In a previous study, we hypothesized that PLA 2 modulates membrane deformation and enables membrane trafficking during pollen development and tube germination ( Kim et al. 2011 ). The remodeling of the lipid composition of the membrane is one of the most dramatic metabolic responses to Pi starvation ( Essigmann et al. 1998 , Jouhet et al. 2003 , Yamaryo et al. 2008 , Pant et al. 2015 ). However, the regulation of lipid remodeling has remained elusive. In the MYB-CC group, PHR1 and PHL1 are the best known key regulatory components of the Pi starvation stress response in Arabidopsis ( Rubio et al. 2001 , Bustos et al. 2010 ). PHR1 largely controls the lipid remodeling in shoots and roots, and additional factors probably control lipid metabolism during Pi starvation, since a PHR-independent response occurs at the levels of both gene expression and lipid composition ( Pant et al. 2015 ). There has been no report about how Pi starvation affects the expression of PLA 2 genes or the lipid composition during pollen development, or which mechanism controls this response. Since γMYB1 and γMYB2 are also expressed in whole-plant tissues ( Fig. 2 ), this raised the question of whether or not the γMYB1–γMYB2 complex controls lipid metabolism during Pi starvation in all tissues including pollen.
γMYB1 directly and specifically bound to the P1BS Pi starvation cis -element, suggesting that it may control the expression of genes with P1BS in their promoters. We identified the promoters of at least six genes as possible direct targets of γMYB1 ( Fig. 6 ). Of these six genes, SPX1 (At5g20150) is strongly induced but SPX2 (At2g26660) is weakly induced by Pi starvation ( Duan et al. 2008 ). Since the expression of SPX1 and SPX2 increased in the pollen grains of the ProLat52:γMYB1 overexpression lines but did not increase in the vegetative tissues of the Pro35S:γMYB1 overexpression lines ( Supplementary Fig. S8 ), we postulate that γMYB1 is involved in the expression of SPX1 and SPX2 during Pi starvation in pollen. Overexpression of γMYB1 in mature pollen also leads to increased expression of CCT1 (At2g32260), which encodes CTP:phosphorylcholine cytidylyltransferase, a regulator of phosphastidylcholine (PC) biosynthesis via the CDP-choline pathway ( Inatsugi et al. 2009 ). Although the function of CCT1 during pollen development still needs to be elucidated, it is possible that γMYB1 is not only related to PC editing via activation of PLA 2 -γ but is also involved in PC biosynthesis via its regulation of CCT1 expression. γMYB1 overexpression in pollen also increased the expression level of the SPS2F gene (At5g11110) that encodes sucrose phosphate synthase in the sucrose biosynthesis pathway ( Kaplan et al. 2007 ). Since SPS2F contributes to the formation of dense baculae in the pollen exine during pollen development ( Suzuki et al. 2008 ), we presume that γMYB1 functions in pollen development by controlling the expression of both PLA 2 -γ and SPS2F.
In summary, we suggest that γMYB1 activates the expression of PLA 2 -γ by binding to the P1BS cis -element, and that this activation can be further enhanced with its co-activator γMYB2. We also suggest that this TF additionally regulates the expression of several target genes, whose promoters contain a P1BS cis -element, and whose protein products are involved in many metabolic reactions in plants. Our findings in this study provide new clues to understand gene expression in mature pollen.
Materials and Methods
Plant materials, growth conditions and construction of transgenic lines
Arabidopsis thaliana (ecotype Col-0) plants were grown in potting mix (Sunshine Mix #5; Sun Gro Horticulture) at 22°C, 50% humidity in a growth chamber under long-day conditions (16/8 h, light/dark) after first exposing the seeds to a period of cold (4 d at 4°C in the dark) to expedite germination. Nicotiana benthamiana plants were grown in soil (Sunshine Mix #5; Sun Gro Horticulture) at 25°C, 50% humidity in a growth chamber under long-day conditions (16/8 h, light/dark).
