-
PDF
- Split View
-
Views
-
Cite
Cite
Jo-Anne H Young, David R Andes, Monica I Ardura, Antonio Arrieta, Eric J Bow, Pranatharthi H Chandrasekar, Sharon C A Chen, Sarah P Hammond, Shahid Husain, Sophia Koo, Valéry Lavergne, M Hong Nguyen, Thomas F Patterson, Miranda So, George R Thompson, C Orla Morrissey, Mindy G Schuster, Modeling Invasive Aspergillosis Risk for the Application of Prophylaxis Strategies, Open Forum Infectious Diseases, Volume 11, Issue 3, March 2024, ofae082, https://doi.org/10.1093/ofid/ofae082
- Share Icon Share
Abstract
The epidemiology of invasive aspergillosis (IA) is evolving. To define the patient groups who will most likely benefit from primary or secondary Aspergillus prophylaxis, particularly those whose medical conditions and IA risk change over time, it is helpful to depict patient populations and their risk periods in a temporal visual model. The Sankey approach provides a dynamic figure to understand the risk of IA for various patient populations. While the figure depicted within this article is static, an internet-based version could provide pop-up highlights of any given flow's origin and destination nodes. A future version could highlight links to publications that support the color-coded incidence rates or other actionable items, such as bundles of applicable pharmacologic or non-pharmacologic interventions. The figure, as part of the upcoming Infectious Diseases Society of America's aspergillosis clinical practice guidelines, can guide decision-making in clinical settings.
Invasive aspergillosis (IA) affects patients whose immune systems are compromised. Since these infections can be fatal, patient populations with predictable high levels of risk often receive universal prophylaxis with antifungal agents during their period(s) of high risk. The next iteration of the Infectious Diseases Society of America (IDSA) aspergillosis practice guidelines is under development. Our group is exploring the challenges in modeling Aspergillus infection risk to update the existing 2016 guidelines [1]. In particular, the group has identified the application of prophylaxis strategies as a fundamental portion of the update. Evaluation of the literature is difficult. Published studies have methodological limitations such as single-center reporting, small sample sizes, retrospective study designs, or heterogeneous patient populations that confound our understanding of the role of Aspergillus prophylaxis. Furthermore, the risk within a specific patient population changes over time within a defined “at risk” period. We are reassessing our understanding of the evolving epidemiology of the risk for IA to define who may benefit from prophylaxis, with what agents, and for how long. We are challenged to develop an easily comprehensible model that depicts the various patient populations at risk for IA, the periods of greatest risk, and whether prophylaxis is indicated to mitigate those risks. We believe that the Sankey approach, as below, provides a visual aid to illustrate the dynamic level of risk for IA in various patient populations and that might, in turn, inform the need for recommending antifungal prophylaxis.
THE SANKEY DIAGRAM
Sankey diagrams are a type of flow diagram in which variables are listed as nodes. The node's height is proportional to the number of patients at risk, and color coding of the flow between nodes can express additional variables [2]. In 1812, a famous diagram by Charles Minard used an overlay of a geographical map, later recognized as a Sankey model, to describe Napoleon's Russian campaign [3]. In 1898, Captain Matthew Sankey used the diagram that now bears his name to illustrate the energy efficiency of a steam engine [4]. A Sankey diagram does not necessarily start at time zero, nor is time necessarily measured by the width between nodes.
Figure 1 models the complexity of IA risk with the Sankey approach. This static figure starts with the large population of patients at variable risks for IA on the left axis, and thus candidates for anti-Aspergillus prophylaxis. The concluding level of immunocompromise (and thus the degree of risk for this mold infection) is depicted on the right axis. Rows represent general medical conditions with some excess risk for IA compared to the general population. Nodes represent risk events estimated from potential trajectories within these conditions. For example, the patient with newly diagnosed acute myelogenous leukemia (AML) undergoes induction and consolidation chemotherapy, but then the medical path may split toward allogeneic hematopoietic cell transplantation (HCT) if in complete remission, or reinduction chemotherapy if the leukemia is not in remission, differentially augmenting the patient’s risk for IA. Another example is the cystic fibrosis patient whose medical path may or may not include IA, and then may or may not include lung transplantation.
