-
PDF
- Split View
-
Views
-
Cite
Cite
Rupesh Kotecha, Joseph M Kim, Jacob A Miller, Aditya Juloori, Samuel T Chao, Erin S Murphy, David M Peereboom, Alireza M Mohammadi, Gene H Barnett, Michael A Vogelbaum, Lilyana Angelov, John H Suh, Manmeet S Ahluwalia, The impact of sequencing PD-1/PD-L1 inhibitors and stereotactic radiosurgery for patients with brain metastasis, Neuro-Oncology, Volume 21, Issue 8, August 2019, Pages 1060–1068, https://doi.org/10.1093/neuonc/noz046
- Share Icon Share
Abstract
The response of brain metastases (BM) treated with stereotactic radiosurgery (SRS) and immune checkpoint inhibitors (ICIs; programmed cell death 1 and its ligand) is of significant interest.
Patients were divided into cohorts based on ICI sequencing around SRS. The primary outcome was best objective response (BOR) that was lesion specific. Secondary outcomes included overall objective response (OOR), response durability, radiation necrosis (RN), and overall survival (OS).
One hundred fifty patients underwent SRS to 1003 BM and received ICI. Five hundred sixty-four lesions (56%) treated with concurrent ICI (±5 half-lives) demonstrated superior BOR, OOR, and response durability compared with lesions treated with SRS and delayed ICI. Responses were best in those treated with immediate (±1 half-life) ICI (BOR: −100 vs −57%, P < 0.001; complete response: 50 vs 32%; 12-month durable response: 94 vs 71%, P < 0.001). Lesions pre-exposed to ICI and treated with SRS had poorer BOR (−45%) compared with ICI naive lesions (−63%, P < 0.001); best response was observed in ICI naive lesions receiving SRS and immediate ICI (−100%, P < 0.001). The 12-month cumulative incidence of RN with immediate ICI was 3.2% (95% CI: 1.3–5.0%). First radiographic follow-up and best intracranial response were significantly associated with longer OS; steroids were associated with inferior response rates and poorer OS (median 10 vs 25 mo, P = 0.002).
Sequencing of ICI around SRS is associated with overall response, best response, and response durability, with the most substantial effect in ICI naive BM undergoing immediate combined modality therapy. First intracranial response for patients treated with immediate ICI and SRS may be prognostic for OS, whereas steroids are detrimental.
High responses are achieved with SRS and immune checkpoint inhibitors in BM patients.
Immune checkpoint inhibitors should be delivered as close to SRS as possible.
Steroids are associated with lower response and poorer survival in BM patients.
Recent reports have demonstrated modest efficacy of treatment with programmed cell death 1 (PD-1) or its ligand (PD-L1) inhibitors alone for select patients with BM. To our knowledge, this report represents the largest study to date of patients treated with SRS and ICI at varying intervals, evaluating over 1000 brain metastases with multiple objective lesion-specific and patient-specific response and toxicity outcomes. We found that responses were best in immunotherapy naive patients treated with SRS and immediate PD-1 or PD-L1 inhibitors, with extremely high response rates and sustained response durability in this cohort. Furthermore, we found that these responses directly correlated with survival. An added novel finding of this study was that steroid use lessened response rates. Therefore, given the results of this study, it is important to remain cognizant of the high response rates of optimally sequenced combined modality therapy when selecting patients for treatment with systemic therapy alone.
Approximately 15–30% of cancer patients will develop brain metastases (BM), with non‒small cell lung cancer (NSCLC) as the most common primary tumor. Other malignancies with high predisposition for intracranial relapse include melanoma and renal cell carcinoma. For patients with metastatic disease from these tumor types, immune checkpoint inhibitor (ICI) blockade against programmed cell death 1 (PD-1) or its ligand (PD-L1) has become a standard treatment option in the first-line setting,1 in combination with chemotherapy2 or as a salvage treatment after failure of chemotherapy.3 Early studies demonstrated modest responses in patients with NSCLC and melanoma BM.4 Given the rapid entry of PD-1/PD-L1 inhibitors into the clinic, their sequencing along with radiotherapy is being actively investigated. Moreover, in several clinical practices, there is preference to withhold radiotherapy and initiate ICIs because of the possibility of a response, delaying radiotherapy as salvage treatment. Definitive data for or against this practice are currently limited and outcomes about combined modality therapy are needed to provide a benchmark for future comparison and trials.
We investigated the impact of timing of ICI in BM patients undergoing stereotactic radiosurgery (SRS). The hypothesis was that the addition of concurrent ICI to SRS would augment intracranial responses and that the sequencing of therapy would affect lesion response and response durability. We also hypothesized that early intracranial response (first post-SRS MRI) may be a surrogate marker of final lesion outcome and overall survival (OS).
Methods
Patient Selection and Treatment
This institutional review board–approved retrospective cohort study included consecutive BM patients from 2010–2017 who underwent SRS at a single tertiary-care institution and received ICI at some point during their disease course. Patient-specific characteristics, diagnostic information, and treatment details were abstracted from the shared medical record.
