-
PDF
- Split View
-
Views
-
Cite
Cite
Grace Noppert, Kathleen Wragg, Chihua Li, Kate Duchowny, Lona Mody, Allison E Aiello, Linda Nyquist, Martin O’Brien, Raymond Yung, Daniel Goldstein, Herpesvirus Antibodies Are Correlated With Greater Expression of p16 in the T Cells of Humans, Open Forum Infectious Diseases, Volume 11, Issue 12, December 2024, ofae693, https://doi.org/10.1093/ofid/ofae693
- Share Icon Share
Abstract
There is an increasing awareness that aging of the immune system, or immunosenescence, is a key biological process underlying many of the hallmark diseases of aging and age-related decline broadly. While immunosenescence can be in part due to normal age-related changes in the immune system, emerging evidence posits that viral infections may be biological stressors of the immune system that accelerate the pace of immunosenescence
We used a convenience sample of 42 individuals aged 65 years and older to examine correlations between antiviral immunoglobulin G (IgG) levels for 4 human herpesviruses (cytomegalovirus [CMV], herpes simplex virus [types 1 and 2], and Epstein-Barr virus) and multiple indicators of T-cell immunosenescence.
We found that most of the sample (n = 33) was antiviral IgG positive for 2 or more of the 4 herpesvirus infections. We also examined correlations between both the total number of viruses for which an individual had antiviral IgG and each individual virus and multiple indicators of T-cell immunosenescence, particularly p16 expression. The strongest correlations were observed between the total number of viruses for which an individual had detectable antiviral IgG and p16 mean fluorescent intensity (MFI) among CD27-CD28-CD57+ CD4+ cells (r = 0.60; P < .001) and between anti-CMV IgG and p16 MFI of CD27-CD57+ CD4+ cells (r = 0.59; P < .001).
Broadly, our findings offer compelling preliminary evidence for future investigations to incorporate multiple indicators of persistent viral infections and a more comprehensive set of markers of T-cell immunosenescence in population-based studies of aging.
Aging of the immune system, or immunosenescence, is emerging as a key process that may be involved in the etiology of many chronic conditions, including aging-related disease and decline. Studies in humans have described the changes that occur during immunosenescence including an increase in memory T cells and a decline in naïve T cells, a shift in the distribution of the T-cell compartment favoring CD8+ T cells over CD4+ T cells, and a functional shift towards a pro-inflammatory phenotype often referred to as “inflammaging.” Previous studies have posited that continual antigenic stimulation primarily by cytomegalovirus (CMV) may drive the expansion of memory and effector T-cell subsets, contributing to an increase in the number of pathogen-specific, highly differentiated T cells. However, CMV is only 1 virus in the herpesvirus family, and new evidence suggests that other herpesviruses may also be involved in the pathophysiology of several chronic diseases. For example, a 2022 study found a causal link between Epstein-Barr virus (EBV) and incident multiple sclerosis [1]. Despite the growing emphasis on understanding immunosenescence and the role of persistent herpesvirus infections in this process, critical questions remain including adjudicating between past and active herpesvirus infections, the role of herpesvirus coinfections, and the specific indicators of T-cell immunosenescence associated with herpesvirus infections.
Added Value of This Study
Here, we found that 33 of the 42 individuals in our study population of healthy older adults were positive for 2 or more of the 4 herpesvirus infections examined (CMV, herpes simplex types 1 and 2 [HSV-1 and -2], EBV). Using both antiviral immunoglobulin (Ig) G and M, we were able to determine that these infections were predominantly past infections that were not currently active, which adds to the evidence that these infections are likely exerting their influence over long periods of time.
We report significant correlations between the total number of viral infections for an individual who is IgG positive and multiple indicators of T-cell immunosenescence, particularly p16 expression in both CD4+ and CD8+ T cells. We also observed these correlations examining anti-CMV IgG alone. These findings suggest that p16 expression may be an especially salient measure of T-cell aging and key to understanding the impacts of persistent viral infections. However, contrary to our expectations, we did not find consistent correlations between anti-EBV, anti-HSV-1, or anti-HSV-2 IgG and T-cell immunosenescence. With these findings, we continue to expand our knowledge of the ways in which herpesvirus infections may be involved in immunosenescence; we may need to consider other immune parameters to understand the impacts of EBV, HSV-1, and HSV-2.
