-
PDF
- Split View
-
Views
-
Cite
Cite
Jacob S Young, Ramin A Morshed, Shawn L Hervey-Jumper, Mitchel S Berger, The surgical management of diffuse gliomas: Current state of neurosurgical management and future directions, Neuro-Oncology, Volume 25, Issue 12, December 2023, Pages 2117–2133, https://doi.org/10.1093/neuonc/noad133
- Share Icon Share
Abstract
After recent updates to the World Health Organization pathological criteria for diagnosing and grading diffuse gliomas, all major North American and European neuro-oncology societies recommend a maximal safe resection as the initial management of a diffuse glioma. For neurosurgeons to achieve this goal, the surgical plan for both low- and high-grade gliomas should be to perform a supramaximal resection when feasible based on preoperative imaging and the patient’s performance status, utilizing every intraoperative adjunct to minimize postoperative neurological deficits. While the surgical approach and technique can vary, every effort must be taken to identify and preserve functional cortical and subcortical regions. In this summary statement on the current state of the field, we describe the tools and technologies that facilitate the safe removal of diffuse gliomas and highlight intraoperative and postoperative management strategies to minimize complications for these patients. Moreover, we discuss how surgical resections can go beyond cytoreduction by facilitating biological discoveries and improving the local delivery of adjuvant chemo- and radiotherapies.
A maximal safe surgical resection is recommended as the upfront management for patients with suspected or proven glioblastoma (GBM) or lower-grade diffuse gliomas (LGGs) by all major American and European Oncology societies.1,2 Surgical technique and technological advances in the operating room have improved the ability of brain tumor surgeons to safely identify and preserve functional cortical and subcortical regions and locate residual tumor cells. Moreover, postoperative patient management has evolved to improve patient safety and recovery following tumor resection. Given the complexity of these specialized procedures, it is not surprising that high-volume surgeons and centers have been shown to improve outcomes for patients undergoing glioma resections.3,4 In this state-of-the-art review, the neurosurgical management of diffuse gliomas is described from initial imaging interpretation of an intra-axial lesion to the intra-operative techniques for maximizing extent of resection safely. Finally, we will address cutting-edge, surgically implemented adjuvant therapy strategies for the purpose of improving patient outcomes.
Understanding Brain Tumor Classification Criteria in the Molecular Era
Any discussion on the surgical management of diffuse gliomas in adult patients must begin with the recently updated Brain Tumor Diagnosis and Classification schema issued by the World Health Organization in 2021.5 This update assigned an even greater emphasis on the molecular characteristics of a tumor when determining a diagnosis, and as a result brain tumor neurosurgeons must be familiar with these molecular markers to understand patient diagnosis, prognosis, and ultimately management.
The most significant change in the 2021 WHO classification criteria for diffuse gliomas was the elimination of the entity IDH-mutant glioblastoma in adults. In addition, the relevance of homozygous CDKN2A/B deletions on grading for IDH-mutant astrocytomas is now formally recognized—with the presence of a homozygous CDKN2A/B co-deletion resulting in a WHO Grade 4 IDH-mutant diffuse astrocytoma diagnosis. Oligodendrogliomas continue to be classified as either Grade 2 or Grade 3 and must possess both an IDH-mutation and whole-arm deletions of Chromosome 1p and 19q.
Importantly, even with the new WHO classification schema, the tumor grade and/or pathology does not change the upfront surgical plan for diffuse gliomas in adults. Guidelines from all the major American and European oncology and neuro-oncology societies state that the initial management strategy for patients with a lesion concerning for a diffuse glioma is a maximal safe resection.1,2 As a result, a biopsy for tissue confirmation prior to definitive surgical resection is not required for lesions consistent with low- or high-grade diffuse glioma on imaging.
The added emphasis on molecular tumor characteristics has placed increased importance on genomic sequencing from tissue specimens to ensure accurate diagnosis.6 This practice has reduced inter-rater variability and improved the diagnostic accuracy for diffuse gliomas, particularly when the tissue specimen volume is limited.7 Nevertheless, neurosurgeons should be aware of the tissue requirements for the molecular and pathological assays performed, particularly when performing smaller resections or biopsies.
Preoperative Planning
Imaging Interpretation
Paramount to surgical planning is imaging evaluation and interpretation. This involves characterization of a tumor’s location—particularly in reference to eloquent cortical and subcortical regions and critical vascular structures. To achieve adequate pre-operative characterization of a lesion, high resolution contrast enhanced T1 weighted sequences, T2/FLAIR sequences, diffusion weighted imaging, susceptibility weighted imaging, and perfusion sequences should routinely be obtained. Certain imaging characteristics, such as T2-FLAIR mismatch, central necrosis, peripheral enhancement, diffuse restriction, and continuity with the cortex can be used to predict if lesions are low- or high-grade and can even be used to predict tumors molecular phenotype.8–10 In addition, if an imaging lesion may represent a low grade diffuse glioma but lacks certain characteristics of a neoplasm such as mass effect or expanded cortex and the diagnosis is in question, advanced MR imaging to detect the oncometabolite 2-hydroxyglutarate (2-HG) with spectroscopy can be quite valuable.11 However, as mentioned above, while the grade and molecular traits of the tumor are prognostic and relevant for adjuvant therapy choices, the surgical goal for all diffuse gliomas is a maximal safe resection, meaning a supramaximal resection of lesional tissue when feasible.
There are several preoperative functional and anatomic imaging studies, including diffusion tensor imaging (DTI) and functional MRI (fMRI) that can be integrated into the overall surgical plan. However, these imaging modalities do not necessarily act as a replacement for intraoperative mapping. While DTI is useful to predict the location of white matter tracts, can aid in surgical planning, and may guide when to begin intraoperative stimulation mapping,12 DTI alone cannot be used as a replacement for intraoperative electrical stimulation mapping.13 In addition, functional MRI (fMRI) can help identify and characterize regions involved in task-specific tasks Yet, this modality lacks the specificity and anatomic spatial resolution necessary to identify regions critical for language function and is not necessarily a replacement for intraoperative mapping.14 Accordingly, many brain regions presumed to be eloquent based on preoperative anatomic or functional imaging are actually not eloquent during direct electrical stimulation mapping intraoperatively.15 As such, “connectomic” maps based on DTI and fMRI are not currently reliable enough to determine eloquence or craft resection plans in the preoperative setting.