All patients underwent SRS using the Gamma Knife platform (Elekta Instruments). Patients were seen in clinic with repeat MRIs 4–8 weeks after SRS and every 2–3 months subsequently. Responses for each lesion were categorized as complete response (CR, 100% diameter reduction, referred to as −100% in the text), partial response (PR, 30–99% diameter reduction, referred to as −30 to −99% in the text), stable disease (SD, 29% diameter reduction, referred to as −29% in the text to 19% diameter increase, referred to as +19% in the text), or progressive disease (PD, ≥20% diameter increase, referred to as +≥20% in the text) according to standardized response assessment criteria for BM as proposed by the Response Assessment in Neuro-Oncology (RANO) working group, with the modification that any lesion of any size was included in this analysis and per-lesion outcomes were reported as well as per-patient outcomes.5
Systemic Therapies
All ICI therapies were recorded, including details regarding the cycles of therapy around the time of SRS. Concurrent ICI therapy with SRS was defined as the agent administered on the same day or within 5 biological half-lives of the date of SRS (either preceding or following).6,7 ICIs that were stopped more than 5 biological half-lives before SRS or initiated more than 5 biological half-lives after SRS were not considered to be concurrent. Immediate ICI therapy was defined similarly, using a stricter threshold of ±1 biological half-life from SRS. All immune checkpoint inhibitor half-lives were obtained from publicly available pharmacy databases.
Outcome Measures
The primary outcome for each lesion was the magnitude of best objective response (BOR) after SRS, relative to the pre-SRS maximum diameter. Secondary outcomes included the proportion of lesions achieving CR, PR, SD, or PD, as well as the durability of response, calculated from the date of treatment. To study change in lesion size over time, median objective response rates (ORRs) were reported at 3, 6, and 12 months after SRS (grouped within ±0.5 mo). Baseline lesion measurements were performed by the treating physician neurosurgeon and radiation oncologist team and recorded in the institutional prospective radiosurgery registry. Response assessments were obtained by review of the shared medical record, including radiology, neurosurgery, and radiation oncology reports, and all posttreatment measurements were reviewed for this study in radiation oncology.
The cumulative incidence of radiation necrosis (RN), both radiographic and symptomatic, was recorded using a previously published institutional algorithm.6,8 In brief, ring-enhancing lesions demonstrating enlargement with surrounding edema were suggestive of RN. Short-interval follow-up imaging was typically performed to distinguish PD from RN by use of the institutional algorithm. For equivocal cases, a multidisciplinary CNS tumor conference was used to achieve a clinical consensus. If a consensus could not be achieved with MR images alone, additional tests were used including positron emission tomography, MRI with cerebral blood volume, short-interval follow-up, or biopsy/resection to establish a diagnosis. Symptomatic RN was considered in patients who were deemed to have radiographic RN and had new onset or worsening neurologic signs or symptoms.
Statistical Analysis
For baseline characteristics, continuous data were compared across cohorts with Student’s t-tests or Wilcoxon rank-sum tests, while categorical data were compared with Fisher’s exact or chi-squared tests. BOR was compared across cohorts using Wilcoxon rank-sum tests. The Kaplan–Meier method was used to estimate OS. Cumulative incidences were used to estimate the time-dependent RN risk. Non-informative censoring was performed only at loss to radiographic follow-up. Death was a second competing cause. To test for differences in cumulative incidences across cohorts, Gray’s tests were utilized.9,10
Multivariable analysis for BOR was conducted on a per-lesion basis using multivariable linear regression. Models were adjusted for the following covariates: use of concurrent ICI, age, presence of extracranial metastases, number of BM, KPS, prior/concurrent whole brain radiotherapy (WBRT), prior surgery, baseline lesion volume, and prescription dose. Covariates were chosen based upon previously described risk factors for local failure, variables prognostic for survival, and use of concurrent ICI to test the research hypotheses. Covariates demonstrating association (P ≤ 0.20) with objective response or OS on univariate analysis were evaluated in a multivariable model including all 2-way interactions. To account for the interdependence of multiple lesions in a given patient, we included patient identity as a random effect in all regression models. Multivariable model quality was evaluated using the Akaike information criterion. Analyses were conducted using the R statistical software package including the cmprsk package (R Core Team, 2015). Two-sided tests with P < 0.05 were considered statistically significant.
Results
Patient and Treatment Characteristics
Within the study period, 150 patients underwent SRS to 1003 BM and received ICIs at varying time intervals (Table 1). Of the 1003 BM treated with SRS, 564 (56%) were treated with concurrent ICIs, of which a subset of these (367 lesions, 37%) were treated with immediate ICIs (Supplementary Tables 1 and 2). Among patients who received concurrent ICIs, the mean cumulative dose of dexamethasone was 37 mg (interquartile rate: 0–42; R: 0–276) and 54% of patients did not receive any corticosteroids.
Characteristic . | All Patients . |
---|---|
No. patients | 150 |
Primary pathology | |
NSCLC–adenocarcinoma | 80 |
Melanoma | 25 |
Renal | 18 |
NSCLC–squamous | 12 |
NSCLC–other* | 7 |
Breast | 2 |
Bladder | 1 |
Endometrial | 1 |
Esophageal | 1 |
Head and neck | 1 |
Prostate | 1 |
Sarcoma | 1 |
Female | 76 (51) |
Age | 61 [24–87] |
<50 | 20 (13) |
50–59 | 46 (31) |
≥60 | 84 (56) |
Extracranial metastasis | 86 (57) |
Karnofsky performance status | 80 [40–100] |
<70 | 7 (5) |
70–80 | 75 (50) |
90–100 | 68 (45) |
Number of BM | 2 [1–15] |
1 | 63 (42) |
2–3 | 37 (25) |
>3 | 50 (33) |
Upfront intracranial therapy | |
WBRT | 12 (8) |
SRS | 145 (97) |
Surgery | 28 (19) |
Immunotherapy (half-life, days)** | |
Nivolumab (25) | 119 (79) |
Pembrolizumab (22) | 22 (15) |
Atezolizumab (27) | 10 (7) |
Avelumab (6.1) | 2 (1) |
Characteristic . | All Patients . |
---|---|
No. patients | 150 |
Primary pathology | |
NSCLC–adenocarcinoma | 80 |
Melanoma | 25 |
Renal | 18 |
NSCLC–squamous | 12 |
NSCLC–other* | 7 |
Breast | 2 |
Bladder | 1 |
Endometrial | 1 |
Esophageal | 1 |
Head and neck | 1 |
Prostate | 1 |
Sarcoma | 1 |
Female | 76 (51) |
Age | 61 [24–87] |
<50 | 20 (13) |
50–59 | 46 (31) |
≥60 | 84 (56) |
Extracranial metastasis | 86 (57) |
Karnofsky performance status | 80 [40–100] |
<70 | 7 (5) |
70–80 | 75 (50) |
90–100 | 68 (45) |
Number of BM | 2 [1–15] |
1 | 63 (42) |
2–3 | 37 (25) |
>3 | 50 (33) |
Upfront intracranial therapy | |
WBRT | 12 (8) |
SRS | 145 (97) |
Surgery | 28 (19) |
Immunotherapy (half-life, days)** | |
Nivolumab (25) | 119 (79) |
Pembrolizumab (22) | 22 (15) |
Atezolizumab (27) | 10 (7) |
Avelumab (6.1) | 2 (1) |
Values presented as number (percent) or median [range].