Implications of All the Available Evidence
First, our findings confirm that older adults are likely living with persistent herpesvirus infections for a long period of time. While this has been the predominant hypothesis in life course epidemiologic investigations of immunosenescence, few studies have had both IgG and IgM to adjudicate current and past infections. Moreover, these findings add to this literature by documenting how individuals are living not only with CMV but multiple other herpesvirus infections. Our findings add to the emerging body of work suggesting that herpesvirus infections may be associated with immunosenescence in older adults and extend this by identifying p16 as a particularly salient indicator of this association. Broadly, our findings offer compelling evidence for population health studies to incorporate multiple indicators of persistent viral infections and a more comprehensive set of markers of T-cell immunosenescence in studies of aging. This has implications for potential interventions that could slow the process of immunosenescence.
There is an increasing awareness that aging of the immune system is a key biological process underlying many of the hallmark diseases of aging and age-related decline broadly [2–7]. As individuals age, there is a marked decline in several aspects of the immune system [8]. Specifically, it is hypothesized that alterations to the immune system observed with older age include a shift in the balance of naïve and memory leukocytes, reduced phagocytic capability of neutrophils, monocytes, and macrophages, a functional shift toward a pro-inflammatory phenotype in monocytes, and decreased cytotoxicity of natural killer cells [9]. Antigenic stimulation across the life course drives expansion of memory and effector T-cell subsets, contributing to an increase in the number of pathogen-specific, highly differentiated T cells [10]. Upon antigen stimulation, the highly differentiated T cells generate a greater inflammatory response than less differentiated cells, further contributing to a process referred to as “inflammaging” [11]. Taken together, these processes comprise an immune phenotype in older age that is characterized by several alterations to the T-cell compartment including an inverted ratio of CD4:CD8 T cells, a decrease in naïve T cells, and an accumulation of memory T cells—particularly late-stage differentiated CD8+ T cells with limited function as well as elevated levels of systemic inflammation [12].
While immunosenescence can be in part due to normal age-related changes in the immune system, emerging evidence posits that viral infections may be biological stressors of the immune system that accelerate the pace of immunosenescence [13–18]. Persistent viral infections, such as those from the human herpesvirus family (eg, CMV, EBV, HSV-1 and -2), are of particular interest because they are highly prevalent in the population, induce lifelong infections with periods of reactivation and latency, and, as a result, are thought to be key drivers of immunosenescence [19, 20]. Moreover, a growing number of studies have linked these persistent infections with the development of a number of chronic conditions in late life such as dementia, multiple sclerosis, cancers, and cardiovascular diseases [1, 21–23].
Yet, despite this compelling evidence, there remain critical unanswered questions as to the mechanisms by which viral infections may drive immunosenescence, adjudication of active vs past, chronic infections, and the role of multiple, persistent viral infections. Moreover, the role of viral coinfections and differential immune control of viral infections, as reflected by Ig antibody levels, remains poorly understood. While some studies have focused on both CMV and EBV, fewer studies have examined HSV-1 and -2 and to what extent these viruses may co-occur. Even fewer studies have examined all 4 of these viruses in a single study, thus precluding the ability to look at the interplay between these prevalent viruses that likely have major implications for human health. We conducted a small pilot study to examine (1) the relationship between 4 of the most prevalent herpesviruses and to what extent they may have unique interactions with the immune system and (2) the correlation between these 4 viruses and markers of T-cell immunosenescence. While this is a pilot study, we believe the findings from this study can be used to inform larger population cohort studies.
METHODS
Study Population
We employed a convenience sample design given the preliminary nature of the study. We recruited individuals who were patients at the University of Michigan health system. Individuals were invited to participate in the study through a University of Michigan registry of ongoing scientific studies. Patients were able to sign up to be considered for the study. Once patients signed up, they were contacted by members of our study team to confirm eligibility, consent to be in the study, and enrollment.
To be included in the study, individuals had to be at least 65 years of age at the time of enrollment and a patient in the University of Michigan health system. Individuals were excluded from the study if they were currently pregnant, taking immunosuppressive medications, or had a current or past diagnosis of malignancy and/or autoimmune disorder. We made an exception for patients who had a history of nonmelanoma skin cancer. A member of our study team determined eligibility for each patient individually. Once patients were deemed eligible, they were provided with information regarding the study, the potential risks, and the study procedures. Patients were provided the option to sign an informed consent. Once consented, patients were invited to enroll in the study. Upon enrollment, study participants participated in a blood draw. Medical record extraction of relevant health and demographic information was also included as part of the informed consent. Study participants went to 1 of 2 Michigan laboratory locations to contribute their blood sample. The samples were then processed and frozen. At the completion of the blood draw, participants received $10 as compensation for participation.