Imaging interpretation is more difficult after initial treatment when there is concern for progressive disease. For instance, anatomical MRI sequences are often not sufficient to distinguish true tumor progression from treatment-related changes or pseudoprogression. Physiologic sequences can be used to aid in this distinction for ambiguous lesions, but even still, tissue sampling is often required to confirm the presence and/or quantity of recurrent, viable tumor.16 O-(2-[18F] fluoroethyl)-l-tyrosine (FET) or 3,4-dihydroxy-6-18F-fluoro-l-phenylalanine (F-DOPA) PET scans are valuable imaging adjuncts that have been shown to have a higher accuracy than conventional MRI to distinguish treatment related changes from glioma recurrence.17 Newer techniques like liquid biopsies are under investigation and may provide additional information about the state of disease, and with the advent of machine learning, radiomic approaches to incorporate artificial intelligence-based decision support into the assessment of MRI lesions have been shown to improve upon the manual assessments of tumor burden (Figure 1). These techniques have begun to be incorporated into clinical trial designs and patient care at some institutions.18,19
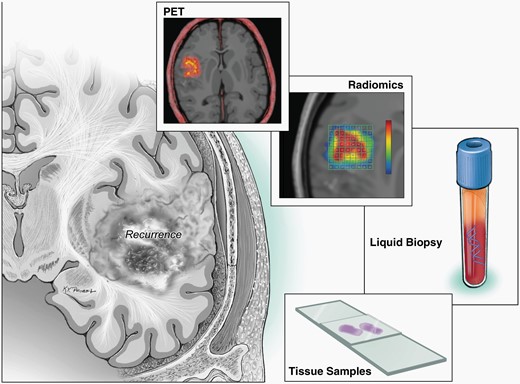
Schematic demonstrating techniques for disease monitoring, such as liquid biopsies, radiomics, repeat biopsies, traditional MRI images.
Preoperative Patient Evaluation
In addition to a standard preoperative evaluation by anesthesia, patients with tumors near language regions should have pre-operative language assessments to determine baseline performance scores and introduce the patient to the tasks that will be used intraoperatively. If there is any question of hemispheric language dominance, preoperative magnetoencephalography, navigated transcranial magnetic stimulation, or fMRI can help localize the side of language function, but similar to DTI, these preoperative functional studies are not suitable to replace intraoperative mapping.20,21 Behavioral and mental health assessments can be considered when patient’s report high levels of distress, depression, or anxiety, all of which are common in patients with diffuse gliomas, impair patient quality of life, and can interfere with intraoperative task performance during awake mapping procedures.22 Moreover, quality of life metrics can be incorporated into the pre- and postoperative patient evaluations and can serve an important role in the discussion of surgical decision-making, particularly for patients with low grade or recurrent lesions. Some surgeons utilize virtual reality and 3D printed models to construct a surgical plan during the preoperative evaluation. Neuropsychological, cognitive, and quality-of-life metrics are becoming more commonplace during the initial work-up of patients with diffuse gliomas, and these measures are useful for understanding the comprehensive impact these diseases have on patients daily functioning over time. Although resource availability and logistical hurdles exist in implementing these multidisciplinary assessments prior to surgery, the incorporation of telehealth into healthcare has created opportunities for patients and providers to perform these evaluations remotely, reducing the burden and some of the challenges associated with obtaining these important preoperative evaluations.22
For patients with large lesions, significant peritumoral edema, midline shift, or pre-operative language function that is too poor for intraoperative mapping (i.e. baseline error rates > 30%), patients can be admitted to the hospital for intravenous dexamethasone and mannitol in an effort to reduce the mass effect from peritumoral edema and preoperative language assessments can be repeated to determine if improvements in baseline performance make awake language mapping feasible.23 If task performance fails to improve sufficiently, the tumor may be resected in a staged approach with the lowest risk language areas removed with asleep motor mapping when appropriate and then a second stage awake approach can be considered to remove the residual lesion depending on the patients performance.24
Surgical Advances for Diffuse Gliomas
Surgical Strategy: The Goal is Supramaximal Safe Resection for All Diffuse Gliomas
Seminal work from the MD Anderson group provided some of the earliest evidence supporting aggressive resections for glioblastoma.25 Although some readers interpreted this paper as supporting an “all-or-nothing” approach with improvements in survival only after EOR surpassed 98% of the contrast-enhancing disease, the data showed increasing survival with increasing degrees of resection above 88% was associated with improved survival regardless of adjuvant treatment. In the era of volumetric tumor measurements, a stepwise increase in survival was shown as EOR of contrast-enhancing disease increased, and this was true even at the highest levels of EOR, with EOR of 100% of the contrast enhancing disease providing substantial survival benefit above 98% EOR.26 Subsequent studies employing volumetric assessment of tumor size affirmed the benefit of maximal EOR of the contrast enhancing (CE-EOR) disease, and even demonstrated a stepwise increase in overall survival as CE-EOR increased from 90% to 100%.26 The best prospective data supporting aggressive, maximal resections for patients with GBM comes from the randomized control trial investigating fluorescent guided surgery aids like 5-ALA, which definitively showed that patients who received fluorescent dyes had improved EOR and better overall survival.27 Importantly, there is also general agreement amongst brain tumor neurosurgeons regarding which tumors are feasible to resect and which lesions are not amenable for safe resection.28
Recent publications in the molecular era have suggested that supramaximal resections that leave the least amount of residual noncontrast enhancing (nCE) disease provide superior outcomes compared to gross total resection (GTR) of only the CE disease for all diffuse gliomas, regardless of histology, tumor mutational profile, or grade (Figure 2).29,30 Li et al. from MD Anderson published in 2016 a study that reported improved overall survival (median survival > 20 months) when more than 50% of the nonenhancing FLAIR abnormality was removed in addition to GTR of the CE component of the tumor.31 In a follow-up study from the University of California, San Francisco, in a homogenous cohort of patients that all received standard Stupp protocol adjuvant therapy after surgery, patients younger than 65 years old who had supramaximal resections that left < 5.4 ml of FLAIR abnormality had the best outcomes in the series.32 Other groups have reported similar benefits of supramaximal resections for IDH-WT GBM, although it remains to be determined if resections taken beyond the FLAIR abnormality and into normal appearing tissue on MRI provide an even greater additional relative benefit to “FLAIRectomies” for GBM.33–35 Indeed, a large multicenter, international retrospective series confirmed the value of supramaximal resections that removed greater than 60% of the nCE disease or left a residual nCE volume < 5 cm3.36 However, the benefits of aggressive resections are diminished or abrogated completely if the patient experiences a postoperative neurological deficit, particularly a postoperative motor deficit.37,38 This makes the interpretation of FLAIR signal abnormality important, as peritumoral edema, despite containing infiltrating tumor cells, that is not mass-like is not always considered non-enhancing tumor in manuscripts describing supramaximal resections. Moreover, in the high grade setting, where this FLAIR signal more commonly invades white matter tracts, instead of “pushing” or displacing the tracts,39 the interpretation of nonenhancing tumor versus surrounding edema is critical to balance aggressive resection with safety and preservation of function. Importantly, while this is an area of ongoing research and an area where consensus definition is crucial for patient care, maximal resection has not been shown to put patients at risk of postoperative NIHSS or KPS decline in the upfront resection setting.40,41
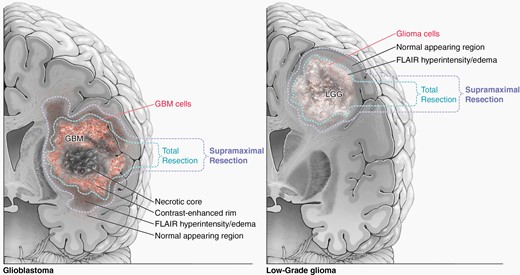
Illustration showing supramaximal resection definitions for high-grade (a) and low-grade (b) gliomas.