*Large cell, NOS, mixed histology.
**Publicly available half-life data obtained from the FDA drug labels (https://www.accessdata.fda.gov/).
Characteristic . | All Patients . |
---|---|
No. patients | 150 |
Primary pathology | |
NSCLC–adenocarcinoma | 80 |
Melanoma | 25 |
Renal | 18 |
NSCLC–squamous | 12 |
NSCLC–other* | 7 |
Breast | 2 |
Bladder | 1 |
Endometrial | 1 |
Esophageal | 1 |
Head and neck | 1 |
Prostate | 1 |
Sarcoma | 1 |
Female | 76 (51) |
Age | 61 [24–87] |
<50 | 20 (13) |
50–59 | 46 (31) |
≥60 | 84 (56) |
Extracranial metastasis | 86 (57) |
Karnofsky performance status | 80 [40–100] |
<70 | 7 (5) |
70–80 | 75 (50) |
90–100 | 68 (45) |
Number of BM | 2 [1–15] |
1 | 63 (42) |
2–3 | 37 (25) |
>3 | 50 (33) |
Upfront intracranial therapy | |
WBRT | 12 (8) |
SRS | 145 (97) |
Surgery | 28 (19) |
Immunotherapy (half-life, days)** | |
Nivolumab (25) | 119 (79) |
Pembrolizumab (22) | 22 (15) |
Atezolizumab (27) | 10 (7) |
Avelumab (6.1) | 2 (1) |
Characteristic . | All Patients . |
---|---|
No. patients | 150 |
Primary pathology | |
NSCLC–adenocarcinoma | 80 |
Melanoma | 25 |
Renal | 18 |
NSCLC–squamous | 12 |
NSCLC–other* | 7 |
Breast | 2 |
Bladder | 1 |
Endometrial | 1 |
Esophageal | 1 |
Head and neck | 1 |
Prostate | 1 |
Sarcoma | 1 |
Female | 76 (51) |
Age | 61 [24–87] |
<50 | 20 (13) |
50–59 | 46 (31) |
≥60 | 84 (56) |
Extracranial metastasis | 86 (57) |
Karnofsky performance status | 80 [40–100] |
<70 | 7 (5) |
70–80 | 75 (50) |
90–100 | 68 (45) |
Number of BM | 2 [1–15] |
1 | 63 (42) |
2–3 | 37 (25) |
>3 | 50 (33) |
Upfront intracranial therapy | |
WBRT | 12 (8) |
SRS | 145 (97) |
Surgery | 28 (19) |
Immunotherapy (half-life, days)** | |
Nivolumab (25) | 119 (79) |
Pembrolizumab (22) | 22 (15) |
Atezolizumab (27) | 10 (7) |
Avelumab (6.1) | 2 (1) |
Values presented as number (percent) or median [range].
*Large cell, NOS, mixed histology.
**Publicly available half-life data obtained from the FDA drug labels (https://www.accessdata.fda.gov/).
Overall Response and Durability of Response
A total of 1073 MRIs were reviewed (355 baseline for the 1003 lesions and 718 follow-up images), resulting in a total of 5508 measurements (average of 5 follow-up measurements per lesion, range: 1–25). The primary outcome, BOR, was superior in the concurrent (inclusive of immediate) ICI group (median −67% vs −57%, P = 0.014) (see Table 2, Fig. 1). In fact, the median objective responses at 3, 6, and 12 months after SRS and concurrent ICI were CRs at all time endpoints (−100% or disappearance of the treated lesion) compared with only −63% at 3 months and −75% at 6 and 12 months for lesions treated with SRS alone (P < 0.001 for all comparisons). Since we had the unusual outcome of a −100% median value at the specified timepoints, we also computed other average statistics; the mean 3, 6, and 12 month objective responses were −74%, −82%, and −86% versus −62%, −66%, and −65%, for concurrent vs nonconcurrent ICI. Secondary outcomes were also superior in those treated with concurrent SRS + ICI versus SRS alone, with a greater number of overall CRs (42% vs 33%, P < 0.05) and higher proportions of sustained responses at 3 (82% vs 75%, P = 0.012), 6 (85% vs 74%, P = 0.002), and 12 months (86% vs 72%, P = 0.005), respectively.
