-
PDF
- Split View
-
Views
-
Cite
Cite
Benjamin M Ellingson, Patrick Y Wen, Timothy F Cloughesy, Evidence and context of use for contrast enhancement as a surrogate of disease burden and treatment response in malignant glioma, Neuro-Oncology, Volume 20, Issue 4, April 2018, Pages 457–471, https://doi.org/10.1093/neuonc/nox193
- Share Icon Share
Abstract
The use of contrast enhancement within the brain on CT or MRI has been the gold standard for diagnosis and therapeutic response assessment in malignant gliomas for decades. The use of contrast enhancing tumor size, however, remains controversial as a tool for accurately diagnosing and assessing treatment efficacy in malignant gliomas, particularly in the current, quickly evolving therapeutic landscape. The current article consolidates overwhelming evidence from hundreds of studies in the field of neuro-oncology, providing the necessary evidence base and specific contexts of use for consideration of contrast enhancing tumor size as an appropriate surrogate biomarker for disease burden and as a tool for measuring treatment response in malignant glioma, including glioblastoma.
A major barrier in the qualification of imaging biomarkers for use in diagnosis and predicting outcomes in clinical trials remains the lack of evidentiary standards to support the specific contexts of use for these biomarkers. The detailed challenges associated with these barriers were discussed at an in-depth workshop sponsored by Dr Anna Barker (Arizona State University) and the National Biomarker Development Alliance, in collaboration with FDA leadership and experts in the field of imaging and biomarker development. The current article describes the comprehensive historic evidence from the field of neuro-oncology, providing the necessary evidence base and specific contexts of use for consideration of contrast enhancing tumor size as a surrogate imaging biomarker for disease burden and as a tool for measuring treatment response in malignant glioma, including glioblastoma (GBM), using “Framework for Defining Evidentiary Criteria for Biomarker Qualification,” which describes a general framework for proposed evidentiary criteria for biomarker qualification and their specific contexts of use (https://fnih.org/sites/default/files/final/pdf/Evidentiary%20Criteria%20Framework%20Final%20Version%20Oct%2020%202016.pdf).
A Brief History of Brain Tumor Imaging Technology
In 1884, Rickman J. Godlee and Dr A. Hughes Bennett performed the first recognized resection of a primary, intracranial glioma.1 This surgery occurred more than 10 years prior to the discovery of any technology that could non-invasively identify tumors within the brain of living humans. In 1895, the X-ray was discovered by Wilhelm Roentgen, which for the first time provided the means of visualizing masses within the brain. In the early twentieth century, X-rays were used extensively to visualize and localize brain tumors,2 although the lack of contrast between normal and malignant brain tissue significantly limited the use of X-ray for diagnosis of gliomas.3 By 1950–1960, the use of cerebral angiography (iodine contrast combined with X-ray films) was the standard for differential diagnosis and serial follow-up evaluations4 of brain tumors, becoming routine in both academic institutions as well as small community hospitals.5 In 1959, the use of cerebral angiography was declared the imaging method of choice over all other techniques (eg, pneumoencephalography, ventriculograms) for suspected brain tumors.6
It was not until the advent of computed tomography (CT) by Sir Godfrey Hounsfield in 1971 that cerebral angiography was replaced with CT as the modality of choice for clinical diagnosis and monitoring of brain tumors. In 1980, a seminal study from the National Cancer Institute declared contrast enhanced CT the new clinical standard for brain tumor diagnosis and clinical monitoring after it was declared to be the best accurate diagnostic test in over 1000 patients with brain tumors,7 as was also suggested by other studies.8,9 Thus began the era of contrast enhanced CT as the new clinical standard for brain tumor diagnosis and clinical monitoring.
Although contrast enhanced CT was the standard for brain tumor imaging, a new technology was on the horizon that was set to revolutionize the field of medical imaging: magnetic resonance imaging (MRI). In 1971, Raymond Damadian first reported that nuclear magnetic resonance characteristics were different between normal and tumor tissues.10 In 1973, Paul C. Lauterbur created the first MR images in the mouse,11 and by 1977 Peter Mansfield created the first MR images in the human.12 In 1984, the first use of gadopentetate dimeglumine (Gd-DTPA) as a contrast agent for detection of primary intra-axial tumors was documented in Europe,13 followed by subsequent studies in 1985 substantiating these findings.14,15 By the late 1980s to early 1990s several studies demonstrated the ability for gadolinium chelates to be used to improve tumor diagnosis and identify areas for biopsy,16–18 with several studies demonstrating that contrast enhanced MRI shows similar lesion measurements when compared directly with contrast enhanced CT.16,19 Since the early 1990s, T1-weighted MRI used with the addition of contrast agents that shorten T1 relaxation time constants has been the gold standard for brain tumor detection, diagnosis, clinical monitoring, and response assessment for new therapies in clinical trials (Figure 1).
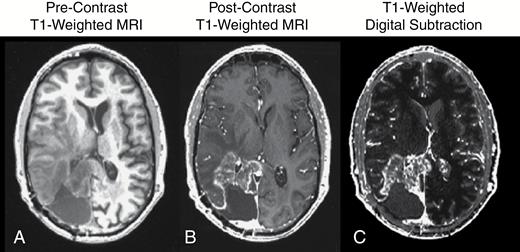
(A) Precontrast T1-weighted MR image; (B) postcontrast T1-weighted MR image; and (C) contrast enhanced T1-weighted digital subtraction maps in a patient with recurrent glioblastoma.
Contrast Enhancement as a Surrogate of Disease in Malignant Glioma
A seminal study by Butler et al20 in 1978 was the first to document the association between contrast enhancement on postcontrast CT and corresponding histological features of malignancy (cellularity, pleomorphism, vascularity, and necrosis) in anaplastic astrocytoma. This was followed by a series of similar studies with improved in vivo21–24 and postmortem stereotactic localization,25 demonstrating similar association between pathology and contrast enhancement (Table 1).
