-
PDF
- Split View
-
Views
-
Cite
Cite
Mohammed A Almatrafi, Victor M Aquino, Tamra Slone, Rong Huang, Michael Sebert, Community Airborne Mold Spore Counts and Invasive Fungal Disease Risk Among Pediatric Hematological Malignancy and Stem Cell Transplant Patients, Open Forum Infectious Diseases, Volume 8, Issue 11, November 2021, ofab481, https://doi.org/10.1093/ofid/ofab481
- Share Icon Share
Abstract
Patients with hematological malignancies and hematopoietic stem cell transplantation (HSCT) recipients are at risk of developing invasive fungal infections, but the quantitative risk posed by exposure to airborne mold spores in the community has not been well characterized.
A single-institution, retrospective cohort study was conducted of pediatric patients treated for hematological malignancies and HSCT recipients between 2014 and 2018. Patients with invasive fungal disease (IFD) due to molds or endemic fungi were identified using published case definitions. Daily airborne mold spore counts were obtained from a local National Allergy Bureau monitoring station and tested for association with IFD cases by 0-inflated Poisson regression. Patients residing outside the region or with symptom onset more than 2 weeks after admission were excluded from the primary analysis.
Sixty cases of proven or probable IFD were identified, of whom 47 cases had symptom onset within 2 weeks of admission and were therefore classified as possible ambulatory onset. The incidence of ambulatory-onset IFD was 1.2 cases per 10000 patient-days (95% CI, 0.9–1.7). A small excess of ambulatory-onset IFD was seen from July through September, during which period spore counts were highest, but this seasonal pattern did not reach statistical significance (P = .09). No significant association was found between IFD cases and community mold spore counts over intervals from 1 to 6 weeks before symptom onset.
There was no significant association between IFD cases and community airborne mold spore counts among pediatric hematological malignancy and HSCT patients in this region.
Invasive fungal diseases (IFDs) occur in ~2% and ~5% of patients with hematological malignances and hematopoietic stem cell transplantation (HSCT) recipients, respectively [1–4]. These infections are life-threatening, with reported mortality rates as high as 30%–90% [1, 5, 6]. Host factors associated with IFD have been previously characterized, but our understanding of the mechanisms and routes of acquisition of such infections is still incomplete [7–11]. Although inhalation of mold spores is considered one of the primary methods of acquisition of IFD, the duration and intensity of exposure required to initiate infection are not well defined [12].
Efforts to prevent invasive fungal infections in patients with hematologic malignancies and following HSCT have focused on reducing exposure during periods of hospitalization. These strategies have been guided by investigations of prior outbreaks among hospitalized patients, often in the setting of construction activities [13–19]. With adoption of measures to reduce inpatient mold spore exposure and the use of granulocyte colony stimulating factor to promote neutrophil recovery, the frequency of hospital-onset invasive mold infections appears to be decreasing [13, 20]. However, there has been a shift in timing of invasive aspergillosis, with such infections occurring as late complications in stem cell transplant patients after discharge [3, 13, 21]. This observation raises questions about the impact of exposures in the community and the relevance of seasonal variation in environmental mold spore levels to the development of invasive fungal disease.
Several studies have examined the relationship between community airborne mold spore counts and community-onset invasive aspergillosis (IA) [4, 22, 23]. The findings of these studies, however, have been inconsistent. Published reports from Grenoble, France, Barcelona, Spain, and Seattle, Washington, found significant positive associations between community-onset IA and such spore counts. However, a report from Houston, Texas, which is located much closer to our institution than the other sites, did not show a similar association. These conflicting results could in part reflect geographic variation in climate and airborne mold spore levels in different locales. The question of whether there may be a seasonal component to risk of invasive mold infections has also not been resolved. Significant seasonal variation has been reported from Seattle in the incidence of both IA among HSCT patients [4] and sinopulmonary Mucorales infections in hematologic malignancy patients [24]. In contrast, a multicenter study from France did not show a seasonal pattern in IA rates using aggregated data [25] but could have obscured local trends at individual sites through pooling of results.
During the late summer and early fall of 2016 and again during the same period in 2017, our institution noted an apparent increase in invasive mold infections among oncology patients, most of which had onset near the time of admission. As part of the investigation of these infections, data on airborne mold spore counts in the community were examined from a local National Allergy Bureau (NAB) monitoring station. These measurements showed strong apparent seasonal variation, with higher counts in summer and early fall when clinicians had reported increased infections.
We therefore sought to assess whether there was a consistent, long-term association between community airborne mold spore counts and risk of fungal infections in ambulatory pediatric patients with hematological malignancies or who had undergone HSCT. Our analysis included both invasive mold infections and endemic mycoses as types of fungal infection likely to be transmitted by an airborne route. We were particularly interested in whether measurements from a single regional station could be used to predict risk across the entire metropolitan area in a way that might be useful for guiding prevention strategies such as antifungal prophylaxis or exposure reduction measures.
