-
PDF
- Split View
-
Views
-
Cite
Cite
Asuka Higo, Masaki Niwa, Katsuyuki T. Yamato, Lixy Yamada, Hitoshi Sawada, Tomoaki Sakamoto, Tetsuya Kurata, Makoto Shirakawa, Motomu Endo, Shuji Shigenobu, Katsushi Yamaguchi, Kimitsune Ishizaki, Ryuichi Nishihama, Takayuki Kohchi, Takashi Araki, Transcriptional Framework of Male Gametogenesis in the Liverwort Marchantia polymorpha L. , Plant and Cell Physiology, Volume 57, Issue 2, February 2016, Pages 325–338, https://doi.org/10.1093/pcp/pcw005
- Share Icon Share
Abstract
In land plants, there are two types of male gametes: one is a non-motile sperm cell which is delivered to the egg cell by a pollen tube, and the other is a motile sperm cell with flagella. The molecular mechanism underlying the sexual reproduction with the egg and pollen-delivered sperm cell is well understood from studies using model plants such as Arabidopsis and rice. On the other hand, the sexual reproduction with motile sperm has remained poorly characterized, due to the lack of suitable models. Marchantia polymorpha L. is a model basal land plant with sexual reproduction involving an egg cell and bi-flagellated motile sperm. To understand the differentiation process of plant motile sperm, we analyzed the gene expression profile of developing antheridia of M. polymorpha. We performed RNA-sequencing experiments and compared transcript profiles of the male sexual organ (antheridiophore and antheridium contained therein), female sexual organ (archegoniophore) and a vegetative organ (thallus). Transcriptome analysis showed that the antheridium expresses nearly half of the protein-coding genes predicted in the genome, but it also has unique features. The antheridium transcriptome shares some common features with male gamete transcriptomes of angiosperms and animals, and homologs of genes involved in male gamete formation and function in angiosperms and animals were identified. In addition, we showed that some of them had distinct expression patterns in the spermatogenous tissue of developing antheridia. This study provides a transcriptional framework on which to study the molecular mechanism of plant motile sperm development in M. polymorpha as a model.
The nucleotide sequences reported in this paper for the first time have been submitted to DDBJ under accession numbers: Mp PACRG (LC102460), Mp LC7 (LC102461), Mp PRM , LC102462. RNA sequencing data have been deposited in the DDBJ BioProject and BioSample databases under accession numbers DRA004243 (DRA), PRJDB4420 (BioProject) and SAMD00044058 to SAMD00044068 (BioSample).
Introduction
Sexual reproduction is one of the most important biological processes in land plants. It involves germ cell specification and functional gamete formation within a multicellular body. The resulting gametes, a small male gamete (sperm cell) and a large female gamete (egg cell), fuse to generate a zygote which starts the next generation. During the evolution of land plants, the process of gamete formation has undergone drastic changes. In haploid (gametophyte)-dominant basal land plants, gamete formation is not coupled to meiosis. In contrast, in diploid (sporophyte)-dominant plants, such as extant seed plants, due to great reduction of the gametophyte generation, gametogenesis immediately follows meiosis which generates microspores (pollen) and megaspores (ovules). Another evolutionarily important change has occurred in the motility of male gametes. In basal land plants including bryophytes (hornworts, liverworts and mosses), lycophytes and monilophytes, male gametes are free-swimming motile cells with two or many flagella ( Renzaglia and Garbary 2001 ). In gymnosperms and angiosperms, sperm cells are delivered by the pollen tube, a structure produced by the male gametophyte, and the motility of sperm cells has been lost in most of the extant gymnosperm lineages (except for Gingko and cycads) and all the angiosperm lineages ( Gifford and Foster 1989 ).
The process of non-motile male gamete formation and the antecedent microspore and gametophyte formation processes have been extensively studied in angiosperms. Genes commonly expressed in the male germ cell (generative cell) and/or the sperm cell of flowering plants have been identified by genetic studies and transcriptome analysis of those cells purified from various plant species ( Engel et al. 2003 , Okada et al. 2006 , Okada et al. 2007 , Borges et al. 2008 , Gou et al. 2009 , Russell et al. 2012 ; reviewed by Berger and Twell 2011 , Twell 2011 ). These genes include conserved key regulators that control the male gamete formation process and subsequent male gamete function such as the R2R3-type MYB transcription factor (TF) DUO POLLEN1 (DUO1), which plays a pivotal role in integrating germline fate and cell cycle progression and regulates mitotic entry of generative cells ( Rotman et al. 2005 , Brownfield et al. 2009 ). It is believed that the function of DUO1 in specification of male germ cells is widely conserved among flowering plants ( Berger and Twell 2011 , Twell 2011 ). In addition to identifying genes commonly expressed in male gametes of flowering plants, transcriptome analysis of isolated gametes showed that flowering plants and animals share common molecular mechanisms underlying gamete formation and function including pre- or post-meiotic gene expression regulation, chromatin remodeling and small RNA-mediated epigenetic regulation ( Pereira et al. 2014 ). In sharp contrast to this wealth of studies of non-motile male gametogenesis in angiosperms, few molecular studies have been carried out on motile male gamete formation in basal land plants with the possible exception of an aquatic fern, Marsilea vestita (e.g. van der Weele et al. 2007 ).
A liverwort, Marchantia polymorpha L., is an emerging model basal land plant that represents Marchantiophyta (liverworts) ( Ishizaki et al. 2016 ). It is a dioecious plant whose sexes are determined by X and Y chromosomes ( Yamato et al. 2007 ). Male gametophyte plants (haploid generation) of M. polymorpha differentiate motile flagellated sperm cells called antherozoids. Antheridia in many complex thalloid liverworts (Marchantiales) including Marchantiaceae are borne on a special branch called an antheridiophore. It is composed of a disk-like structure called the antheridial receptacle, which contains antheridia and a supporting stalk ( Shimamura 2016 ). An antheridium has a simple structure composed of an inner spermatogenous tissue and an outer single surrounding layer of jacket cells, and is attached to the bottom of a flask-like cavity in the antheridial receptacle by a short stalk ( Durand 1908 , Shimamura 2016 ).
During antheridium development, an antheridial initial is formed near the periphery of the antheridial receptacle. By periclinal division of an antheridial initial, a distal antheridium proper mother cell (APMC) and a proximal stalk mother cell are generated. Successive cell divisions of the APMC result in an antheridial primordium with usually two or three tiers of four antheridial cells each. Subsequent periclinal division of each antheridial cell then gives rise to an outer jacket cell and an inner spermatogenous cell. Continuous cell divisions, both transversely and vertically, of an inner spermatogenous cell result in a group of cuboidal cells. These proliferative divisions are in synchrony within a sector derived from a single progenitor spermatogenous cell which corresponds to a germline cell. The outer jacket cells divide only anticlinally, and the progeny cells remain within a single cell layer. After proliferation of spermatogenous cells, the resultant sperm mother cells divide diagonally and generate a pair of triangular sperm cells ( Durand 1908 ). Complex cell morphogenesis (spermiogenesis) ensues within the cell wall of each sperm cell to generate mature motile sperm with an elongated and twisted cell body and two flagella attached near the anterior end. Spermiogenesis, in which a sperm cell develops into a structurally complex, naked (i.e. devoid of a cell wall), flagellated cell, involves many distinct cellular events, such as reduction of cytoplasm, formation of a backbone-like microtubular structure called the spline, structural change and condensation of the nucleus, assembly of flagella and cell wall degradation ( Renzaglia and Garbary 2001 ). Thus, the whole process of sperm formation (spermatogenesis), from the specification of the male germ (spermatogenous) cell lineage and its proliferation by successive synchronous cell divisions to the complex cell morphogenesis (spermiogenesis), gives rise to many questions regarding cell and developmental biology ( Renzaglia and Garbary 2001 ). These are beginning to be addressed, with the antheridium of M. polymorpha as a particularly tractable model system.
In this study, we constructed a transcriptional framework of antheridium development in M. polymorpha as an initial step in understanding the molecular mechanism of male germ cell specification and motile male gamete formation. We compared the transcriptome of developing antheridia with that of thalli, antheriophore receptacles and archegoniophore receptacles by RNA-sequencing experiments. These comparisons revealed the complexity as well as uniqueness of the transcriptome of the antheridium, which essentially contains only two cell types. Antheridium-enriched genes included the homologs of Arabidopsis genes involved in germ cell formation and function, homologs of animal genes involved in spermatogenesis or sperm function, and genes encoding various metabolic enzymes. The transcriptional framework elucidated in this study and some of the genes identified by our analysis will enable us to develop fully the antheridium of M. polymorpha as an ideal model to study male gametogenesis in land plants.