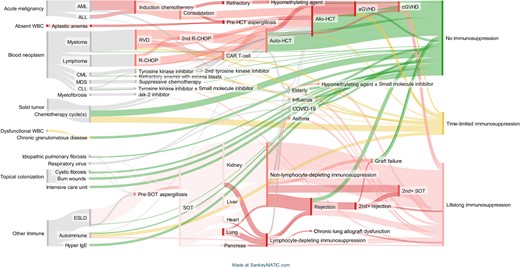
A Sankey plot of patient population groups at risk for invasive aspergillosis (IA), stratified by dynamic changes in immunocompetence. This plot displays transitions in cohort risk. The left axis shows medical conditions that place a patient at risk for IA. Each risk group has a node representing the number of patients, not the relative risk of infection. The height of nodes is weighted to the number of patients within the risk group, except for a minimal risk in the smallest cohorts. The right axis shows the level of immunocompetence at the latest node. This multistate heatmap shows risk change between nodes. Red is major immunosuppression, and there are gradations in color. The deepest red represents high-risk situations where prophylaxis is strongly recommended. Yellow represents variable or brief episodes of immunosuppression signifying an intermediate level of risk where prophylaxis may be needed, particularly where multiple risk factors exist. Green represents circumstances with limited or no ongoing immunocompromise where prophylaxis is not needed. Gray represents limited, but poorly defined, IA risk. Abbreviations: aGVHD, acute graft-vs-host disease; ALL, acute lymphoid leukemia; Allo-HCT, allogeneic hematopoietic cell transplantation; AML, acute myeloid leukemia; Auto-HCT, autologous hematopoietic cell transplantation; CAR T-cell, chimeric antigen receptor T-cell therapy; cGVHD, chronic graft-vs-host disease; CLL, chronic lymphocytic leukemia; CML, chronic myelogenous leukemia; COVID-19, coronavirus disease 2019; ESLD, end-stage liver disease; HCT, hematopoietic cell transplantation; IgE, immunoglobulin E; Jak-2, Janus kinase 2; MDS, myelodysplastic syndrome; R-CHOP, chemotherapy with rituximab, cyclophosphamide, doxorubicin hydrochloride, vincristine sulfate, and prednisone; RVD, chemotherapy with lenalidomide, bortezomib, and dexamethasone; SOT, solid organ transplantation; WBC, white blood cells.
The size of a risk group, the denominator derived from the published literature, is roughly analogous to the node's height. For patient groups at risk of IA that are too small to study, the node's height must maintain a certain legible default minimum. The color coding is descriptive rather than based on published incidence rates. In this figure, any node's width denotes a transition to a new state, not time.
Events with a known high risk of IA have a color code to the node itself. Color coding follows the general traffic light scheme. If there is no exogenous immune system compromise, green is used. If the incidence of IA is known for a general medical condition between events, the flow between nodes is tagged in shades of red through yellow. Since incidence can vary over time (between events) for a single medical condition (a single row), a single row may have different color schemes as the patient's risk journey progresses from left to right across the figure. The figure uses gray to color nodes or nodal flow where the epidemiology is not defined enough to state specific IA risk (a limitation of this figure is that it cannot be interpreted by individuals with color blindness). Additional medical conditions such as uncontrolled cytomegalovirus infection may increase the risk as potentially additive risk factors, but may not be accounted for in the Sankey figure.
When an internet platform is used to host an interactive diagram, the visualization process becomes dynamic. Ben Schmidt used Sankey modeling to show how mapping of >50 college majors predicted later professions [5]. For example, biology majors tended to be associated with a medical career. Clicking the origin node, the college major, will expand all of the professions associated with that node, and vice versa. Clicking on the risk pathway (nodal flow) lists how many individuals answered the survey for this particular node flow.
