-
PDF
- Split View
-
Views
-
Cite
Cite
Joshua A Hill, Sarah Y Park, Kiran Gajurel, Randy Taplitz, A Systematic Literature Review to Identify Diagnostic Gaps in Managing Immunocompromised Patients With Cancer and Suspected Infection, Open Forum Infectious Diseases, Volume 11, Issue 1, January 2024, ofad616, https://doi.org/10.1093/ofid/ofad616
- Share Icon Share
Abstract
Patients with cancer are increasingly vulnerable to infections, which may be more severe than in the general population. Improvements in rapid and timely diagnosis to optimize management are needed. We conducted a systematic literature review to determine the unmet need in diagnosing acute infections in immunocompromised patients with cancer and identified 50 eligible studies from 5188 records between 1 January 2012 and 23 June 2022. There was considerable heterogeneity in study designs and parameters, laboratory methods and definitions, and assessed outcomes, with limited evaluation of diagnostic impact on clinical outcomes. Culture remains the primary diagnostic strategy. Fewer studies employing molecular technologies exist, but emerging literature suggests that pathogen-agnostic molecular tests may add to the diagnostic armamentarium. Well-designed clinical studies using standardized methodologies are needed to better evaluate performance characteristics and clinical and economic impacts of emerging diagnostic techniques to improve patient outcomes.
As cancer therapy has advanced, patients have become increasingly vulnerable to life-threatening infections. Higher mortality rates, longer hospitalization, and higher healthcare costs in patients with cancer can be attributable to their high infection risk [1, 2]. Prompt and accurate identification of infections is essential to improving outcomes [3] and reducing costs in patients with cancer [4].
Standard culture-based diagnostic approaches are limited in immunocompromised patients by relatively poor yield for pathogen identification [5]. Extensive diagnostic workups employing multiple tests are often the norm. However, some diagnostic tests such as those relying on antibody responses may not be reliable in immunocompromised hosts unable to mount sufficient antibodies. The performance of others such as biomarkers (eg, galactomannan [GM] or (1,3)-β-d-glucan [BDG]) may be impacted by sample type or host factors such as antimicrobial exposure or have issues with cross-reaction [6].
Molecular diagnostic methods have undergone explosive growth in the last decade. These methods can rapidly detect microbial nucleic acids in a specimen without many of the challenges associated with cultivating an isolate such as nonviability from empiric antibiotic treatment or fastidious growth characteristics. For example, quantitative cytomegalovirus (CMV) polymerase chain reaction (PCR) assays have overtaken the previously traditional mainstays of viral culture and then antigen detection to facilitate detecting and monitoring CMV reactivation and disease [7–9]. From sensitive, pathogen-specific molecular methods to now multiplex PCR tests (eg, gastrointestinal, respiratory, or meningitis-encephalitis panels), these more advanced diagnostic tests facilitate concurrently assessing the presence of a wide array of microbes with high sensitivity and specificity [10–12]. Such tests have decreased empiric therapies dependent on clinical findings or microbial viability for detection and have facilitated optimizing antimicrobial therapy [10, 11]. Notably, multiplex and/or broad-range PCRs require predefined targets, necessitating consideration for differential diagnoses and appropriate panel selection [13, 14]. Additionally, the frequent need for invasive (eg, bronchoalveolar lavage [BAL]) samples is a common limitation for many diagnostic methods [5], particularly in immunocompromised patients. The pathogen-agnostic molecular technology of metagenomic sequencing, the next potential leap in molecular diagnostic techniques, offers the possibility of additional improvement in infection diagnosis, especially for immunocompromised patients with cancer given their increased risk for diagnostically challenging and potentially co-occurring opportunistic infections. In the appropriate setting of high clinical pretest probability for infection, this technology may provide noninvasive sampling, improved diagnostic sensitivity, and timely therapeutic targeting.
We conducted a systematic literature review (SLR) to determine the unmet need in broadly approaching the diagnosis of acute infections in immunocompromised patients with cancer. We evaluated the diagnostic tools and approaches currently used to identify the potential value of a pathogen-agnostic technology such as metagenomic sequencing in comparison with these tests.