METHODS
Study Design and Population
A retrospective cohort study was performed that included all hospitalized patients at our pediatric teaching hospital in Dallas, Texas, who received therapy for acute lymphocytic leukemia (ALL), acute myelogenous leukemia (AML), biphenotypic leukemia, or lymphoma during the study period from January 1, 2014, through December 31, 2018. Patients were also included who during this period were cared for at our facility within 6 months after receipt of HSCT. The Institutional Review Board of the University of Texas Southwestern Medical Center approved the study.
Patients were identified through an electronic medical record search for International Classification of Diseases, Ninth Revision and Tenth Revision, codes. Lists generated through these searches were cross-referenced with Oncology and HSCT departmental databases to identify gaps in the coding-based search. For each patient, dates of entry and exit from the cohort were determined so that the size of the at-risk cohort could be calculated for each day of the study period. Cohort entry was defined by either initiation of oncology care at our institution or date of HSCT. Exit dates were defined as the first occurrence of completion of therapy, transfer to another institution, or death. For leukemia and lymphoma patients, HSCT or chimeric antigen receptor T-cell therapy also ended inclusion in the former subsets of the cohort. HSCT patients exited the cohort 6 months after transplant if another termination event did not occur earlier. Patients who relapsed off therapy were reentered at the date of relapse.
Medical records were reviewed for every encounter where antifungal therapy was given, and autopsy records were also reviewed to identify patients who may not have received pre-mortem antifungal therapy. Baseline demographic data were collected on all IFD patients including gender, ethnicity, underlying diagnosis, age at diagnosis, and county of residence. Residence within the Dallas–Fort Worth (DFW) metroplex was defined as living in 1 of 11 counties at the time of symptom onset.
Patients were classified into proven, probable, and possible disease categories based on case definitions developed by the European Organization for Research and Treatment of Cancer/Invasive Fungal Infections Cooperative Group and the National Institute of Allergy and Infectious Diseases Mycoses Study Group (EORTC/MSG) Consensus Group [8]. Criteria for proven invasive yeast infection and probable disseminated candidiasis were not employed due to the focus of our study on environmental mold exposure. We have retained use of the term IFD to describe this set of infections because patients with invasive mold infections and endemic mycoses are both included. One patient with candidemia who also met criteria for probable pulmonary invasive fungal infection was included, but other patients with only Candida infections were excluded. Analysis was limited to patients with proven or probable IFD. In order to focus on the patients most likely to have acquired infection through exposure to mold spores in the community, we defined ambulatory-onset infections as those with symptom onset no more than 14 days after admission and limited our primary analysis to these cases. Because the range of incubation periods for such environmental fungal infections is uncertain, we included all patients with proven or probable IFD in a secondary analysis regardless of onset timing.
Environmental Data and Samples
Daily readings of airborne mold spore counts were obtained from data published online by an NAB monitoring station in north Dallas (http://entdocs.com/pollen-count/). Culturable airborne mold data were obtained from the outdoor control measurements taken by the hospital’s Infection Prevention department as part of monthly surveillance of indoor air quality. These specimens were obtained by passing 1-m3 volumes of air through a Zefon (Ocala, FL, USA) A6 Bioaerosol sampler and sent to Aerobiology Laboratory Associates, Inc. (Golden, CO, USA), for culture and identification. Precipitation data were obtained from the DFW Metroplex Weather Records database (https://www.iweathernet.com/texas-dfw-weather-records).
Statistical Analyses
The primary analysis tested for an association between community airborne mold spore counts and ambulatory-onset IFD in patients with hematological malignancies and HSCT residing within the DFW area. Secondary analyses included testing for associations between spore counts and all IFD cases (regardless of residence or onset classification), testing for seasonal variation in rates of IFD, and testing for an association between precipitation and IFD.
Data on IFD cases were collected in a RedCap database [26], and analyses were performed using SAS statistical software, version 9.4. Categorical variables were expressed as counts or percentages, whereas continuous data were described as means, medians, and interquartile ranges. Seasonal variation in environmental mold spore counts was analyzed using nonlinear regression (GraphPad Prism, version 8.3.1). The association between community airborne mold spore counts and risk of IFD was tested using 0-inflated Poisson regression. Effects of changes in size of the patient population over the study period on the number of observed IFD cases were taken into account by including the daily cohort size as a variable in the model. The threshold for statistical significance was set at P < .05.