Contrast enhancement on CT or MRI as a surrogate of disease in malignant glioma*
Biomarker . | Incremental Context of Use . | Incremental Evidentiary Standards for Regulatory Qualification . | ||||
---|---|---|---|---|---|---|
Risk-Benefit . | Evidentiary Standards . | |||||
Purpose . | Drug Development Circumstance . | Decision/Action . | Benefit . | Risk . | ||
Prognosis | ||||||
Initial/Residual Contrast- Enhancing Tumor Size (with or without digital image subtraction) | Prognosis (pretreatment) | Risk stratification (homogenize risk) enables drug development and use in more clearly defined patient groups (risk stratification) | Establish as “required/ necessary” test/ measurement | Reduced bias associated with large and small enhancing lesions, which tend to have dramatically different prognoses, independent of therapy. Better enable drug development by homogenizing risk. | Not accounting for enhancing tumor size prior to therapy may result in premature “failure” of new therapies due to risk bias associated with initial tumor size. Larger sample sizes needed to observe a treatment effect for a drug in the phase III setting. | Surgical studies demonstrating contrast- enhancing tumor contains the highest tumor cell density and proliferation rate, along with other histologic measures of malignancy.16,18,20–31 Prospective single and/or multicenter data associating initial enhancing tumor size or residual enhancing tumor size after resection with time to event outcome (TTP, PFS, objective response rate, OS).32–77,79–91,129,191–194 Prospective phase I–III trials associating initial tumor size, residual enhancing tumor size, or extent of resection with time to event outcome (TTP, PFS, objective response rate, OS).61,92–99,166 |
Biomarker . | Incremental Context of Use . | Incremental Evidentiary Standards for Regulatory Qualification . | ||||
---|---|---|---|---|---|---|
Risk-Benefit . | Evidentiary Standards . | |||||
Purpose . | Drug Development Circumstance . | Decision/Action . | Benefit . | Risk . | ||
Prognosis | ||||||
Initial/Residual Contrast- Enhancing Tumor Size (with or without digital image subtraction) | Prognosis (pretreatment) | Risk stratification (homogenize risk) enables drug development and use in more clearly defined patient groups (risk stratification) | Establish as “required/ necessary” test/ measurement | Reduced bias associated with large and small enhancing lesions, which tend to have dramatically different prognoses, independent of therapy. Better enable drug development by homogenizing risk. | Not accounting for enhancing tumor size prior to therapy may result in premature “failure” of new therapies due to risk bias associated with initial tumor size. Larger sample sizes needed to observe a treatment effect for a drug in the phase III setting. | Surgical studies demonstrating contrast- enhancing tumor contains the highest tumor cell density and proliferation rate, along with other histologic measures of malignancy.16,18,20–31 Prospective single and/or multicenter data associating initial enhancing tumor size or residual enhancing tumor size after resection with time to event outcome (TTP, PFS, objective response rate, OS).32–77,79–91,129,191–194 Prospective phase I–III trials associating initial tumor size, residual enhancing tumor size, or extent of resection with time to event outcome (TTP, PFS, objective response rate, OS).61,92–99,166 |
*Descriptions of the context of use (prognosis), how it will be used in drug development (risk stratification), balance of potential benefits and risks, as well as the evidentiary standards available to support use of contrast enhancement as a surrogate of disease in malignant glioma, per recommendations by the FDA and NIH.
Contrast enhancement on CT or MRI as a surrogate of disease in malignant glioma*
Biomarker . | Incremental Context of Use . | Incremental Evidentiary Standards for Regulatory Qualification . | ||||
---|---|---|---|---|---|---|
Risk-Benefit . | Evidentiary Standards . | |||||
Purpose . | Drug Development Circumstance . | Decision/Action . | Benefit . | Risk . | ||
Prognosis | ||||||
Initial/Residual Contrast- Enhancing Tumor Size (with or without digital image subtraction) | Prognosis (pretreatment) | Risk stratification (homogenize risk) enables drug development and use in more clearly defined patient groups (risk stratification) | Establish as “required/ necessary” test/ measurement | Reduced bias associated with large and small enhancing lesions, which tend to have dramatically different prognoses, independent of therapy. Better enable drug development by homogenizing risk. | Not accounting for enhancing tumor size prior to therapy may result in premature “failure” of new therapies due to risk bias associated with initial tumor size. Larger sample sizes needed to observe a treatment effect for a drug in the phase III setting. | Surgical studies demonstrating contrast- enhancing tumor contains the highest tumor cell density and proliferation rate, along with other histologic measures of malignancy.16,18,20–31 Prospective single and/or multicenter data associating initial enhancing tumor size or residual enhancing tumor size after resection with time to event outcome (TTP, PFS, objective response rate, OS).32–77,79–91,129,191–194 Prospective phase I–III trials associating initial tumor size, residual enhancing tumor size, or extent of resection with time to event outcome (TTP, PFS, objective response rate, OS).61,92–99,166 |
Biomarker . | Incremental Context of Use . | Incremental Evidentiary Standards for Regulatory Qualification . | ||||
---|---|---|---|---|---|---|
Risk-Benefit . | Evidentiary Standards . | |||||
Purpose . | Drug Development Circumstance . | Decision/Action . | Benefit . | Risk . | ||
Prognosis | ||||||
Initial/Residual Contrast- Enhancing Tumor Size (with or without digital image subtraction) | Prognosis (pretreatment) | Risk stratification (homogenize risk) enables drug development and use in more clearly defined patient groups (risk stratification) | Establish as “required/ necessary” test/ measurement | Reduced bias associated with large and small enhancing lesions, which tend to have dramatically different prognoses, independent of therapy. Better enable drug development by homogenizing risk. | Not accounting for enhancing tumor size prior to therapy may result in premature “failure” of new therapies due to risk bias associated with initial tumor size. Larger sample sizes needed to observe a treatment effect for a drug in the phase III setting. | Surgical studies demonstrating contrast- enhancing tumor contains the highest tumor cell density and proliferation rate, along with other histologic measures of malignancy.16,18,20–31 Prospective single and/or multicenter data associating initial enhancing tumor size or residual enhancing tumor size after resection with time to event outcome (TTP, PFS, objective response rate, OS).32–77,79–91,129,191–194 Prospective phase I–III trials associating initial tumor size, residual enhancing tumor size, or extent of resection with time to event outcome (TTP, PFS, objective response rate, OS).61,92–99,166 |
*Descriptions of the context of use (prognosis), how it will be used in drug development (risk stratification), balance of potential benefits and risks, as well as the evidentiary standards available to support use of contrast enhancement as a surrogate of disease in malignant glioma, per recommendations by the FDA and NIH.
In 1987, a series of studies published by Kelly et al26,27 acquired 195 serial biopsies from various locations within volumes defined by CT and MRI, noting that contrast enhancement most often corresponded to highest density of tumor tissue. Studies by Burger et al,28 Earnest et al,16 Dean et al,18 and others subsequently29–31 have since confirmed that areas of contrast enhancement on MRI or CT consistently contain the highest density of tumor cells along with the most aggressive histological features in malignant glioma.