The cassettes containing promoters and target genes were introduced into Arabidopsis plants by the floral dip method ( Clough and Bent 1998 ) using Agrobacterium tumefaciens strain GV3101 ( Koncz and Schell 1986 ). Transgenic plants were selected on 1 × MS ( Murashige and Skoog 1962 ) medium containing 250 mg l −1 carbenicillin and 50 mg l –1 kanamycin or 70 mg l –1 gentamicin, and then transferred to potting mix. T 3 homozygous lines were selected for further study. For all experiments using the protoplast system, plasmid constructs were introduced into Arabidopsis or N. benthamiana protoplasts by the polyethylene glycol (PEG) method ( Yoo et al. 2007 ).
RNA isolation and real-time PCR
Mature pollen grains were harvested from open flowers (stage 13–14) as described previously by Johnson-Brousseau and McCormick (2004) and Huang et al. (1997) . Total RNA was isolated using TRI REAGENT ® (Molecular Research Center) and treated with DNase (Invitrogen) according to the manufacturer’s instructions. Total RNA samples (5 µg) were reverse transcribed using the Transcriptor First Strand cDNA Synthesis kit (Roche Applied Science) in a 20 µl reaction. Primers used for qRT–PCR and their sequences are listed in Supplementary Table S4 . Real-time PCRs contained 20 ng of cDNA in a total volume of 20 µl and 200 nM of each primer, and used the KAPA SYBR ® FAST qPCR kit (Kapa Biosystems). Quantitative PCR was performed in a LightCycler ® 480 II system (Roche Applied Science). The PCR cycling conditions consisted of 45 cycles of 95°C for 10 s, 55°C for 10 s and 7°C for 20 s. C T (threshold cycle number) was determined by analysis with LightCycler ® 480 II software. The relative gene expression was calculated using the wild-type plants as the control, and using the equation Y = 2 ddCt , where ddCt = test gene (Ct Overexpresser – Ct wild type ) – normalization gene (Ct Overexpresser – Ct wild-type ), with eIF4a1 as the internal control. Each RNA sample was used for qRT–PCR in triplicate, and these data were combined in two or three independent experiments.
Yeast one-hybrid screening
To produce a yeast strain carrying the PLA 2 -γ promoter, a 660 bp fragment containing the whole intergenic region from the first ATG (designated as + 1) of the PLA 2 -γ gene to the 3′- UTR (–660) of the preceding PLA 2 -δ gene was amplified by PCR. The amplified fragments were cloned in-frame upstream of both the minimal promoter of the HIS3 reporter gene in the pHISi-1 vector and the minimal promoter of the LacZ reporter gene in the pLacZi vector (Clontech). Yeast one-hybrid screening was carried out using the Matchmaker One-hybrid System (Clontech). Briefly, 1 µg of pLacZi (containing ProPLA 2 -γ:LacZ ) and of pHISi-1 (containing ProPLA 2 -γ:HIS3 ) were digested with Nco I/ Xho I and transformed into the YM4271 yeast strain to form the dual YM4271 strain. As prey, we used the Arabidopsis TF-only library in which 1,050 TF open reading frames (ORFs) are fused in the correct reading frame to the Gal4 activation domain and cloned into the pDEST22 vector ( Welchen et al. 2009 ). Prey constructs (10 µg) were transformed into the dual YM4271 strain with the standard lithium acetate transformation method ( Gietz et al. 1995 ). A total of 6 × 10 5 transformants were plated on SD medium lacking uracil, histidine and tryptophan and supplemented with 45 mM 3-aminotriazole, a competitive inhibitor of the His3 protein. The resulting putative clones from the initial screening were streaked on the same selective SD medium to purify the colonies and perform the lacZ reporter assay. The plasmid DNAs containing the ORFs of the TFs were rescued and retransformed into the dual YM4271 yeast for further confirmation.