The study was approved by the University of Michigan Institutional Review Board.
Sample Processing
Samples were collected in two 8-mL sodium heparin tubes and one 8-mL CPT tube. For the plasma collection, the sodium heparin tubes were inverted 8–10 times. The plasma was separated by centrifuging at 1000–2000 times gravity (×g) for 10 minutes in a 4°C refrigerated centrifuge. The samples were then separated into1-mL aliquots and placed in a −80°C freezer.
For the peripheral blood mononuclear cell (PBMC) isolation, the tubes were inverted 8–10 times. The tubes were then centrifuged at 1700× relative centrifugal force (RCF) for 20 minutes at room temperature. After centrifugation, the mononuclear cells were transferred to a 15-mL conical tube. Phosphate-buffered saline (PBS) was added to wash the cells, and the tube was inverted 3–5 times. The tubes were then centrifuged at 400×g for 15 minutes, at which point the supernatant was discarded. PBS was again mixed with the cell pellet, the tubes were centrifuged at 400×g for 10 minutes, and the supernatant was discarded. The cells were then counted with the hemocytometer. Cell viability was determined with Trypan Blue by mixing 10 μL of the PBMCs with the Trypan Blue solution 1:1 in a microtiter plate. Cells were loaded in the hemocytometer and counted after 30 seconds. Finally, the cells were resuspended in 10% dimethyl sulfoxide (DMSO) in fetal bovine serum (FBS) to a concentration of 5 million in 1 mL. The cells were then placed in aliquots of 5 million cells to 1 mL of solution. The aliquots were stored in a −80°C freezer overnight and then placed in liquid nitrogen.
Assessment of Plasma IgG and IgM Levels
We quantified antiviral IgG and IgM antibody levels for 4 human herpesviruses including CMV, HSV-1 and -2, and EBV. Plasma IgG and IgM levels to HSV-1 (ab108737 & ab108738; Abcam), HSV-2 (ab108739 & ab108740; Abcam), CMV (ab108724 & ab108725; Abcam), and EBV (ab108730 & ab108732; Abcam) were assessed via enzyme-linked immunosorbent assay (ELISA) according to the manufacturer's instructions. Samples were assayed in duplicate, with seropositivity determined relative to a cutoff control. Samples with a mean absorbance value 10% greater than the cutoff control were considered seropositive for the viral antigen of interest. Seropositivity for each infection was determined based on antiviral IgG.
We used antiviral IgM titers to adjudicate between active and past herpesvirus infections. IgM levels increase following a primary infection, reactivation of a latent infection, or reinfection [24]. Thus, the presence of IgG without detectable IgM may signify a chronic infection that is not currently active.
Flow Cytometry Analysis of T-cell Phenotypes
Cryopreserved PBMCs were thawed in RPMI media (Gibco) supplemented with 10% FBS (Sigma) and 5% penicillin-streptomycin (Gibco). Cells were washed in PBS and stained with LIVE/DEAD aqua viability dye (ThermoFisher Scientific) at room temperature for 5 minutes, before addition of the surface staining antibody cocktail for 30 minutes at 4°C. Surface stain antibodies included CD3 APC/Fire 750 (SK7), CD4 BV605 (RPA-T4), CD8 BV650 (RPA-T8), CD27 FITC (M-T271), CD28 APC (CD28.2), and CD57 BV421 (QA17A04; all BioLegend). Following washing with PBS + 2% FBS, cells were fixed and permeabilized with Transcription Factor Fix/Perm Buffer (BD Biosciences) and stained intracellularly with p16 PE (EPR1473; Abcam) for 40 minutes at room temperature. Cells were washed twice, resuspended in PBS + 2% FBS, and acquired on a Ze5 Analyzer (BioRad). Data were analyzed using FlowJo, version 10 (BD Biosciences).
Lymphocytes were identified based on forward scatter (FSC) and side scatter (SSC), singlets by FSC-A vs FSC-H, and live T cells by CD3-positive, viability dye–negative events. CD4 and CD8 T cells were further identified as CD4+ CD8- and CD4- CD8+, respectively. For assessment of phenotypic markers, gates were set using fluorescence minus one (FMO) controls.
Gates for all phenotypic markers, including p16, were set based on FMO controls. For additional details, see Supplementary Figure 1.