Results
Structure and development of male sexual organs in M. polymorpha and sample collection
To investigate the molecular mechanisms involved in the male gametogenesis of M. polymorpha , we examined transcript profiles of developing antheridia by RNA-sequencing experiments. In M. polymorpha , antheridia are embedded in the antheridial receptacle formed on a stalk ( Fig. 1 A, B). They are arranged in a concentric pattern in the receptacle, and the younger ones are located near the periphery where new primordia are continuously initiated, while the older ones are displaced toward the center of the receptacle ( Fig. 1 C). An antheridium has a simple structure and is composed essentially of only two types of cells, spermatogenous cells, which eventually give rise to antherozoids, and a surrounding single layer of jacket cells ( Fig. 1 D).
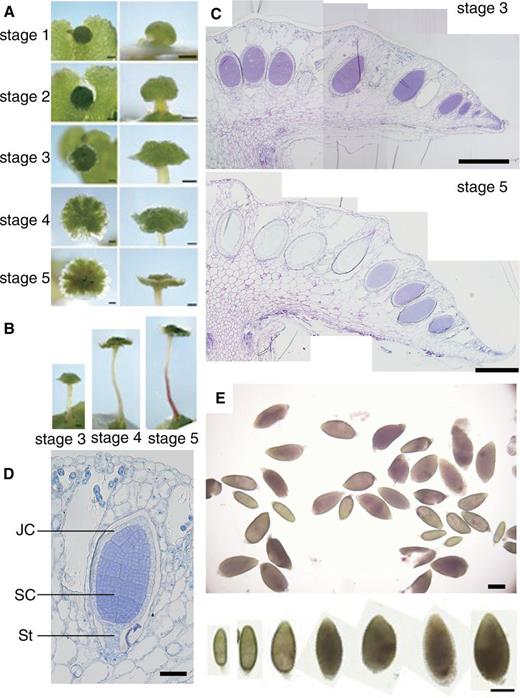
Developmental stages and structure of antheridiophore and sampling of antheridia. (A) Developmental stages of antheridiophores of Tak-1. Photographs in the left column were taken from above and those in the right column are side views. Scale bar = 1 mm. (B) Side views of antheridiophores at stages 3–5. Scale bar = 1 mm. (C) Longitudinal section of antheridiophores at stage 3 (top panel) and stage 5 (bottom panel) stained with Toluidine Blue O. Scale bar = 500 µm. (D) Longitudial section of a young antheridium stained with Toluidine Blue O. JC, jacket cell; SC, spermatogenous cell; St, stalk. Scale bar = 50 µm. (E) Antheridia manually dissected from antheridial receptacles. Similar samples were used in RNA sequencing. Enlarged photographs of antheridia in various sizes are shown in the bottom panel. Scale bar = 200 µm.
To aid consistent sampling and analysis, we first divided antheridiophore development of a standard laboratory male strain, Takaragaike-1 (Tak-1), into five stages in our growth conditions ( Fig. 1 A, B). Stage 1 is defined as a button-like antheridial receptacle with little elongation of the stalk. Visible stalk elongation defines stage 2. The antheridial receptacle becomes disk like with the outward development of the edge during stage 3. At stage 4, an upward curling of the edge begins, and it gives the antheridial receptacle the appearance of a shallow cup with a bump at the center. Bi-lateral symmetry of the receptacle is evident at this stage. The mature antheridiophore with a fully elongated stalk and anthocyanin accumulation in the receptacle and stalk defines stage 5. Because antheridiophores at stages 3–5 contain developing immature antheridia of various stages ( Fig. 1 C), we chose these stages to collect developing antheridia by manually dissecting them out of antheridial receptacles ( Fig. 1 E). Collected samples (hereafter referred to as ‘antheridium’) contained antheridia of the cell proliferation stage and the cell morphogenesis (i.e. spermiogenesis) stage, but those at earlier stages (less than approximately 200 μm) were not included ( Fig. 1 E, bottom panel). In addition to antheridia, we collected 11-day-old Tak-1 vegetative thalli grown from gemmae (hereafter referred to as ‘thallus’) ( Supplementary Fig. S1A ), whole antheridial receptacles from Tak-1 at stage 4 (‘antheridiophore’) ( Fig. 1 A, B) and archegonial receptacles of a standard laboratory female strain (Tak-2) at stage 4 (‘archegoniophore’) ( Supplementary Fig. S1B ). Archegoniophore development was similarly divided into four stages (see Supplementary Fig. S1B ).
Transcriptional profiles of the antheridium and other organs
We obtained roughly 14 × 10 6 reads, at least, from each of the samples by RNA-sequencing experiments, and mapped >70% of each to the draft M. polymorpha genome sequences with TopHat ( Trapnell et al. 2009 ) ( Supplementary Table S1 ). A Venn diagram shows the number of genes expressed uniquely or commonly among tested organs ( Fig. 2 A). Genes whose RPKM (read per kilobase per million) value was >1 were considered as ‘expressed’. Among the 23,498 genes that were predicted by the Cufflinks program using the draft genome sequences (provided by the Joint Genome Institute in December, 2011), 11,169 genes (47.5%) were expressed in the antheridium. Genes expressed in sexual organs but not in vegetative thallus comprised 11.7% (2,753 genes), and 7.3% (1,713 genes) were only expressed in male sexual organs (antheridium and antheridiophore). In contrast, genes expressed exclusively in the vegetative thallus comprised only 3.5% (815 genes). On the other hand, 9,007 genes (38.3%) were expressed in all the tested organs of gametophyte plants (i.e. the haploid generation). Expression profiles of representative genes in gametophyte generation were confirmed by reverse transcription–PCR (RT–PCR) analysis ( Fig. 3 ).
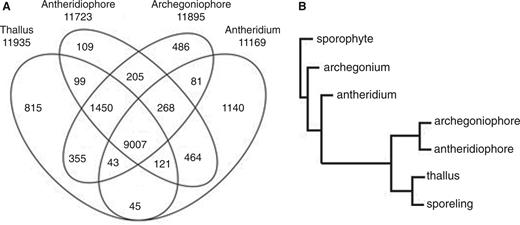
Antheridium had a distinct transcript feature. (A) Venn diagram, depicting the number of genes uniquely or commonly expressed in each tested organ. The total number of genes expressed in each organ is shown below the sample name. Genes whose RPKM value was > 1 were considered as ‘expressed’ in each organ. (B) Hierarchical clustering of gene expression profiles. RNA-sequencing data of thallus, antheridium, antheridiophore and archegoniophore were compared with those of archegonium, sporophyte and sporeling obtained separately. The distance showed a measure of closeness of individual clusters.
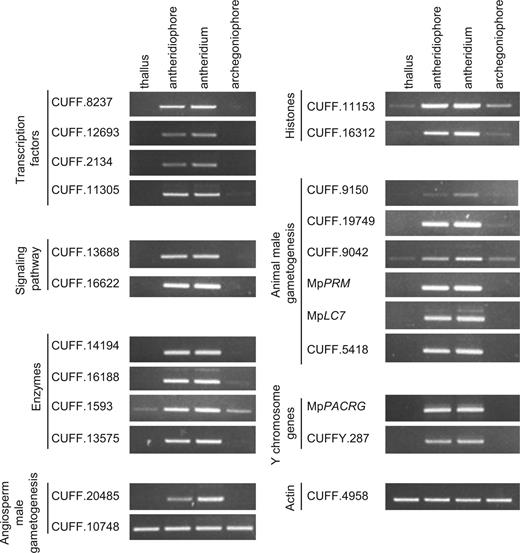
Verification of expression profiles of representative genes by RT–PCR. The expression of representative genes from each table was validated in thallus, antheridiaophore, antheridiaum and archegoniaophore by RT–PCR. A constitutively expressed actin gene (CUFF.4958) was used as an internal control. CUFF.8237, CUFF.12693, CUFF.2134 and CUFF.11305 are listed in Table 2 . CUFF.13688 and CUFF. 16622 are listed in Supplementary Table S2 . CUFF.14194, CUFF.16188, CUFF.1539 and CUFF.13575 are listed in Supplementary Table S3 . CUFF.20485 and CUFF.10748 are listed in Supplementary Table S4 . CUFF.16312 and CUFF.11153 are listed in Table 3 . CUFF.9150, CUFF.19749, CUFF.9042 and CUFF.5418 are listed in Supplementary Table S5 . CUFFY.287 is listed in Supplementary Table S6 . Note that Mp PRM is included in ‘Animal male gametogenesis’ but has an independent evolutionary origin.