Prognostic Significance of Tumor Size: Single and/or Multicenter Data
A multitude of single and/or multicenter studies have confirmed that contrast enhancing tumor size (volume or bidirectional measurements) is a significant prognostic factor contributing to overall survival (OS) in GBM, including pretreatment tumor size, postsurgical residual tumor size, and extent of surgical resection. One of the first studies examining the extent of surgical resection was from Reeves et al32 in 1979, who examined the prognostic significance of tumor size on contrast enhanced CT, age, radiation dose, and performance status. This study noted that tumor size as measured by contrast enhancement plus surrounding edema was not prognostic; however, results suggested small tumors (<300 mm2) trended toward longer OS compared with large tumors (>300 mm2). A study by Andreou et al33 in 1983 examined CT scans in 115 patients in the Cooperative Brain Tumor Study and found that postoperative residual tumor burden was inversely related to OS. In 1987, a study by Ammirati et al34 demonstrated that gross total resection, or resection without any remaining contrast enhancement, is directly associated with longer survival and better quality of life compared with subtotal resection.
A large study in 1988 performed by the Brain Tumor Cooperative Group identified postsurgical residual tumor size as a significant prognostic factor for malignant gliomas, independent of other prognostic variables, including age, tumor grade, or neurological status.35 A study by Vecht et al36 from the Netherlands confirmed this observation. By 1993, a large study by Curran et al37 had examined 1578 malignant gliomas from 3 Radiation Therapy Oncology Group (RTOG) trials from 1974 to 1989. Using a partition analysis consisting of a variety of potential prognostic variables, authors noted that extent of resection divided patients by almost 4 months difference in OS. Also in 1993, a study by Devaux et al38 demonstrated a significant survival advantage in 263 malignant glioma patients with resection compared with biopsy, even when both groups were given radiation therapy. A study in 1994 by Albert et al39 showed that approximately 80% of tumor recurrences emerged from enhancing tumor remnants revealed after postoperative MRI and that patients with residual, postoperative enhancing tumor were at a 6.6× higher risk of death compared with patients without residual enhancing tumor. In 2003, the Glioma Outcomes Project collected data from 788 patients accrued from multiple sites over a 4-year period (1997–2001), providing Class II evidence to support that resection, compared with biopsy, was a strong prognostic factor for newly diagnosed malignant gliomas.40
In 2009, McGirt et al41 published a large study of 949 cases with MR images that underwent surgical procedures at Johns Hopkins University between 1996 and 2006, again noting that extent of resection was associated with improved survival independent of age, World Health Organization (WHO) grade, disability, or subsequent treatments. In 2010, a large French epidemiology study by Bauchet et al42 involving 952 patients clearly determined extent of resection to be a significant prognostic factor in patients with newly diagnosed GBM. RTOG investigators again used partitioning analysis in 2011 to reexamine prognostic factors associated with GBM, again noting that extent of resection is an important factor in determining OS in patients with GBM.43 Additionally, another study in 2011 indicated that extent of resection was prognostic and showed that subtotal resection as low as 78% still had a survival benefit.44 This is similar to a 2014 study by Chaichana et al,45 who found a survival benefit for GBM obtaining more than a 70% extent of resection and a 2014 study by Oppenlander et al,46 who observed an improvement in survival for GBM patients with an extent of resection greater than 80%.
In 2013, investigators from the National Cancer Institute Clinical Center again confirmed the relationship between tumor size and location on prognosis in 92 patients with GBM treated with surgery followed by radiation and temozolomide, noting that patients with the smallest tumor size had a significantly longer OS compared with patients having the largest tumors.47 Additionally, a study by Gutman et al48 confirmed that contrast enhancing tumor volume was strongly associated with poor survival when examining 75 patients with GBM from The Cancer Genome Atlas. Also in 2013, Zinn et al49 conducted possibly the largest study evaluating extent of resection to date, evaluating a total of 21783 patients from 1973–2007 from the population-based Surveillance, Epidemiology, and End Results (SEER) registry. Results from this study again confirmed that gross total resection had a significantly longer median survival compared with subtotal resection. This observation was subsequently confirmed in 2015 by Pan et al50 using the SEER registry to examine 14675 GBM patients from 2000–2009, again noting a significant survival advantage in patients with gross total surgical resection. Additionally, a large 2015 Chinese study in 816 patients by Qin et al51 examined a wide range of potential prognostic variables and again confirmed that extent of surgical resection was a strong, independent predictor of OS.
Together, these single and multicenter studies, along with an extensive list of noteworthy studies that could not be adequately described in detail within the current document,52–91 provide overwhelming evidence that contrast enhancing tumor size—including baseline enhancing tumor size prior to therapy, residual tumor size after surgical resection, and extent of surgical resection—is a significant prognostic factor for malignant glioma, including GBM.
Prognostic Significance of Tumor Size: Prospective Phase I–III Trials
In addition to single and/or multicenter studies, a few prospective phase I–III clinical trials have also demonstrated that baseline tumor size, extent of resection, and residual tumor volume are significant prognostic factors for survival in malignant glioma, including GBM. In 1994, Rostomily et al92 determined that a larger extent of resection and smaller postoperative tumor volumes were associated with prolonged progression-free survival (PFS) in a phase II study of multi-agent chemotherapy in recurrent malignant gliomas. Similarly, a phase II trial of pre-irradiation carboplatin and etoposide along with accelerated hyperfractionated radiotherapy showed that extent of resection prior to therapy was a significant independent predictor of survival.93
In 2003, Vuorinen et al94 performed a prospective, randomized trial directly comparing prognosis in malignant glioma patients who received either stereotactic biopsy or open craniotomy and resection of the tumor. Results confirmed findings in previous studies, indicating that surgical resection provides approximately a 2.8× greater benefit in survival compared with biopsy alone. In 2005, Hauch et al95 performed a meta-analysis summarizing findings from 220 publications containing results from phase II and III trials in high-grade gliomas, totaling 17213 cases mostly treated with tumor resection, radiation therapy, and chemotherapies, including nitrosoureas. Results from this study suggested that extent of tumor resection had a significant positive impact on median OS when combining data from multiple studies. In 2008, Stummer et al61 provided Level 2b evidence that the extent of surgical resection of enhancing tumor is a significant predictor of survival in patients with GBM by examining 243 GBM patients enrolled in a study of 5-aminolevulinic acid (5-ALA), a parallel, randomized, balanced, group-sequential, 2-armed, controlled multicenter phase III study of 5-ALA fluorescence-guided resection versus conventional microsurgery.
In 2009, Stupp et al96 published results from a randomized phase III trial by the European Organisation for Research and Treatment of Cancer and the National Cancer Institute of Canada examining the effects of radiotherapy with concomitant and adjuvant temozolomide versus radiotherapy alone in a total of 573 patients with newly diagnosed GBM. Results of the study clearly indicated that extent of resection was a significant, independent predictor in both treatment arms. In 2015, Suchorska et al97 reported results from the well-characterized Dose-Intensified Temozolomide Rechallenge in Progressive Glioblastoma (DIRECTOR) study (ClinicalTrials.gov #NCT00941460), noting that a complete resection of contrast enhancing tumor on MRI versus residual enhancing tumor at recurrence was associated with a significantly improved OS (median OS = 11.5 mo vs 6.7 mo).