A bootstrap approach was also used as a supplemental method for estimating P values for the association between spore counts and IFD risk. In this approach, the distribution of possible spore counts (under the null hypothesis of no association between spore counts and IFD cases) was determined based on the preceding spore counts for each day of the study period. These preceding spore counts for each date were weighted according to the cohort size on that date. Spore counts were randomly drawn from this distribution to generate test sets equal in size to the observed set of IFD cases. After generation of 10000 test sets, the bootstrap probability of observing at random a median spore count as high as or higher than that seen for the actual IFD cases was determined.
RESULTS
IFD Cases
Sixty patients with proven or probable IFD were identified during the period from 2014 to 2018. Baseline demographic and clinical details are summarized in Table 1. The median age at IFD diagnosis (interquartile range [IQR]) was 10 (4–15) years, and 37 (62%) patients were male. The most common underlying diagnoses were ALL (70%) and HSCT (22%). Forty-seven IFD cases were classified as having probable ambulatory onset (78%). Common fungal organisms identified in patient specimens included Aspergillus (17%) and Curvularia (15%), followed by Rhizopus, Bipolaris, and Alternaria (7% each). Three patients had co-infections with more than 1 fungal type.
. | DFW Area Patients . | Non–DFW Area Patients . |
---|---|---|
n = 42, No. (%) . | n = 18, No. (%) . | |
Age, median (IQR), y | 11 (4.5–15) | 9 (2–13) |
Male gender | 25 (60) | 12 (67) |
Race | ||
White | 34 (81) | 14 (78) |
Black | 2 (5) | 3 (17) |
Asian | 2 (5) | 0 |
Native American | 1 (2) | 0 |
Multiple/unknown/not reported | 3 (7) | 1 (6) |
Ethnicity | ||
Hispanic | 20 (48) | 8 (44) |
Non-Hispanic | 22 (52) | 10 (56) |
Underlying condition | ||
ALL | 30 (71) | 12 (67) |
AML | 0 | 1 (6) |
Lymphoma | 2 (5) | 1 (6) |
Mixed phenotype leukemia | 0 | 1 (6) |
HSCT | 10 (24) | 3 (17) |
IFD category | ||
Proven IFD | 21 (50) | 10 (56) |
Probable IFD | 21 (50) | 8 (44) |
Symptom onset | ||
≤14 d from admission | 35 (83) | 12 (67) |
>14 d from admission | 7 (17) | 6 (33) |
Infection site | ||
Pulmonary | 26 (62) | 10 (56) |
Sinonasal | 10 (24) | 8 (44) |
Abdominal/pelvic | 1 (2) | 0 |
Cutaneousa | 4 (10) | 1 (6) |
Disseminated | 2 (5) | 1 (6) |
Cerebral | 1 (2) | 0 |
Fungal pathogenb | ||
Aspergillus | 7 (17) | 3 (17) |
Penicillium | 1 (2) | 0 |
Fusarium | 2 (5) | 1 (6) |
Rhizopus | 4 (10) | 0 |
Alternaria | 2 (5) | 2 (11) |
Bipolaris | 2 (5) | 2 (11) |
Curvularia | 5 (12) | 4 (22) |
Exserohilum | 1 (2) | 0 |
Histoplasma | 2 (5) | 0 |
. | DFW Area Patients . | Non–DFW Area Patients . |
---|---|---|
n = 42, No. (%) . | n = 18, No. (%) . | |
Age, median (IQR), y | 11 (4.5–15) | 9 (2–13) |
Male gender | 25 (60) | 12 (67) |
Race | ||
White | 34 (81) | 14 (78) |
Black | 2 (5) | 3 (17) |
Asian | 2 (5) | 0 |
Native American | 1 (2) | 0 |
Multiple/unknown/not reported | 3 (7) | 1 (6) |
Ethnicity | ||
Hispanic | 20 (48) | 8 (44) |
Non-Hispanic | 22 (52) | 10 (56) |
Underlying condition | ||
ALL | 30 (71) | 12 (67) |
AML | 0 | 1 (6) |
Lymphoma | 2 (5) | 1 (6) |
Mixed phenotype leukemia | 0 | 1 (6) |
HSCT | 10 (24) | 3 (17) |
IFD category | ||
Proven IFD | 21 (50) | 10 (56) |
Probable IFD | 21 (50) | 8 (44) |
Symptom onset | ||
≤14 d from admission | 35 (83) | 12 (67) |
>14 d from admission | 7 (17) | 6 (33) |
Infection site | ||
Pulmonary | 26 (62) | 10 (56) |
Sinonasal | 10 (24) | 8 (44) |
Abdominal/pelvic | 1 (2) | 0 |
Cutaneousa | 4 (10) | 1 (6) |
Disseminated | 2 (5) | 1 (6) |
Cerebral | 1 (2) | 0 |
Fungal pathogenb | ||
Aspergillus | 7 (17) | 3 (17) |
Penicillium | 1 (2) | 0 |
Fusarium | 2 (5) | 1 (6) |
Rhizopus | 4 (10) | 0 |
Alternaria | 2 (5) | 2 (11) |
Bipolaris | 2 (5) | 2 (11) |
Curvularia | 5 (12) | 4 (22) |
Exserohilum | 1 (2) | 0 |
Histoplasma | 2 (5) | 0 |
Abbreviations: ALL, acute lymphocytic leukemia; AML, acute myelogenous leukemia; DFW, Dallas–Fort Worth; HSCT, hematopoietic stem cell transplantation; IFD, invasive fungal disease; IQR, interquartile range.