As the definition of a GTR has historically been based on the contrast-enhancing portion of the tumor for high-grade lesions, consistent terminology for “total” and “supramaximal” resections is critical and recently was defined by Karschnia et al.42 For glioblastomas, supramaximal resections are defined as resections beyond the contrast-enhancing margin into tumor regions of FLAIR abnormality. For nonenhancing, low grade gliomas, supramaximal resections are those carried beyond the areas of FLAIR abnormality, and recent evidence suggests this provides additional benefit for LGG compared to traditional GTR.43,44 Similar to glioblastoma, studies arguing in favor of aggressive resection for LGG reported survival benefits with GTR of the FLAIR abnormality, as this delayed malignant transformation and improved overall survival.45–48 The majority of these reports took place before the molecular era of neuro-oncology, and there have been some retrospective series that state outcomes for patients with 1p/19q co-deleted IDH mutant tumors (i.e. oligodendrogliomas), which are more chemosensitive than astrocytomas, are not as dependent on EOR. However, a recent single-center observation study with long-term follow-up reported lower residual tumor volume after initial resection was a strong prognostic factor in both IDH-mutant astrocytomas and oligodendrogliomas49 and a large multicenter retrospective cohort with over 750 patients and 20 years of follow-up found EOR > 75% and minimal residual tumor volumes resulted in the longest overall survival for oligodendroglioma patients.44
When low- or high-gliomas recur, the data suggests reoperation should be considered if the patient has good functional status and the tumor recurrence is amenable to re-resection. Although these patients are more heterogenous with respect to their prior therapy, multiple reports have indicated that reoperation can be performed safely and improve progression free and overall survival for patients with recurrent disease.50–54 The international, multicenter RANO-Resect consortium recently published results from nearly 700 cases of recurrent IDH-WT glioblastoma and found that patients who underwent re-resection and had < 1 cm3 of contrast-enhancing disease had better overall survival than patients who did not undergo re-resection.36 Interestingly, patients who underwent supramaximal re-resections did not have added benefit beyond the maximal re-resection cohort, although supramaximal resections in the recurrent GBM setting were frequently accompanied by new neurological deficits, and the distinction of FLAIR disease from treatment related gliosis is challenging. In the setting of recurrent IDH-mutant LGG, the recently published INDIGO clinical trial results demonstrated that vorasidenib, an oral brain penetrant mutant IDH1/2 inhibitor, could delay time to subsequent intervention and progression free survival for these patients when compared to placebo.55 These recent studies highlight the rapidly changing and exciting landscape for patients with recurrent low- and high-grade gliomas, and the decision to re-operate on recurrent disease is nuanced and often benefits from the input of a multi-disciplinary tumor board consisting of neurosurgeons, neuro-oncologists, radiation oncologists, and neuroradiologists.
When to Perform a Biopsy Only and How to Catalog Tissue During Surgery
While maximal safe resection is the standard for lesions amenable to resection, certain patient and tumor characteristics can shift the risk calculus in favor of a biopsy only. Recent work has shown that there is general agreement amongst high-volume tumor surgeons with respect to which lesions should be biopsied only, such as those that cross the midline or extend into more than 3 lobes (previously termed gliomatosis) with no mass effect, or cases where following adjuvant treatment disease recurrence versus pseudoprogression cannot be determined with noninvasive imaging.56 Certain patient characteristics, such as very advanced age, extreme frailty, very poor performance status, severe cardiopulmonary comorbidities, and patient preference can also influence the decision to pursue a biopsy rather than resection. Nevertheless, in situations where a resection is not safe or feasible, it is still important to obtain tissue to confirm the diagnosis, identify targetable genomic mutations, and guide therapeutic decision making.
When obtaining a biopsy, or collecting tissue specimens during tumor resection, brain tumor neurosurgeons should capture the location of the sample on the neuronavigation software, and efforts are underway to facilitate a more standardized approach to catalog the location and characterize of the tissue collected. Careful attention to sample location is especially important for Phase 0 studies where subsequent molecular analyses are needed to determine drug pharmacokinetics/pharmacodynamics. If the institution has the necessary brain tumor research infrastructure, after tissue has been confirmed as pathological on frozen analysis and some tissue has been sent for formalin-fixation and paraffin embedding for diagnostic purposes, any additional tissue should collected as a fresh specimen for biological analyses and the generation of human PDX tissue culture lines or human tumor organoid models.57
Surgical Methods: Stimulation Mapping Techniques
Direct electrical stimulation for the purpose of mapping cortical and subcortical function has repeatedly been shown to minimize post-operative deficits for patients undergoing glioma resection (Figure 3). In a large meta-analysis with 8,091 adult patients who underwent resection of glioma, authors found that awake mapping reduced the risk of late, severe neurological deficits from 8.2% to 3.4%.58 Additionally, a gross total resection was achieved in 75% of cases with intra-operative stimulation mapping, compared to only 58% of cases without stimulation mapping. Critically, minimizing postoperative deficits not only improves patients quality of life but also preserves the survival benefit of aggressive resections.38,59 These results were reinforced by a recent multicenter propensity score-matched analysis study (GLIOMAP), where in the matched cohort of over 500 patients there was a longer PFS and OS with fewer neurological deficits in the awake craniotomy cohort.41 These findings will be further explored in two upcoming prospective clinical trials: (1) SAFE (NCT03861299), a randomized prospective trial comparing awake craniotomy versus general anesthesia for patients with GBM in eloquent or near eloquent regions and (2) PROGRAM (NCT04708171) a prospective international trial investigating if specific types of intra-operative stimulation mapping (e.g. bipolar, monopolar, transcranial stimulation) yield better results for patients with GBM. While the vast majority of intra-operative mapping tasks aim to preserve motor and language function, evidence exists that executive functions can be identified with stimulation mapping and preservation of these cognitive domains will also be critical for preserving quality of life after glioma surgery.60 For certain patients with low grade gliomas whose professional life requires very high-functioning or skilled tasks, additional cognitive mapping tasks can be included in the intra-operative mapping paradigm.