Bevacizumab and anti-angiogenic agents
In 2014, Ellingson et al98 demonstrated that pretreatment enhancing tumor volume was a significant prognostic factor associated with shorter PFS and OS in recurrent GBM treated with bevacizumab with and without adjuvant irinotecan by examining 160 patients in the BRAIN trial, an open-label, multicenter, randomized, noncomparative phase II trial performed to assess the effectiveness of bevacizumab with or without irinotecan. In 2015, Ellingson et al99 confirmed these findings and demonstrated that pretreatment enhancing tumor volume was a significant prognostic factor for survival in recurrent GBM treated with bevacizumab and chemotherapy by examining 123 recurrent GBM patients in ACRIN 6677/RTOG 0625, a multicenter, randomized, phase II trial of bevacizumab and chemotherapy in recurrent GBM.
In summary, there is a general consensus that extent of resection is one of the most important prognostic factors for GBM, largely based on the multitude of single and multicenter studies outlined previously. A recent study by Ellingson et al100 that included 497 patients from 4 different data sources, including 2 single-center sites and 2 multicenter phase II trials, solidified this point by showing that baseline contrast enhancing tumor volume was a significant predictor of OS in temozolomide, lomustine, bevacizumab (with or without irinotecan), and cabozantinib. It is important to point out, however, that most clinical trials with >2 treatment arms do not directly account for baseline tumor size or extent of resection despite overwhelming evidence that this is important. Instead, most studies balance the proportion of patients with different extents of resection between the various treatment arms.
Change in Contrast Enhancing Lesion Size as a Surrogate for Treatment Efficacy in Malignant Glioma
Change in contrast enhancing tumor size (MRI or CT) has been used to inform clinical decision making in malignant gliomas for more than 50 years and remains the gold standard for treatment efficacy in GBM (Table 2). Until relatively recently, response was determined by the criteria proposed by Macdonald et al in 1990, 101 which significantly improved upon earlier methods of response assessment, including the Levin criteria102 and the WHO oncology response criteria,103 by accounting for corticosteroid use and changes in neurological status. These “Macdonald criteria” used changes in the measurement of contrast enhancing tumor burden to determine treatment efficacy, similar to the previous criterion and similar to the Response Evaluation Criteria in Solid Tumors (RECIST).104
Change in contrast enhancing lesion size as a surrogate for treatment efficacy in malignant glioma*
Biomarker . | Incremental Context of Use . | Incremental Evidentiary Standards for Regulatory Qualification . | ||||
---|---|---|---|---|---|---|
Risk-Benefit . | Evidentiary Standards . | |||||
Purpose . | Drug Development Circumstance . | Decision/Action . | Benefit . | Risk . | ||
Control/Relieve/Eliminate | ||||||
Change in Contrast- Enhancing Tumor Size (with or without digital image subtraction) | Response indicator (posttreatment prognosis) | Use as posttreatment measure of a favorable effect of a drug. | Inform decision to move to “continue a therapy” in a patient or inform the decision to move to the next phase of development | Absence of favorable effect informs the decision to change therapy, limiting patient exposure to ineffective therapy. Reduced drug development and trial costs as total trial durations needed to observe a treatment effect are reduced. Ability to isolate individual therapeutic effects in the recurrent setting (whereas OS can be complicated by combinations of various therapies). | Effective therapies that significantly modulate vascular permeability may result in premature “failure” or “acceptance” of new therapies (eg, anti-angiogenic or immunotherapies). Favorable effect may not reflect “clinical benefit” in terms of neurologic symptoms or quality of life. | Change in contrast enhancing tumor size (on MRI or CT) has been used to inform clinical decision making in malignant gliomas for >50 years and is the gold standard for response assessment.101–103,106–109 Prospective single and/or multicenter studies using change in contrast enhancing tumor size as a surrogate endpoint for treatment efficacy or associating change in enhancing tumor size with time to event outcome (TTP, PFS, ORR, QOL, PRO, neurocognitive function, and/or OS).89,118–123,127–132,147,195,196 Phase I–III trials in recurrent malignant gliomas using change in contrast enhancing tumor size as a surrogate endpoint for treatment efficacy (ie, PFS, TTP, or ORR), or have shown an association between radiographic response and outcome (QOL, PRO, neurocognitive function, PFS, TTP, ORR, or OS).92,93,98,129,130,133–146, 148–153,155,156,158,160–165, 167–189,196–204 |
Biomarker . | Incremental Context of Use . | Incremental Evidentiary Standards for Regulatory Qualification . | ||||
---|---|---|---|---|---|---|
Risk-Benefit . | Evidentiary Standards . | |||||
Purpose . | Drug Development Circumstance . | Decision/Action . | Benefit . | Risk . | ||
Control/Relieve/Eliminate | ||||||
Change in Contrast- Enhancing Tumor Size (with or without digital image subtraction) | Response indicator (posttreatment prognosis) | Use as posttreatment measure of a favorable effect of a drug. | Inform decision to move to “continue a therapy” in a patient or inform the decision to move to the next phase of development | Absence of favorable effect informs the decision to change therapy, limiting patient exposure to ineffective therapy. Reduced drug development and trial costs as total trial durations needed to observe a treatment effect are reduced. Ability to isolate individual therapeutic effects in the recurrent setting (whereas OS can be complicated by combinations of various therapies). | Effective therapies that significantly modulate vascular permeability may result in premature “failure” or “acceptance” of new therapies (eg, anti-angiogenic or immunotherapies). Favorable effect may not reflect “clinical benefit” in terms of neurologic symptoms or quality of life. | Change in contrast enhancing tumor size (on MRI or CT) has been used to inform clinical decision making in malignant gliomas for >50 years and is the gold standard for response assessment.101–103,106–109 Prospective single and/or multicenter studies using change in contrast enhancing tumor size as a surrogate endpoint for treatment efficacy or associating change in enhancing tumor size with time to event outcome (TTP, PFS, ORR, QOL, PRO, neurocognitive function, and/or OS).89,118–123,127–132,147,195,196 Phase I–III trials in recurrent malignant gliomas using change in contrast enhancing tumor size as a surrogate endpoint for treatment efficacy (ie, PFS, TTP, or ORR), or have shown an association between radiographic response and outcome (QOL, PRO, neurocognitive function, PFS, TTP, ORR, or OS).92,93,98,129,130,133–146, 148–153,155,156,158,160–165, 167–189,196–204 |
Abbreviations: ORR, objective response rate; QOL, quality of life; PRO, patient reported outcome.
*Descriptions of the context of use (response indicator), how it will be used in drug development (measure of efficacy), balance of potential benefits and risks, as well as the evidentiary standards available to support use of change in contrast enhancement as a surrogate of posttreatment prognosis or outcome in malignant glioma.