Statistical Analyses
Descriptive statistics were used to characterize the study population. Correlation analyses were conducted with and without adjusting for chronological age to assess the relationship between antiviral IgG and advanced differentiation of T cells. Spearman's rank correlation analysis was used with the total count of the 4 viral infections mentioned above (ranging from 0 to 4), and Pearson correlation analysis was used with antiviral IgG antibody levels corresponding to each of the 4 viral types. Heatmaps were created to summarize the correlation coefficients between viral infections and advanced differentiation of T cells. All analyses were executed using R, version 4.2.
Role of the Funding Source
The funders had no influence or role in the design or conduct of this study. They were not involved in data collection, statistical analysis, preparation of the manuscript, or the decision to submit the manuscript.
RESULTS
Study Sample Description
The sample included 42 individuals with a median age (range) of 72 (66–89) years (Table 1). The majority of the sample was female (n = 27), and it was predominantly non-Hispanic White (n = 40). Over half of the sample was antiviral IgG seropositive for CMV (n = 23), EBV (n = 36), and HSV-1 (n = 22). Eight individuals in the sample were antiviral IgG seropositive for HSV-2.
Demographic Characteristics of the Study Sample and Distribution for Viral Indicators (n = 42)
Demographics . | . | . | . |
---|---|---|---|
n = 42 . | . | . | . |
Age, y | Median (range) | 72 (66–89) | |
Gender | No. (%) | Women | 27 (64) |
Men | 15 (36) | ||
Race/ethnicity | No. (%) | Non-Hispanic White | 40 (95) |
Non-Hispanic Black | 2 (5) | ||
Viral infections | |||
CMV IgG | No. (%) | Positive | 23 (55) |
Negative | 19 (45) | ||
EBV IgG | No. (%) | Positive | 36 (86) |
Negative | 6 (14) | ||
HSV-1 IgG | No. (%) | Positive | 22 (52) |
Negative | 20 (48) | ||
HSV-2 IgG | No. (%) | Positive | 8 (19) |
Negative | 34 (81) |
Demographics . | . | . | . |
---|---|---|---|
n = 42 . | . | . | . |
Age, y | Median (range) | 72 (66–89) | |
Gender | No. (%) | Women | 27 (64) |
Men | 15 (36) | ||
Race/ethnicity | No. (%) | Non-Hispanic White | 40 (95) |
Non-Hispanic Black | 2 (5) | ||
Viral infections | |||
CMV IgG | No. (%) | Positive | 23 (55) |
Negative | 19 (45) | ||
EBV IgG | No. (%) | Positive | 36 (86) |
Negative | 6 (14) | ||
HSV-1 IgG | No. (%) | Positive | 22 (52) |
Negative | 20 (48) | ||
HSV-2 IgG | No. (%) | Positive | 8 (19) |
Negative | 34 (81) |
Abbreviations: CMV, cytomegalovirus; EBV, Epstein-Barr virus; HSV-1/2, herpes simplex virus type 1/2; IgG, immunoglobulin G.
Demographic Characteristics of the Study Sample and Distribution for Viral Indicators (n = 42)
Demographics . | . | . | . |
---|---|---|---|
n = 42 . | . | . | . |
Age, y | Median (range) | 72 (66–89) | |
Gender | No. (%) | Women | 27 (64) |
Men | 15 (36) | ||
Race/ethnicity | No. (%) | Non-Hispanic White | 40 (95) |
Non-Hispanic Black | 2 (5) | ||
Viral infections | |||
CMV IgG | No. (%) | Positive | 23 (55) |
Negative | 19 (45) | ||
EBV IgG | No. (%) | Positive | 36 (86) |
Negative | 6 (14) | ||
HSV-1 IgG | No. (%) | Positive | 22 (52) |
Negative | 20 (48) | ||
HSV-2 IgG | No. (%) | Positive | 8 (19) |
Negative | 34 (81) |
Demographics . | . | . | . |
---|---|---|---|
n = 42 . | . | . | . |
Age, y | Median (range) | 72 (66–89) | |
Gender | No. (%) | Women | 27 (64) |
Men | 15 (36) | ||
Race/ethnicity | No. (%) | Non-Hispanic White | 40 (95) |
Non-Hispanic Black | 2 (5) | ||
Viral infections | |||
CMV IgG | No. (%) | Positive | 23 (55) |
Negative | 19 (45) | ||
EBV IgG | No. (%) | Positive | 36 (86) |
Negative | 6 (14) | ||
HSV-1 IgG | No. (%) | Positive | 22 (52) |
Negative | 20 (48) | ||
HSV-2 IgG | No. (%) | Positive | 8 (19) |
Negative | 34 (81) |
Abbreviations: CMV, cytomegalovirus; EBV, Epstein-Barr virus; HSV-1/2, herpes simplex virus type 1/2; IgG, immunoglobulin G.