GUS expression and fluorometric assay
The 660 bp full-length promoter and 5′-deleted truncated promoter series (421, 260 and 153 bp, respectively) of PLA 2 -γ were amplified by PCR from Arabidopsis genomic DNA, digested with Hin dIII and Bam HI, and cloned into the Hin dIII/ Bam HI sites of the pBI121 vector (Clontech) (respectively designated as ProPLA 2 -γ:GUS , ProPLA 2 -γA:GUS , ProPLA 2 -γB:GUS and ProPLA 2 -γC:GUS ) ( Supplementary Table S2 ). In the case of two TFs, the 1,405p and 968 bp (without the 5′-UTR) γMYB1 promoter and the 2,061 bp γMYB2 promoter were also cloned in-frame into the pBI121 vector (designated as ProγMYB1:GUS , ProγMYB1ΔUTR:GUS and ProγMYB2:GUS , respectively) ( Supplementary Table S2 ). The primers used for cloning of the promoters are listed in Supplementary Table S3 .
For GUS histochemical staining, tissue samples of T 3 plants were incubated overnight in X-Gluc solution [1 mM 5-bromo-4-chloro-3-indolyl-β- d -glucuronate, 10 mM Na 2 EDTA, 0.5 mM potassium ferrocyanide, 0.5 mM potassium ferricyanide, 0.1% (v/v) Triton X-100, 50 mM sodium phosphate buffer, pH 7.0] at 37°C. After staining, Chl was cleared by immersion in an ethanol series (70% and 100%, each concentration for 1 d) and the tissue samples were observed under a microscope (Olympus model BX-51).
For the fluorometric assay, a 100 mg sample of open flowers was collected and GUS activity was measured using the GUS fluorescent reporter gene activity detection kit GUS-A (Sigma ® ) according to the manufacturer’s instructions. Fluorescence of the reaction product, namely, 4-methylumbelliferone (4-MU), was measured with the Synergy ™ H1 Hybrid Multi-Mode Microplate Reader (Biotek ® ) at 460 nm with excitation at 35 nm.
Subcellular localization of the γMYB1–GFP and γMYB2–GFP fusion proteins
Pro35S:γMYB1-GFP and Pro35S:γMYB2-GFP constructs were generated using pCAMBIA2300 ( Supplementary Table S2 ). The resulting Pro35S:γMYB1-GFP and Pro35SγMYB2-GFP constructs were subsequently introduced into onion epidermal cells using a helium biolistic PDS-1000 gene delivery system (Bio-Rad) with 1,100 p.s.i. rupture disks and 1.0 µm diameter gold microcarriers. The bombarded onion epidermal cells were incubated for 24 h in the dark at 22°C and then observed under an Olympus BX-51 fluorescence microscope equipped with XF116-2 and XF-06 filter sets (Olympus).
Construction of γMYB1- and γMYB2-overexpression and γMYB1-knock down lines
ProLat52:γMYB1 and ProLat52:γMYB1 overexpression lines were constructed using pPZP221 ( Hajdukiewicz et al. 1994 ) ( Supplementary Table S2 ). Knock-down lines of γMYB1 in pollen used a pollen-specific promoter and were constructed by using amiRNA ( Lat52:amiγMYB1 ), according to the instructions of the Web MicroRNA Designer Tool ( http://wmd3.weigelworld.org ). To exclude possible off-targets, we selected the sequences located at the 635 bp site and 1,098 bp site of AT2G20400.1 cDNA as the target sequences and named them Lat52:amiγMYB1-1 and Lat52:γMYB1-8 , respectively.
Electrophoretic mobility shift assay
The MYB and CC-domain regions of γMYB1 and γMYB2 were amplified, digested with Eco RI/ Xho I and then cloned into the corresponding site of the pGEX-6P-1 vector (GE Healthcare). These constructs were transformed into Escherichia coli BL21-CodonPlus ® -(DE3)-RIL (Agilent Technologies). Expression of the recombinant proteins was induced by 1 mM isopropyl-β- d -1-thiogalactopyranoside (Duchefa) for 12 h at 30°C (for γMYB1) or for 3 h at 37°C (for γMYB2) and purified using glutathione–Sepharose ™ 4B beads (GE Healthcare) in Poly-Prep ® Chromatography columns (Bio-Rad). The purified proteins were kept at –20°C until use.