Patients with disseminated fusariosis and skin biopsies showing fungal elements were classified as disseminated rather than cutaneous infection.
Pathogens were identified by culture, polymerase chain reaction, or Aspergillus galactomannan assay.
. | DFW Area Patients . | Non–DFW Area Patients . |
---|---|---|
n = 42, No. (%) . | n = 18, No. (%) . | |
Age, median (IQR), y | 11 (4.5–15) | 9 (2–13) |
Male gender | 25 (60) | 12 (67) |
Race | ||
White | 34 (81) | 14 (78) |
Black | 2 (5) | 3 (17) |
Asian | 2 (5) | 0 |
Native American | 1 (2) | 0 |
Multiple/unknown/not reported | 3 (7) | 1 (6) |
Ethnicity | ||
Hispanic | 20 (48) | 8 (44) |
Non-Hispanic | 22 (52) | 10 (56) |
Underlying condition | ||
ALL | 30 (71) | 12 (67) |
AML | 0 | 1 (6) |
Lymphoma | 2 (5) | 1 (6) |
Mixed phenotype leukemia | 0 | 1 (6) |
HSCT | 10 (24) | 3 (17) |
IFD category | ||
Proven IFD | 21 (50) | 10 (56) |
Probable IFD | 21 (50) | 8 (44) |
Symptom onset | ||
≤14 d from admission | 35 (83) | 12 (67) |
>14 d from admission | 7 (17) | 6 (33) |
Infection site | ||
Pulmonary | 26 (62) | 10 (56) |
Sinonasal | 10 (24) | 8 (44) |
Abdominal/pelvic | 1 (2) | 0 |
Cutaneousa | 4 (10) | 1 (6) |
Disseminated | 2 (5) | 1 (6) |
Cerebral | 1 (2) | 0 |
Fungal pathogenb | ||
Aspergillus | 7 (17) | 3 (17) |
Penicillium | 1 (2) | 0 |
Fusarium | 2 (5) | 1 (6) |
Rhizopus | 4 (10) | 0 |
Alternaria | 2 (5) | 2 (11) |
Bipolaris | 2 (5) | 2 (11) |
Curvularia | 5 (12) | 4 (22) |
Exserohilum | 1 (2) | 0 |
Histoplasma | 2 (5) | 0 |
. | DFW Area Patients . | Non–DFW Area Patients . |
---|---|---|
n = 42, No. (%) . | n = 18, No. (%) . | |
Age, median (IQR), y | 11 (4.5–15) | 9 (2–13) |
Male gender | 25 (60) | 12 (67) |
Race | ||
White | 34 (81) | 14 (78) |
Black | 2 (5) | 3 (17) |
Asian | 2 (5) | 0 |
Native American | 1 (2) | 0 |
Multiple/unknown/not reported | 3 (7) | 1 (6) |
Ethnicity | ||
Hispanic | 20 (48) | 8 (44) |
Non-Hispanic | 22 (52) | 10 (56) |
Underlying condition | ||
ALL | 30 (71) | 12 (67) |
AML | 0 | 1 (6) |
Lymphoma | 2 (5) | 1 (6) |
Mixed phenotype leukemia | 0 | 1 (6) |
HSCT | 10 (24) | 3 (17) |
IFD category | ||
Proven IFD | 21 (50) | 10 (56) |
Probable IFD | 21 (50) | 8 (44) |
Symptom onset | ||
≤14 d from admission | 35 (83) | 12 (67) |
>14 d from admission | 7 (17) | 6 (33) |
Infection site | ||
Pulmonary | 26 (62) | 10 (56) |
Sinonasal | 10 (24) | 8 (44) |
Abdominal/pelvic | 1 (2) | 0 |
Cutaneousa | 4 (10) | 1 (6) |
Disseminated | 2 (5) | 1 (6) |
Cerebral | 1 (2) | 0 |
Fungal pathogenb | ||
Aspergillus | 7 (17) | 3 (17) |
Penicillium | 1 (2) | 0 |
Fusarium | 2 (5) | 1 (6) |
Rhizopus | 4 (10) | 0 |
Alternaria | 2 (5) | 2 (11) |
Bipolaris | 2 (5) | 2 (11) |
Curvularia | 5 (12) | 4 (22) |
Exserohilum | 1 (2) | 0 |
Histoplasma | 2 (5) | 0 |
Abbreviations: ALL, acute lymphocytic leukemia; AML, acute myelogenous leukemia; DFW, Dallas–Fort Worth; HSCT, hematopoietic stem cell transplantation; IFD, invasive fungal disease; IQR, interquartile range.