Characteristic . | All Lesions . | SRS + Concurrent PD-1/ PD-L1 Inhibitor . | SRS Alone . | P-value . |
---|---|---|---|---|
No. lesions | 1003 | 564 (56) | 439 (44) | |
Best objective response (median [range]) | −61% [−100%, +164%] | −67% [−100%, +129%] | −57% [−100%, +164%] | 0.014 |
Lesions ≥3 mo follow-up | −80% [−100%, +164%] | − 100% [−100%, +129%] | −63% [−100, +164%] | <0.0001 |
Lesions ≥6 mo follow-up | −100% [−100%, +164%] | − 100% [−100%, +100%] | −75% [−100, +164%] | <0.0001 |
Lesions ≥12 mo follow-up | −100% [−100%, +164%] | − 100% [−100%, +100%] | −75% [−100, +164%] | <0.0001 |
Objective response categories | 0.042 | |||
Complete response | 385 (38) | 348 (42) | 147 (33) | |
Partial response | 349 (35) | 181 (32) | 168 (38) | |
Stable disease | 175 (18) | 94 (17) | 81 (19) | |
Progressive disease | 94 (9) | 51 (9) | 43 (10) | |
Durable response (CR or PR) ≥3 mo* | 758 (76) | 329 (82) | 266 (75) | 0.012 |
Durable response (CR or PR) ≥6 mo** | 505 (51) | 206 (85) | 194 (74) | 0.002 |
Durable response (CR or PR) ≥12 mo*** | 293 (29) | 96 (86) | 130 (72) | 0.005 |
Characteristic . | All Lesions . | SRS + Concurrent PD-1/ PD-L1 Inhibitor . | SRS Alone . | P-value . |
---|---|---|---|---|
No. lesions | 1003 | 564 (56) | 439 (44) | |
Best objective response (median [range]) | −61% [−100%, +164%] | −67% [−100%, +129%] | −57% [−100%, +164%] | 0.014 |
Lesions ≥3 mo follow-up | −80% [−100%, +164%] | − 100% [−100%, +129%] | −63% [−100, +164%] | <0.0001 |
Lesions ≥6 mo follow-up | −100% [−100%, +164%] | − 100% [−100%, +100%] | −75% [−100, +164%] | <0.0001 |
Lesions ≥12 mo follow-up | −100% [−100%, +164%] | − 100% [−100%, +100%] | −75% [−100, +164%] | <0.0001 |
Objective response categories | 0.042 | |||
Complete response | 385 (38) | 348 (42) | 147 (33) | |
Partial response | 349 (35) | 181 (32) | 168 (38) | |
Stable disease | 175 (18) | 94 (17) | 81 (19) | |
Progressive disease | 94 (9) | 51 (9) | 43 (10) | |
Durable response (CR or PR) ≥3 mo* | 758 (76) | 329 (82) | 266 (75) | 0.012 |
Durable response (CR or PR) ≥6 mo** | 505 (51) | 206 (85) | 194 (74) | 0.002 |
Durable response (CR or PR) ≥12 mo*** | 293 (29) | 96 (86) | 130 (72) | 0.005 |
Values are presented as percent change [range] or as number (percent).
*Among lesions with at least 3 months of radiographic follow-up.
**Among lesions with at least 6 months of radiographic follow-up.
**Among lesions with at least 12 months of radiographic follow-up.
Characteristic . | All Lesions . | SRS + Concurrent PD-1/ PD-L1 Inhibitor . | SRS Alone . | P-value . |
---|---|---|---|---|
No. lesions | 1003 | 564 (56) | 439 (44) | |
Best objective response (median [range]) | −61% [−100%, +164%] | −67% [−100%, +129%] | −57% [−100%, +164%] | 0.014 |
Lesions ≥3 mo follow-up | −80% [−100%, +164%] | − 100% [−100%, +129%] | −63% [−100, +164%] | <0.0001 |
Lesions ≥6 mo follow-up | −100% [−100%, +164%] | − 100% [−100%, +100%] | −75% [−100, +164%] | <0.0001 |
Lesions ≥12 mo follow-up | −100% [−100%, +164%] | − 100% [−100%, +100%] | −75% [−100, +164%] | <0.0001 |
Objective response categories | 0.042 | |||
Complete response | 385 (38) | 348 (42) | 147 (33) | |
Partial response | 349 (35) | 181 (32) | 168 (38) | |
Stable disease | 175 (18) | 94 (17) | 81 (19) | |
Progressive disease | 94 (9) | 51 (9) | 43 (10) | |
Durable response (CR or PR) ≥3 mo* | 758 (76) | 329 (82) | 266 (75) | 0.012 |
Durable response (CR or PR) ≥6 mo** | 505 (51) | 206 (85) | 194 (74) | 0.002 |
Durable response (CR or PR) ≥12 mo*** | 293 (29) | 96 (86) | 130 (72) | 0.005 |
Characteristic . | All Lesions . | SRS + Concurrent PD-1/ PD-L1 Inhibitor . | SRS Alone . | P-value . |
---|---|---|---|---|
No. lesions | 1003 | 564 (56) | 439 (44) | |
Best objective response (median [range]) | −61% [−100%, +164%] | −67% [−100%, +129%] | −57% [−100%, +164%] | 0.014 |
Lesions ≥3 mo follow-up | −80% [−100%, +164%] | − 100% [−100%, +129%] | −63% [−100, +164%] | <0.0001 |
Lesions ≥6 mo follow-up | −100% [−100%, +164%] | − 100% [−100%, +100%] | −75% [−100, +164%] | <0.0001 |
Lesions ≥12 mo follow-up | −100% [−100%, +164%] | − 100% [−100%, +100%] | −75% [−100, +164%] | <0.0001 |
Objective response categories | 0.042 | |||
Complete response | 385 (38) | 348 (42) | 147 (33) | |
Partial response | 349 (35) | 181 (32) | 168 (38) | |
Stable disease | 175 (18) | 94 (17) | 81 (19) | |
Progressive disease | 94 (9) | 51 (9) | 43 (10) | |
Durable response (CR or PR) ≥3 mo* | 758 (76) | 329 (82) | 266 (75) | 0.012 |
Durable response (CR or PR) ≥6 mo** | 505 (51) | 206 (85) | 194 (74) | 0.002 |
Durable response (CR or PR) ≥12 mo*** | 293 (29) | 96 (86) | 130 (72) | 0.005 |
Values are presented as percent change [range] or as number (percent).