Most of the sample (n = 40) was antiviral IgG positive for 1 or more of the viral infections (Supplementary Figure 2A). We also examined the most common combinations of coinfections and found that infection with CMV, HSV-1, and EBV was the most common coinfection, occurring in 9 of the individuals (Table 2).
CMV . | HSV-1 . | HSV-2 . | EBV . | No. . | % . |
---|---|---|---|---|---|
0 | 0 | 0 | 0 | 2 | 5 |
0 | 0 | 0 | 1 | 7 | 17 |
0 | 0 | 1 | 1 | 2 | 5 |
0 | 1 | 0 | 0 | 2 | 5 |
0 | 1 | 0 | 1 | 6 | 14 |
1 | 0 | 0 | 1 | 6 | 14 |
1 | 0 | 1 | 1 | 3 | 7 |
1 | 1 | 0 | 0 | 2 | 5 |
1 | 1 | 0 | 1 | 9 | 21 |
1 | 1 | 1 | 1 | 3 | 7 |
CMV . | HSV-1 . | HSV-2 . | EBV . | No. . | % . |
---|---|---|---|---|---|
0 | 0 | 0 | 0 | 2 | 5 |
0 | 0 | 0 | 1 | 7 | 17 |
0 | 0 | 1 | 1 | 2 | 5 |
0 | 1 | 0 | 0 | 2 | 5 |
0 | 1 | 0 | 1 | 6 | 14 |
1 | 0 | 0 | 1 | 6 | 14 |
1 | 0 | 1 | 1 | 3 | 7 |
1 | 1 | 0 | 0 | 2 | 5 |
1 | 1 | 0 | 1 | 9 | 21 |
1 | 1 | 1 | 1 | 3 | 7 |
0 indicates IgG seronegative; 1 indicates IgG seropositive. n refers to the full study population; No. refers to the number of participants in subpopulation of the full sample.
Abbreviations: CMV, cytomegalovirus; EBV, Epstein-Barr virus; HSV-1/2, herpes simplex virus type 1/2; IgG, immunoglobulin G.
CMV . | HSV-1 . | HSV-2 . | EBV . | No. . | % . |
---|---|---|---|---|---|
0 | 0 | 0 | 0 | 2 | 5 |
0 | 0 | 0 | 1 | 7 | 17 |
0 | 0 | 1 | 1 | 2 | 5 |
0 | 1 | 0 | 0 | 2 | 5 |
0 | 1 | 0 | 1 | 6 | 14 |
1 | 0 | 0 | 1 | 6 | 14 |
1 | 0 | 1 | 1 | 3 | 7 |
1 | 1 | 0 | 0 | 2 | 5 |
1 | 1 | 0 | 1 | 9 | 21 |
1 | 1 | 1 | 1 | 3 | 7 |
CMV . | HSV-1 . | HSV-2 . | EBV . | No. . | % . |
---|---|---|---|---|---|
0 | 0 | 0 | 0 | 2 | 5 |
0 | 0 | 0 | 1 | 7 | 17 |
0 | 0 | 1 | 1 | 2 | 5 |
0 | 1 | 0 | 0 | 2 | 5 |
0 | 1 | 0 | 1 | 6 | 14 |
1 | 0 | 0 | 1 | 6 | 14 |
1 | 0 | 1 | 1 | 3 | 7 |
1 | 1 | 0 | 0 | 2 | 5 |
1 | 1 | 0 | 1 | 9 | 21 |
1 | 1 | 1 | 1 | 3 | 7 |
0 indicates IgG seronegative; 1 indicates IgG seropositive. n refers to the full study population; No. refers to the number of participants in subpopulation of the full sample.
Abbreviations: CMV, cytomegalovirus; EBV, Epstein-Barr virus; HSV-1/2, herpes simplex virus type 1/2; IgG, immunoglobulin G.
We only found detectable antiviral IgM for CMV and EBV (Supplementary Figure 2B). Twenty-three individuals were antiviral IgG positive for CMV, 5 of whom had detectable IgM. Of the 36 individuals with detectable anti-EBV IgG, only 1 had detectable anti-EBV IgM.