To compare the features of the transcript profiles further, we performed hierarchical clustering of the transcriptomes ( Fig. 2 B). Data from similar RNA-sequencing experiments performed separately (accessible at http://genome.jgi.doe.gov/Marpolnscriptome/Marpolnscriptome.download.html ) for young gametophytes after spore germination (‘sporeling’), developing archegonia (‘archegonium’) and developing sporophytes with surrounding, protective female gametophyte tissues (‘sporophyte’) were also included. The sporophyte, which includes diploid cells, was first separated from the other haploid organs. Then, the female and male gametangia, archegonium and antheridium, respectively, were dissociated from the rest of the haploid organs. Male and female sexual branches, antheridiophore and archegoniophore, respectively, which contain much of the vegetative tissues surrounding gametangia, were clustered with thallus and sporeling.
Functional classification of genes preferentially expressed in antheridium
To delineate the transcriptomic features of developing antheridia, we performed a Gene Ontology (GO) enrichment analysis of the genes preferentially expressed in antheridium using the Biological Networks Gene Ontology tool (BiNGO) ( Maere et al. 2005 ). Genes with a log2-fold change of >3 between antheridium and thallus or archegoniophore were considered as ‘preferentially expressed’ in antheridium. By this criterion, 731 out of 1,713 genes only expressed in male sexual organs (antheridium and antheridiophore) were preferentially expressed in antheridium. Among 731 antheridium-preferential genes, 441 genes had homology to known genes with GO terms. These genes were further analyzed. The highly represented functional categories in antheridium included genes associated with assembly of flagella and motility of sperm, namely ‘cytoskeleton’, ‘cellular component organization’ and ‘motor activity’ ( Table 1 ). Genes involved in ‘cell cycle’, ‘reproduction’ and ‘regulation of gene expression, epigenetic’ were also enriched ( Table 1 ). The ‘cell cycle’ category included general cell cycle regulators and centrosome formation-associated genes. The ‘regulation of gene expression, epigenetic’ category included genes involved in chromatin modification, DNA repair and RNA silencing.
GO . | Corrected P -value . | Antheridium . | Genome . | |||
---|---|---|---|---|---|---|
ID . | Description . | . | x . | Rate (%) . | n . | Rate (%) . |
5856 | Cytoskeleton | 6E-29 | 75 | 17 | 418 | 4 |
16043 | Cellular component organization | 3E-16 | 121 | 27 | 1,460 | 13 |
3774 | Motor activity | 7E-09 | 17 | 4 | 66 | 1 |
7049 | Cell cycle | 3E-07 | 43 | 10 | 444 | 4 |
3 | Reproduction | 3E-06 | 55 | 13 | 704 | 6 |
30154 | Cell differentiation | 3E-06 | 44 | 10 | 507 | 4 |
7267 | Cell–cell signaling | 3E-21 | 11 | 3 | 54 | 1 |
7610 | Behavior | 9E-05 | 12 | 3 | 68 | 1 |
9653 | Anatomical structure morphogenesis | 2E-04 | 56 | 13 | 830 | 7 |
3677 | DNA binding | 1E-03 | 63 | 14 | 1,053 | 9 |
7275 | Multicellular organismal development | 2E-03 | 90 | 20 | 1,657 | 14 |
5634 | Nucleus | 2E-03 | 139 | 32 | 2,824 | 24 |
5515 | Protein binding | 2E-03 | 154 | 35 | 3,207 | 27 |
5622 | Intracellular | 7E-03 | 312 | 71 | 7,465 | 64 |
40029 | Regulation of gene expression, epigenetic | 2E-02 | 12 | 3 | 124 | 1 |
5488 | Binding | 3E-02 | 324 | 74 | 7,916 | 68 |
9790 | Embryonic development | 3E-02 | 22 | 5 | 318 | 3 |
9605 | Response to external stimulus | 3E-02 | 22 | 5 | 322 | 3 |
7154 | Cell communication | 5E-02 | 15 | 3 | 200 | 2 |
9987 | Cellular process | 5E-02 | 314 | 71 | 7,717 | 66 |
GO . | Corrected P -value . | Antheridium . | Genome . | |||
---|---|---|---|---|---|---|
ID . | Description . | . | x . | Rate (%) . | n . | Rate (%) . |
5856 | Cytoskeleton | 6E-29 | 75 | 17 | 418 | 4 |
16043 | Cellular component organization | 3E-16 | 121 | 27 | 1,460 | 13 |
3774 | Motor activity | 7E-09 | 17 | 4 | 66 | 1 |
7049 | Cell cycle | 3E-07 | 43 | 10 | 444 | 4 |
3 | Reproduction | 3E-06 | 55 | 13 | 704 | 6 |
30154 | Cell differentiation | 3E-06 | 44 | 10 | 507 | 4 |
7267 | Cell–cell signaling | 3E-21 | 11 | 3 | 54 | 1 |
7610 | Behavior | 9E-05 | 12 | 3 | 68 | 1 |
9653 | Anatomical structure morphogenesis | 2E-04 | 56 | 13 | 830 | 7 |
3677 | DNA binding | 1E-03 | 63 | 14 | 1,053 | 9 |
7275 | Multicellular organismal development | 2E-03 | 90 | 20 | 1,657 | 14 |
5634 | Nucleus | 2E-03 | 139 | 32 | 2,824 | 24 |
5515 | Protein binding | 2E-03 | 154 | 35 | 3,207 | 27 |
5622 | Intracellular | 7E-03 | 312 | 71 | 7,465 | 64 |
40029 | Regulation of gene expression, epigenetic | 2E-02 | 12 | 3 | 124 | 1 |
5488 | Binding | 3E-02 | 324 | 74 | 7,916 | 68 |
9790 | Embryonic development | 3E-02 | 22 | 5 | 318 | 3 |
9605 | Response to external stimulus | 3E-02 | 22 | 5 | 322 | 3 |
7154 | Cell communication | 5E-02 | 15 | 3 | 200 | 2 |
9987 | Cellular process | 5E-02 | 314 | 71 | 7,717 | 66 |
The rate of each GO term among antheridium-enriched genes and total genes present in the genome is shown in the ‘rate’ columns.
x , number of genes belonging to the functional category and expressed in antheridium; n , number of genes in the genome belonging to the functional category.
GO . | Corrected P -value . | Antheridium . | Genome . | |||
---|---|---|---|---|---|---|
ID . | Description . | . | x . | Rate (%) . | n . | Rate (%) . |
5856 | Cytoskeleton | 6E-29 | 75 | 17 | 418 | 4 |
16043 | Cellular component organization | 3E-16 | 121 | 27 | 1,460 | 13 |
3774 | Motor activity | 7E-09 | 17 | 4 | 66 | 1 |
7049 | Cell cycle | 3E-07 | 43 | 10 | 444 | 4 |
3 | Reproduction | 3E-06 | 55 | 13 | 704 | 6 |
30154 | Cell differentiation | 3E-06 | 44 | 10 | 507 | 4 |
7267 | Cell–cell signaling | 3E-21 | 11 | 3 | 54 | 1 |
7610 | Behavior | 9E-05 | 12 | 3 | 68 | 1 |
9653 | Anatomical structure morphogenesis | 2E-04 | 56 | 13 | 830 | 7 |
3677 | DNA binding | 1E-03 | 63 | 14 | 1,053 | 9 |
7275 | Multicellular organismal development | 2E-03 | 90 | 20 | 1,657 | 14 |
5634 | Nucleus | 2E-03 | 139 | 32 | 2,824 | 24 |
5515 | Protein binding | 2E-03 | 154 | 35 | 3,207 | 27 |
5622 | Intracellular | 7E-03 | 312 | 71 | 7,465 | 64 |
40029 | Regulation of gene expression, epigenetic | 2E-02 | 12 | 3 | 124 | 1 |
5488 | Binding | 3E-02 | 324 | 74 | 7,916 | 68 |
9790 | Embryonic development | 3E-02 | 22 | 5 | 318 | 3 |
9605 | Response to external stimulus | 3E-02 | 22 | 5 | 322 | 3 |
7154 | Cell communication | 5E-02 | 15 | 3 | 200 | 2 |
9987 | Cellular process | 5E-02 | 314 | 71 | 7,717 | 66 |
GO . | Corrected P -value . | Antheridium . | Genome . | |||
---|---|---|---|---|---|---|
ID . | Description . | . | x . | Rate (%) . | n . | Rate (%) . |
5856 | Cytoskeleton | 6E-29 | 75 | 17 | 418 | 4 |
16043 | Cellular component organization | 3E-16 | 121 | 27 | 1,460 | 13 |
3774 | Motor activity | 7E-09 | 17 | 4 | 66 | 1 |
7049 | Cell cycle | 3E-07 | 43 | 10 | 444 | 4 |
3 | Reproduction | 3E-06 | 55 | 13 | 704 | 6 |
30154 | Cell differentiation | 3E-06 | 44 | 10 | 507 | 4 |
7267 | Cell–cell signaling | 3E-21 | 11 | 3 | 54 | 1 |
7610 | Behavior | 9E-05 | 12 | 3 | 68 | 1 |
9653 | Anatomical structure morphogenesis | 2E-04 | 56 | 13 | 830 | 7 |
3677 | DNA binding | 1E-03 | 63 | 14 | 1,053 | 9 |
7275 | Multicellular organismal development | 2E-03 | 90 | 20 | 1,657 | 14 |
5634 | Nucleus | 2E-03 | 139 | 32 | 2,824 | 24 |
5515 | Protein binding | 2E-03 | 154 | 35 | 3,207 | 27 |
5622 | Intracellular | 7E-03 | 312 | 71 | 7,465 | 64 |
40029 | Regulation of gene expression, epigenetic | 2E-02 | 12 | 3 | 124 | 1 |
5488 | Binding | 3E-02 | 324 | 74 | 7,916 | 68 |
9790 | Embryonic development | 3E-02 | 22 | 5 | 318 | 3 |
9605 | Response to external stimulus | 3E-02 | 22 | 5 | 322 | 3 |
7154 | Cell communication | 5E-02 | 15 | 3 | 200 | 2 |
9987 | Cellular process | 5E-02 | 314 | 71 | 7,717 | 66 |
The rate of each GO term among antheridium-enriched genes and total genes present in the genome is shown in the ‘rate’ columns.