*Among lesions with at least 3 months of radiographic follow-up.
**Among lesions with at least 6 months of radiographic follow-up.
**Among lesions with at least 12 months of radiographic follow-up.
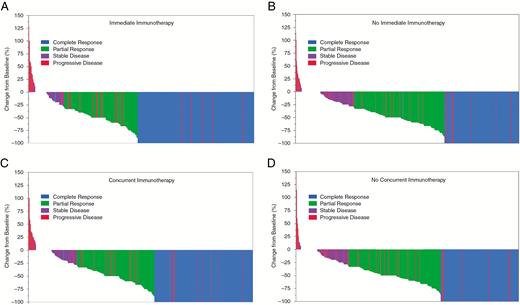
Waterfall plots of best objective response after treatment of 1003 brain metastases with (A) immediate immunotherapy, (B) no immediate immunotherapy, (C) concurrent immunotherapy, and (D) no concurrent immunotherapy. Colors indicate overall objective response after full radiographic follow-up.
The primary and secondary response outcomes were even more pronounced among the immediate ICI subset (compared with those not treated with immediate ICI), including BOR (−100% vs −57%, P < 0.001), higher rate of CR (50% vs 32%, P < 0.001), as well as the durable responses at 6 months (89% vs 74%, P < 0.001) and 12 months (94% vs 71%, P < 0.001). Among lesions with 12 months of follow-up (293 lesions, 29%), those treated with immediate ICI had markedly improved CR rates (85% vs 43%, P < 0.001) and significantly reduced rates of PD (4% vs 19%, P < 0.001). Among patients who received any corticosteroids with concurrent ICI during the week of SRS, median BOR trended inferior (67% vs 83%, P = 0.116), with higher rates of local failure (10% vs 4%, P = 0.002). This remained independently predictive of inferior BOR on multivariate linear regression (−14.97%, P < 0.001). The multivariate-adjusted probability of CR was 31% vs 48% among patients receiving any versus no steroids (P < 0.001). When all other key variables were kept at the mean (including volume, classic graded assessment prognosticators, PD, heterogeneity index, etc.), the predicted probabilities of PD were 12%, 23%, and 18% with 0, 1–60, and >60 mg (P < 0.0004); the predicted probabilities of CR were 48%, 35%, and 23% with the same levels; and CR + PR were 85%, 62%, and 65%. When comparing patients receiving immediate versus nonconcurrent (ie, eliminating the non-immediate but concurrent cohort from the above comparison), the resulting differences were even more striking, with a median BOR of −100% versus −57% (P < 0.001) and average response of −69% versus −59% (P < 0.001).
Multivariable linear regression was performed to adjust for differences in confounding covariates for BOR (Table 3). After adjustment, concurrent ICI was associated with a 5.95% adjusted improvement (P = 0.040), whereas immediate ICI was associated with a 10.55% improvement (P < 0.001). In addition to the timing of ICI, patient KPS, receipt of prior WBRT, lesion volume, and prescription dose were also significantly associated with best response.
Covariate . | Best Objective Response . | |||
---|---|---|---|---|
Univariate . | Multivariate . | . | . | |
P-value . | Est. . | 95% CI . | P-value . | |
Concurrent immunotherapy | 0.014 | −5.95% | −8.92%, −1.04% | 0.040 |
Age | 0.518 | − | − | − |
Female | 0.010 | |||
Extracranial metastasis | 0.993 | − | − | − |
Karnofsky performance status* | 0.034 | −2.99% | −5.80%, −0.17% | 0.038 |
Number of BM | 0.627 | − | − | − |
Prior WBRT | 0.019 | 16.12% | 7.49%, 24.76% | <0.001 |
Prior surgery | 0.003 | −6.01% | −13.67%, +1.65% | 0.124 |
Volume (cc)** | <0.001 | 1.73% | 1.03%, 2.43% | <0.001 |
Prescription dose (Gy) | <0.001 | −1.91% | −2.95%, −0.87% | <0.001 |
Conformality index | 0.100 | −0.46% | −2.48%, 1.55% | 0.652 |
Heterogeneity index | <0.001 | 2.72 | −2.10%, 7.54% | 0.270 |
Gradient index | <0.001 | −0.65% | −1.57%, 0.26% | 0.161 |
Covariate . | Best Objective Response . | |||
---|---|---|---|---|
Univariate . | Multivariate . | . | . | |
P-value . | Est. . | 95% CI . | P-value . | |
Concurrent immunotherapy | 0.014 | −5.95% | −8.92%, −1.04% | 0.040 |
Age | 0.518 | − | − | − |
Female | 0.010 | |||
Extracranial metastasis | 0.993 | − | − | − |
Karnofsky performance status* | 0.034 | −2.99% | −5.80%, −0.17% | 0.038 |
Number of BM | 0.627 | − | − | − |
Prior WBRT | 0.019 | 16.12% | 7.49%, 24.76% | <0.001 |
Prior surgery | 0.003 | −6.01% | −13.67%, +1.65% | 0.124 |
Volume (cc)** | <0.001 | 1.73% | 1.03%, 2.43% | <0.001 |
Prescription dose (Gy) | <0.001 | −1.91% | −2.95%, −0.87% | <0.001 |
Conformality index | 0.100 | −0.46% | −2.48%, 1.55% | 0.652 |
Heterogeneity index | <0.001 | 2.72 | −2.10%, 7.54% | 0.270 |
Gradient index | <0.001 | −0.65% | −1.57%, 0.26% | 0.161 |
Est., parameter estimate; [ref], reference.