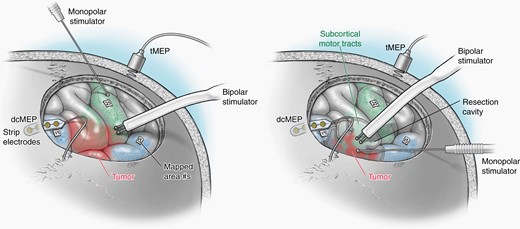
Illustration showing cortical and subcortical monopolar and bipolar mapping techniques.
Positive Versus Negative Mapping Techniques
Historically, a positive mapping site was considered necessary during mapping to ensure that “negative” sites were true negatives; however, negative mapping, where no positive sites are identified, was determined to be as safe as the positive mapping technique in a landmark article.61 Subsequently, negative mapping has repeatedly been shown to be safe for cortical and subcortical language mapping and is used to facilitate tailored craniotomy windows over the tumor.62 While negative mapping allows for smaller craniotomies and exposures, positive mapping remains a viable strategy and the approach should be tailored according to the lesions location and the patient’s symptoms based on the surgeon’s discretion.
Motor Mapping
Motor mapping, which is critical to minimizing postoperative hemiparesis that abrogates the survival benefit for extended restrictions and dramatically impairs patient’s quality of life, has evolved in recent years to include multiple stimulation modalities. Preserving motor function to a degree that maintains a high quality of life requires that patients retain their ability to perform complex motor skills requiring contraction and coordination of multiple muscle groups. Typically, an intraoperative decline in motor evoked potentials (MEPs) more than 50% from the baseline level is indicative of a permanent postoperative deficit.63 Multiple technical advances in motor mapping have facilitated the safe resection of gliomas in or near the Rolandic cortex, and these advances have made both cortical and subcortical motor fiber detection reliable and safe in both the awake and asleep setting.64 In the awake setting, transcranial MEPs often require current that generates a painful muscle contraction for the patient, but direct cortical MEPs are usually well tolerated for patients. The incorporation of monopolar, high-frequency subcortical motor mapping into either awake or asleep mapping strategy has allowed for a quantitative measurement of how close the resection is to the descending CST fibers (every 1 mA increase in amplitude is approximately 1 mm further from the descending CST with traditional train of 5 high frequency stimulation), which is critical to preventing iatrogenic or ischemic injuries to the motor fibers.65,66 The electrode and stimulation parameters influence the sensitivity and specificity of both subcortical and monopolar mapping, but in general bipolar motor mapping can produce more selective activation of the corticospinal tract (CST) when the CST is close to the stimulation site whereas monopolar motor mapping is more robust and effective when the CST is far from the stimulation site.67 Decreasing the high frequency monopolar subcortical motor mapping monophasic wave pulse to a train of 2 stimulation paradigm rather than the standard train of 5 paradigm can be helpful as the resection approaches the CST as the decreased stimulation train can improve subcortical CST detection specificity.68,69 One effective strategy is to utilize the bipolar stimulation to identify the M1 cortex and then rely on frequent monopolar subcortical stimulation during tumor resection to cautiously approach the subcortical motor fibers in combination with frequent MEPs to ensure integrity of the motor pathway.
Testing for apraxia, particularly for tumors in the supplementary motor area (SMA), can be done in addition to cortical and subcortical mapping of the corticospinal tract when the patient is awake,70 although this is not necessary to ensure normal long-term neurological function for tumors in the SMA region.71 For tumors located within the primary motor cortex (M1), Rossi et al. report a high-frequency cortical stimulation approach to identify somatotopic regions within M1 (a fast, more excitable posterior border and a slower anterior border), and while the architecture of these regions can be distorted by the tumor, if the faster, more excitable component of M1 is preserved, patients strength is much more likely to remain functional.72
Language Mapping
Bipolar Language Mapping
Low-frequency (50–60 Hz) biphasic pulses delivered via a bipolar probe to cortical and subcortical regions in awake patients has been the foundation of language mapping for decades. Various tasks are utilized to identify sites that are critical for the semantic, phonetic, and syntactic components of language function, with picture-naming serving as the work horse for intra-operative language mapping.73 Beyond picture-naming, other tasks such as text reading, picture-word interference, and sentence generation are often employed depending on tumor location and the patient’s preoperative language performance.74 While this approach is very safe, particularly in high-volume centers, there is some individual variability in the intraoperative electrostimulation parameters required to induce a positive response,75 and biphasic stimulation carries a small risk of inducing a seizure. Additionally, bipolar mapping lacks quantitative information regarding the distance to subcortical tracts.62
If patients are struggling to complete the intraoperative tasks, the surgical team can try a few different strategies to obtain a useable intraoperative map. For example, by minimizing the cortical map to the highest risk region that allows for an equatorial approach to the center of the tumor, the mapping portion of the procedure can be expedited and completed in patients who are fatigued, uncomfortable, or anxious. Additionally, limiting the intraoperative testing to words or pictures that patients correctly identified during the preoperative assessment can help ensure intraoperative positive mapping sites are true positives. Finally, using short (e.g. one or two syllable) words can improve the intra-operative performance for patients with moderate to severe pre-operative aphasia.