METHODS
Systematic Literature Review
The SLR was designed and conducted with support from IQVIA (https://www.iqvia.com/). A comprehensive search strategy across multiple bibliographic databases and other public sources from 1 January 2012 through 23 June 2022 was employed to identify data related to diagnosing acute infections in immunocompromised patients with cancer (Supplementary Methods, Supplementary Tables 1 and 2). Databases were searched on 21 March 2022 for studies published in the English language in the past 10 years (from 1 January 2012) and without geographic limits. Supplementary searches (Supplementary Table 3) were conducted on 23 June 2022 as outlined in the Supplementary Materials. Study eligibility criteria included those reporting results in adult patients with cancer and suspected infection in whom diagnostic interventions such as conventional diagnostic tests (approved standard diagnostic tests, eg, culture, serology, PCR, antigen testing) and metagenomic sequencing–based diagnostic tests were evaluated. Given the objective to consider the broad infectious disease diagnostic landscape, pathogen-specific studies were excluded.
Synthesis of Results
The approach and methods of the SLR followed the guidelines set forth by the Cochrane Handbook for Systematic Reviews of Interventions [15] and the Preferred Reporting Items for Systematic Reviews and Meta-Analyses (PRISMA) 2020 statement [16]. Results were categorized and presented according to cancer type (solid tumor, hematological cancer, and hematopoietic cell transplant [HCT]) and outcomes (test characteristics—including test accuracy or performance and diagnostic findings or pathogen yield, clinical course, health outcomes, and healthcare resource utilization [HCRU] and cost). Studies were grouped by region to account for differences in the types of approved diagnostics, test accessibility, clinical practice, and infection epidemiology.
RESULTS
Study Selection
The database searches identified 5188 records. Following de-duplication, classification based on the original Population, Intervention, Comparators, Outcomes, Timing, and Study (PICOTS) design (Supplementary Table 4) as well as additional exclusion criteria (Supplementary Table 5) and full text review, 41 records met the eligibility criteria (Figure 1). An additional 10 records were included from supplementary searches (Supplementary Table 3). Overall, 51 records from 50 unique studies were included.
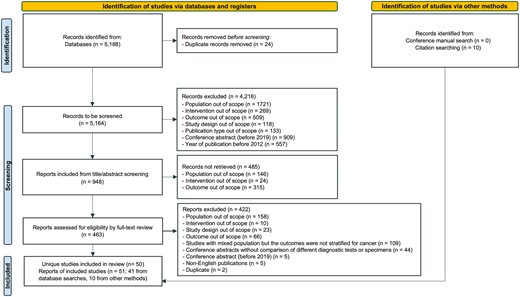
Study selection. Preferred Reporting Items for Systematic Reviews and Meta-Analyses (PRISMA) flow diagram for the systematic literature review, 1 January 2012–23 June 2022.
Study Characteristics
A summary of the study characteristics is shown in Figure 2. The majority (n = 34 [68%]) of studies enrolled acute care hospital inpatients. There were 2 clinical trials (1 randomized clinical trial [30], 1 single-arm trial [31]) and 48 (96%) observational studies. Within the latter, there were 6 cross-sectional studies [22, 26, 32, 52–54], 26 retrospective cohort studies [17–20, 23–25, 27, 28, 33–41, 57–63, 66], and 16 prospective cohort studies [3, 29, 42–51, 55, 56, 64, 65]. Sample size varied; the majority (n = 29 [58%) 18, 20, 24, 25, 30–34, 36, 37, 39, 43, 45, 46, 48–50, 52–58, 62–65] reported including between 50 and 200 participants (Figure 2A). Cancer types and suspected infection also varied among studies (Figure 2B and 2C). Studies were conducted across multiple geographic regions (Supplementary Figure 1A). While diagnostic test types assessed in studies varied among regions, culture was universally assessed (Supplementary Figure 1B). Half (n = 25) [17–20, 22–24, 26–29, 33–35, 44, 46–48, 52, 53, 59–62, 67] of the studies assessed culture methods alone, 19 studies [3, 21, 25, 30–32, 36, 37, 42, 43, 45, 49, 50, 55, 57, 58, 63–65] assessed PCR diagnostic techniques, and 4 studies [38, 49, 57, 64] assessed metagenomic sequencing technologies. Other diagnostic tools were also evaluated or described, including diagnostic tests of BAL fluid, BDG, Aspergillus GM, other biomarkers, and serology.