*Per 10 unit increase.
**Per 1 cc increase.
Covariate . | Best Objective Response . | |||
---|---|---|---|---|
Univariate . | Multivariate . | . | . | |
P-value . | Est. . | 95% CI . | P-value . | |
Concurrent immunotherapy | 0.014 | −5.95% | −8.92%, −1.04% | 0.040 |
Age | 0.518 | − | − | − |
Female | 0.010 | |||
Extracranial metastasis | 0.993 | − | − | − |
Karnofsky performance status* | 0.034 | −2.99% | −5.80%, −0.17% | 0.038 |
Number of BM | 0.627 | − | − | − |
Prior WBRT | 0.019 | 16.12% | 7.49%, 24.76% | <0.001 |
Prior surgery | 0.003 | −6.01% | −13.67%, +1.65% | 0.124 |
Volume (cc)** | <0.001 | 1.73% | 1.03%, 2.43% | <0.001 |
Prescription dose (Gy) | <0.001 | −1.91% | −2.95%, −0.87% | <0.001 |
Conformality index | 0.100 | −0.46% | −2.48%, 1.55% | 0.652 |
Heterogeneity index | <0.001 | 2.72 | −2.10%, 7.54% | 0.270 |
Gradient index | <0.001 | −0.65% | −1.57%, 0.26% | 0.161 |
Covariate . | Best Objective Response . | |||
---|---|---|---|---|
Univariate . | Multivariate . | . | . | |
P-value . | Est. . | 95% CI . | P-value . | |
Concurrent immunotherapy | 0.014 | −5.95% | −8.92%, −1.04% | 0.040 |
Age | 0.518 | − | − | − |
Female | 0.010 | |||
Extracranial metastasis | 0.993 | − | − | − |
Karnofsky performance status* | 0.034 | −2.99% | −5.80%, −0.17% | 0.038 |
Number of BM | 0.627 | − | − | − |
Prior WBRT | 0.019 | 16.12% | 7.49%, 24.76% | <0.001 |
Prior surgery | 0.003 | −6.01% | −13.67%, +1.65% | 0.124 |
Volume (cc)** | <0.001 | 1.73% | 1.03%, 2.43% | <0.001 |
Prescription dose (Gy) | <0.001 | −1.91% | −2.95%, −0.87% | <0.001 |
Conformality index | 0.100 | −0.46% | −2.48%, 1.55% | 0.652 |
Heterogeneity index | <0.001 | 2.72 | −2.10%, 7.54% | 0.270 |
Gradient index | <0.001 | −0.65% | −1.57%, 0.26% | 0.161 |
Est., parameter estimate; [ref], reference.
*Per 10 unit increase.
**Per 1 cc increase.
Timing of Systemic Therapy
Given the variation in practice on the timing of ICI around SRS, its effect on response rate was also investigated on a per-lesion basis. The efficacy of combined modality therapy was evaluated in 2 cohorts of lesions for ease of analysis: lesions in patients who previously received ICI due to systemic disease progression (ICI pre-exposed) and those who received ICI only following BM diagnosis (ICI naive). ICI pre-exposed lesions had a lower response rate compared with ICI naive lesions (45% vs 63% lesion reduction, n = 196 vs 553, P < 0.001). The best response rates were observed in ICI naive lesions that underwent treatment with SRS and immediate ICI (n = 83, −100%, CR, P < 0.001). Furthermore, when evaluating those lesions treated with immediate ICI, there was no additional benefit of immediate neoadjuvant ICI (both −100%, n = 284 vs 83, P = 0.52).
The timing of therapy did not adversely affect the risk of treatment-related toxicity. The lesion-specific 12-month cumulative incidence of radiographic RN was 3.5% (95% CI: 2.3–4.7%) and 3.2% (95% CI: 1.3–5.0%) for those treated with immediate ICI. Of the cases of radiographic RN, 7 patients in this series (5% of the entire patient cohort) developed symptomatic RN, none of which required resection.
Overall Response and Survival
Median OS was 30 months (95% CI: 24–38 mo). On a per-patient basis, the overall best intracranial response rate was 51% regardless of timing of ICI. Among all patients, 25 (17%) had an intracranial CR while 90 (60%) had a PR, using the standard RANO-BM criteria (vs per-lesion outcomes reported above). Patients who received immediate ICI had superior best intracranial response rates compared with those who did not receive immediate therapy (71% vs 53%, P = 0.008); in contrast, this difference was not observed in patients who received concurrent ICI versus those who did not (59% vs 56%, P = 0.34).