Patients with disseminated fusariosis and skin biopsies showing fungal elements were classified as disseminated rather than cutaneous infection.
Pathogens were identified by culture, polymerase chain reaction, or Aspergillus galactomannan assay.
The overall incidence of ambulatory-onset IFD was estimated to be 1.2 cases per 10000 cohort patient-days (95% CI, 0.9–1.7). There was no statistically significant difference in IFD incidence among different underlying diagnoses (Table 2). Ambulatory-onset IFD risk was likewise similar among these diagnostic categories when calculated per patient treated rather than per patient-day under treatment. Thirty-five of the 47 patients with ambulatory-onset IFD lived within the DFW area (74%). These patients constituted the primary analysis population for assessment of association between community mold spore counts and ambulatory IFD risk.
Underlying Condition . | No. of IFD Cases . | Incidence per 10000 Patient-Days (95% CI) . | Infection Frequency per 100 Patients (95% CI) . |
---|---|---|---|
ALL | 36 | 1.3 (0.9–1.8) | 7.7 (5.6–10.4) |
AML | 1 | 0.4 (0.02–2.2) | 1.3 (0.1–6.9) |
Lymphoma | 3 | 1.3 (0.4–3.8) | 2.6 (0.7–7.3) |
HSCT | 7 | 2.2 (1.1–4.6) | 3.6 (1.8–7.3) |
All diagnoses (total) | 47 | 1.2 (0.9–1.7) | 5.3 (4.0–7.0) |
Underlying Condition . | No. of IFD Cases . | Incidence per 10000 Patient-Days (95% CI) . | Infection Frequency per 100 Patients (95% CI) . |
---|---|---|---|
ALL | 36 | 1.3 (0.9–1.8) | 7.7 (5.6–10.4) |
AML | 1 | 0.4 (0.02–2.2) | 1.3 (0.1–6.9) |
Lymphoma | 3 | 1.3 (0.4–3.8) | 2.6 (0.7–7.3) |
HSCT | 7 | 2.2 (1.1–4.6) | 3.6 (1.8–7.3) |
All diagnoses (total) | 47 | 1.2 (0.9–1.7) | 5.3 (4.0–7.0) |
Abbreviations: ALL, acute lymphocytic leukemia; AML, acute myelogenous leukemia; HSCT, hematopoietic stem cell transplantation; IFD, invasive fungal disease.
Underlying Condition . | No. of IFD Cases . | Incidence per 10000 Patient-Days (95% CI) . | Infection Frequency per 100 Patients (95% CI) . |
---|---|---|---|
ALL | 36 | 1.3 (0.9–1.8) | 7.7 (5.6–10.4) |
AML | 1 | 0.4 (0.02–2.2) | 1.3 (0.1–6.9) |
Lymphoma | 3 | 1.3 (0.4–3.8) | 2.6 (0.7–7.3) |
HSCT | 7 | 2.2 (1.1–4.6) | 3.6 (1.8–7.3) |
All diagnoses (total) | 47 | 1.2 (0.9–1.7) | 5.3 (4.0–7.0) |
Underlying Condition . | No. of IFD Cases . | Incidence per 10000 Patient-Days (95% CI) . | Infection Frequency per 100 Patients (95% CI) . |
---|---|---|---|
ALL | 36 | 1.3 (0.9–1.8) | 7.7 (5.6–10.4) |
AML | 1 | 0.4 (0.02–2.2) | 1.3 (0.1–6.9) |
Lymphoma | 3 | 1.3 (0.4–3.8) | 2.6 (0.7–7.3) |
HSCT | 7 | 2.2 (1.1–4.6) | 3.6 (1.8–7.3) |
All diagnoses (total) | 47 | 1.2 (0.9–1.7) | 5.3 (4.0–7.0) |
Abbreviations: ALL, acute lymphocytic leukemia; AML, acute myelogenous leukemia; HSCT, hematopoietic stem cell transplantation; IFD, invasive fungal disease.