x , number of genes belonging to the functional category and expressed in antheridium; n , number of genes in the genome belonging to the functional category.
Genes for signaling pathways preferentially expressed in antheridium
Antheridium-enriched functional categories also included terms associated with signaling pathways ( Table 1 ). These contained genes for proteins such as TFs, channels, transporters and protein-modifying enzymes. We found that 14 genes, whose homologs in Arabidopsis are categorized as TF genes belonging to diverse families, were specifically expressed in antheridium among the tested organs ( Table 2 ). Arabidopsis homologs of these TF genes, as represented by the top hits by the BLAST search of the TAIR10 database, included those involved in the male gamete formation process such as DUO1 and DUO1-ACTIVATED ZINC FINGER2 ( DAZ2 ). Interestingly, genes involved in pollen formation in Arabidopsis, such as MALE MEIOCYTE DEATH1 ( MMD1)/DUET, MALE STERILITY1 ( MS1 ) and ABORTED MICROSPORES ( AMS ), were also found among the top hits for the 14 TF genes.
Gene ID . | TAIR10 BLAST top hit . | RPKM value a . | |||
---|---|---|---|---|---|
. | TF family . | Gene name . | AGI code . | E-value . | . |
CUFF.7228 | HMG-box | 3XHIGH MOBILITY GROUP-BOX2 | AT4G23800 | 4E-59 | 399.2 |
CUFF.1993 | C3H-type 1 (Zn) | KH domain-containing protein/zinc finger family protein | AT5G06770 | 9E-29 | 356.8 |
CUFF.17499 | C3H-type 1 (Zn) | ENHANCER OF AG-4 1 | AT3G12680 | 2E-30 | 261.0 |
CUFF.8237 b | PHD-finger | MALE STERILITY1 | AT5G22260 | 5E-131 | 254.7 |
CUFF.4436 | Nin-like | RWP-RK DOMAIN-CONTAINING 5 | AT4G35590 | 5E-12 | 82.5 |
CUFF.17973 | MYB | FOUR LIPS | AT1G14350 | 5E-32 | 70.8 |
CUFF.12693 b | TUB | TUBBY LIKE PROTEIN 11 | AT5G18680 | 3E-47 | 69.5 |
CUFF.5166 | MYB | DUO POLLEN1 | AT3G60460 | 6E-49 | 47.8 |
CUFF.19723 | Trihelix | Homeodomain-like superfamily protein | AT5G01380 | 4E-24 | 40.8 |
CUFF.12199 | GRAS | RGA-like 2 | AT3G03450 | 1E-80 | 33.6 |
CUFF.3429 | C2H2 (Zn) | DUO1-ACTIVATED ZINC FINGER2 | AT4G35280 | 3E-18 | 32.2 |
CUFF.2134 b | PHD-finger | MALE MEIOCYTE DEATH1 | AT1G66170 | 2E-92 | 26.0 |
CUFF.15606 | MYB | MYB DOMAIN PROTEIN 98 | AT4G18770 | 2E-25 | 4.2 |
CUFF.11305 b | bHLH | ABORTED MICROSPORES | AT2G16910 | 2E-26 | 3.2 |
Gene ID . | TAIR10 BLAST top hit . | RPKM value a . | |||
---|---|---|---|---|---|
. | TF family . | Gene name . | AGI code . | E-value . | . |
CUFF.7228 | HMG-box | 3XHIGH MOBILITY GROUP-BOX2 | AT4G23800 | 4E-59 | 399.2 |
CUFF.1993 | C3H-type 1 (Zn) | KH domain-containing protein/zinc finger family protein | AT5G06770 | 9E-29 | 356.8 |
CUFF.17499 | C3H-type 1 (Zn) | ENHANCER OF AG-4 1 | AT3G12680 | 2E-30 | 261.0 |
CUFF.8237 b | PHD-finger | MALE STERILITY1 | AT5G22260 | 5E-131 | 254.7 |
CUFF.4436 | Nin-like | RWP-RK DOMAIN-CONTAINING 5 | AT4G35590 | 5E-12 | 82.5 |
CUFF.17973 | MYB | FOUR LIPS | AT1G14350 | 5E-32 | 70.8 |
CUFF.12693 b | TUB | TUBBY LIKE PROTEIN 11 | AT5G18680 | 3E-47 | 69.5 |
CUFF.5166 | MYB | DUO POLLEN1 | AT3G60460 | 6E-49 | 47.8 |
CUFF.19723 | Trihelix | Homeodomain-like superfamily protein | AT5G01380 | 4E-24 | 40.8 |
CUFF.12199 | GRAS | RGA-like 2 | AT3G03450 | 1E-80 | 33.6 |
CUFF.3429 | C2H2 (Zn) | DUO1-ACTIVATED ZINC FINGER2 | AT4G35280 | 3E-18 | 32.2 |
CUFF.2134 b | PHD-finger | MALE MEIOCYTE DEATH1 | AT1G66170 | 2E-92 | 26.0 |
CUFF.15606 | MYB | MYB DOMAIN PROTEIN 98 | AT4G18770 | 2E-25 | 4.2 |
CUFF.11305 b | bHLH | ABORTED MICROSPORES | AT2G16910 | 2E-26 | 3.2 |
a RPKM value of the antheridium.
b The expression in antheridia was validated by RT–PCR ( Fig. 3 ).
Gene ID . | TAIR10 BLAST top hit . | RPKM value a . | |||
---|---|---|---|---|---|
. | TF family . | Gene name . | AGI code . | E-value . | . |
CUFF.7228 | HMG-box | 3XHIGH MOBILITY GROUP-BOX2 | AT4G23800 | 4E-59 | 399.2 |
CUFF.1993 | C3H-type 1 (Zn) | KH domain-containing protein/zinc finger family protein | AT5G06770 | 9E-29 | 356.8 |
CUFF.17499 | C3H-type 1 (Zn) | ENHANCER OF AG-4 1 | AT3G12680 | 2E-30 | 261.0 |
CUFF.8237 b | PHD-finger | MALE STERILITY1 | AT5G22260 | 5E-131 | 254.7 |
CUFF.4436 | Nin-like | RWP-RK DOMAIN-CONTAINING 5 | AT4G35590 | 5E-12 | 82.5 |
CUFF.17973 | MYB | FOUR LIPS | AT1G14350 | 5E-32 | 70.8 |
CUFF.12693 b | TUB | TUBBY LIKE PROTEIN 11 | AT5G18680 | 3E-47 | 69.5 |
CUFF.5166 | MYB | DUO POLLEN1 | AT3G60460 | 6E-49 | 47.8 |
CUFF.19723 | Trihelix | Homeodomain-like superfamily protein | AT5G01380 | 4E-24 | 40.8 |
CUFF.12199 | GRAS | RGA-like 2 | AT3G03450 | 1E-80 | 33.6 |
CUFF.3429 | C2H2 (Zn) | DUO1-ACTIVATED ZINC FINGER2 | AT4G35280 | 3E-18 | 32.2 |
CUFF.2134 b | PHD-finger | MALE MEIOCYTE DEATH1 | AT1G66170 | 2E-92 | 26.0 |
CUFF.15606 | MYB | MYB DOMAIN PROTEIN 98 | AT4G18770 | 2E-25 | 4.2 |
CUFF.11305 b | bHLH | ABORTED MICROSPORES | AT2G16910 | 2E-26 | 3.2 |
Gene ID . | TAIR10 BLAST top hit . | RPKM value a . | |||
---|---|---|---|---|---|
. | TF family . | Gene name . | AGI code . | E-value . | . |
CUFF.7228 | HMG-box | 3XHIGH MOBILITY GROUP-BOX2 | AT4G23800 | 4E-59 | 399.2 |
CUFF.1993 | C3H-type 1 (Zn) | KH domain-containing protein/zinc finger family protein | AT5G06770 | 9E-29 | 356.8 |
CUFF.17499 | C3H-type 1 (Zn) | ENHANCER OF AG-4 1 | AT3G12680 | 2E-30 | 261.0 |
CUFF.8237 b | PHD-finger | MALE STERILITY1 | AT5G22260 | 5E-131 | 254.7 |
CUFF.4436 | Nin-like | RWP-RK DOMAIN-CONTAINING 5 | AT4G35590 | 5E-12 | 82.5 |
CUFF.17973 | MYB | FOUR LIPS | AT1G14350 | 5E-32 | 70.8 |
CUFF.12693 b | TUB | TUBBY LIKE PROTEIN 11 | AT5G18680 | 3E-47 | 69.5 |
CUFF.5166 | MYB | DUO POLLEN1 | AT3G60460 | 6E-49 | 47.8 |
CUFF.19723 | Trihelix | Homeodomain-like superfamily protein | AT5G01380 | 4E-24 | 40.8 |
CUFF.12199 | GRAS | RGA-like 2 | AT3G03450 | 1E-80 | 33.6 |
CUFF.3429 | C2H2 (Zn) | DUO1-ACTIVATED ZINC FINGER2 | AT4G35280 | 3E-18 | 32.2 |
CUFF.2134 b | PHD-finger | MALE MEIOCYTE DEATH1 | AT1G66170 | 2E-92 | 26.0 |
CUFF.15606 | MYB | MYB DOMAIN PROTEIN 98 | AT4G18770 | 2E-25 | 4.2 |
CUFF.11305 b | bHLH | ABORTED MICROSPORES | AT2G16910 | 2E-26 | 3.2 |
a RPKM value of the antheridium.