Given the high intracranial responses with combined SRS and ICI, we further investigated the role of first follow-up response and best intracranial objective response as prognostic factors for OS. First radiographic follow-up was performed typically 4–8 weeks from SRS (95% CI: 2.1–9.4 wk) and an intracranial CR at first follow-up was also associated with extended median survival (32 mo [CR] vs 29 mo [SD/PD], P = 0.012). Patients who achieved an intracranial CR during their clinical course had a significantly extended median survival compared with all other patients (not reached [CR] vs 27 mo [PR] vs 25 mo [SD/PD], P = 0.044; Fig. 2). Patients treated with concurrent ICI therapy who achieved an intracranial CR had a 100% 12-month survival. These also translated into a reduction in salvage therapies; the median intracranial response rate among patients who required salvage SRS or WBRT was 34%, compared with 81% among those who required no salvage irradiation (P < 0.001). Moreover, for patients who received SRS and concurrent ICI, the median survival was markedly better with avoidance of all steroids during the time of treatment (0 mg dexamethasone: 25.1 mo vs ≤60 mg dexamethasone: 10.2 mo vs >60 mg dexamethasone: 10.2 mo, P = 0.002) (Fig. 3).
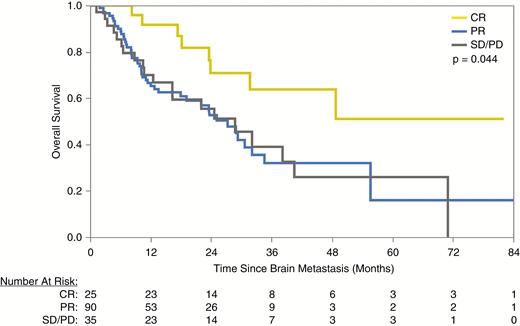
Overall survival after first brain metastasis among patients who achieved an intracranial CR, PR, or either SD or PD after SRS.
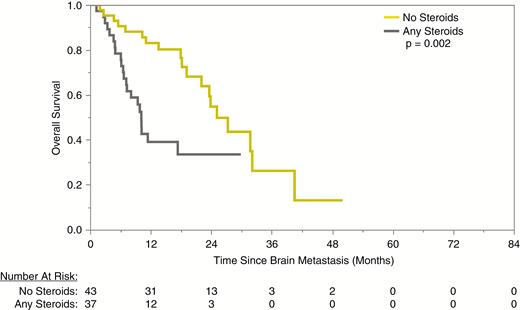
Overall survival after first BM among patients who received any versus no corticosteroids in combination with SRS and concurrent immunotherapy.
Discussion
In this study, we evaluated the outcomes of 150 patients who received ICIs and SRS to 1003 BM, representing one of the most comprehensive analyses to date. The response endpoints were based on over 5000 measurements, allowing for lesion-specific outcomes to be carefully analyzed. This study provides several important findings to guide current clinical practice as well as inform clinical trial design. First, when added to SRS, concurrent ICI therapy is associated with a higher rate of best ORR, with complete disappearance on follow-up imaging for many treated metastases. When measured with time-dependent endpoints, concurrent ICI therapy is also associated with an earlier maximal response. Second, concurrent ICI adds to the durability of local control that SRS provides, which is especially important in the face of extended survival with these therapies (median of 30 mo in this cohort of patients pretreated with chemotherapy) and translates to a significant reduction in need for salvage therapies. Third, concurrent ICI yields higher CR rates as well as lower PD rates (vs simply stabilizing disease). Fourth, regarding sequencing of therapy, the benefit of combined modality therapy is maximized when ICI naive patients receive SRS and immediate ICI. Fifth, immediate ICI improves intracranial outcomes with an acceptably low risk of RN. Sixth, for patients undergoing combined modality therapy, steroid use is associated with lower response rate and poorer survival. Finally, not only is the high intracranial response rate in patients undergoing SRS and immediate ICI impressive, early evaluation of this response is predictive of final ORR and OS. Together, these results are hypothesis generating for the next generation of future prospective analyses and clinical trials.
Although the influence of timing of anti–cytotoxic T lymphocyte antigen 4 ICI and SRS on treatment outcome has been reported in a number of institutional studies,11,12 few reports have strictly investigated timing of anti–PD-1/PDL-1 ICI with SRS. In a similar study, Schapira and colleagues reported on improved local control (using a definition of stable or decreasing lesion size) and OS in 37 patients treated for 85 BM with SRS and concurrent (defined as within 1 mo) anti–PD-1 ICI.13 Our report confirms their findings in a larger sample size of patients and lesions, provides multiple clear lesion-specific objective response criteria (including objective response type, overall response, and response durability), as well as evaluates multiple agents. Therefore, the high response rates with SRS and immediate ICI in this study provide additional robust data, and underscore the clinical value of the synergistic benefit of both modalities.
Although cross-study comparisons are difficult to interpret, it is important to assess the baseline intracranial response rates of ICI alone versus ICI with SRS to determine the optimal sequencing. Pembrolizumab has showed modest response rates in melanoma (22%) and NSCLC BM (33%).4 Nivolumab resulted in a 20% response rate in melanoma BM, though this was in a cohort of only 27 patients, and the CR rate was only 12%.14 Atezolizumab data are limited to a pooled analysis which included 27 BM patients (23 of whom received radiotherapy) but did not present any data on efficacy,15 and other studies have limited enrollment to patients without active BM (had to undergo radiotherapy prior to study entry)16 or who had asymptomatic disease. Most recently, the combination of nivolumab and ipilimumab demonstrated a CR rate of 26% and PR of 30% in asymptomatic melanoma BM (10% prior SRS).17 Comparatively, combined SRS and ICI in our study demonstrated similarly defined response rates of 81% and 90% with concurrent and immediate ICI, respectively, which compare favorably with those observed with systemic therapy alone without significant adverse effect. With increasing intracranial efficacy of multiple ICIs used simultaneously, prospective clinical trials are clearly needed to compare efficacies and toxicities of each combination to determine the best upfront intracranial therapy.