![Characteristics of the studies (n = 50) included in the systematic literature review, 1 January 2012–23 June 2022. One study [17] did not specify population age group but was assumed to be adult patients, since there was no mention of pediatric population in the study. A, Patient sample sizes for the studies included in the systematic literature review are indicated. One study [17] did not report the number of patients enrolled and only reported the total number of culture samples or fever episodes. B, Patient cancer type focus for the included studies: patients only with solid tumor (ST;ie, sarcomas, carcinomas, general tumors, and solid malignancies; n = 4) [18–21]; patients with hematological malignancy (HM) and ST but either primarily ST (n = 5) [3, 22–25] or separate outcome data for ST available (n = 4) [26–29]; patients with HM (ie, acute lymphocytic leukemia, acute myeloid leukemia, myelogenous leukemia, acute undifferentiated leukemia, chronic myelogenous leukemia, non-Hodgkin lymphoma, Hodgkin lymphoma, myelodysplastic syndrome, macroglobulinemia, chronic myeloid leukemia, aplastic anemia, and chronic myelomonocytic leukemia; n = 23) [17, 30–51]; patients with HM and ST but primarily HM (n = 5) [52–56] or separate outcome data for HM available (n = 4) [26–29]; and patients with HM who received hematopoietic cell transplant (allogeneic or autologous; n = 9) [57–65]. C, Number of studies per suspected infection, including febrile neutropenia (n = 22) [20, 24, 26, 27, 29–31, 33–35, 44–50, 53, 54, 56, 62, 65], fever (n = 5) [17, 32, 41, 52, 61], respiratory infections (n = 11) [21, 23, 25, 36, 37, 39, 40, 42, 57, 58, 63], sepsis (n = 2) [3, 28], gastrointestinal infections (n = 2) [55, 59], urinary tract infections (n = 2) [18, 22], central nervous system infections (n =1) [64], and mixed types of suspected infections where patients experienced different symptoms or sites of infections (n = 5) [19, 38, 43, 51, 60].](https://oup.silverchair-cdn.com/oup/backfile/Content_public/Journal/ofid/11/1/10.1093_ofid_ofad616/3/m_ofad616f2.jpeg?Expires=1749122743&Signature=md0tz4SST-Fjc8r6YCRrMTl1VglO4xvKvW3EcWZZMmG2l4d7zTaWuXoVHLiwfKk12KE8R3HhXn5-SCf0WgmovreX0Eej~mzpPba8wlJREo74XQtOtvvKK7KB5wmRiqQM6ZmDIs3vUeRM1lm41tnzW7q2A~p7WjeRwQS~dXFSR7-3Y1hgnSKsCEOrCSBG9fqNrSPoIft7E2LZrT6J5pVYNS-bpw2~IV2W4bJM5G0v5UfoIRVJzHANfH65TKrBAhU-X3WKey-tPwWRteXOwWEfhoMI704JAwZ8~6CVcP9wMnid0VPHPt1Jn4XDjXY7Orrq9wvn8RR8ddP1PylJKAjI4Q__&Key-Pair-Id=APKAIE5G5CRDK6RD3PGA)
Characteristics of the studies (n = 50) included in the systematic literature review, 1 January 2012–23 June 2022. One study [17] did not specify population age group but was assumed to be adult patients, since there was no mention of pediatric population in the study. A, Patient sample sizes for the studies included in the systematic literature review are indicated. One study [17] did not report the number of patients enrolled and only reported the total number of culture samples or fever episodes. B, Patient cancer type focus for the included studies: patients only with solid tumor (ST;ie, sarcomas, carcinomas, general tumors, and solid malignancies; n = 4) [18–21]; patients with hematological malignancy (HM) and ST but either primarily ST (n = 5) [3, 22–25] or separate outcome data for ST available (n = 4) [26–29]; patients with HM (ie, acute lymphocytic leukemia, acute myeloid leukemia, myelogenous leukemia, acute undifferentiated leukemia, chronic myelogenous leukemia, non-Hodgkin lymphoma, Hodgkin lymphoma, myelodysplastic syndrome, macroglobulinemia, chronic myeloid leukemia, aplastic anemia, and chronic myelomonocytic leukemia; n = 23) [17, 30–51]; patients with HM and ST but primarily HM (n = 5) [52–56] or separate outcome data for HM available (n = 4) [26–29]; and patients with HM who received hematopoietic cell transplant (allogeneic or autologous; n = 9) [57–65]. C, Number of studies per suspected infection, including febrile neutropenia (n = 22) [20, 24, 26, 27, 29–31, 33–35, 44–50, 53, 54, 56, 62, 65], fever (n = 5) [17, 32, 41, 52, 61], respiratory infections (n = 11) [21, 23, 25, 36, 37, 39, 40, 42, 57, 58, 63], sepsis (n = 2) [3, 28], gastrointestinal infections (n = 2) [55, 59], urinary tract infections (n = 2) [18, 22], central nervous system infections (n =1) [64], and mixed types of suspected infections where patients experienced different symptoms or sites of infections (n = 5) [19, 38, 43, 51, 60].