Monopolar Language Mapping
Traditionally, cortical and subcortical language mapping has been performed with low-frequency, bipolar stimulation. Similar to motor mapping, some groups have explored the use of high-frequency, monopolar, train of five stimulation to map language pathways both cortical and subcortically and found the technique to be safe and effective for mapping anomia and paraphasia.76,77 Importantly, cortical monopolar mapping using anodal stimulation while subcortical monopolar stimulation requires cathodal stimulation.78 As with the motor system, monopolar stimulation for speech mapping has a much lower risk of inducing a seizure and with similar sensitivity and specificity as bipolar stimulation. Also like motor mapping, some groups have pioneered the use of continuous subcortical monopolar stimulation with suction tips that also serve as a cathodal probe and shown safety with this technique.79
Avoiding Postoperative Ischemia
As mapping techniques have improved and facilitated an accurate, quantitative representation of subcortical white matter tracts, confluent areas of ischemia on the early postoperative MRI scan have been shown to be a major cause of new and persistent postoperative neurological deficits.80 Importantly, intraoperative relative hypotension has not been shown to be a risk factor for ischemic changes, suggesting tight intraoperative blood pressure regulation to minimize the risk of a hemorrhagic complication is safe.80,81 Certain tumor locations, such as the insula, appear to be risk factors for postoperative ischemia and brain tissue adjacent to the tumor may particularly susceptible to transient drops in blood pressure due to a relative regional under-perfusion compared to the tumor itself.82 To minimize the risk of postoperative ischemia, meticulous surgical technique to avoid direct or thermal injury to draining veins and perforating arteries is required, and the bipolar electrocautery ideally should be judiciously used during all subcortical tumor resection and must be avoided when within 1 cm of the CST. Additionally, papaverine can be used to avoid vasospasm of delicate lenticulostriate and insular arteries during tumor resection. When an irreversible MEP drop > 50% from baseline is detected intraoperatively, the majority of cases are due to an ischemic lesion that can be identified on the postoperative MRI scan; however, ischemic lesions can also be present and associated with neurological deficits in the absence of intraoperative MEP changes, suggesting the event may occur in a delayed manner.63
Surgical Approaches
Open Craniotomy
An open craniotomy for approaching intra-axial lesions provides a wide cortical exposure, allowing for the selection of a safe cortical entry site following cortical mapping that facilitates a trajectory to the equator of the tumor.83 During these operations, patients are positioned to utilize gravity so that the amount of healthy cortex obscuring the trajectory to the tumor is minimized. Prior to the craniotomy, mannitol and hyperventilation are often used for brain relaxation. If the patient is awake, prior to opening the dura the patient is awakened and asked to take a few deep breaths to exhale carbon dioxide and relax the brain. The patient’s body temperature must be kept euthermic during the operation, as hypothermia makes intraoperative mapping less reliable and can reduce patient cooperation. During the resection, the patient’s blood pressure is well-controlled to minimize the risk of hemorrhage into the resection bed. Following the craniotomy, the tumor is most commonly approached via a transcortical trajectory to the center or equator of the tumor, ideally along the tumor’s long axis to minimize retraction during resection of deep-seated tumors.83 The larger exposure allows for improved visualization of cortical windows and subcortical mapping as the resection progresses. Bimanual dynamic retraction is used to expand the subcortical resection margins within a relatively small cortical window, and fixed retraction should be minimized to avoid injury to the surrounding parenchyma.
Some groups have advocated for a minicraniotomy approach, which is typically described as a linear incision followed by a 3 cm or smaller craniotomy, which have become popular for nonintra-axial lesions such as aneurysms. Some surgeons use an exoscope rather than an operative microscope to improve visualization with these small openings. There have been reports of shorter operative times, shorter hospitalizations, and fewer complications with preserved rates of gross total resection following mini-craniotomy compared to traditional open craniotomies in case series84; however, these smaller openings limit the ability to map cortically and subcortically, which as mentioned above, can limit the ability to safely extend resections beyond the contrast-enhancing disease with a minicraniotomy approach. Furthermore, incisions and craniotomies at the time of initial tumor resection should always be made with the mindset that, at the time of tumor recurrence, they will still adequately facilitate tumor exposure. Moreover, the lengths of stay reported in these studies often are 5–7 days on average for both techniques, which is significantly longer that reported lengths of stay reported by some high volume centers following craniotomy for tumor resection.81,85
Keyhole Craniotomy
Even more minimally invasive approaches to intraaxial lesions have been used, termed keyhole or burr hole craniotomies. These very small openings offer limited exposure of the cortical surface for mapping, restricted visualization of deep white matter structures, and a narrow working-space, which may prove risky in the setting of positive cortical/subcortical mapping sites or intraoperative hemorrhage, but proponents of this technique argue that the small working corridor provided is adequate for awake, negative cortical mapping and safe, maximal tumor resections without the need for brain retraction.86 Like open craniotomies, surgical experience is critical to become facile and comfortable with these approaches utilizing a narrow working corridor. Reports have shown that these strategies can be used for tumors located in the nondominant and dominant frontal and temporal lobes and with good rates of gross total resection, supramaximal lobectomy, and acceptable complication profiles.86,87 Some surgeons have employed the endoscope or tubular retractors in combination with keyhole approaches to improve visualization.88 While tubular retractors may be useful for tumors in certain locations (e.g. lesions approximately 2–4 cm from the brain surface where the pathology is easy to differentiate from the normal brain parenchyma),89 and these approaches are important components of a brain tumor surgeon’s treatment arsenal, there has never been a trial exploring the neurological morbidity and extent of resection between keyhole and traditional open craniotomies, and as the goal of glioma surgery has shifted to more aggressive resections, these techniques may not offer the safest strategy towards achieving this goal.
Ablative Strategies
Ablative techniques are a modification on the keyhole approach, where a minimally invasive burr hole is made but then the tumor is ablated with hyperthermia via a laser (e.g. LITT) rather than resected with traditional techniques.90 Like open resections, LITT ablations should avoid being near functional subcortical pathways or blood vessels. Early reports with this approach have shown a correlation between extent of ablation (EOA) and progression free survival (PFS), with > 70% EOA as the cutoff for benefit.91 The ablation approach may be most useful for deeper, relatively well-circumscribed lesions that are not contacting the ventricular lining, although the ability to safely pursue supramaximal ablations of large volumes of tumor tissue remains unknown. Laser ablation was explored in a prospective, multi-site registry (LAANTERN, NCT02392078) and reported overall survival outcomes are similar to those of other trials, although the complication rate was slightly higher than that seen for traditional resections in high volume centers, and open surgical resections remain the standard of care as ablative techniques have not been used to achieve supramaximal ablations, do not consider function during the ablation, and there have not been any direct comparisons of EOA against EOR to date.92
Another ablative technique is to use high-frequency focused ultrasound (HiFU) to generate a precise region of thermal ablation. Although this approach is more commonly been used for focal lesions or functional conditions like essential tremor, and there are significant limitations with this approach for large volume tumor ablations or nonfocal disease.93 These ablative strategies are important tools in the surgeon’s arsenal and can be considered as long as the surgeon is comfortable that a maximal ablation with minimal morbidity can be achieved.