Change in contrast enhancing lesion size as a surrogate for treatment efficacy in malignant glioma*
Biomarker . | Incremental Context of Use . | Incremental Evidentiary Standards for Regulatory Qualification . | ||||
---|---|---|---|---|---|---|
Risk-Benefit . | Evidentiary Standards . | |||||
Purpose . | Drug Development Circumstance . | Decision/Action . | Benefit . | Risk . | ||
Control/Relieve/Eliminate | ||||||
Change in Contrast- Enhancing Tumor Size (with or without digital image subtraction) | Response indicator (posttreatment prognosis) | Use as posttreatment measure of a favorable effect of a drug. | Inform decision to move to “continue a therapy” in a patient or inform the decision to move to the next phase of development | Absence of favorable effect informs the decision to change therapy, limiting patient exposure to ineffective therapy. Reduced drug development and trial costs as total trial durations needed to observe a treatment effect are reduced. Ability to isolate individual therapeutic effects in the recurrent setting (whereas OS can be complicated by combinations of various therapies). | Effective therapies that significantly modulate vascular permeability may result in premature “failure” or “acceptance” of new therapies (eg, anti-angiogenic or immunotherapies). Favorable effect may not reflect “clinical benefit” in terms of neurologic symptoms or quality of life. | Change in contrast enhancing tumor size (on MRI or CT) has been used to inform clinical decision making in malignant gliomas for >50 years and is the gold standard for response assessment.101–103,106–109 Prospective single and/or multicenter studies using change in contrast enhancing tumor size as a surrogate endpoint for treatment efficacy or associating change in enhancing tumor size with time to event outcome (TTP, PFS, ORR, QOL, PRO, neurocognitive function, and/or OS).89,118–123,127–132,147,195,196 Phase I–III trials in recurrent malignant gliomas using change in contrast enhancing tumor size as a surrogate endpoint for treatment efficacy (ie, PFS, TTP, or ORR), or have shown an association between radiographic response and outcome (QOL, PRO, neurocognitive function, PFS, TTP, ORR, or OS).92,93,98,129,130,133–146, 148–153,155,156,158,160–165, 167–189,196–204 |
Biomarker . | Incremental Context of Use . | Incremental Evidentiary Standards for Regulatory Qualification . | ||||
---|---|---|---|---|---|---|
Risk-Benefit . | Evidentiary Standards . | |||||
Purpose . | Drug Development Circumstance . | Decision/Action . | Benefit . | Risk . | ||
Control/Relieve/Eliminate | ||||||
Change in Contrast- Enhancing Tumor Size (with or without digital image subtraction) | Response indicator (posttreatment prognosis) | Use as posttreatment measure of a favorable effect of a drug. | Inform decision to move to “continue a therapy” in a patient or inform the decision to move to the next phase of development | Absence of favorable effect informs the decision to change therapy, limiting patient exposure to ineffective therapy. Reduced drug development and trial costs as total trial durations needed to observe a treatment effect are reduced. Ability to isolate individual therapeutic effects in the recurrent setting (whereas OS can be complicated by combinations of various therapies). | Effective therapies that significantly modulate vascular permeability may result in premature “failure” or “acceptance” of new therapies (eg, anti-angiogenic or immunotherapies). Favorable effect may not reflect “clinical benefit” in terms of neurologic symptoms or quality of life. | Change in contrast enhancing tumor size (on MRI or CT) has been used to inform clinical decision making in malignant gliomas for >50 years and is the gold standard for response assessment.101–103,106–109 Prospective single and/or multicenter studies using change in contrast enhancing tumor size as a surrogate endpoint for treatment efficacy or associating change in enhancing tumor size with time to event outcome (TTP, PFS, ORR, QOL, PRO, neurocognitive function, and/or OS).89,118–123,127–132,147,195,196 Phase I–III trials in recurrent malignant gliomas using change in contrast enhancing tumor size as a surrogate endpoint for treatment efficacy (ie, PFS, TTP, or ORR), or have shown an association between radiographic response and outcome (QOL, PRO, neurocognitive function, PFS, TTP, ORR, or OS).92,93,98,129,130,133–146, 148–153,155,156,158,160–165, 167–189,196–204 |
Abbreviations: ORR, objective response rate; QOL, quality of life; PRO, patient reported outcome.
*Descriptions of the context of use (response indicator), how it will be used in drug development (measure of efficacy), balance of potential benefits and risks, as well as the evidentiary standards available to support use of change in contrast enhancement as a surrogate of posttreatment prognosis or outcome in malignant glioma.
For nearly 20 years the Macdonald criteria and general use of contrast enhancement as a surrogate of tumor burden were used for the evaluation of new therapies, including use for the accelerated approval of temozolomide in recurrent anaplastic astrocytoma and identification of the chemotherapy responsiveness of anaplastic oligodendroglioma, which was subsequently confirmed in OS benefit in phase III trials.105 In 2010, the Response Assessment in Neuro-Oncology (RANO) criteria were developed106 to comprehensively reform the Macdonald criteria by using the evolving principles outlined in previous work.107–109 At the core of the RANO criteria remains the use of changes in contrast enhancing tumor burden as a biomarker for treatment efficacy; however, the RANO criteria also added language for the evaluation of non-enhancing tumor progression, better definitions of measurable and nonmeasurable disease, definitions of progression for patients being considered for enrollment into clinical trials, recommendations to address pseudoprogression and pseudoresponse, the requirement of confirmatory scans for response, and recommendations for dealing with patients with equivocal imaging changes. Use of the RANO criteria is the current standard for response assessment in GBM clinical trials, and the details and practical implementation strategies are well documented.110–116
Change in Contrast Enhancing Tumor Size as a Surrogate for Treatment Efficacy: Single and/or Multicenter Data
As early as 1979, a study by McCullough et al117 examined 2 children with GBM undergoing chemotherapy and demonstrated that volumetric measurements of enhancing tumor revealed good correlations between radiographic and anatomic data obtained at autopsy and suggested for the first time that volumetric measurements using contrast enhanced CT may be a practical method for monitoring results of radiotherapy and chemotherapy in GBM. In 1988, a study by the Brain Tumor Cooperative Group involving 510 patients revealed that change in contrast enhancing tumor size was prognostic.35 Also in 1988, a study by Kumar et al118 reported the correlation between radiographic response and survival in 38 GBM patients treated with intraoperative endocurietherapy using cobalt-60.