Correlations Between the Number of Viruses for Which an Individual Had Detectable Antiviral IgG and T-cell Expression of Advanced Differentiation
We assessed CD4+ and CD8+ T-cell phenotypes for markers of advanced cellular differentiation, focusing specifically on expression of CD27, CD28, CD57, and p16. We first estimated correlations among CD8+ cells, adjusting for continuous chronological age. We found statistically significant, albeit weak, correlations between total frequency of viral positivity (ie, the number of viruses for which an individual had detectable antiviral IgG) and the frequency of p16-expressing cells (Figure 1A).
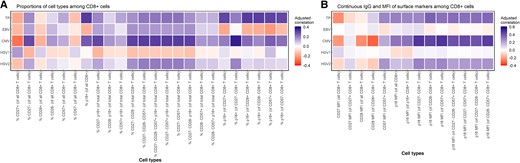
Correlation heat map of total number of viruses for which an individual had detectable antiviral IgG with proportions of cell types and continuous IgG and MFI of surface markers among CD8+ cells. A, Correlations of proportions of cell types among CD8+ cells. B, Correlations between IgG and MFI of surface markers among CD8+ cells. Correlations were adjusted for continuous chronological age. Spearman's rank correlation analysis was used with TP (ranging from 0 to 4), and Pearson correlation analysis was used with antiviral IgG antibody levels corresponding to each of the 4 viral types. Abbreviations: CMV, cytomegalovirus; EBV, Epstein-Barr virus; HSV-1/2, herpes simplex virus type 1/2; IgG, immunoglobulin G; MFI, mean fluorescent intensity; TP, the number of viruses for which an individual had detectable antiviral IgG.
We also examined correlations of p16 mean fluorescent intensity (MFI) among subpopulations of CD8+ T cells expressing various markers of advanced cellular differentiation. Among CD8+ cells, adjusting for chronological age, we found multiple statistically significant correlations with the total frequency of antiviral IgG and the MFI of p16 expression among multiple cells (Figure 1B). The strongest correlation was observed between total frequency of antiviral IgG and p16 MFI among CD28- CD8+ cells (r = 0.37; P = .02).
Similarly, among CD4+ cells, we only found statistically significant age-adjusted correlations between the number of viruses for which an individual had detectable IgG and the expression of p16 (Figure 2A). Results showed a statistically significant, weak correlation between total frequency of viral positivity and p16 expression among multiple cell types.
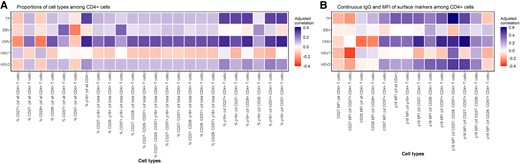
Correlation heat map of total number of viruses for which an individual had detectable antiviral IgG with proportions of cell types and continuous IgG and MFI of surface markers among CD4+ cells. A, Correlations of proportions of cell types among CD4+ cells. B, Correlations between IgG and MFI of surface markers among CD4+ cells. Correlations were adjusted for continuous chronological age. Spearman's rank correlation analysis was used with TP (ranging from 0 to 4), and Pearson correlation analysis was used with antiviral IgG antibody levels corresponding to each of the 4 viral types. Abbreviations: CMV, cytomegalovirus; EBV, Epstein-Barr virus; HSV-1/2, herpes simplex virus type 1/2; IgG, immunoglobulin G; MFI, mean fluorescent intensity; TP, the number of viruses for which an individual had detectable IgG.
In both CD8+ and CD4+ cells, we observed that p16 positivity was correlated with the total number of viruses for which an individual had detectable antiviral IgG regardless of the expression of other markers of advanced cellular differentiation (Figures 1 and 2).
Among CD4+ cells, we also found multiple statistically significant correlations between the number of viruses for which an individual had detectable IgG and the MFI of p16 expression (Figure 2B). The strongest correlation was observed between the total number of viruses for which an individual had detectable antiviral IgG and p16 MFI among CD27- CD28-CD57+ CD4+ cells (r = 0.60; P < .001). We also observed a statistically significant, age-adjusted correlation between total frequency of viral positivity and p16 MFI among CD27-CD57+ CD4+ cells (r = 0.45; P = .01).
Correlation coefficients and associated P values are reported in Supplementary Tables 1–4. Heat maps of the unadjusted correlations are reported in Supplementary Figures 3–4.
Correlations Between Antiviral IgG Antibody Titers for Individual Viral Infections and T-cell Markers of Advanced Differentiation
We also estimated whether antiviral IgG for each of the 4 viral infections separately was correlated with both singular and multiple markers of advanced T-cell differentiation in the CD4+ and CD8+ compartments.