To construct probes for the EMSA, we divided the 660 bp PLA 2 -γ promoter into six regions (designated as ProPLA 2 -γP1 to ProPLA 2 -γP6 ; Fig. 3 A) based on the location of MYB-binding cis -elements. Each fragment was amplified by PCR, purified using a PCR purification kit (Intron), labeled with [γ- 32 P]ATP using T4 polynucleotide kinase, and finally diluted to at least 50,000 c.p.m. µl –1 . For the EMSA reaction, 100 ng of pure recombinant protein, 100,000 c.p.m. of 32 P-end-labeled probe, unlabeled competitors and 1 µg of poly(dI–dC) were mixed and incubated for 30 min at 4°C in an EMSA buffer [10 mM Tris–HCl pH 7.5, 5 mM NaCl, 1 mM EDTA, 5 mM dithiothreitol (DTT), 5% glycerol]. The unlabeled competitors were used at 50×and 100 × the molar amount of labeled probe. Electrophoresis was carried out on a 6% acrylamide/bisacrylamide (29 : 1) non-denaturing gel which was pre-run at 100 V for 1 h at 4°C and then run at 150 V for 3–4 h at 4°C. Following electrophoresis, the gels were dried on Whatman 3MM filter paper and the signals in the dried gel were detected on BAS-MS Imaging plates (Fujifilm) using a bio-imaging analyzer (BAS-2500; Fujifilm). For the EMSA with 20–40 nucleotide segments of ProPLA 2 -γP4 and P6 ( Fig. 3 C), the Biotin 3′-End DNA Labeling kit (Thermo Scientific) was used for labeling and the LightShift ® Chemiluminescent EMSA kit was used for detection (Thermo Scientific).
Trans-activation assay of γMYB1 and γMYB2
To generate the constructs for the transient assay using the GAL4 system, the transient expression vector pBI221 (Clontech) was modified ( Supplementary Table S2 ). DNA of luciferase from the firefly ( P. pyralis ) ( Gould and Subramani 1988 ) was used for this assay. GAL4(3×):LUC containing a triplicate yeast GAL4-binding site fused to a minimal TATA box and a LUC gene was used as a reporter in the transient assay. The GAL4DB fused to all TFs, which were controlled by the 35S promoter. Both 35S:GAL4DB-γMYB1 and - γMYB2 were used as effectors, and 35S:GAL4DB-VP16 was the positive control ( Sadowski et al. 1988 ). The basal level of transcription was determined by transfecting protoplasts with 35S:GAL4DB. Construct 35S:GUS was used as the internal control. These three constructs (reporter : effector : internal control = 4 : 5 : 1) were co-transferred into Arabidopsis protoplasts.
To examine direct regulation of γMYB1 and γMYB2 on the PLA 2 -γ promoter, the transient expression vector pBI221 (Clontech) was modified ( Supplementary Table S2 ). ProPLA 2 -γ:GUS was used as the reporter in the transient expression assay, and two constructs, 35S:γMYB1 and 35S:γMYB2 , were used as effectors. The basal level of transcription was determined by transfecting protoplasts with pBI221 plasmid with the GUS gene removed. For this experiment, 35S:LUC was used as the internal control. These three constructs (reporter : effector : internal control = 4 : 5 : 1) were co-transferred into N. benthamiana protoplasts.
The protoplast transient expression assay was performed as previously described by Yoo et al. (2007) . The PEG-transfected protoplasts were harvested and cell lysates were extracted. LUC mix (Promega) or MUG-4 (Sigma-Aldrich) was added to the cell lysates. The GAL4(3×):LUC reporter system or the ProPLA 2 -γ:GUS reporter system was quantified using the Synergy ™ H1 Hybrid Multi-Mode microplate reader (BioTek Instruments).