b The expression in antheridia was validated by RT–PCR ( Fig. 3 ).
To investigate spatial and temporal expression patterns of these TF genes, we performed RNA in situ hybridization experiments. We chose a Plant Homeodomain zinc finger (PHD) family gene (CUFF.8237), because the RPKM value was high and its Arabidopsis homolog ( MS1 ) has been well characterized ( Table 2 ) ( Ito et al. 2007 , Yang et al. 2007 ). RNA in situ hybridization of antheridiophores at stage 3 or 4 with antisense and sense probes showed that the expression was observed only in the spermatogenous tissue of developing antheridia ( Fig. 4 ; Supplementary Fig. S2 ).
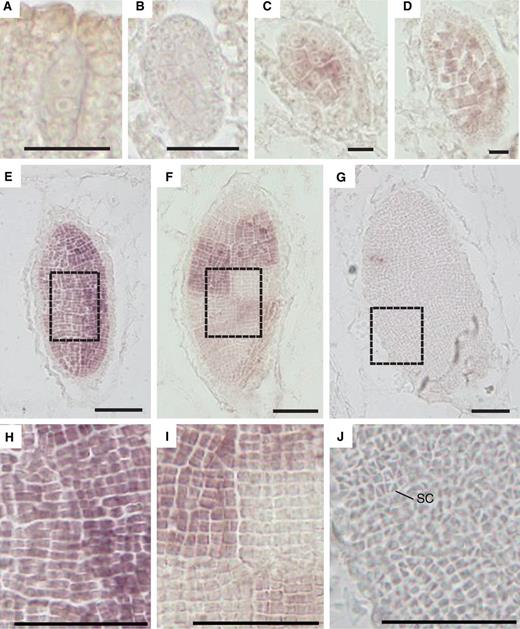
Spatial expression pattern of a PHD-finger TF gene (CUFF.8237) in developing antheridia. RNA in situ hybridization images with an antisense probe of developing antheridia from early to late stages (A–G). Enlargements of spermatogenous tissue in dashed line boxes of E–G are shown (H–J, respectively). SC, sperm cell (J). Low magnification images of the sections hybridized with antisense and sense probes are shown in Supplementary Fig. S2 . Scale bar = 10 µm (A–D) and 200 µm (E–J).
Expression of the PHD family gene was not detected during the early stages of antheridium development and was first detected in spermatogenous cells after a few rounds of proliferative cell divisions ( Fig. 4 A–C). The expression continued during the cell proliferation phase, but ceased toward its end ( Fig. 4 D–F, H, I). After the final diagonal cell division of sperm mother cells, which gives rise to triangular sperm cells, expression was no longer detected ( Fig. 4 G, J). Individual sectors of spermatogenous cells, which represent progeny of individual progenitor cells, showed a patchwork pattern of signals in antheridia at the later stage of cell proliferation ( Fig. 4 F, I). This may reflect differential onset and/or cessation of the proliferation phase among sectors. In addition to TF genes, genes for some channels, transporters, kinases, E3 ubiquitin-protein ligases, a Ras-related protein, a SNARE and a protein phosphatase were preferentially expressed in antheridium ( Supplementary Table S2 ).
Genes for metabolic enzymes preferentially expressed in antheridium
Supplementary Table S3 shows a list of antheridium-enriched genes whose products have homology to metabolic enzymes of other organisms. One interesting category is that of enzymes involved in biosynthesis of cell wall components. CUFF.16188, CUFF.15957 and CUFF.433 are homologs of genes for xyloglucan endotransglucosylase/hydrolase involved in the construction of the primary cell wall in growing tissues. Homologs of genes for biosynthetic enzymes of the secondary cell wall were also found. CUFF.1593 and CUFF.18269 have homology to a hemicellulose glucuronoxylan synthesis enzyme. As was the case for the TF genes described above, genes whose homologs in angiosperms are known to be involved in pollen formation were found among metabolic enzyme genes preferentially expressed in antheridium. In addition to these cell wall-related enzyme genes, other genes encoding enzymes catalyzing the biosynthesis of some lipids or fatty acids were also highly expressed in antheridium.
The homologs of angiosperm genes essential for male gametophyte and gamete formation are expressed in developing antheridia of M. polymorpha
In previous transcriptome studies of pollen or sperm cells of angiosperm plants, it was shown that many of the male gametophyte-specific and male gamete-specific genes are conserved among various angiosperm species. Our transcriptome analysis in this study showed that homologs of some of these conserved genes were preferentially expressed in developing antheridia of M. polymorpha ( Supplementary Table S4 ). In addition to the two TF genes ( DUO1 and DAZ2 ) mentioned above, CUFF.12382 encodes a transmembrane protein with homology to GCS1/HAP2 (GENERATIVE CELL SPECIFIC 1/HAPLESS2; Mori et al. 2006 , von Besser et al. 2006 ) and CUFF.17608 encodes a protein that shares homology with GAMETE EXPRESSED 2 (GEX2) ( Mori et al. 2014 ).
In angiosperms, sperm cell-specific histones distinct from somatic H3.3, such as H3.10 in Arabidopsis, are known ( Okada et al. 2005 , Ingouff and Berger 2010 ). We found that one histone H3.3 gene (CUFF.16312) was specifically expressed in antheridium ( Table 3 ). Among five genes for histone H2B and six genes for histone H4 ( Kawashima et al. 2015 ), one H2B gene and one H4 gene were also specifically expressed in antheridium, and some other core histone genes were not exclusively but were preferentially expressed in antheridium ( Table 3 ).
Gene ID . | Protein name . | RPKM value a . |
---|---|---|
Specifically expressed in antheridium | ||
CUFF.8543 | H1 b | 605.9 |
CUFF.2574 | H2A b, c | 5,032.0 |
CUFF.11153 d | H2B | 655.4 |
CUFF.16312 d | H3.3 | 617.2 |
CUFF.21099 | H4 | 3,047.5 |
Preferentially expressed in antheridium | ||
CUFF.8133 | H2A.Z c | 39.1 |
CUFF.21078 | H2B | 619.5 |
CUFF.1095 | H3.1 e | 1,120.9 |
CUFF.16654 | H3.1 e | 1,826.2 |
CUFF.15356 | cenH3 | 68.1 |
CUFF.17714 | H4 | 600.8 |
CUFF.20690 | H4 | 1.990.6 |
Gene ID . | Protein name . | RPKM value a . |
---|---|---|
Specifically expressed in antheridium | ||
CUFF.8543 | H1 b | 605.9 |
CUFF.2574 | H2A b, c | 5,032.0 |
CUFF.11153 d | H2B | 655.4 |
CUFF.16312 d | H3.3 | 617.2 |
CUFF.21099 | H4 | 3,047.5 |
Preferentially expressed in antheridium | ||
CUFF.8133 | H2A.Z c | 39.1 |
CUFF.21078 | H2B | 619.5 |
CUFF.1095 | H3.1 e | 1,120.9 |
CUFF.16654 | H3.1 e | 1,826.2 |
CUFF.15356 | cenH3 | 68.1 |
CUFF.17714 | H4 | 600.8 |
CUFF.20690 | H4 | 1.990.6 |
a RPKM value of the antheridium.
b A single-copy gene for the protein ( Kawashima et al. 2015 ), but classified as ‘specifically expressed in antheridium’ based on criteria described in the Materials and Methods.
c The protein names according to Kawashima et al. (2015) .
d The expression in antheridia was validated by RT–PCR ( Fig. 3 ).
e The protein names according to Ingouff and Berger (2010) .