Among those individuals with positive antiviral IgG for EBV, we did not find any statistically significant, age-adjusted correlations in either the CD8+ or CD4+ compartment (Figures 1 and 2). We also did not observe any statistically significant, age-adjusted correlations with the MFI of any of the surface markers among either CD4+ or CD8+ cells.
We found statistically significant correlations between antiviral IgG antibody titers for CMV and markers of cellular differentiation among both CD8+ and CD4+ T cells. In the CD8+ compartment, we observed statistically significant, age-adjusted correlations between anti-CMV IgG antibody titers and both singular and multiple markers of advanced cellular differentiation (Figure 1A). The strongest correlations were observed between anti-CMV IgG and the proportion of p16+ cells of CD27- CD8+ cells (r = 0.36; P = .02), proportion of CD27- CD28- CD57+ p16+ of total CD8+ cells (r = 0.31; P = .05), proportion of CD27- CD57+ p16+ of total CD8+ cells (r = 31; P = .04), and proportion of CD27- CD57+ of total CD8+ cells (r = 31; P = .04).
We also observed multiple statistically significant age-adjusted correlations with anti-CMV IgG and the MFI of multiple surface markers in CD8+ cells. The strongest correlations were observed between CMV IgG and p16 MFI of CD27- CD8+ cells (r = 0.48; P < .001) and p16 MFI of CD57+ CD8+ cells (r = 0.49; P < .001) (Figure 1B).
Among CD4+ cells, we also observed statistically significant, age-adjusted correlations between anti-CMV IgG antibody titers and markers of advanced cellular differentiation (Figure 2A). The strongest correlation was between anti-CMV IgG and the proportion of p16+ cells among CD57- CD4+ cells (r = 0.35; P = .02). Consistent with CD8+ cells, we found the strongest correlations with the MFI of multiple surface markers in CD4+ cells. For example, there was a statistically significant age-adjusted correlation between anti-CMV IgG and p16 MFI of CD27- CD57+ CD4+ cells (r = 0.59; P < .001) (Figure 2B).
We did not observe any statistically significant associations between antiviral IgG antibody titers to HSV-1 or HSV-2 and markers of advanced cellular differentiation among both CD4+ and CD8+ T cells (Figures 1 and 2). We did, however, observe a statistically significant, negative age-adjusted correlation between anti-HSV-1 IgG and CD27 MFI among CD27+ CD4+ cells (r = –0.36; P = .02) (Figure 2B). We also observed multiple modest correlations between anti-HSV-2 IgG and p16 MFI among CD4+ cells. However, only 8 individuals were IgG positive for anti-HSV-2, and thus interpretation of correlations should be made with caution.
Correlation coefficients and associated P values are reported in Supplementary Tables 1–4. Heat maps of the unadjusted correlations are reported in Supplementary Figures 2 and 3.
DISCUSSION
A growing body of research is examining persistent viral infections as a potential driver of immunosenescence over the life course. Yet to date, the few studies that have been able to examine associations between persistent viral infections and T-cell immunosenescence have been limited to singular viral infections and had data on a limited range of T-cell phenotypes [2, 5, 25–27]. In this study, we correlated antiviral IgG for 4 herpesvirus infections with a diverse array of T-cell phenotypes consistent with an aged immune system in a sample of healthy older adults. There were 2 major findings from this study. First, we found that the majority of the significant correlations we observed between the viral infections and T-cell phenotypes involved p16 expression. This finding suggests that p16 expression may be an especially salient measure of T-cell aging. In contexts where there may be limited resources to test for multiple markers of T-cell aging, measuring p16 expression may be an efficient method to understand the impact of viral infections and T-cell aging. Second, in analyses of correlations between T-cell aging and individual viral infections, most of the correlations we observed involved CMV infection. This is broadly consistent with the human health literature, which has long focused on infection with CMV as an aging-related indicator of immune fitness [10, 28]. However, the lack of consistent findings with EBV, HSV-1, and HSV-2 necessitates further study and requires an expansive set of immune indicators.