Seasonal Patterns
More IFD infections were seen in August and September than during other months, but this trend did not reach statistical significance (chi-square test by month, P = .84) (Figure 1A). Post hoc analysis by quarter of the year showed a marginally stronger seasonal association, with 12 IFD cases observed for July to September as compared with 23 during the remainder of the year. This trend again did not reach the predefined threshold for statistical significance (chi-square test by quarter, P = .09).
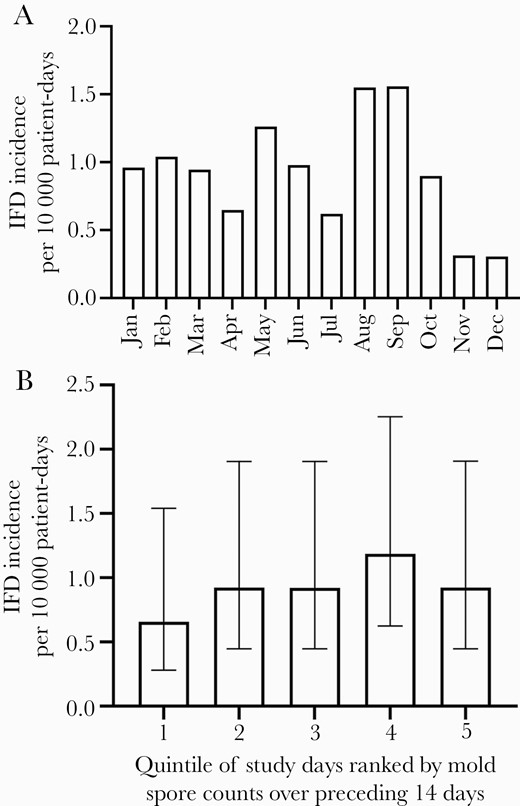
Incidence of onset of ambulatory-onset invasive fungal disease (IFD; from either invasive molds or endemic fungi) in the pediatric hematologic malignancy patients residing in the DFW area (n = 35) by calendar month over the 5-year study period (A) (chi-square test by month, P = .84). Incidence of IFD during quintiles of patient-days sorted from lowest (first quintile) to highest (fifth quintile) according to the average airborne mold spore counts measured over the previous 14 days (B) (chi-square test for trend, P = .47). Error bars give 95% CIs.
Daily airborne mold spore counts measured at the NAB station showed seasonal variation over the study period (Figure 2), with the lowest counts being observed from December through March (median [IQR], 2403 [1860–3178] spores/m3) and highest levels measured from June through October (median [IQR], 5891 [4884–6899] spores/m3). The significance of this annual variation was confirmed by nonlinear regression, which fit the spore count data better when amplitude of the sine wave function was unconstrained (r2 = .45) than when amplitude was constrained to 0 (r2 < .001).
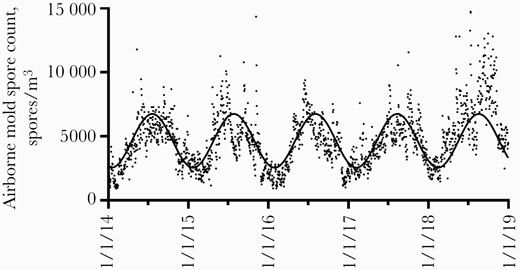
Daily airborne mold spore counts measured at a National Allergy Bureau monitoring station in northern Dallas. Nonlinear regression curve fit to the data is shown (r2 = .45).
Cultured Air Samples
Daily mold spore counts from the NAB station represented an aggregate measure of viable and nonviable spores from potentially pathogenic as well as nonpathogenic fungi. We therefore took advantage of periodic air sampling sent for fungal culture (samples that had been obtained outside our hospital as controls for indoor air quality surveillance) in order to better understand the variability in viable fungal spores present within such community outdoor air samples. These cultured air samples were available approximately monthly from October 2018 through January 2020 and therefore could not be directly correlated with the NAB station counts but likely reflected the general distribution of viable airborne mold spores in our area (Figure 3). Among these cultured air samples, the nonpathogenic species Cladosporium was predominant (median, 27 colony-forming units [CFU]/m3) and represented a median (IQR; maximum) of 78% (54%–86%; 98%) of all fungal CFU isolated in any individual sample. In contrast, pathogenic species represented a much smaller but variable portion of the total fungal CFU, including Aspergillus (median [IQR; maximum], 2% [0%–7%; 23%]) and the dematiaceous molds Curvularia, Bipolaris, and Alternaria (when taken together, median [IQR; maximum], 1% [0%–4%; 17%]).