While the figure within this article is static, an interactive internet-based version could provide pop-up highlights of any given risk flow's origin and destination nodes. A link will be provided once it is available. Placement of the computer cursor over the flow curve could supply evidence links to references supporting the flow and risk level changes between nodes. For example, several patient populations have clearly defined epidemiological risk periods for IA. These populations could be targeted for antifungal prophylaxis of differing intensity or different surveillance intensity during their at-risk period(s).
To support learners, links to classic and contemporary papers defining and contributing to knowledge of Aspergillus epidemiology could be placed here, as well as clinical trial data supporting antifungal prophylaxis in these scenarios. For example, in the 1990s, the bone marrow transplant population was the model for understanding the rapid development of IA after the acute onset of profound neutropenia in the preengraftment period [6]. Hence, antifungal prophylaxis is typically given during periods of neutropenia that are anticipated to last several weeks in these populations. The Sankey figure could link to classic references and to updated high-quality evidence.
UTILITY OF THE SANKEY DIAGRAM AS APPLIED TO INVASIVE ASPERGILLOSIS
The target audience for this figure is broad and includes adult and pediatric infectious disease physicians, hematologists, oncologists, pulmonologists, intensive care physicians, pharmacists, microbiologists, advanced practice providers, clinical caregivers, radiologists, burn and wound specialists, otolaryngologists, transplant surgeons, thoracic surgeons, and perhaps institutional resource utilization administrators. The diagram may also be applicable to outpatient (including hospital in the home) and hospital-based practices. Risk assessment could guide monitoring practices and prophylaxis, but also guide budgeting models for cost estimates of care.
This figure may be useful to providers with varying experience. An early-stage medical provider reviewing this figure will see that HCT recipients and aplastic anemia patients have a high risk for IA. For early-stage learners who are going through their own discovery process to understand the context of when primary antifungal prophylaxis applies to patient populations under their care, color coding can provide a rapid glimpse into the epidemiology.
Clinicians in practice, seasoned or otherwise, who are involved in making decisions regarding stopping prophylaxis based on declining risk, may benefit from anticipating a new upcoming period of risk and the need to restart prophylaxis or continued active surveillance as the level of risk rises. The figure also highlights when secondary prophylaxis may be needed for persons previously successfully treated for IA infection who are entering a new risk phase.
For now, the Sankey diagram is an illustration, which has yet to be validated as an interactive tool before introducing it for clinical applications.
INVASIVE ASPERGILLOSIS IN HEMATOLOGIC MALIGNANCY: DISPLAYING NEW AND SHIFTING RISKS IN THE SANKEY DIAGRAM
Over the last 50 years the treatment of hematological malignancies has evolved [7]. Allogeneic HCT practices have changed to include peripheral blood as a stem cell source [8] and a wider spectrum of alternative donor sources such as cord blood, haploidentical donors, and mismatched unrelated donors [9]. Furthermore, autologous immune effector cell (IEC) therapies are now commonly used to treat lymphoma and multiple myeloma. Trials have supported Aspergillus prophylaxis with posaconazole during induction chemotherapy for AML or myelodysplastic syndrome (MDS) [10] or during the treatment of severe graft-versus-host disease (GVHD) [11, 12]. Furthermore, advanced mold-active azole agents and/or intravenous echinocandin agents are used as antifungal prophylaxis during HCT-related neutropenia based on key randomized clinical trials [13, 14].
There are many nuances in the questions about antifungal prophylaxis that are still unanswered. Hematological malignancies are treated with regimens with multiple concurrent agents over sequential phases such as induction followed by postremission consolidation for AML in adults, and induction, consolidation, late intensification, central nervous system treatment, and maintenance therapy for acute lymphoblastic leukemia (ALL). Treatment duration may span months to years. The Sankey figure shows when many of those phases develop; the gray color reflects uncertain Aspergillus epidemiology and risk. Patient characteristics are also changing; older adults and patients with multiple comorbidities now receive antineoplastic treatment of variable intensity.