In 1990, a single-center study by Finlay et al119 reported radiographic response of histologically confirmed malignant astrocytoma treated with high-dose bis-cloroethylnitrosourea (BCNU) followed by bone marrow infusion, noting that 4 of 10 patients showed early complete radiographic response, or complete shrinkage of contrast enhancing tumor, which resulted in remission for more than 290 days. This study even noted that early stability of enhancing tumor size resulted in sustained disease control for more than 13 months and that total response rate for this therapy was nearly 60%. In 1993, Couldwell et al120 reported that a minority of patients with recurrent malignant gliomas showed radiographic response to high-dose tamoxifen, which corresponded to long-term clinical benefit, whereas patients showing rapid early progression died within 3–6 months from start of therapy. A single-center study by Jakacki et al89 examining dose-intensive, time-compressed probarbazine, CCNU, procarbazine/lomustine/vincristine (PCV), and peripheral blood stem cell support with concurrent radiation in newly diagnosed high-grade gliomas reported early radiographic response via a decrease on contrast enhancing tumor in only 3 of 12 patients, which corresponded to a decrease in clinical symptoms. Additionally, 4 patients showed early radiographic progression coinciding with rapid clinical deterioration.
In 2004, See et al121 performed a retrospective analysis of recurrent GBM patients treated with 13-cis-retinoic acid, noting low radiographic response rates and longer PFS in patients with partial or minor radiographic response via change in contrast enhancement. In 2009, a single-center study by Patel et al122 investigated the efficacy of performing salvage re-irradiation for recurrent GBM using radiosurgery. Results indicated that radiographic response via contrast enhancement was observed in nearly 40% of patients, and these patients had a significant survival advantage compared with nonsurvivors (15.8 vs 7.3 mo). In 2011, a single-center study by Gladwish et al123 involving 30 patients with GBM demonstrated that the magnitude of early imaging response in patients treated with standard radiochemotherapy was predictive of outcome, particularly when observing early decreases in tumor size.
Bevacizumab and anti-angiogenic agents
The use of bevacizumab and other anti-angiogenic agents has been shown to alter vascular permeability, often resulting in large radiographic responses as measured using contrast enhancement on postcontrast T1-weighted images. However, this decrease in contrast enhancement often is associated with a real change in tumor biology and, since bevacizumab targets blood vasculature by neutralizing vascular endothelial growth factor (VEGF), suggests increased biological activity in these tumors. Further, there is evidence from single-center studies as well as multicenter trials showing that radiographic response to anti-angiogenic agents results in improved clinical symptoms, increased PFS, and often increased OS.
In 2006, Pope et al124 first noticed the high number of patients exhibiting a decrease in contrast enhancing tumor treated with bevacizumab. Investigators noted that patients who did not exhibit stability or radiographic response experienced rapid tumor growth on postcontrast MRI resulting in death. In 2008, a single-center study by Bokstein et al125 examined treatment response of bevacizumab and irinotecan for recurrent high-grade gliomas, noting 2 of 19 patients having a complete response and 7 of 19 patients exhibiting a partial radiographic response via a decrease in contrast enhancement. The authors note that patients with early radiographic response, partial or complete, had a substantially higher PFS and OS. A single-center retrospective study involving bevacizumab in recurrent malignant gliomas by Norden et al126 in 2008 further outlined challenges associated with using early changes in contrast enhancement as a surrogate for drug efficacy. However, authors note that progression is difficult to evaluate using contrast enhancement alone, as progression on bevacizumab was shown to result in an increase in T2 hyperintense, infiltrative tumor. The authors do show only a modest radiographic response to bevacizumab, with only a 2.3% complete response in these patients. In 2009, a single-center study of bevacizumab plus irinotecan reported by Zuniga et al127 again demonstrated dramatic radiographic response rates largely attributed to the decrease in vascular permeability accompanying anti-VEGF therapy.
These dramatic radiographic responses via contrast enhancing tumor did, however, correspond with improved clinical outcome compared with historical controls, including a longer PFS. A retrospective evaluation of salvage therapy using single agent bevacizumab in recurrent GBM reported by Chamberlain et al128 showed no patients with complete shrinkage of contrast enhancing tumor, 42% of patients demonstrating partial response on the first scan, and 58% of patients demonstrating contrast enhancing tumor growth. Interestingly, the data show that patients who had a partial radiographic response via a decrease in contrast enhancement had significantly longer OS from the start of bevacizumab compared with patients who did not respond (median OS = 12 vs 5.5 mo). A 2013 study by Huang et al129 examining volumetric change in contrast enhancing tumor in 91 recurrent GBM patients treated with bevacizumab found that patients exhibiting a large decrease on contrast enhancing tumor had significantly longer PFS and OS.
This evidence, combined with evidence from a wealth of additional single-center studies that were not described in detail,130–132 suggests that the change in contrast enhancing tumor size is useful for determining early drug efficacy in malignant glioma, including GBM.
Change in Contrast-Enhancing Tumor Size as a Surrogate for Treatment Efficacy: Phase I–III Clinical Trials
Change in contrast enhancing tumor size has historically been used to measure response to new therapies in phase I–III clinical trials for more than 30 years. In 1986, Greenberg et al133 utilized change in contrast enhancement on CT to assess treatment response in a phase I–II evaluation of intra-arterial diaziquone for recurrent malignant astrocytomas, noting that 2 of 20 patients experienced partial radiographic responses, 4 of 20 patients showed stabilization of disease over a period of a few months, and 1 patient had tumor shrinkage. In 1994, Rostomily et al92 evaluated 51 patients with recurrent malignant gliomas in a phase II trial of multidrug therapy (6-thioguanine, dibromodulcitol, procarbazine, etc.), noting that approximately 57% of patients had an objective radiographic response, or at least partial shrinking of contrast enhancing tumor, or stabilization of enhancing tumor, which translated to a significantly higher PFS (38 wk vs 7 wk) and OS (79 wk vs 18 wk).
A study reported by Macdonald et al134 in 1996 explored the use of topotecan in a phase II trial in patients with recurrent malignant glioma. Results showed that 2 of 31 patients had either a complete or a partial radiographic response with respect to contrast enhancing tumor, whereas 21 of 31 patients had stable disease. Investigators used the lack of radiographic response as defined by change in contrast enhancing tumor to conclude that topotecan has only modest activity in recurrent malignant glioma. In a single-arm phase I–II multicenter trial of BCNU-fluosol and oxygen inhalation in recurrent malignant glioma, Hochberg et al135 reported longer PFS and OS in patients with radiographic response or stabilization compared with patients showing early failure.