Assessed Outcomes
Twelve (24%) studies reported test performance (Figure 3, Supplementary Table 6). Forty-four studies (88%) reported on clinical course, especially the prescription of antimicrobials (Figure 3, Supplementary Table 6). Thirty studies (60%) reported health outcomes, with 26 studies reporting mortality [19, 20, 23, 24, 27–30, 33, 35, 37, 41, 42, 44, 46–48, 51, 54–56, 58, 60, 61, 64, 68]. Sixteen studies (32%) reported HCRU or cost (Figure 3, Supplementary Table 6), including hospital length of stay (LOS) (n = 16) [18, 20, 24, 27, 28, 30, 44, 46, 51, 53–55, 59–62], intensive care unit (ICU) LOS (n = 5) [28, 30, 51, 59, 62], hospital admission (n = 4) [18, 20, 27, 55], and direct cost (n = 1) [59].
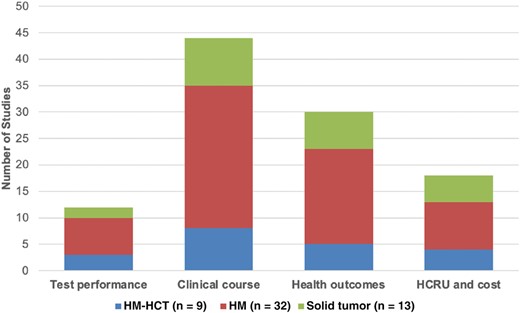
Number of studies by cancer type and outcomes of interest identified in the systematic literature review, 1 January 2012–23 June 2022. Abbreviations: HCT, hematopoietic cell transplant; HCRU, healthcare resource utilization; HM, hematological malignancy; ST, solid tumor.
Test Performance
All 50 studies reported pathogen yield (ie, percentage positive) for the respective diagnostic test assessed. Conventional diagnostic tests remain the major diagnostic methods utilized by researchers and clinicians. The proportion of cases with positive test results varied across study populations, pathogens, diagnostic methods, and specimens. Blood culture yield for any pathogen across 32 studies [17, 19, 20, 23, 24, 26–32, 34, 35, 41–45, 47–50, 52–54, 56, 58, 60–62, 65] demonstrated a range from 0% to 57.7% (Figure 4). For cultures of BAL fluid, 8 studies [19, 25, 39–42, 57, 63] reported 0.2% to 67.2% positive cultures (Figure 4). The 10 studies that assessed molecular diagnostic tools (ie, PCR and metagenomic sequencing) demonstrated varied yield compared with culture, although 2 studies demonstrated generally better yield by metagenomic sequencing (Figure 4). Only 3 studies commented on turnaround time. One study reported that the mean turnaround time from sample collection to communicating results was 33 hours for blood cultures and 20 hours for PCR [30], while another study reported mean time from sample collection to results of 10 hours and 4 hours for blood culture and PCR, respectively [45]. Turnaround time to communicate a result for microbial cell-free DNA (mcfDNA) metagenomic sequencing in the third study [49] was 52 hours for most samples.