Acute leukemia treatment regimens are evolving, resulting in changing IA risks and need for mold-active prophylaxis. For example, the classic “7 + 3” chemotherapy AML cytoreducing induction regimen, based on the antimetabolite cytarabine, is administered continuously for 7 days, along with short infusions of an anthracycline on each of 3 days; this results in a duration of severe neutropenia lasting a median of 26 days. Myelosuppressive agents such as cyclophosphamide, etoposide, antimetabolites (methotrexate, cytarabine, mercaptopurine, and thioguanine), tyrosine kinase inhibitors, or monoclonal antibody constructs added to this core regimen may prolong the period of myelosuppression or immune dysfunction and enhance risk for IA. The IA risk may be significantly lower among recipients of intermediate- or high-dose cytarabine during postremission AML consolidation receiving hematopoietic growth factor support. Newer and somewhat less intensive approaches including venetoclax and other agents (eg, azacitidine) yield risks for IA that are not well defined.
Many clinical treatment regimens for hematologic malignancy place individuals at significant risk for IA, but the efficacy of antifungal prophylaxis has not been studied in these specific populations in randomized clinical trials. There are unanswered questions regarding the periods of highest risk for IA and how to capture these periods in the Sankey diagram. For example, should universal mold-active prophylaxis be used during all phases of the initial treatment of the acute leukemias AML or ALL, or should prophylaxis be targeted for select circumstances (eg, induction chemotherapy for AML where there are high-quality clinical trial data to support its use [10]; salvage chemotherapy for relapsed or refractory disease; preengraftment after myeloablative conditioned allogeneic HCT; and post-allogeneic HCT GVHD [15])? In older or frail adults with ALL, does treatment with a less intensive antileukemic regimen confer the same IA risk as intensive pediatric protocols administered in otherwise healthy adults? What criteria should be used to support discontinuation of prophylaxis following myeloid reconstitution or engraftment? Should drug interactions between novel target cancer therapies and triazole antifungal agents trigger a change in Aspergillus prophylaxis to an antifungal agent with a different mechanism of action, with fewer drug interactions, or to observation without prophylaxis? Does the presence of certain concomitant viral infections such as influenza or coronavirus disease 2019 (COVID-19) impact the aspergillosis risk in this population such that prophylaxis should be extended or reinstituted?
The spectrum of patients potentially at risk for Aspergillus is also evolving in parallel with changes in the types of treatments for various medical conditions [7]. Nonmyeloablative conditioning regimens have permitted older patients to be considered as candidates for allogeneic HCT. The combination of hypomethylating agents and B-cell lymphoma (BCL)-2 inhibition for the treatment of AML and MDS has also extended the candidacy for treatment to older and frailer patients. The use of in vivo allogeneic T-cell depletion or posttransplant cyclophosphamide has extended the spectrum of eligible grafts from haploidentical and mismatched unrelated donors. Similarly, the literature on IA risk conferred by hypomethylating agents combined with the BCL-2 inhibitor venetoclax in AML and MDS patients is very limited, confounding the ability to make robust recommendations regarding mold-active prophylaxis. Several early single-center studies have shown varying risks for IA (between 1% and 6%) and have suggested that mold-active prophylaxis may not impact this risk [16–19].
There are challenges to defining who should receive prophylaxis, the duration of prophylaxis, and when to reevaluate a patient for additional prophylaxis. Risks can be additive or synergistic over time since risk groups and factors are not mutually exclusive. As such, a single patient may manifest differential risk during transition from one state to another. Multiple concurrent risks for a single patient may warrant antifungal prophylaxis when each single risk may not. Additionally, some patients may remain at risk for IA indefinitely.