Determining Resection Margins and Boundaries—Maximizing Safety and Extent of Resection
Successfully identifying the margin between tumor border and normal brain parenchyma is critical to safe maximal resections for gliomas (Figure 4). The integration of new technologies and tumor labeling agents into the operating room has advanced the intra-operative detection of tumor cells and facilitated better, safer tumor resections. Currently, no techniques in practice allow for rapid, intraoperative detection of a tumors molecular genotype although pseudo-immunohistochemistry and sequencing techniques are rapidly advancing. While the surgical management of diffuse gliomas is not altered by the tumor’s molecular status, should targeted therapies become more effective for certain tumors, this rapid assessment of tumor genomics may facilitate tailoring the surgical and treatment strategy in a personalized medicine manner. In the following sections, different intra-operative strategies for identifying residual tumor cells are discussed.
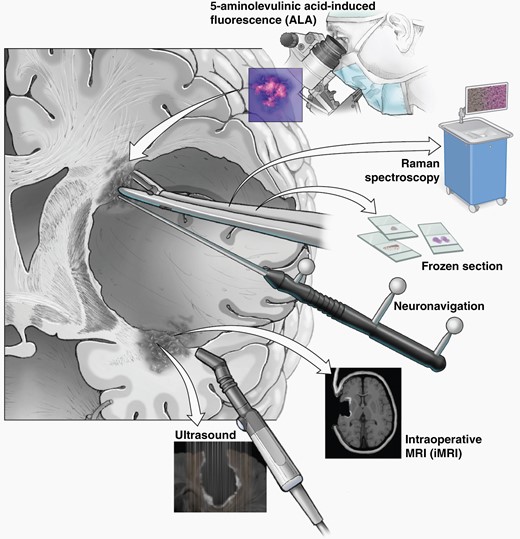
Neuronavigation, DTI, Intraoperative Ultrasound, and Mapping
The use of neuronavigation and DTI in the operating room has been shown to improve EOR and when these technologies are combined with direct electrical stimulation mapping, safety and extent of resection have been shown to be improved than using either technology alone.12,47 As resections progress, brain shift decreases the accuracy of navigation systems and tract projections, highlighting the critical need to only stop resections based on function identified with intra-operative mapping, and not on the location of tract fibers generated from pre-operative imaging.94 Intraoperative ultrasound, which can now be combined with navigation systems, has the advantage of providing real time information on the location of residual tumor at the resection margin or small satellite lesions around resection cavities, can be used to improve resections when the lesions are subtle or small.95 The ultrasound characteristics of the tumor depend on the type of lesion, with the necrotic core of a GBM appearing hypoechoic and low grade lesions appearing slightly hyperechoic to white matter, making this technique versatile and effective for most gliomas; importantly blood and air in the resection cavity can hinder the utility of this tool, particularly for recurrent lesions where gliosis is present.96 While navigated ultrasound can be particularly useful for localizing subcortical lesions at the beginning of resections, the tumor “margin” observed by ultrasound at the end of a resection is often blurred by the iatrogenic manipulation of the tissue during resection and blood products.97
Fluorescence Labeling
Agents that can highlight tumor cells under the operative microscope have been explored for decades to improve tumor resections. The two most commonly used fluorescent labels are 5-ALA and fluorescein. Both prospective and retrospective trials, including the Phase 2 multicenter prospective trial FLUOGLIO, have shown that fluorescein is safe and rates of GTR are much higher (around 90%) when fluorescein is used to identify tumor borders intraoperatively.98 Importantly, fluorescein stains both contrast-enhancing and nonenhancing tumor regions with a high positive predictive value (PPV > 96%).99
5-ALA has been used as an alternative to fluorescein and works in an apparent tumor specific manner,100 where it gets metabolized by the heme biosynthesis pathway into the fluorescent protoporphyrin IX. Notably, recent single-cell sequencing data suggests that non-neoplastic cells in the tumor microenvironment such as immune cells can also fluoresce, raising some questions about the specificity of these agents for tumor cells.100,101 A large randomized Phase 3 trial comparing 5-ALA to standard surgery found higher rates of GTR and increased PFS and OS in the 5-ALA group.27 Similar to fluorescein, the PPV is very high for contrast-enhancing regions, however the negative predictive value is only around 40%, limiting it’s utility to distinguish the absence of tumor in nonfluorescent tissue.102 Supporting the concept of supramaximal resections that go beyond the contrast enhancing disease, patients who had residual 5-ALA fluorescence despite complete resection of enhancing tissue on the post-operative MRI had worse overall survival than patients who had no residual fluorescence at the conclusion of the resection.103 The specificity and sensitivity of fluorescent labeling adjuncts in the recurrent disease setting has been less well established and there are limitations due to infiltrating inflammatory cells that cause a false positive 5-ALA signal.104,105 Nevertheless, fluorescence-guided surgery is a useful adjunct for identifying regions of residual tumor during tumor resection.
Stimulated Raman Histology
Stimulated Raman Histology (SRH) has emerged as a rapid, label-free, nondestructive method that utilizes light to create pseudo-H&E images from fresh tissue specimens. The intraoperative use of SRH has been demonstrated to yield a diagnosis quicker than a tradition frozen specimen (9.7 min vs 43 min) with no difference in the diagnostic accuracy between the two modalities.106 SRH has also been used to identify residual tumor at the margins of resection cavities, with a high degree of agreement between SRH specimens and traditional H&E specimens when reviewed by pathologists.107 The speed of Raman histology facilitates the assessment of multiple, serially collected samples to determine if the tumor cell burden is diminished or near-absent at the tumor margin.
Intraoperative MRI
Intraoperative MRI (iMRI) to identify regions of residual tumor before completing the resection and closure has been shown in multiple retrospective and one prospective randomized trial (NCT01394692) to improve extent of resection without increasing surgical morbidity compared to traditional neuronavigation alone.108,109 Improved EOR has been reported for both low- and high-grade lesions with iMRI.110,111 In practice, iMRI significantly prolongs the surgical procedure and adds complexity for the OR and anesthesia teams.112 Moreover, iMRI has never been shown to result in superior EOR when directly compared to tumor labeling with fluorescent compounds like 5-ALA.113 More work is needed to determine how iMRI integrates with other rapid intraoperative tumor margin detection methods like 5-ALA and Raman spectroscopy to ultimately establish the role of iMRI in the surgical management of diffuse gliomas.