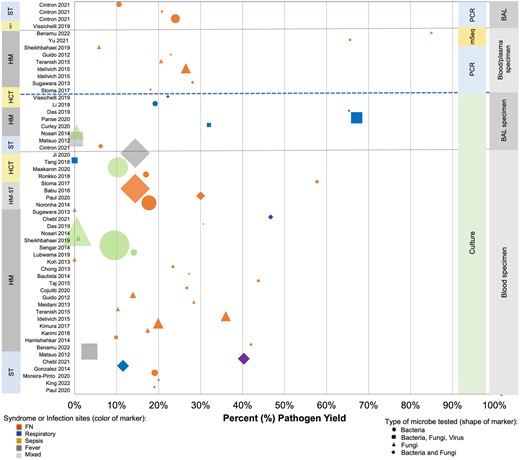
Reported pathogen yield from diagnostic testing of blood and bronchoalveolar lavage fluid. Data yielded from systematic literature review, 1 January 2012–23 June 2022. Percent yield of the specified diagnostic test (ie, culture, polymerase chain reaction, or metagenomic sequencing) based on specimen type is indicated for each study reporting these data. Size of the marker indicates relative study size with numbers ranging from 22 to 2751. Abbreviations: BAL, bronchoalveolar lavage; FN, febrile neutropenia; HCT, hematopoietic cell transplant; HM, hematological malignancy; mSeq, metagenomic sequencing; PCR, polymerase chain reaction; ST, solid tumor.
Infection detection performance of various diagnostic tests assessed in studies are shown in Figure 5. In 6 (50%) studies [3, 25, 45, 49, 50, 57], the reference test for comparison was culture. For the others, especially when a pathogen-agnostic test (eg, metagenomic sequencing) was the index test, clinical adjudication, a composite of conventional diagnostic tests, or clinical practice guidelines definitions, all usually including culture among the criteria, were the comparison reference. Conventional diagnostic tests, including blood cultures, commonly did not report test performance. Limited studies of biomarkers, including Aspergillus GM and BDG, from respiratory samples demonstrated variable performance depending on cutoff values (Figure 5). Molecular tests, both PCR and metagenomic sequencing, demonstrated generally good performance.
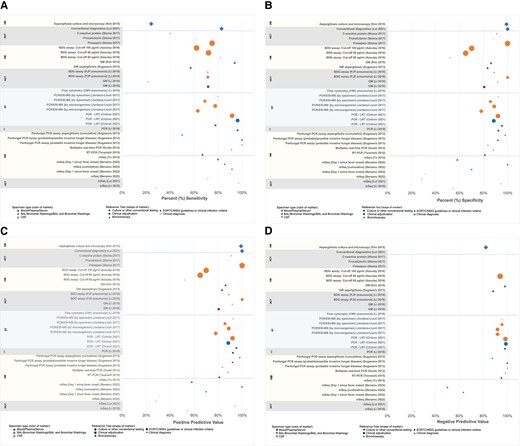
Test performance characteristics by cancer type, test, and sample. Data yielded from systematic literature review, 1 January 2012–23 June 2022. A, Sensitivity. B, Specificity. C, Positive predictive value. D, Negative predictive value. Size of marker represents the number of samples or patients in a study used to calculate data and ranged from 7 to 773. If no data are presented, the study did not report that value. Abbreviations: BAL, bronchoalveolar lavage; BDG, (1→3)-β-d-glucan; CMV, cytomegalovirus; CSF, cerebrospinal fluid; EORTC/MSG, European Organization for Research and Treatment of Cancer/Mycoses Study Group; GM, galactomannan; HCT, hematopoietic cell transplant; HM, hematological malignancy; LRT, lower respiratory tract; mSeq, metagenomic sequencing; PCR/ESI-MS, polymerase chain reaction–electrospray ionization mass spectrometry; PJP, Pneumocystis jirovecii pneumonia; RT-PCR, reverse-transcription polymerase chain reaction; ST, solid tumor.