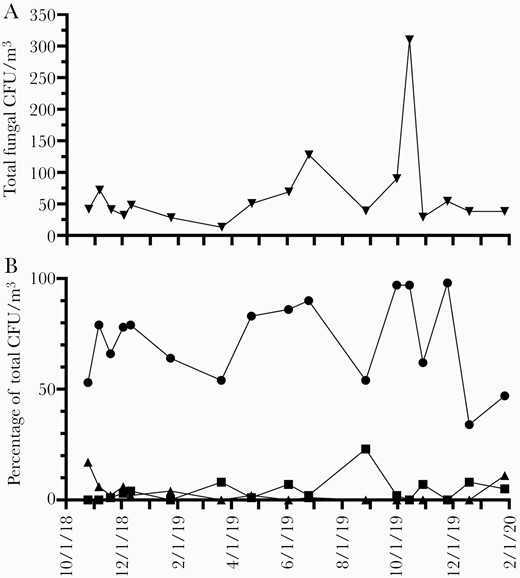
Temporal variation in concentration of total viable fungal colony-forming units (CFU) per cubic meter cultured from outdoor air (A). Relative abundance of representative common (Cladosporium, circles) and pathogenic (Aspergillus, squares; dematiaceous molds, triangles) fungal types cultured from these outdoor air samples (B).
Mold Spore Levels and IFD Risk
The potential for an association between ambulatory-onset IFD cases among patients residing in the DFW area and spore counts reported by the NAB station was examined. Because the incubation period and infectious dose are not well characterized for environmental fungal infections in immunocompromised hosts, we analyzed spore counts over a number of time intervals before onset of symptoms and calculated the exposure burden during these intervals as both the average and maximum spore counts for the time periods. These exposure windows included the periods 1–7 days before symptom onset as well as 1–14 days, 15–28 days, and 29–42 days before symptom onset. Although positive regression coefficients were observed using both the average and maximum spore counts for the period 1–7 days before symptom onset, none of these associations reached statistical significance (coefficient of 0.0001 for both comparisons, with P values of .55 and .37, respectively). Secondary analysis using a bootstrap method for calculating P values likewise did not identify significant associations between spore counts and ambulatory-onset IFD cases. Study period dates were additionally ranked according to the average spore count over the prior 2 weeks and sorted into quintiles on that basis for analysis of association with cases. IFD rates were not significantly higher in date quintiles with higher preceding spore counts (Figure 1B).
Because the geographic range over which the NAB station measurements could reflect mold spore exposure was uncertain and because the ability to differentiate fungal infections originating in the community from those that are health care acquired is imperfect, we conducted secondary analyses in which patients living outside the DFW area and those with symptom onset >14 days after admission were included. Eight additional cases were also included that had not met the 2008 EORTC/MSG definition for IFD (due to lack of a qualifying host factor such as neutropenia) under which the study was designed but that met the revised 2019 definitions [27] from the same group (based on the addition of hematologic malignancy as a separate qualifying host factor). These analyses expanded the number of cases from 35 in the primary analysis to 54 (using updated IFD definitions and including patients outside the DFW area) and 68 (all IFD cases including those with suspected hospital onset) but also failed to identify significant associations.
Biological risk for IFD on the host side is not evenly distributed across the days a patient is under therapy for hematological malignancy or after HSCT. We were unable to generate detailed patient-level data on the time-varying factors associated with biologic risk for IFD such as neutropenia and graft-vs-host disease status for the entire cohort across the duration of each patient’s treatment. We therefore conducted a secondary analysis focusing on the interval within 100 days after diagnosis, relapse, or HSCT to examine the period when host risk for IFD would be expected to be highest. During this period, incidence of IFD was indeed enriched relative to that during the entire study period (incidence rate ratio, 2.6; 95% CI, 1.4–4.8). Within this high-risk cohort, however, there was not a significant association between ambulatory-onset IFD cases and spore counts from the NAB station (including for average counts during the 1–7-day window before symptom onset; coefficient of 0.0000 with P = .96).
Because endemic mycoses may either present with infection shortly after exposure or demonstrate reactivation after a prolonged period of latency such that only a fraction of the risk would be expected to correlate with recent environmental conditions, the data were also analyzed after exclusion of the 2 cases of histoplasmosis. The association between ambulatory-onset IFD and spore counts remained nonsignificant.
Precipitation and IFD Risk
There was no significant association between another environmental variable, daily precipitation, and ambulatory-onset IFD cases over the same time intervals preceding symptom onset.
DISCUSSION
Prior studies examining the relationship between outdoor airborne mold spore exposure and invasive fungal infections have focused specifically on invasive aspergillosis (IA) [4, 22, 23]. Our study encompassed pediatric hematologic malignancy and HSCT patients with a broader range of invasive mold infections as well as endemic mycoses and to our knowledge is the first evaluating the association between environmental airborne mold spore counts and such infections. Despite a strong seasonal pattern in spore counts, there was only a weak trend toward a seasonal pattern in IFD cases in our population, and there was no evidence of a significant association between community airborne mold spore counts and ambulatory-onset IFD. This conclusion is similar to that reported regarding IA cases in Houston, Texas (240 miles from our medical center), but differs from findings regarding IA in several more distant locales [4, 22, 23].