Chimeric antigen receptor (CAR) T-cell therapy targeting CD19 and B-cell maturation antigen are novel approved IEC therapies for patients with several B-cell malignancies such as recurrent diffuse large B-cell lymphoma, ALL, and relapsed multiple myeloma, respectively. It is expected that the use of CAR T-cell therapy with novel targets in other cancer populations will grow in the next decade. Recipients of currently available CAR T-cell therapy for hematologic malignancy are at some risk for IA; however, because these are novel therapies the epidemiology of this risk remains understudied [20–23]. CAR T-cell–specific complications include cytokine release syndrome and IEC-associated neurotoxicity syndrome, which are treated with immunosuppressive therapies (eg, corticosteroids and tocilizumab) that are associated with an increased risk of infectious complications [24–26]. Another important aspect of the risk for IA after CAR T-cell therapy is the underlying hematologic malignancy; for example, the risk for IA in individuals with relapsed ALL receiving CD19 CAR T-cell therapy would be expected to be higher at baseline than that of an individual receiving CD19 CAR T-cell therapy for relapsed diffuse large B-cell lymphoma where risk of IA is low. Most infectious complications including aspergillosis occur in the first 30 days, but prolonged cytopenias seen in some recipients may extend the IA risk longer. These B-cell–targeting agents also yield prolonged hypogammaglobulinemia, which may augment infectious risks.
Other unanswered questions include whether there should be an absolute threshold of Aspergillus risk applied to all risk groups to trigger prophylaxis or whether there might be some differential mortality by risk group (ie, if some risk groups could have a higher mortality rate if infected, such as older patients, those with pulmonary comorbidities, warranting the recommendation for prophylaxis even if their incidence is lower).
INVASIVE ASPERGILLOSIS IN PEDIATRICS: HOW TO DISPLAY AGE-SPECIFIC RISK IN THE SANKEY DIAGRAM
Studies defining the risk for IA in pediatric populations are needed. There is a broad consensus that pediatric patients receiving chemotherapy for AML should receive primary systemic antifungal prophylaxis while neutropenic; however, the recommendation for ALL is weaker since the risk for IA is treatment protocol- and phase-specific and depends on several factors, such as remission status, adolescent age at malignancy diagnosis, corticosteroid utilization, and subtype of ALL (B- vs T-cell; presence or absence of specific high-risk cytogenetic abnormalities such as the Philadelphia chromosome that need extended chemotherapy). Some subtypes may have intrinsic immunodeficiency tied to the subtype of leukemia or its treatment. Similarly, antifungal prophylaxis should be administered to pediatric patients undergoing allogeneic HCT during preengraftment/early postengraftment periods and for those with active GVHD and ongoing therapy as for adults. Risk of IA appears to be greater during HCT for severe aplastic anemia and Fanconi anemia. Some data also suggest increased risk in nonmyeloablative HCT.
Chronic granulomatous disease is associated with increased invasive Aspergillus pulmonary, bone, and brain infections; prophylaxis has been well studied. Other primary immune deficiencies are also associated with increased risk of Aspergillus infections, but prophylaxis has not been well studied, such as Wiskott-Aldrich, hyper-immunoglobulin E syndromes, and STAT-3 loss-of-function mutations. Increasingly, data support the use of a mold-active agent, particularly a mold-active triazole, where prophylaxis is indicated. Caution is necessary when using triazole agents in children for prophylaxis since there is great interpatient pharmacokinetic variability.
PORTRAYING INVASIVE ASPERGILLOSIS RISK IN SOLID ORGAN TRANSPLANTATION IN THE SANKEY DIAGRAM
The incidence of IA among solid organ transplant (SOT) recipients varies depending on the organ transplanted, with renal transplant carrying the lowest rate (1%–4%) and lung transplant the highest rate (8%–23%) [27, 28]. The risk of IA may be higher with lymphocyte-depleting induction immunosuppression for SOT recipients. Additionally, the depth and breadth of immunosuppression increases during rejection episode treatment courses, subsequent organ transplant, and the fact that SOT recipients mostly receive lifelong immunosuppression. IA after SOT is associated with high rates of graft loss and mortality. Understanding the risk factors for IA among SOT recipients is crucial to select those who might benefit from targeted prophylaxis or preemptive therapy. Risk factors for IA differ according to timeline after SOT, with complicated posttransplant period, repeated bacterial or cytomegaloviral diseases, and need for hemodialysis as risk factors for early onset IA (within 3 months of transplant) and older age, chronic transplant rejection, or graft dysfunction requiring immunosuppression augmentation and renal failure as risk factors for late-onset IA (>3 months after transplant) [29]. Furthermore, besides common risk factors for IA among SOT recipients, each type of organ transplant also imposes its unique risk (eg, extracorporeal membrane oxygenation and generally more intense immunosuppression in heart transplant and single lung transplant, and early airway ischemia in lung transplant). The upcoming IDSA guidelines will review data for lung, heart, liver, pancreas, and kidney.