Clinical Course
Thirteen studies (30%; solid tumor: 2 [3, 25]; hematological cancer: 9 [30, 37–40, 42, 43, 49, 54]; HCT: 2 [58, 63]) evaluated diagnostic test impact on the clinical course specifically antimicrobial therapy. Given that culture was often the reference test, its impact on antimicrobial therapy was usually assessed as a comparison for other tests. In a randomized controlled study assessing the impact of blood multiplex PCR results on antimicrobial therapy, multiplex PCR results led to a significantly shorter median time to the first change in targeted antimicrobial therapy compared with blood culture results (PCR: 21.4 hours range 16.2–46.3 hours vs control: 47.5 hours; P = .02). Further, PCR led to targeted antimicrobial therapy in 33.3% (7/21) of patients with a positive result and 9.5% (7/74) of all study group patients [30]. In a study of 429 patients with suspected sepsis, PCR/electrospray ionization mass spectrometry, a technology based on broad-range PCR amplification coupled with mass spectrometry, was able to identify bloodstream infections in 4–8 hours with 64.3% sensitivity by specimen as compared with blood culture, which required 24–48 hours [3]. A lower respiratory tract PCR panel study, in which results returned in approximately 4.5 hours, determined that while positive results did not likely impact therapy given the need to maintain broad coverage in the absence of sensitivity testing, negative results led to de-escalation in therapy in 4.8% [25]. In another retrospective study, the combined use of BAL bacterial culture, Aspergillus GM, multiplex viral PCR, and cytology led to antimicrobial therapy adjustments in 48 of 54 HCT recipients [63]. The diagnostic impact of plasma mcfDNA metagenomic sequencing was evaluated in 2 studies, which determined that antimicrobial therapy was or would have been changed in 47% (26/55) [49] and 59% (19/32) [38] of the included patients [38, 49]. This approach was assessed as likely enabling early treatment optimization, improving appropriate antibiotic use, and avoiding overtreatment [38, 49].
Health Outcomes
Mortality was the primary clinical outcome reported by most studies (n = 26) with percentages (0.4%–52% [19, 20, 23, 24, 27–30, 33, 35, 37, 41, 42, 44, 46–48, 51, 54–56, 58, 60, 61, 64, 68]) varying widely and not comparable given previously noted population differences, study design, and follow-up duration. Several studies assessed a potential association between a positive diagnostic result and mortality and found no significant correlation [30, 37, 55, 58], although study sizes were underpowered for such analyses.
HCRU and Cost
While 16 studies reported on HCRU or cost, they rarely assessed the impact of diagnostic tests on HCRU. The studies were not designed or powered to evaluate the diagnostic impact on HCRU. Studies included PCR [30, 37, 55, 58], culture [18, 20, 24, 27, 28, 30, 44, 46, 51, 53, 54, 59–62], or other types of conventional diagnostic methods (ie, BDG assay [51], Aspergillus GM [54], and a multiplex gastrointestinal PCR panel [55]). One study reported that a positive multiplex gastrointestinal panel was not significantly correlated with ICU LOS, while a negative panel was correlated with longer hospital LOS [55]. In another study, the hospital or ICU LOS did not differ among patients with febrile neutropenia who were diagnostically managed with or without the use of multiplex PCR [30].
DISCUSSION
Despite the recognized need for improved infectious disease diagnostic tests and the emergence of newer technologies, the findings of this SLR reflect that cultures either alone or in combination with other conventional diagnostic tests have persisted as the primary gold standard for the current diagnostic approach to acute infections in immunocompromised patients with cancer. The yield from culture was repeatedly demonstrated to be poor from blood and better but still limited from invasive samples. Molecular technologies, including PCR and metagenomic sequencing, may offer attractive alternatives to meet the complex diagnostic challenges presented by immunocompromised patients with cancer. However, assessing their performance by comparing with imperfect gold standards such as culture, or a composite of low sensitivity conventional diagnostic tests and clinical adjudication, complicates a true understanding of test performance. Nonetheless, pathogen-agnostic metagenomic sequencing technologies may fill distinct gaps noted in this population such as the need for accurate, noninvasive testing in medically fragile patients and broad pathogen identification in patients who have often atypical and nonspecific presentations and may be infected with >1 pathogen. Well-designed clinical studies evaluating diagnostic impacts on key outcomes with standardized definitions for determining clinical impact are needed rather than traditional comparisons to diagnostic tests of lower accuracy.