In 1997, Fine et al136 reported results from a phase I trial of a new recombinant human beta-interferon (BG9015) in patients with recurrent gliomas. Results demonstrated a strong association between radiographic response using change in contrast enhancing tumor and BG9015 serum levels along with survival, again supporting the use of change in contrast enhancing tumor as a surrogate of early treatment efficacy. In a 1997 phase II multicenter study by Fetell et al137 exploring the efficacy of pre-irradiation paclitaxel in GBM, investigators noted that none of the 15 patients showed radiographic response as determined by change in contrast enhancing tumor size, suggesting to the investigators that paclitaxel has only minimal activity in GBM. In 1998, Chang et al138 described results from a phase II trial of high dose oral tamoxifen and subcutaneous interferon alpha-2a in recurrent gliomas, for which radiographic response was used as the primary endpoint. Despite extreme toxicity issues associated with the treatment resulting in early study closure, the majority (12 of 16) of evaluable patients showed early progressive disease indicative of treatment failure 6 weeks after therapy, suggesting to the authors that this treatment strategy is not effective in recurrent gliomas.
A 1999 phase II study of pre-irradiation carboplatin and etoposide and accelerated hyperfractionated radiation therapy in patients with high-grade astrocytomas by Jeremic et al93 again used radiographic response using change contrast enhancing tumor size on postcontrast CT as a surrogate for drug efficacy. Results of this study reported that no patients had complete shrinkage of enhancing tumor, and the lack of radiographic evidence of treatment effect resulted in study failure as reported by the investigators. In 1999, Yung et al139 reported results from a phase II trial of temozolomide in anaplastic gliomas at first relapse, again using radiographic response as a measure of drug efficacy. This study reported that radiographic response, as evaluated by a decrease in enhancing tumor volume, was associated with benefits to health-related quality of life. In line with this finding, a 1999 report by Hess et al140 summarized the relationship between radiographic response via a change in contrast enhancement and outcomes in 375 patients previously enrolled in 8 consecutive phase II chemotherapy trials. The investigators conclude that when response was treated as a covariate in a multivariate Cox proportional hazards regression model, contrast enhancing tumor shrinkage was associated with a significantly lower treatment failure rate.
In support of these observations, a 2000 report on a phase II trial of thalidomide in recurrent high-grade gliomas by Fine et al141 reported 4 of 36 patients had at least a partial radiographic response as determined by a change in contrast enhancement, while 12 had stable disease. Again in support of the use of change in contrast enhancing tumor size as a surrogate of treatment efficacy, median time to tumor progression (TTP) for nonresponders was approximately 8 weeks compared with 15 and 33 for stable and responding patients, respectively. Additionally, patients exhibiting radiographic response via a decrease in contrast enhancing tumor had a median OS of 74 weeks, whereas patients with stable or progressing disease had a survival of 30 and 22 weeks, respectively. In 2000, Yung et al142 reported results from a phase II study of temozolomide versus procarbazine in recurrent GBM, noting that radiographic response was higher in patients receiving temozolomide, which also corresponded to longer PFS and OS. A phase II study in 2002 by Kahn et al143 evaluating efficacy of extended low-dose temozolomide also used radiographic response via contrast enhancement to determine efficacy, in addition to PFS and OS. The choice to use these metrics was largely based on radiographic response rates initially observed in the phase I study.144
In 2003, Fine et al145 used objective radiographic response compared with historical controls as a measure of efficacy to evaluate thalidomide and carmustine in patients with recurrent high-grade gliomas as part of a phase II trial, noting that the favorable radiographic response was evidence of antitumor activity. A 2003 phase I study by Sampson et al146 evaluating intracerebral micro-infusion of a recombinant chimeric targeted toxin composed of an epidermal growth factor receptor binding ligand transforming growth factor alpha and pseudomonas exotoxin (PE-38) showed that patients with radiographic responses via contrast enhancing tumor had long-term survival advantages compared with patients exhibiting no signs of radiographic response. This was followed up by a study by Sampson et al147 showing that radiographic response as indicated by a shrinkage in contrast enhancing tumor corresponded directly with histologic evidence of response via biopsy as well as hypometabolism on positron emission tomography (PET).
In 2004, a series of both phase I and phase II trials examining irinotecan plus BCNU148,149 demonstrated “clear antitumor activity as measured by radiographic response,” again supporting the use of change in contrast enhancing tumor size as a measure of early drug efficacy in clinical trials. In 2005, Pipas et al150 reported low radiographic response rates, but high rates of radiographic stability in a phase II trial of paclitaxel and topotecan with filgrastim in patients with recurrent malignant gliomas, which the investigators used as evidence that this combination has only modest activity. In a 2005 phase II study of 131-I-labeled anti-tenascin 81C6 murine monoclonal antibody in patients with newly diagnosed malignant gliomas, regions showing an increase in contrast enhancement indicative of treatment failure were found to be hypermetabolic on PET, providing molecular imaging evidence that change in enhancing tumor often corresponds with nonresponsive tumor.151 Also in 2005, Galanis et al152 showed that median time to tumor progression was significantly longer in patients who demonstrated radiographic response via contrast enhancement compared with patients not showing a response (5.4 vs 2.3 mo) in a phase II trial of temsirolimus in recurrent GBM. Similarly, a phase I trial of irinotecan plus temozolomide in recurrent malignant gliomas by Reardon et al153 also showed that radiographic response via a decrease in contrast enhancing tumor size corresponded to a longer median time to tumor progression compared with patients who did not show a response. A phase II study of imatinib mesylate plus hydroxyurea in recurrent GBM also reported similar findings in terms of radiographic response rate and PFS.154
A 2007 study by Lustig et al155 explicitly examined whether imaging response via change in contrast enhancement on CT or MRI correlated with survival in 453 patients enrolled in RTOG 90-06, a phase I/II study to compare standard radiation with BCNU versus hyperfractionated radiation with BCNU in newly diagnosed malignant gliomas. Results of the study suggested that CT or MRI response was a significant predictor of median survival and 2-year OS, further establishing the relationship of response to treatment and survival in malignant gliomas. A 2010 phase II trial of intratumoral BCNU injection and radiotherapy on newly diagnosed malignant glioma156 used radiographic response via contrast enhancing tumor as the primary endpoint for the trial. The 2 of 12 patients who exhibited a partial response had PFS and OS of more than 5 years, which was substantially longer than the other patients (average, PFS = 9 mo, OS = 15 mo).
Bevacizumab and anti-angiogenic agents
As mentioned in single-center studies above, the use of bevacizumab and other anti-angiogenic agents often results in radiographic response as measured using contrast enhancement on postcontrast T1-weighted images. Generally speaking, most of the clinical trials involving bevacizumab or other anti-angiogenic agents have used radiographic response as either a primary or a secondary endpoint for the trial,157,158 but relatively few studies have explored whether radiographic response in bevacizumab-treated patients results in patient benefit or biological changes within the tumor associated with favorable drug activity. Of the studies that did examine these associations, the decrease in contrast enhancement can often be associated with increased drug activity in malignant gliomas. For example, a study in 2007 by Vredenburgh et al159 was one of the first phase II trials of bevacizumab in recurrent malignant gliomas, reporting only complete shrinkage of contrast enhancing tumor in 1 of 32 patients, but 19 of 32 patients exhibited a partial radiographic response via a decrease in contrast enhancement. Despite noting higher than expected radiographic response rates, the authors failed to mention whether an association was found between radiographic responders and prolonged survival. This was addressed in a 2009 phase II trial of single-agent bevacizumab followed by bevacizumab plus irinotecan in recurrent tumors reported by Kreisl et al,160 which showed that early response on postcontrast MRI was predictive of long-term PFS (ie, longer local control of the disease).