There are several possible reasons for this dissociation between community airborne mold spore counts and invasive fungal infections. Sampling at a single location of the NAB station, for instance, may not have been sufficiently granular to reflect risk to patients throughout the area. Our sample size of IFD cases, however, was too small to restrict analysis to those patients living closest to the measurement station. Moreover, the intent of the study was to determine whether quantitative regional-scale measurements such as provided by this station—which serves as the basis for daily qualitative aeroallergen reporting in the news media—could provide information about disease risk that would be applicable across the region. In addition, total spore counts measured by the NAB station reflect a mixture of viable and nonviable fungal elements. In cultured air samples, we found that the nonpathogenic species Cladosporium predominated but, importantly, that the mixture of organisms changed over time. This variability may detract from the utility of total spore counts as a measure of disease risk. Variation in species composition and relative abundance of pathogenic fungi may also contribute to differences among published studies from different geographic regions. Although other climatic factors may impact risk of fungal infection in different locations [4], we did not find an association with precipitation, consistent with a prior report on IA [22]. Finally, the exposure threshold necessary to initiate infection in an immunocompromised patient is unknown. If this threshold is below even the lower range of spore counts seen in our study, then fluctuations above that range may have little impact on infection risk.
Limitations of our study include that it was a retrospective, observational study at a single institution and that the findings may not be generalizable to other locations. The small sample size of proven/probable IFD cases furthermore limited the power of the study. In addition, the knowledge gap regarding the latent incubation period between spore exposure and symptom onset prevented use of a well-defined exposure interval preceding infection. We attempted to manage this uncertainty, however, by analyzing spore counts during a number of different intervals from 1 to 6 weeks before symptom onset and did not find significant associations. The degree of exposure to outdoor air among our pediatric patients was also not measured. It is uncertain how their level of exposure would compare to that of adult hematological malignancy and HSCT patients.
We did not have detailed information on host-level risk factors for IFD such as periods of neutropenia and receipt of antifungal prophylaxis. Limiting analysis to within 100 days after diagnosis, relapse, or receipt of HSCT identified a population with increased incidence of IFD but nevertheless did not yield a significant association between cases and spore counts. The lack of impact from this adjustment may reflect the fact that relevant patient-level risk factors are unlikely to correlate with environmental variables such as spore counts. From 2014 to 2018, 72% of the HSCTs performed at our institution were allogeneic procedures. These patients routinely received voriconazole prophylaxis for 6 months following transplant or until immunosuppression was discontinued. In contrast, mold-active antifungal prophylaxis was not routinely used at our institution for leukemia and lymphoma patients in the ambulatory setting, with the exception of AML patients.
This study was strengthened by the availability of a detailed daily record of spore counts extending over 5 years as well as by periodic cultured air samples. The inclusion of patients with a broader range of invasive mold infections expands the scope of this work beyond previous reports focusing on IA. Notably, a substantial number of invasive infections with dematiaceous molds were seen in our population. Our results indicate that there is not a strong correlation between total airborne spore counts and invasive fungal infection risk in pediatric patients with hematologic malignancies or HSCT in our region. These findings argue against using spore count data to guide recommendations for antifungal prophylaxis or exposure avoidance strategies despite the strong seasonal variation apparent in the reported counts.
Acknowledgments
We thank the Infection Prevention Department at Children’s Health, directed by Pat Jackson, RN, MA, CIC, FAPIC, for collection of cultured air samples.
Financial support. This work was supported by a service package grant from Children’s Health System of Texas for statistical analysis services conducted by one of the authors (R.H.). The content is solely the responsibility of the authors and does not necessarily reflect the official views of Children’s Health.
Potential conflicts of interest. The authors have no conflicts of interest to declare. All authors: no reported conflicts of interest. All authors have submitted the ICMJE Form for Disclosure of Potential Conflicts of Interest. Conflicts that the editors consider relevant to the content of the manuscript have been disclosed.
Author contributions. M.A. and M.S. conceived of the study and its design. V.A. and T.S. assisted with identification of the study cohort. M.A. collected the data. M.A., M.S., and R.H. analyzed the data. M.A. and M.S. wrote the manuscript. All authors reviewed and edited the manuscript.
Patient consent. This study was reviewed by the Institutional Review Board at UT Southwestern Medical Center, and patient consent was determined not to be required.
Comments