One unique finding from this study was the negative impact of corticosteroids in patients receiving concurrent ICI with SRS. Traditionally, corticosteroids are prescribed at diagnosis of symptomatic BM as well as following high dose SRS to limit acute treatment-related toxicities. Perilesional edema,12,18 treatment-related imaging changes,19 or other generalized SRS-induced acute toxicities13 have been described in various institutional reports of SRS and ICIs, leading many to use extended corticosteroid tapers. In this study, a variety of corticosteroid courses were prescribed around the time of SRS per physician discretion (and statistically unrelated to disease burden) and when quantified, cumulative corticosteroid dose was associated with inferior lesion-specific response as well as poorer survival, specifically in patients receiving concurrent ICIs. A phase II trial of ipilimumab also suggested reduced disease control rates in BM patients on corticosteroids compared with those not on corticosteroids,20 and most recently, a review of NSCLC patients treated across 2 institutions with PD-1 inhibitors demonstrated reduced response rates as well as reductions in progression-free survival and OS in patients on high-dose baseline corticosteroids.21 Given the array of negative systemic side effects and the potential for decreased response to immune checkpoint blockade, we recommend judicious corticosteroid use, especially in those receiving combined modality therapy. We await further prospective trials evaluating the effects of steroid administration on the risk of disease progression and survival in patients receiving ICI and SRS.
One concern with the use of immediate ICI around SRS is the potential for increased RN risk. This is not easily diagnosed or published upon frequently and only a few retrospective studies thus far have evaluated this in patients receiving ICI,12,19,22,23 none of which clearly delineated timing parameters as was performed in this study. A similar number of studies have reported an increased risk12,19,22 as those that have not observed this effect,24–26 and when averaged across studies, there does appear to be a significant risk.27 In the current study, we carefully evaluated over 1000 metastases to clarify this issue and report RN risk using a multidisciplinary standardized algorithm and review process.6,8 We attribute the low risk in this series (<5%) to the use of PD-1/PDL-1 inhibitors in this study (vs anti–cytotoxic T lymphocyte antigen 4 ICI)11 and institutional SRS dosing strategies28; however, an in-depth analysis of additional parameters is currently under way.
As this study is retrospective, several limitations must be considered. First, this is a single institutional review and therefore may have limited the generalizability as the results are potentially subject to institutional treatment biases of key variables, including the availability and choice of ICI therapies, radiosurgery dosing strategies, etc. Moreover, differences across patients in important variables such as presence of extracranial metastasis, previous use of ICI because of systemic and/or intracranial disease, or timing of BM occurrence within the patient’s overall disease course are difficult to account for in covariate modeling. Patients in this study received corticosteroids and ICIs at varying timepoints from their diagnosis and treatment, in a nonrandomized fashion, and therefore sequencing conclusions are hypothesis generating and require prospective validation. On the other hand, the lesion-based measurement analysis made through serial follow-up for a large number of patients and lesions adds considerable weight to our findings and contributes to the literature on this topic.
Given the increasing complexity of managing patients with BM in the current era of widespread use of ICI and SRS, there is a clear need for multidisciplinary clinics and multidisciplinary tumor conferences to ensure optimal patient management. The decisions regarding sequencing of therapies, selection of patients for single versus combined modality therapy, need for steroid administration, and overall optimal systemic disease management cannot be performed by individual subspecialists making independent decisions. Therefore, at our institution, BM patients are seen in multidisciplinary clinic visits and formal twice-weekly CNS consensus conferences. Open communication between providers of a patient’s treatment team as well as open and thorough discussion with the patient are used to personalize therapy decisions. Finally, at our institution, we encourage enrollment onto clinical trials that prospectively evaluate the outcomes of BM patients treated with SRS and systemic therapy. Future prospective studies are clearly needed to clarify the optimal sequencing of therapies and evaluate potential for SRS or systemic therapy dose adjustments, and future translational studies are needed to determine the cellular and biological mechanisms underlying these exciting clinical results.
Funding
This research received no specific grant from any funding agency in the public, commercial, or not-for-profit sector.
Conflict of interest statement. R. Kotecha: Chrysalis BioTherapeutics Inc, Elsevier PracticeUpdate, Accuray Inc, Elekta AB. S. T. Chao: honorarium, Varian Medical Systems. A. M. Mohammadi: consultant for Monteris Medical Inc. G. H. Barnett: consultant for Monteris Medical Inc, royalty interests for Mako Surgical Corp, Roche. M. A. Vogelbaum: fiduciary role, equity, royalty interests in Infuseon Therapeutics Inc. J. H. Suh: Chrysalis BioTherapeutics Inc. M. Ahluwalia: consultant for Monteris Medical Inc, AstraZeneca, BMS, Kadmon Pharmaceuticals, CBT Pharmaceuticals, Abbvie, Flatiron, Varian Medical Systems, VBI Vaccines, Elsevier PracticeUpdate. Stocks: Mimivax, Doctible.
Authorship statement: R. Kotecha: study conception and design, analysis and interpretation of data, drafting of manuscript, critical revision. J. M. Kim: analysis and interpretation of data, critical revision. J. A. Miller: analysis and interpretation of data, drafting of manuscript, critical revision. A. Juloori: drafting of manuscript, critical revision. S. T. Chao: critical revision. E. S. Murphy: critical revision. D. M. Peereboom: critical revision. A. M. Mohammadi: critical revision. G. H. Barnett: critical revision. M. A. Vogelbaum: critical revision. L. Angelov: critical revision. J. H. Suh: critical revision. M. Ahluwalia: study conception and design, drafting of manuscript, critical revision.
Presented in part at the 2018 ASTRO Annual Meeting in San Antonio, Texas, October 21–24, 2018.