A phase II trial of metronomic chemotherapy with daily oral etoposide plus bevacizumab for recurrent malignant gliomas demonstrated that patients with sustained radiographic response had a favorable prognosis, including no evidence of hypermetabolic tumor on PET scans. In a phase II trial of cetuximab, bevacizumab, and irinotecan for patients with primary and recurrent GBM,161 approximately 26% (11 of 32 patients) showed some favorable radiographic response, which translated to a significant difference in TTP. A 2010 phase II trial of bevacizumab and erlotinib in patients with recurrent malignant glioma162 also showed a significant correlation between radiographic response and PFS in GBM along with a significant correlation between radiographic response and both PFS and OS in anaplastic astrocytomas. In 2011, Prados et al163 performed landmark analysis to evaluate the association between radiographic response as measured by a change in contrast enhancing tumor at 9, 18, or 26 weeks and OS for 167 patients with recurrent GBM who participated in the BRAIN trial, a phase II trial evaluating the efficacy of bevacizumab alone or in combination with irinotecan. After correcting for known prognostic factors, including age, neurological status, number of prior relapses, and treatment arms, investigators clearly showed that radiographic response measured at each time point evaluated (9, 18, and 26 wk) predicted OS.
A 2011 study by Wefel et al164 also examined the same patients in the BRAIN trial and found that patients who exhibited radiographic response as indicated by a decrease in contrast enhancing tumor size had stable or improved performance on a variety of neurocognitive tests at the time of the response as well as 24 weeks from the start of therapy. In 2013, Boxerman et al165 examined whether radiographic response as indicated by a change in contrast enhancing tumor size (volume and bidirectional) was predictive of OS in ACRIN 6677/RTOG 0625, a multicenter, randomized, phase II trial of bevacizumab with irinotecan or temozolomide in recurrent GBM. Results suggested that patients with stable or partial response at 8 or 16 weeks had significantly longer survival compared with those who showed growing contrast enhancing tumor. Lastly, a 2014 study by Ellingson et al98 reexamined the BRAIN trial data using contrast enhanced digital subtraction of precontrast T1-weighted images from postcontrast T1-weighted images to highlight areas of subtle enhancement. Results of this study clearly indicated that contrast enhancement was apparent in almost all patients when using T1 subtraction, suggesting that the high radiographic response rates previously reported in bevacizumab may have lacked sensitivity for detecting subtle enhancement in the underlying tissue. Further, results indicated that patients exhibiting >25% decrease in contrast enhancing tumor volume after the first dose of bevacizumab had significantly longer PFS and OS. A follow-up study166 subsequently showed that prediction of PFS and OS could be improved by including a second confirmatory scan, again using T1 subtraction to better delineate areas of true contrast enhancement from surrounding tissues.
The radiographic response as measured by a change in contrast enhancing tumor size has not been appreciable for anti-angiogenic agents other than bevacizumab. In a phase II trial of pazopanib,167 an oral multitargeted angiogenesis inhibitor in recurrent GBM, radiographic response was low (2 partial responses out of 35 patients), which translated to equally low PFS and OS. In 2010, Batchelor et al168 presented results from a phase II study of cediranib, an oral pan-VEGF receptor tyrosine kinase inhibitor, in recurrent GBM. Results showed that patients exhibiting a partial or minor radiographic response via contrast enhancement corresponded with longer PFS as well as improvement in Karnofsky performance status. In 2011, de Groot et al169 described results from a phase II trial of aflibercept, a VEGF trap, in recurrent malignant glioma, noting that patients with anaplastic gliomas or GBM exhibiting a partial radiographic response had longer median PFS compared with the entire study cohort stratified by grade (23 wk vs 12 wk for GBM; 45 wk vs 24 wk for anaplastic gliomas).
In summary, a very large number of phase I–III clinical trials, including additional noteworthy studies not described in detail,129,130,169–189 have used radiographic response via change in contrast enhancement as a measure of drug efficacy or as a primary or secondary endpoint and/or have shown a clear relationship between radiographic response and outcomes, including histologic response, metabolic changes, patient reported outcomes, quality of life measures, PFS, or OS.
Limitations to Interpretation
It is important to note that other clinical, genetic, neurological, and medical factors (isocitrate dehydrogenase status, O6-methylguanine-DNA methyltransferase methylation status, age, performance status, prior therapies, etc.) may have significantly impacted outcomes and may not have been considered at the time of the initial studies. Additionally, many early studies had differing eligibility requirements, which may have biased recurrent studies by allowing enrollment or identifying early progression in patients with potential pseudoprogression, as well as slightly different definitions for disease progression, which may slightly adjust measures of TTP or PFS. Also, many studies may not have used centralized, blinded review, which may account for some additional discrepancies. Despite these and other potential caveats, including radiation and treatment effects that can mimic disease (summarized by Ellingson et al190), there appears to be overwhelming evidence to suggest that contrast enhancement is a significant biomarker for baseline risk stratification and as a posttherapeutic measure of drug efficacy.
Conclusion
Altogether, there is an abundance of scientific evidence spanning decades supporting the use of contrast enhancement as a surrogate biomarker for disease burden and as a tool for measuring treatment response in malignant glioma. The current article explicitly describes multiple levels of verification, or evidentiary standards, to support the use of contrast enhancement in these specific contexts of use. This comprehensive review should be valuable for regulatory institutions involved in drug approval and for use in the biomarker qualification process.
Funding
None.
Conflict of interest statement. Consultant and/or Advisory Role: B. Ellingson: MedQIA, Roche/Genentech, Agios, Insys, OmniOx, Nativis, BMS, Siemens, Janssen, Medicenna, Imaging Endpoints, Novogen; P. Wen: Agios, Genentech/Roche, Cavion, Cortice Biosciences, Foundation Medicine, Insys, Monteris, Novartis, Vascular Biogenics, VBI Vaccines; T. Cloughesy: Roche/Genentech, Amgen, Tocagen, NewGen, LPath, Proximagen, Celgene, Vascular Biogenics Ltd, Insys, Agios, Cortice Bioscience, Pfizer, Human Longevity, BMS, Merck, Notable Lab, MedQIA.
References