Intra-Operative and Post-Operative Surgical Adjuncts to Improve Therapeutic Delivery of Agents
Surgically implanted biologically degradable carmustine, or Gliadel® wafers, were one of the first intra-operative drug delivery strategies, and were FDA-approved for newly diagnosed and recurrent glioblastoma after being shown to minimally improve overall survival compared to surgery alone.114,115 Recently, there has been a rejuvenated emphasis on interventional means for improving drug delivery or radiation therapy immediately after a tumor resection that incorporate intraoperative adjuncts (Figure 5).
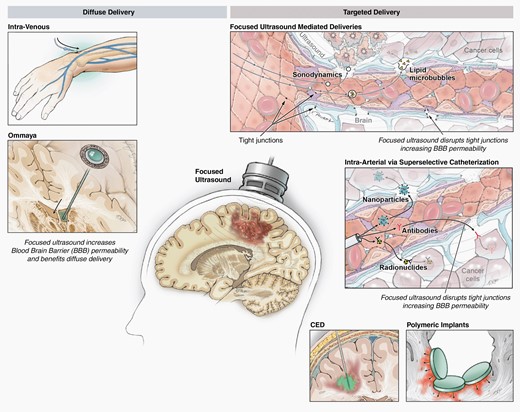
Drug delivery strategies/adjuncts that involve neurosurgeons, such as convection-enhanced delivery, radiation implants, low frequency focused ultrasound.
Radiation Implants
Given the majority of GBM recurrences are adjacent to the initial resection cavity where the highest density of residual tumor cells is located, radioactive brachytherapy implants have used to improve local control rates following tumor resections. Brachytherapy seeds applied at the time of surgery accelerate the time of radiation delivery compared to standard post-operative radiotherapy and may be less costly as this approach can cut down on the number of patient visits associated with adjuvant therapy.116 A variety of radioactive isotypes have been explored, with 131Cs (a low dose rate of brachytherapy) emerging as a promising agent for CNS tumors given its short half-life compared to 125I which translates to lower rates of radiation necrosis.117–119 Challenges with brachytherapy include the creation of “hot’ and “cold” radiation spots within the tumor due to strand distribution and the threat of radiation necrosis. Currently, there is no data to support intraoperative radiation or brachytherapy for diffuse glioma, with two clinical trials investigating 125I failing to show a survival benefit.120
The development and FDA-clearance of GammaTile for gliomas and brain metastases, which has 131Cs titanium encapsulated seeds embedded into a resorbable collagen-based carrier tile, has reinvigorated the interest in brachytherapy and launched the concept of surgically targeted radiation therapy (STaRT).121,122 Currently, GammaTile is a 2 cm × 2 cm square tile with four 131Cs seeds approved for newly diagnosed and recurrent malignant brain tumors capable of delivering 120–150 Gy at the cavity surface and 60–80 Gy at a depth of 5 mm design in a more uniform distribution than traditional brachytherapy seeds.123,124 The early clinical reports provide evidence of a reasonable safety profile for GammaTile implants, although there is the potential for delayed seed settling when the collagen scaffold is absorbed and the efficacy remains unknown. The safety and efficacy of GammaTile for CNS tumors is being explored in actively recruiting clinical trials (e.g. ROADS clinical trial)125 and early single-arm clinical trial data suggest a reasonable safety profile in recurrent GBM with relatively low rates of radiation adverse effects and modest, short term local control rates.126 Importantly, surgeons considering GammaTile should discuss with radiation oncology and neuro-oncology colleagues to ensure that patients remain eligible to enroll in any relevant clinical trials.
Focused Ultrasound
Low-intensity focused ultrasound combined with microbubbles, has emerged as a means of temporarily opening the blood brain barrier for the purpose of improving drug delivery to the tumor without causing tissue damage.93 The low-frequency ultrasound systems currently being explored in clinical trials include an implantable device, called SonoCloud (Carthera, Paris, France),127 that does not use image guidance, the ExAblate (InSightec, Haifa, Israel) system, which uses MRI images to guide external, transcranial ultrasound beams to the target, and the NaviFUS system which uses neuronavigation and a pretreatment MRI to localize the ultrasound.128 Preliminary results for these early-phase clinical trials indicates a substantial increase in drug levels in the peritumoral brain tissue after sonication. Another potentially cytotoxic strategy with focused ultrasound uses this modality in combination with sonosensitizers, such as 5-ALA, to produce a local cytotoxic effect, termed sonodynamic therapy.129
Some groups are also utilizing low frequency FUS mediated BBB disruption to augment the amount of circuiting tumor genetic material and tumor antigens, either to enable more accurate liquid biopsies to monitor for tumor progression or treatment response or expose the immune system to more potential targets.130,131 The diverse potential of focused ultrasound for neuro-oncology is appealing, and as more studies emerge around this technology neurosurgeons will need to be familiar with the advantages and practical applications of each device.
Convection Enhanced Delivery
Convection enhanced delivery (CED) utilizes stereotactically placed catheters to deliver drugs via bulk flow to the tumor region, allowing for high concentrations of the drug to be achieved in the tumor without systemic toxicity.132,133 Better catheter design and improved understanding of catheter positioning has considerably improved CED approaches,134 but the inability to deliver repeated treatments has limited this strategy. One solution to this problem is to embed the therapeutic agents in nanoliposomes to prolong the drugs release after administration (NCT02022644), while a recent publication explores implantable drug pumps as another strategy to facilitate repeated drug dosing with CED.135 As technology and the agents delivered via CED continue to improve, neurosurgical oncologists should remain familiar with this drug delivery technique.
Postoperative Care Following Craniotomy for Tumor Resection
The postoperative management of glioma patients is critical to minimizing morbidity. Preventative interventions can be focused on numerous factors including blood pressure control to minimize the risk of a postoperative hematoma, short-term antibiotics for infection prevention, antiepileptics to minimize seizure risk, implementation of steroids to decrease cerebral edema-induced symptoms, and pharmacologic prophylaxis for prevent of deep vein thrombosis. Although randomized control trials are lacking with respect to postoperative patient management, the available evidence can be used to guide effective and safe postsurgical patient care and the establishment of enhanced recovery after surgery (ERAS) pathways following a craniotomy are beginning to introduce standards to the practice.136
Blood Pressure Management
Postoperative hypertension has been associated with a risk of intracranial hemorrhage both within the resection cavity and remotely after a craniotomy for tumor resection.137,138 A prospective observational study demonstrated that a history of hypertension is the only independent risk factor for postcraniotomy hypertension, and a large percentage of patients require anti-hypertensive treatments transiently after craniotomy for glioma resection.139 Despite the risk of post-operative hypertension induced hemorrhage, standardized blood pressure goals after a craniotomy for glioma resection are not well-defined. Within the spontaneous intracerebral hemorrhage patient population, a goal systolic blood pressure < 140 mm Hg has been shown to improve functional outcomes compared to more lenient blood pressure goals, and a target systolic blood pressure < 140 has been described in the literature following elective brain tumor resection as well.140,141 Further implementation of even lower systolic blood pressure goals (≤120 mm Hg) may be considered following craniotomy for tumor resection since the low intracranial pressure after surgery minimizes the risk of inadequate cerebral perfusion that can be seen with very low systolic pressures.