Bimolecular fluorescence complementation (BiFC) assay
γMYB1 and γMYB2 cDNAs were cloned in-frame into the Bam HI/ Xho I sites of the pSPYNE and pSPYCE vectors ( Supplementary Table S2 ) ( Walter et al. 2004 ). Plasmid constructs were then introduced into Arabidopsis protoplasts. After transformation, protoplasts were incubated in the dark for 24 h at 25°C and fluorescence was assessed under an Olympus BX-51 fluorescence microscope (Olympus).
Immunoprecipitation
γMYB1 and γMYB2 cDNAs were cloned in-frame into the p326-sGFP-1 and pCterm-HA vectors to yield the sGFP- and HA-tagged proteins ( Supplementary Table S2 ), then introduced into Arabidopsis protoplasts. Immunoprecipitation was performed as described previously ( Kim et al. 2013 ). Protein extracts were prepared in immunoprecipitation buffer and incubated with α-GFP antibody (Clontech). Immunocomplexes were precipitated by incubating them with Immobilized Protein A resin (RepliGen Bioprocessing). After washing, the resins were subjected to immunoblot analysis using α-GFP and α-HA antibody (Cell Signaling Technology).
Bioinformatic analysis
To identify cis -elements that may regulate gene expression and pollen specificity of the PLA 2 -γ promoter, we used the PLACE database of plant cis -acting regulatory elements ( Higo et al. 1999 ). To compare the deduced amino acid sequences of γMYB1 and γMYB2 with sequences in the protein database, we used NCBI BLAST ( http://www.ncbi.nlm.nih.gov/BLAST/ ). The sequences of these four MYB-CC proteins were aligned using Clustal Omega ( https://www.ebi.ac.uk/Tools/msa/clustalo/ ) ( Sievers et al. 2011 ). To find gene promoters containing the P1BS cis -element (GGATATTC), we used the Regulatory Sequence Analysis Tools ( http://rsat.ulb.ac.be/ ) ( van Helden 2003 ).
Accession numbers
Sequence data from this study can be found in the Arabidopsis Genome Initiative or GenBank/EMBL databases under the following accession numbers; PLA 2 -γ (At4g29460), PLA 2 -δ (At4g29470), γMYB1 (At2g20400), γMYB2 (At3g13040), PHR1 (At4g28610), PHL1 (At5g29000), eIF4a-1 (At3g13920), CCT1 (At2g32260), SPX1 (At5g20150), SPX2 (At2g26660), ISA2 (At1g03310), SPS2F (At5g11110) and EMF1 (At5g11530).
Funding
This work was supported by the Ministry of Science and Technology [National Research Foundation of Korea (NRF) grant NRF-2014R1A2A2A01005974]; the Rural Development Administration, Republic of Korea [the Next-Generation BioGreen 21 Program grant PJ011065); Korea University.
Abbreviations
- amiRNA
artificial microRNA
- BiFC
bimolecular fluorescence complementation, GFP, green fluorescent protein
- EMSA
electrophoretic mobility shift assay
- GAL4DB
GAL4 DNA-binding domain
- GUS
β-glucuronidase
- HA
hemagglutinin
- P1BS
phosphate starvation response 1-binding sequence
- PLA 2
phospholipase A 2
- qRT-PCR
quantitative reverse transcription–PCR
- TF
transcription factor
- UTR
untranslated region
- YC
C-terminal fragment of yellow fluorescent protein
- YN
N-terminal fragment of yellow fluorescent protein
Acknowledgements
We thank Professor Brian M. Waters (University of Nebraska-Lincoln) for English editing, critical reading and valuable comments on our manuscript.
Disclosures
The authors have no conflicts of interest to declare.
References