As expected, we found that chronic herpesvirus infections were generally highly prevalent in our study population, particularly CMV, EBV, and HSV-1. However, the proportions of those seropositive for anti-CMV IgG (55%) and anti-HSV-1 IgG (52%) were notably lower than in other studies in older populations in the United States, which have reported a prevalence of CMV of 65% and 79% for HSV-1 [29, 30]. Additionally, the majority of the sample was antiviral IgG positive for 2 or more viral infections. We were able to adjudicate between active and past infections by measuring both IgG and IgM. In line with our expectation, the majority of the viral infections we detected were past infections that were not currently active, as indicated by the presence of antiviral IgG and a lack of detectable antiviral IgM. Also, consistent with the literature, we observed the strongest correlations between anti-CMV IgG and multiple indicators of T-cell immunosenescence [15, 31–33]. As noted above, the majority of the significant correlations we observed involved the expression of p16. While multiple studies have highlighted p16 as an important biomarker of cellular aging [34–43], few studies have investigated p16 expression in the context of multiple viral infections. These findings provide foundational evidence supporting increased efforts for population-based studies to collect robust immune data including data on persistent viral infections and indicators of T-cell immunosenescence, specifically p16.
There were multiple limitations to this study that should be noted. First, this was a convenience sample of healthy adults enrolled in the University of Michigan Health System. Thus, it is not representative of the larger population of older adults. Second, the sample was comprised of 42 individuals, which limits the statistical inferences that can be made from these data. Larger cohort studies need to integrate a more comprehensive set of immune measures in their data collection to allow these findings to be replicated and extended.
With regards to the specific immune markers we investigated, the T cells we examined were not antigen-specific T cells. Rather, we examined changes to the entire T-cell repertoire. To further understand the relationship between herpesvirus infections and T-cell aging, future studies should examine antigen-specific T cells, with particular attention to expression of p16. Additionally, as few studies have measured IgM, future studies are needed that can replicate our findings. Finally, while we investigated a more robust set of measures for both the viral infections and T-cell immunosenescence than many previous studies have been able to do, these measures still cannot not fully capture the complexity and dynamism of the human immune system.
CONCLUSIONS
There is growing evidence demonstrating the importance of persistent viral infections in the etiology of aging-related disease development. One major mechanism through which persistent viral infections may influence aging-related disease development is through driving immunosenescence over the life course. Yet few population-based studies have collected measures of persistent viral infections, and even fewer population-based studies have collected data on aging in the immune system beyond singular measures of inflammation. This study provides compelling initial evidence that herpesviruses, and herpesvirus coinfections, are highly prevalent in older populations and are correlated with the composition of the T-cell pool and the extent to which T cells express markers of advanced differentiation. Future population-based studies should continue to expand the biological measures included in their studies as they hold critical clues to understanding how the immune system ages, as well as implications for the development of a vast array of aging-related diseases.
Supplementary Data
Supplementary materials are available at Open Forum Infectious Diseases online. Consisting of data provided by the authors to benefit the reader, the posted materials are not copyedited and are the sole responsibility of the authors, so questions or comments should be addressed to the corresponding author.
Acknowledgments
We are very grateful to the Claude D. Pepper Older Americans Independence Center at the University of Michigan, and especially Linda Nyquist for providing to support to carry out this project. This would not have been possible without the financial and technical support they provided. We are also grateful to all the study participants who volunteered their time and contributed their biological samples to make this study possible.
We would like to dedicate this work in memory of Dr. Daniel Goldstein, whose expertise, mentorship, and commitment to this project were invaluable. He played a critical role in shaping our work, and his insights will be profoundly missed. We are grateful for his contributions and the impact he has had on this research and the field of immunology.
Author contributions. G.N., D.G., and R.Y. designed the study. G.N., L.N., and M.O.B. were involved in collecting and processing the samples. L.N. provided technical support throughout the study design process, participant recruitment, and sample processing. K.W. performed the laboratory experiments, performed statistical analyses, and assisted in drafting the manuscript. C.L. performed statistical analyses and assisted in drafting the manuscript. K.D., L.M., A.A., and R.Y. provided feedback on the study design, statistical analyses, and draft manuscript. G.N. oversaw the project, provided support for the statistical analyses, and drafted and revised the manuscript. All authors read and approved the final version of the manuscript.
Financial support. This work was partially funded by the Claude D. Pepper Older Americans Independence Center at the University of Michigan (AG 024824) and the US National Institute on Aging (NIA) Biomarker Network at the University of Michigan (AG 054365). Additionally, G.A.N. received support from the NIA (AG 62749), and G.A.N./A.E.A. received support from the NIA (AG 075719).
Data sharing. The data presented in this study are not publicly available due to human subjects restrictions because they relate to information that could compromise research participant privacy or consent.
References
Author notes
Raymond Yung and Daniel Goldstein co-senior authors
Potential conflicts of interest. The authors have no conflicts of interest to disclose.
Comments