Corticosteroid Use
Corticosteroids, most commonly dexamethasone, have been used to alleviate vasogenic edema after tumor resections for decades. The initial experience with dexamethasone typically utilized high-dose regimens like 4 mg dosed every 6 hours to minimize neurological deficits after surgery; however, high-dose and/or prolonged steroid use after resection has been associated with numerous side-effects and even worse survival in patients with glioblastoma.142 Additionally, given the half-life of dexamethasone is between 36 and 54 hours, prior reports have advocated for less frequent dosing including a twice-daily dosing.143 Moreover, if patients are receiving any immune-activating therapies, steroids can reduce the efficacy of the immunotherapy.144 Indeed, our group has transitioned from a every 6-hr dosing regimen postoperatively to less frequent dosing, often twice daily with a shorter steroid taper if tolerated by the patient.145
Pharmacologic Deep Vein Thrombosis Prophylaxis
Patients with gliomas are at an increased risk of developing venous thromboembolism (VTE) like deep vein thrombosis (DVT) and pulmonary embolism (PE), with VTE rates as high as 20–30% in some series.146 While pneumatic compression devices are safe to use and have efficacy in preventing DVTs for craniotomy patients, there are few studies providing guidance on the safety of pharmacological agents for VTE prophylaxis in the postcraniotomy setting.147 The timing for initiation of DVT prophylaxis has ranged from 1 to 7 days after a craniotomy with some groups preferring to completely avoid pharmacological DVT prophylaxis postoperatively.148 A study by Smith et al. examined the efficacy of prophylactic with either heparin or enoxaparin and found that earlier initiation on postoperative day 1 was associated with decreased incidence of VTE.149 In terms of agent selection and dosing, general oncology practice guidelines have recommended low-molecular-weight heparin (LMWH) like enoxaparin for VTE prophylaxis in patients with systemic cancer.150 However, unfractionated heparin (UH) dosed up to three times a day has been reported to be safe in several studies with glioma patients and a recent meta-analysis suggested there was an increased risk of hemorrhage with low molecular weight heparin (LMWH) compared to UH.151,152 More work is needed to define the most appropriate agents to use in the postoperative setting as well as the timing of initiation.
Postoperative Antiepileptics
The use of antiepileptic medications for seizure prevention in patients with brain tumors is somewhat controversial. Consensus statements state that there is no clear benefit for antiepileptics for patients with a brain tumor who do not have a history of seizures.153 However, many neurosurgeons give intraoperative anti-epileptic medications and continue them postoperatively to minimize risk of seizures.154 Yet, randomized trials examining the efficacy of perioperative seizure prophylaxis has failed to show a substantial benefit for seizure prevention.155
When patients are continued on antiepileptics postoperatively, the duration of treatment varies, often from 1 week to 6 months postoperatively. In a recent study by Rahman et al. with patients who underwent a craniotomy and did not have prior seizures, the overall rate of seizures was low whether AEDs were continued for 1 or 6 weeks postoperatively.156 Furthermore, there was no difference in the measurable change in cognition or mood irrespective of duration of therapy. While the agent of choice has not been standardized, many recent studies have investigated levetiracetam given its low side effect profile. In summary, while the current prospective and retrospective literature on antiepileptics for postoperative seizure control have not shown a definitive benefit to seizure control, the side effect profile for newer agents such as levetiracetam is generally favorable with a low risk of cognitive or mood side effects.
Postoperative Imaging
Following tumor resection, an MRI scan typically obtained with 48 hours to evaluate the tumor resection. This early postoperative MRI is valuable as it both shows any residual contrast enhancing or nonenhancing disease, but it also reveals any postoperative ischemic changes on DWI that may enhance on follow up imaging and be mistaken for tumor progression. Furthermore, any ischemic changes are useful for determining the etiology of postoperative neurological deficits when present. Finally, postoperative diffusion tensor imaging may be considered to determine the integrity of white matter tracts around the resection cavity.157
Rehabilitation
In the event a patient experiences a postoperative neurological deficit, particularly acute paresis, aphasia, or apraxia, a short time period in an acute rehabilitation unit may be necessary for patients to recover to the point that they can return home safely and tolerate adjuvant therapy. During this time, the patient undergoes intensive physical and occupational therapy and equipment necessarily to improve their mobility and quality of life at home can be obtained. In addition to therapy and rehabilitation, there is interest in utilizing technology such as repetitive transcranial magnetic stimulation (rTMS) to enhance plasticity and hopefully improve the speed and magnitude of recovery.158
Conclusions
In this review statement on the state-of-the-art for neurosurgical oncology, we discuss the prognostic importance of molecular characteristics in the latest diagnostic criteria for diffuse gliomas. Next, although operative approaches may vary, we define the surgical goal for diffuse gliomas and the recent evidence establishing the standard of care for low- and high-grade diffuse gliomas is a focused, open craniotomy for supramaximal resection when safe. The benefit of aggressive resections is limited by postoperative neurological deficits, highlighting the critical importance of safety when performing these surgeries. We discuss a variety of operative techniques for identifying and preserving functional regions, tracts, and networks intraoperatively and discuss why relying on preoperative data to localize function is not sufficient. We describe emerging technologies for drug delivery, tumor ablation, and adjuvant therapy that have begun to be used for patients with newly diagnosed and recurrent diffuse gliomas, although the data supporting these more novel approaches is somewhat limited. Finally, the postoperative management of patients following a craniotomy for tumor resection is essential for minimizing early post-operative complications; however, there is a general lack of consensus in the field regarding the best patient management practices. Future work is needed to improve standardization across the field of neurosurgical oncology to ensure optimal patient safety and outcomes.
Conflicts of Interest
The authors have no conflicts of interest to disclose.
Acknowledgements
The authors would like to acknowledge and thank Ken Probst for providing the illustrations included in this manuscript.