Gene ID . | Protein name . | RPKM value a . |
---|---|---|
Specifically expressed in antheridium | ||
CUFF.8543 | H1 b | 605.9 |
CUFF.2574 | H2A b, c | 5,032.0 |
CUFF.11153 d | H2B | 655.4 |
CUFF.16312 d | H3.3 | 617.2 |
CUFF.21099 | H4 | 3,047.5 |
Preferentially expressed in antheridium | ||
CUFF.8133 | H2A.Z c | 39.1 |
CUFF.21078 | H2B | 619.5 |
CUFF.1095 | H3.1 e | 1,120.9 |
CUFF.16654 | H3.1 e | 1,826.2 |
CUFF.15356 | cenH3 | 68.1 |
CUFF.17714 | H4 | 600.8 |
CUFF.20690 | H4 | 1.990.6 |
Gene ID . | Protein name . | RPKM value a . |
---|---|---|
Specifically expressed in antheridium | ||
CUFF.8543 | H1 b | 605.9 |
CUFF.2574 | H2A b, c | 5,032.0 |
CUFF.11153 d | H2B | 655.4 |
CUFF.16312 d | H3.3 | 617.2 |
CUFF.21099 | H4 | 3,047.5 |
Preferentially expressed in antheridium | ||
CUFF.8133 | H2A.Z c | 39.1 |
CUFF.21078 | H2B | 619.5 |
CUFF.1095 | H3.1 e | 1,120.9 |
CUFF.16654 | H3.1 e | 1,826.2 |
CUFF.15356 | cenH3 | 68.1 |
CUFF.17714 | H4 | 600.8 |
CUFF.20690 | H4 | 1.990.6 |
a RPKM value of the antheridium.
b A single-copy gene for the protein ( Kawashima et al. 2015 ), but classified as ‘specifically expressed in antheridium’ based on criteria described in the Materials and Methods.
c The protein names according to Kawashima et al. (2015) .
d The expression in antheridia was validated by RT–PCR ( Fig. 3 ).
e The protein names according to Ingouff and Berger (2010) .
In addition to these conserved genes for male gametophyte development and gametogenesis of angiosperms, some antheridium-expressed genes had homologs which are involved in pollen wall formation in Arabidopsis ( Supplementary Table S4 ). Among these were the aforementioned TFs with homology to MS1 and AMS, respectively ( Table 2 ).
Putative homologs of mammalian genes essential for spermatogenesis and/or sperm function are expressed in developing antheridia of M. polymorpha
We also found antheridium-expressed genes whose homologs are involved in sperm formation and/or function in animals ( Supplementary Table S5 ). As expected, homologs of many flagellar component and assembly genes were expressed in antheridium of M. polymorpha ( Supplementary Table S5 , ‘flagella composition and function’). Spatial and temporal expression patterns of two representative genes were examined by RNA in situ hybridization. The two genes were Mp PACRG (CUFFY.349) ( Yamato et al. 2007 ), a homolog of Parkin co-regulated gene (PACRG) whose product is possibly associated with the axoneme and is essential for normal sperm formation ( Lorenzetti et al. 2004 , Yanagimachi et al. 2004 , Ikeda et al. 2007 ), and Mp LC7 (CUFF.869) encoding one of the light chains of the inner arm dynein in the axoneme. Both genes were specifically expressed in sperm cells undergoing cell morphogenesis (spermiogenesis) ( Fig. 5 ; Supplementary Fig. S3 ). Among the genes involved in sperm formation and function were a homolog of human CHD5 which is required for chromatin remodeling and condensation during spermiogenesis ( Li et al. 2014 , Zhuang at al. 2014 ) ( Supplementary Table S5 , ‘chromosome modification’) and a homolog of adenylate cyclase, which is important for sperm motility in mammals ( Schmid et al. 2007 ) (CUFF.20439 in Supplementary Table S5 ).

Spatial expression patterns of flagella component genes in developing antheridia. RNA in situ hybridization images with antisense probes of Mp PACRG (A–F) and Mp LC7 (G–L) of developing antheridia from early to late stages. Enlargement of spermatogenous tissue in dashed line boxes of C, D, I and J are shown (E, F, K and L, respectively). Low magnification images of the sections hybridized with antisense and sense probes are shown in Supplementary Fig. S3 . Scale bar = 50 µm.
In animal spermiogenesis, a histone to protamine transition represents a distinct event, and this transition is important for condensing chromatin in spermatozoa to change the structure of the nucleus and to protect the paternal genome from damage ( Ward 2010 , Teperek and Miyamoto 2013 , Rathke et al. 2014 ). In previous studies, small basic proteins similar to animal protamines, as defined by a low molecular weight and high arginine content, were detected in nuclear proteins extracted from sperm of Chara (Charophyte), Marchantia (Marchantiophyte) and Marsilea (Monilophyte) ( Reynolds and Wolfe 1978 , Reynolds and Wolfe 1984 ). It was suggested that these protamine-like small basic nuclear proteins replace histones during spermatogenesis in M. polymorpha ( Reynolds and Wolfe 1978 , Kasinsky et al. 2014 ). We therefore searched sperm proteome data for sperm-enriched proteins with high arginine content. We identified a candidate gene from the draft genome sequence, and its antheridium-specific expression was confirmed by our transcriptome data. The identified gene (not included in Supplementary Table S5 , because there is no homology with animal protamines, see below) encodes a protein with an N-terminal linker histone H1-like domain and a C-terminal arginine-rich region that contains two partial amino acid sequences from protamine-like proteins of M. polymorpha recently reported by Kasinsky et al. (2014) ( Supplementary Fig. S4 ). Although the predicted protein has a larger molecular weight than known animal protamines ( Balhorn 2007 ) and previously reported plant protamine-like proteins whose molecular weight was estimated by column chromatography ( Reynolds and Wolfe 1978 , Reynolds and Wolfe 1984 , Kasinsky et al. 2014 ), we named the encoded protein MpPROTAMINE-LIKE (MpPRM). The C-terminal arginine-rich region of MpPRM has no homology to animal protamines, suggesting an independent evolutionary origin. The Mp PRM gene was not expressed during the cell proliferation phase, or in sperm mother cells, but it was expressed in sperm cells undergoing cell morphogenesis at a later stage than those expressing flagella genes ( Fig. 6 A–D; Supplementary Fig. S5 ). We generated transgenic plants expressing the MpPRM protein fused to Citrine fluorescent protein (MpPRM–Citrine) under the control of its own promoter. In these plants, MpPRM–Citrine was localized in the nucleus in mature sperm ( Fig. 6 E, F; Supplementary Fig. S6 ).
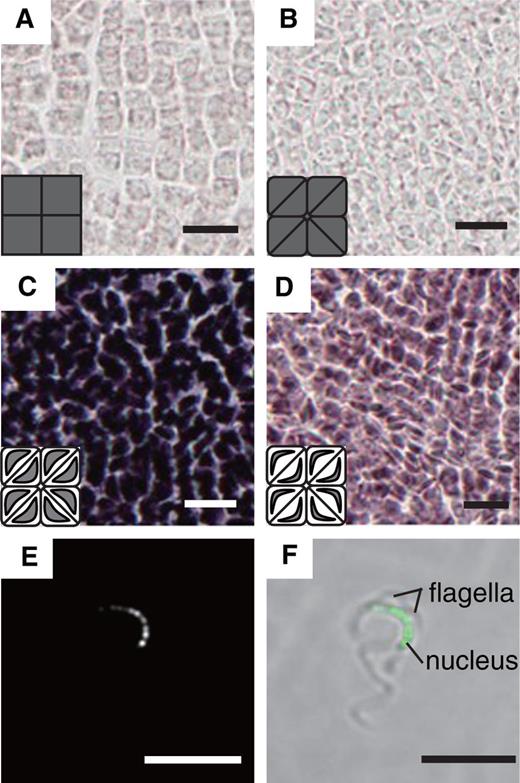
Spatial expression pattern of Mp PRM in developing antheridia and accumulation of MpPRM–Citrine in the sperm nucleus. Low magnification images of the sections hybridized with antisense and sense probes are shown in Supplementary Fig. S5 . Accumulation of MpPRM–Citrine fusion proteins in the nucleus in a mature sperm (E, F). The Citrine fluorescence image (E) and the merged image with a bright-field photograph (F) are shown. Scale bar = 10 µm.