INVASIVE ASPERGILLOSIS AFTER VIRAL INFECTIONS AND IN OTHER HIGH-RISK SITUATIONS IN THE SANKEY DIAGRAM
Fungal-after-viral infections, including influenza-associated pulmonary aspergillosis (IAPA) and COVID-19–associated pulmonary aspergillosis (CAPA), have become more recognized since the COVID-19 pandemic, even in people without known immunocompromising conditions [30–33]. The incidence of IAPA and CAPA varies widely depending on geographic location, the diagnostic screening methods used at each center, seasonable viral epidemiology, and the case definitions used. The clinical and radiographic findings may differ from classic neutropenia-related invasive Aspergillus infections because of a reduced propensity for angioinvasion. Risk factors for fungal-after-viral infections include the receipt of dexamethasone, tocilizumab, prolonged mechanical ventilation, underlying solid tumors, and recipients of SOT.
Temporary risks of IA among otherwise immunocompetent individuals include dexamethasone treatment of COVID-19 infection, intensive care unit stay, influenza infections in some countries, orthopedic procedures or other surgeries done in operating rooms without HEPA (high-efficiency particulate air) filtration, wounds of burn victims, topical or systemic treatment of asthma, or immune inhibitor treatments for autoimmune conditions for diseases ranging from psoriasis or eczema to inflammatory bowel disease. These concurrent risk factors are listed in gray on the Sankey diagram. Other viral coinfections may also occur, and these are even less well defined.
CONCLUSIONS
While Figure 1 highlights primary Aspergillus prophylaxis needs, the nuances of additional complexities related to prophylaxis require further detail. For example, at what incidence rate of IA should patients with newly diagnosed hematologic malignancy be treated with prophylaxis outside of well-defined patient populations such as those undergoing induction chemotherapy for AML? How do universal prophylaxis strategies compare to diagnostic-driven strategies of careful monitoring with radiography and serodiagnosis and a preemptive or empiric treatment approach? That depends on levels of risk stratification, even within 1 diagnosis. Treatment of the patient with hematological malignancy or MDS may include observation, course(s) with various immune effector therapies if the patient is fit enough, course(s) of gentler but prolonged therapy if elderly, swift progression to allogeneic transplant if there is an evolution to advanced MDS or leukemia, and, in lung transplant recipients, how to define when colonization transitions to infection requiring treatment.
Defining the risk of IA and the best antifungal prophylaxis for each of these treatment approaches requires an understanding of the quantitative risks within the disease process, as well as knowledge of the literature to define the known incidence of aspergillosis for each approach. Local epidemiology probably also matters. Future endeavors include using data from our guideline development to update individual underlying diseases (ie, individual rows on the figure). Separate expanded individual risk diagrams could be based on chemo- and immunotherapeutic management of these varying medical conditions.
Therefore, IA prophylaxis does matter. Prophylaxis can successfully help patients navigate through defined IA high-risk periods to those with lower degrees of immunosuppression or restored immunocompetence. The risk of IA is not static. For example, interim medical complications such as allogeneic HCT–related GVHD-medicated augmented immunosuppression require consideration of reinstitution of prophylaxis for the duration of risk. It is helpful yet challenging to depict these risks in a visual model to define who needs fungal prophylaxis, particularly for the multistate medical patient whose condition and risks change over time.
Notes
Acknowledgments. The authors thank Nitipong Permpalung and Dan Weisdorf for their comments on this manuscript.
References
Author notes
Potential conflicts of interest. All authors: No reported conflicts.
Comments