Biomarkers such as Aspergillus GM and BDG may prove useful in specific clinical contexts, although they demonstrate differing test performance depending on clinical site sampled (BAL > serum) [69, 70], have targeted pathogen spectrums for application [5], and may have limited availability in a region or institution [71, 72]. Despite lack of standardization and dependence on in-house methodologies and validations [5, 6], PCR-based assays were the most commonly studied tests, providing a reasonable turnaround time (ie, hours to days). Similar to biomarkers, they demonstrated generally good but variable performance depending on PCR type and differing performance depending on the clinical site sampled, including improved diagnosis of proven or probable invasive fungal disease with tissue or body fluid samples compared with blood [6]. However, although invasive sampling such as BAL may be useful when positive in guiding appropriate therapy for lower respiratory tract infections, such a procedure may not be feasible in many immunocompromised and fragile patients with cancer. Furthermore, positive results, whether from an invasive sample or blood, may not distinguish infection from colonization, such as with PCR detection of Aspergillus [72, 73] or Pneumocystis jirovecii [74] in BAL samples. PCR assays are targeted to specific microbes or specified panels of microbes [14], and while pathogen-specific biomarkers and PCR assays may have reasonable negative predictive value to exclude infection, their utility as individual screening tools to identify infection remains somewhat limited when the differential diagnosis is broad [70, 75, 76]. PCR has been combined with other technologies to capitalize on the advantages of each, such as real-time PCR plus 16S/18S ribosomal RNA sequencing. However, extended PCR tools also have limitations, including potential for contamination owing to their high sensitivity, lack of standardization, and cost and accessibility issues [45, 77].
Metagenomic sequencing technologies demonstrate promise for diagnosing suspected acute infections in immunocompromised patients with cancer with high test performance and additive detection and identification compared with conventional diagnostics. An advantage of the pathogen-agnostic characteristic of these tests is the observed detections of a wide breadth of diagnostically challenging and uncommon pathogens, and noninvasive sample testing may allow avoidance of invasive testing in some circumstances [38, 49]. Metagenomic sequencing can identify rare and challenging microorganisms such as Nocardia, Legionella, Toxoplasma, and Pneumocystis jirovecii [38]. Yet, it can also detect microbes of currently unclear clinical significance [38], and antimicrobial resistance marker detection capability is not yet routinely available as it is currently being validated. Therefore, like all diagnostic tests, metagenomic sequencing results need careful interpretation and will similarly benefit from orthogonal confirmatory testing.
The value of advanced molecular diagnostic tests such as extended PCR or metagenomic sequencing may offer the most utility in the immunocompromised host given their broad susceptibility to multiple opportunistic pathogens and often atypical presentations. In both immunocompromised and immunocompetent populations, advanced diagnostic tests tend to be used as a last resort in patients lacking diagnoses after conventional testing [14, 78] or in specific scenarios presenting diagnostic challenges [79–82]. However, in immunocompromised patients in particular, rapid identification of the etiologic pathogen is critical. Early application of advanced molecular tests in the infection course in well-defined scenarios, such as pneumonia or neutropenic fever, should be considered to improve the diagnostic yield [83, 38, 49], as well as consideration for serial sampling in specific patient populations like allogeneic HCT recipients [84]. Studies of the clinical utility of systematic application of advanced molecular tests in clearly defined contexts will be important to inform best practice, especially for immunocompromised patients with cancer.
Diagnostic strategies employing a combination of tests in the appropriate clinical setting have been reported to improve overall diagnostic performance and pathogen yield [3, 45, 50]. Similarly, the utility of metagenomic sequencing, in particular, has been noted as a valuable adjunctive assay to conventional diagnostic tests in immunocompromised patients with cancer or HCT and suspected infection [49, 71, 85]. In a prospective observational clinical study of the use of metagenomic sequencing of cell-free DNA from plasma samples obtained within 24 hours of enrollment of patients who had hematological malignancy or received HCT and had suspected pneumonia and a BAL sample collected or tested, the combination of plasma mcfDNA sequencing and usual care testing identified a probable cause of pneumonia in 42.2% patients, equating to a 12.1% absolute increase in diagnostic yield [83]. This finding further supports the utility of broad, pathogen-agnostic testing early in the diagnostic workup of an immunocompromised patient in conjunction with other testing.