Most of the protein-coding genes on the Y chromosome are expressed in antheridium
In previous work, protein-coding genes located on the Y chromosome were identified ( Yamato et al. 2007 ), and additional genes were found thereafter. We analyzed the expression profiles of these Y-chromosome genes (CUFFY.) in thallus, antheridiophore and antheridium. There were 76 Y-chromosome genes whose RPKM values were >1 (regarded as ‘expressed’ in this study) in at least one of the three organs. All 76 genes were expressed in antheridium ( Supplementary Fig. S7 ). More than 60% (48 genes) were expressed only in male sexual organs (antheridium and antheridiophore), confirming previous findings ( Yamato et al. 2007 ). These genes included homologs of flagella component genes, such as coiled-coil domain-containing protein lobo homolog and dynein regulatory complex protein 1 of Chlamydomonas , and a PACRG homolog (see above) ( Supplementary Table S6 , ‘flagella component’). Other antheridium-expressed Y-chromosome genes included homologs of ubiquitin–proteasome-related proteins, protein kinases or phosphatases, a regulator of gene expression and a Ras-related protein.
Discussion
In this study, we constructed a transcriptional framework of antheridium development in M. polymorpha and compared transcript profiles of developing antheridia with those of other organs. Transcriptome analysis showed that nearly half of the protein-coding genes predicted in the draft genome are expressed in antheridium, and about 15% are specific to antheridium among tested organs. Transcripts from the Y chromosome have higher proportions: 100% of predicted protein-coding genes are expressed in antheridium, and 63% of these are specifically expressed in antheridium. Demands by extensive proliferation of spermatogenous cells, dynamic cell morphogenesis of sperm cells and functional differentiation of jacket cells may explain a high proportion of expressed genes and the uniqueness of the antheridium transcriptome. In Arabidopsis, comparative transcriptome analysis revealed a similar proportion of expressed genes for sperm cell and pollen transcriptomes (26.0% and 32.1%, respectively, of total protein-coding genes) ( Borges et al. 2008 ). GO enrichment analysis suggests that the male germ cell differentiation in developing antheridia of M. polymorpha shares common features of male germ cell development in angiosperms and animals.
As expected in a developmental program that involves extensive cell proliferation and subsequent cell differentiation with dynamic cellular morphogenesis, many genes for signaling pathways including TF genes are preferentially or specifically expressed in developing antheridia. We identified 14 antheridium-specific TF genes which constitute about 4–5% of the TF repertoire of M. polymorpha. Some of them have homology with Arabidopsis TFs known to be involved in male germ cell formation and function. DUO1 and DAZ2 are of particular interest to elucidate regulatory modules conserved among land plants. Because DAZ2 , GCS1/HAP2 and GEX2 are among the transcriptional targets of DUO1 in Arabidopsis ( Brownfield et al. 2009 , Borg et al. 2011 , Borg et al. 2014 ), it is likely that some of the pathways regulated by DUO1 are shared by Arabidopsis and M. polymorpha. The phylogenetic relationship with angiosperm homologs, the spatial and temporal expression patterns and the specific roles in male germ cell formation and function of the four M. polymorpha genes will be particularly interesting to elucidate. Other TFs include those with homology to Arabidopsis TFs that have important roles in formation of pollen and the pollen wall (MMD1/DUET, MS1 and AMS1) ( Reddy et al. 2003 , Sorensen et al. 2003 , Yang et al. 2003 , Ito et al. 2007 , Yang et al. 2007 , Xu et al. 2010 , Xu et al. 2014 ). Interestingly, homologs of genes for biosynthetic enzymes involved in pollen wall formation in angiosperms were also found among antheridium-enriched genes. Because these TFs and enzymes function in diploid cells in angiosperms (e.g. pollen mother cells and tapetum cells) whose counterparts do not exist in antheridium, it is of particular interest to elucidate the functional roles of M. polymorpha genes in antheridium development. One of these (a gene for a PHD family TF which has homology to MS1) may have a role in the proliferation of spermatogenous cells (germ cells in M. polymorpha ). Extensive cell proliferation, which entails synthesis of the primary cell wall and cell membrane, may explain the enrichment of biosynthesis genes for cell wall components and some lipids or fatty acids. Jacket cells, which develop a thick cell wall during maturation of antheridia, could be another reason for enrichment of cell wall biosynthesis genes. In Marchantiaceae including M. polymorpha , jacket cells of antheridia play an important role in discharging mature antherozoid cell mass by extensive swelling of the cell wall upon water absorption ( Bergdolt 1926 , Goebel 1930 , Andersen 1931 ).
Although male gametogenesis in M. polymorpha and angiosperms shares many common features, there are interesting differences besides those associated with motility. One is the presence of a small arginine-rich protein (MpPRM) in the nucleus of mature antherozoids in M. polymorpha. The N-terminal histone H1-like domain in MpPRM is reminiscent of protamine-like proteins from chordates ( Lewis et al. 2004 , Balhorn 2007 ). Whether MpPRM undergoes a similar post-translational processing as reported for tunicate protamine-like proteins to produce a smaller protein containing only the C-terminal arginine-rich domain and whether MpPRM is essential for nuclear compaction in mature antherozoids are important issues to be investigated. Another interesting difference is the absence of specific histone H3 variants distinct from somatic H3.3 found in angiosperms ( Ingouff and Berger 2010 , Talbert et al. 2012 ). The antheridium-specific H3 variant does not differ from somatic H3.3 in amino acid sequence in M. polymorpha. It was shown that sperm cell-specific variant H3.10 is completely removed and replaced by another H3.3 upon fertilization in Arabidopsis ( Ingouff et al. 2007 ). Therefore, the role of the antheridium-specific H3.3 in sperm cell differentiation and its dynamics during fertilization will be an interesting problem to resolve.
In conclusion, our present work provides a useful information resource to study male germ cell development and function in a basal land plant, M. polymorpha. The relative ease of collection of antheridia of similar size and availability of marker genes such as those described in this study will facilitate further analysis with fine temporal resolution. Isolated antheridia are also suitable for proteome and metabolome analysis. Because genes for a wide variety of metabolic enzymes are expressed in developing antheridia, combined omics approaches should help us understand the cellular processes during male germ cell differentiation. Homologous recombination and genome editing techniques are available in M. polymorpha ( Ishizaki et al. 2013 , Sugano et al. 2014 ). Therefore, comprehensive analysis of the functional roles of genes of interest such as those for 14 TFs, are feasible. Because most of the antheridium-specific genes are expected to be dispensable for vegetative development and asexual propagation by gemmae, M. polymorpha should be particularly amenable to the study of conserved processes and pathways in male germ cell formation, which are sometimes hindered in angiosperms or animals due to sterility. Marchantia polymorpha represents basal land plants and shares recent evolutionary history with flowering plants; yet, at the same time, it shares motile gametes with animals. Therefore, further study of gametogenesis in M. polymorpha should provide us with evolutionary insights into the sexual reproduction process in land plants as well as developmental insights into formation and function of motile gametes in general.
Materials and Methods
Plant materials and growth conditions
Male and female accessions of Marchantia polymorpha L., Tak-1 and Tak-2, respectively ( Ishizaki et al. 2008 ), were used. Plants were cultured on half-strength Gamborg’s B5 medium containing 1% sucrose and 1.3% agar under continuous light condition from white fluorescent tubes (50–60 µmol m −2 s −1 ) at 22°C. To induce sexual reproduction, thalli developed from gemmae on half-strength Gamborg’s B5 medium were transferred to soil under continuous white light supplemented with far-red light irradiation as described previously ( Chiyoda et al. 2008 ).
Sampling of antheridia, RNA extraction and Illumina sequencing
Developing antheridia above approximately 200 µm in length were manually dissected out of hand-sliced antheridiophore receptacles excised from stage 3 to stage 5 antheridiophores (see Fig. 1 E). Collected samples were divided into two pools of approximately 200 antheridia. Antheridial receptacles from stage 4 antheridiophores and archegonial receptacles from stage 4 archegoniophores were collected and each divided into three pools. Three thallus samples were collected from 11-day-old gametophytes in vegetative phase developed from gemmae.
Total RNA was extracted with an RNeasy Plant Mini Kit (QIAGEN) according to the manufacturer’s protocol. The quality and quantity of resultant total RNA were evaluated using a NanoDrop 2000c spectrophotometer (Thermo Fisher Scientific), Qubit 2.0 Fluorometer (Life Technologies) and a Bioanalyzer RNA6000 Nano Kit (Agilent Technologies). The sequence libraries were constructed with a TruSeq RNA Sample Prep Kit v2 (Illumina) according to the manufacturer’s protocol. The quality and quantity of each library were determined by using a Bioanalyzer with High Sensitivity DNA Kit (Agilent Technologies) and KAPA Library Quantification Kit for Illumina (Kapa Biosystems). Equal amounts of each library were mixed to make the 2 nM pooled library. Illumina sequencing was performed using a Hiseq2500 platform (Illumina) to produce 101 bp single-end data. All of the reads obtained have been deposited in the DDBJ BioProject and BioSample databases under accession numbers DRA004243 (DRA), PRJDB4420 (BioProject) and SAMD00044058–SAMD00044068 (BioSample).
Data analysis
The resultant reads were mapped to the draft genome of M. polymorpha by TopHat ver. 2.0.14 ( Trapnell et al. 2009 ) with default parameters. To identify differentially expressed genes (DEGs), the mapped reads from different samples were compared by Cuffdiff ver. 2.2.1 ( Trapnell et al. 2013 ) using default parameters and our in-house transcript annotation generated on a preliminary genome assembly provided by the Joint Genome Institute in December, 2011. We selected genes showing an i -value of ≤0.05 between antheridium and thallus and between antheridium and archegoniophore. Among DEGs, genes with a log2-fold change of >3 were considered as preferentially expressed in antheridium or antheridium enriched, and those with an RPKM value of ≥1 in antheridium and with an RPKM value of <1 in thallus were considered as antheridium specific. GO enrichment analysis was performed using the biological networks gene ontology tool, BiNGO ver.2.44 with a corrected P -value of <0.05 ( Maere et al. 2005 ). Hierarchical clustering was performed in the R (ver. 3.1.1) programming environment using the hclust function. The supercomputers of the Academic Center for Computing and Media Studies at Kyoto University and of the Computing Center at the Faculty of Biology-Oriented Science and Technology, Kinki University were used for computation.
RNA in situ hybridization
A fragment for each gene was amplified from M. polymorpha cDNA with a set of gene-specific primers ( Supplementary Table S2 ) and was cloned into the pCR-BluntII-TOPO vector (Life Technologies), which contains SP6 and T7 polymerase-binding sites ( Supplementary Table S2 ). Digoxigenin-labeled RNA probes were synthesized with a DIG RNA Labeling kit (SP6/T7) (Roche) according to the manufacturer’s instructions, using PCR-amplified fragments with a gene-specific primer and M13DL Fw or another gene-specific primer and M13DL Rv ( Supplementary Table S2 ) as templates for antisense probe and sense probe, respectively.
Antheridiophores were fixed in a solution containing 3% paraformaldehyde and 0.25% glutaraldehyde in 0.1 M phosphate buffer, pH 7.0, and 0.05% Triton X-100, dehydrated with an ethanol series, then a lemosol series, and finally embedded in Paraplast (Leica). Samples were sectioned at a thickness of 8 µm with a microtome (RM2155; Leica) and applied to an APS-coated glass slide (Matsunami Glass Ind.). Sections were prepared for in situ hybridization according to Kouchi and Hata (1993) with some optimization for M. polymorpha. Sections were deparaffinized twice in lemosol for 10 min each, rehydrated through a graded ethanol series and then air-dried. They were treated with 1 µg ml –1 proteinase K (Thermo Fisher Scientific) in 100 mM Tris–HCl, pH 8.0, 50 mM EDTA, pH 8.0 at 37°C for 30 min and then washed with phosphate-buffered saline (PBS; 7 mM Na 2 HPO 4 , 3 mM NaH 2 PO 4 , 130 mM NaCl) for 2 min. Proteinase K treatment was terminated with a 2 mg ml –1 glycine solution for 2 min and then sections were washed twice with PBS for 2 min each. The samples were subsequently fixed in 4% paraformaldehyde in PBS for 10 min and washed twice with PBS for 5 min each. Then they were treated with 0.5% acetic anhydride in 100 mM triethanolamine for 10 min, washed twice with PBS for 5 min each, dehydrated through an ethanol series and air-dried. Sections were incubated at 55°C for 2 h in pre-hybridization buffer (50% formamide, 5× SSC, 40 µg ml –1 salmon sperm DNA) and then in the same buffer with 100 ng of probes for >16 h. After hybridization, washing steps were performed as follows: once in 2 × SSC at 55°C for 1 h, once in RNase A (50 µg ml –1 , Sigma) in RNase buffer (10 mM Tris–HCl, pH 8.0, 500 mM NaCl, 1 mM EDTA) at 37°C for 30 min, three times in RNase buffer at 37°C for 5 min, once in 0.2 × SSC at 55°C for 1 h and once in PBS for 5 min. The slides were soaked twice with buffer 1 (100 mM Tris–HCl, pH 7.5, 150 mM NaCl) for 5 min each, incubated with 1% Blocking Reagent (Roche) in buffer 1 for 1 h and then incubated with 1/1,000 diluted anti-digoxigenin-AP, Fab fragments (Roche) with buffer 1 containing 1% blocking reagent for 1 h. The slides were subsequently washed three times with buffer 1 for 10 min each, rinsed with buffer 3 (100 mM Tris–HCl, pH 9.5, 100 mM NaCl, 50 mM MgCl 2 ) for 5 min and then covered with NBT/BCIP (Roche) diluted 1/125 in buffer 3. After incubation at 22°C for >16 h in the dark, the reaction was stopped by immersing the slides in TE.
Analysis of MpPRM–Citrine localization by confocal microscopy
In order to generate the MpPRM–Citrine construct, a genomic fragment of Mp PRM , containing a 2 kb region upstream of the putative start codon and the coding region before the stop codon, was amplified by PCR using a set of specific primers ( Supplementary Table S2 ) and cloned into pENTER/D-TOPO (Life Technologies). The Mp PRM genomic fragment was subcloned into pMpGWB107 ( Ishizaki et al. 2015 ) using LR Clonase II (Life Technologies) following the manufacturer’s protocol. The resulting plasmid was transformed into regenerating thalli of Tak-1 as described previously ( Kubota et al. 2013 ). Selection was conducted with 10 mg l –1 hygromycin. The accumuration of MpPRM–Citrine was observed in isolated mature sperm of MpPRM–Citrine line #1. The Citrine fluorescence was excited at 515 nm by an argon laser and detected in a range from 525–565 nm with confocal laser scanning microscopy (FV1000; Olympus).
Detailed procedures of morphological analysis of male sexual organs, RT–PCR analysis and proteomic analysis of mature antherozoids are described in the Supplementary Text .
Funding
This work was supported by the Ministry of Education, Culture, Sports, Science & Technology (MEXT) [Grant-in-Aids for Scientific Research on Innovative Areas 25113005 (to T.A.) and 22112514 and 24112715 (to K.T.Y.)]; the Japan Society for the Promotion of Science (JSPS) [a Grant-in-Aid for Scientific Research (B) 23370033 (to T.A.), a Grant-in-Aid for Challenging Exploratory Research 24657031 (to T.A.) and a Grant-in-Aid for Fellows (26-1153 to A.H. and 24-5453 to M.S)]; the JSPS and the Plant Global Education Project of the Nara Institute of Science and Technology supported by MEXT (to A.H.).
Abbreviations
- AMS
ABORTED MICROSPORES
- APMC
antheridium proper mother cell
- CHD
Chromodomain-helicase-DNA-binding protein
- DAZ
DUO1-ACTIVATED ZINC FINGER
- DEG
differentially expressed gene
- DUO1
DUO POLLEN1
- GCS1/HAP2
GENERATIVE CELL SPECIFIC 1/HAPLESS2
- GEX2
GAMETE EXPRESSED 2
- GO
Gene Ontology
- MMD1
MALE MEIOCYTE DEATH1
- MS1
MALE STERILITY1
- PACRG
Parkin co-regulated gene
- PBS
phosphate-buffered saline
- PHD
Plant Homeodomain zinc finger
- PRM
PROTAMINE-LIKE
- RPKM
read per kilobase per million
- RT–PCR
reverse transcription–PCR
- Tak
Takaragaike
- TF
transcription factor
Disclosures
The authors have no conflicts of interest to declare.
Acknowledgments
We thank Dr. Y. Tomita of our laboratory for excellent technical assistance, Dr. Y. Sakai for providing photographs of gametangiophores in Fig. 1 and Supplementary Fig. S1 , the past and present members of the Araki lab for helpful discussions and comments, and Professor J.A. Hejna for English proofreading. We thank the US Department of Energy Joint Genome Institute for providing the genomic assemblies and transcriptomes of the ongoing M. polymorpha genome project. RNA sequencing experiments were performed as a part of a NIBB Collaborative Research Program (project number 14-726 to K.I.) of the National Institute for Basic Biology, Okazaki, Japan.
References