Clear gaps exist in the literature for determining diagnostic impact on clinical course or outcome, HCRU, and cost. Few studies have assessed the clinical impact of specific diagnostic tests, although studies of PCR and metagenomic sequencing did highlight their respective abilities to accelerate treatment optimization and impact clinical management by providing faster and more specific pathogen identification. The number of molecular tests with better performance characteristics will continue to grow. Efforts to improve diagnostic stewardship require better diagnostic tools as well as a more comprehensive understanding of how well these diagnostic tests correlate with clinical outcomes [86].
Limitations of SLR Study
The SLR identified 50 studies meeting inclusion criteria, and although the search was comprehensive, the parameters of the search criteria may have been too restrictive or the terms used for searching databases may not have matched those used in some relevant references, so some studies may not have been identified. To address the potential for missing references, a supplementary manual search through relevant conference abstracts and bibliographies of recent reviews and selected studies of the topic was conducted and yielded additional studies. The included studies had various limitations, including retrospective and noncomparative designs, relatively small sample sizes, and single center data. Pathogen-specific diagnostic results were only included in the findings if they were assessed in the context of the broader infectious disease diagnostic landscape. There was notable variability in diagnostic approaches (ie, how, when, and which tests were used) and management decisions (ie, which, when, and how antimicrobials were administered). Finally, given that studies from multiple regions and institutions were included, differences in individual clinical practices and hospital-level policies as well as accessibility of various diagnostic tests may have impacted the respective study outcomes. The relatively small number of included studies identified for the 10-year study period highlight the need for targeted, well-designed comparative studies of both existing and novel diagnostic platforms to enhance understanding of the optimal approaches to diagnostic testing and improve antimicrobial stewardship and patient outcomes.
CONCLUSIONS
The findings of this SLR emphasized the continued dependence on conventional diagnostic tests, especially culture, which have poor diagnostic yield in immunocompromised patients with cancer. Limited data exist overall, but this SLR demonstrated an almost complete lack in key areas—health outcome, HCRU, and cost—to inform the potential value of various diagnostic tools. Both PCR and metagenomic sequencing can potentially improve diagnostic gaps and provide rapid detection and pathogen identification to facilitate earlier and appropriate escalation or de-escalation of antimicrobials. However, relying on comparisons with less sensitive conventional diagnostic tests to demonstrate their optimal utility is unrealistic for advancing diagnostic stewardship. Real-world evidence for novel molecular tests regarding test performance and clinical course, specifically as an adjunct to conventional diagnostic tests, and diagnostic approaches to optimize antimicrobial therapy in immunocompromised patients [38, 49, 71, 85, 87], suggest the potential for cost savings and improved patient outcomes. However, studies are critically needed to determine the optimal clinical scenarios for implementing improved diagnostic strategies, particularly their potential impacts on health outcomes such as antimicrobial use, HCRU, cost, and mortality.
Supplementary Data
Supplementary materials are available at Open Forum Infectious Diseases online. Consisting of data provided by the authors to benefit the reader, the posted materials are not copyedited and are the sole responsibility of the authors, so questions or comments should be addressed to the corresponding author.
Notes
Acknowledgments. We thank Victoria E. Wagner, PhD, a medical writer contracted by Karius, for assembly and preparation of tables and figures as well as assistance with editing text sections in accordance with Good Publication Practice (GPP3) guidelines (http://www.ismpp.org/gpp3). The authors also thank Frederick (Rick) S. Nolte, PhD, for his thoughtful comments on the manuscript.
Author contributions. S. Y. P. coordinated the study design and data acquisition. All authors contributed to analyzing and interpreting the data. S. Y. P. drafted the initial manuscript. K. J., R. T., and J. A. H. critically reviewed and revised the manuscript and approved the final version. The corresponding author (J. A. H.) attests that all listed authors meet authorship criteria.
Patient consent. Patient consent does not apply as this study does not include factors necessitating patient consent.
Data sharing. All relevant data are available in the article and the Supplementary material.
Financial support. This study was supported by Karius, Inc.
All authors have submitted the ICMJE Form for Disclosure of Potential Conflicts of Interest. Conflicts that the editors consider relevant to the content of the manuscript have been disclosed.
References
Author notes
Potential conflicts of interest. R. T. and J. A.H. are compensated members of the Scientific Advisory Board for Karius. S. Y. P. is employed by Karius. K. J. reports no conflicts.
Comments