-
PDF
- Split View
-
Views
-
Cite
Cite
Collin J. Farrell, Brett M. Johnson, Adam G. Hansen, Christopher A. Myrick, Eric C. Anderson, Thomas A. Delomas, Andrea D. Schreier, Joel P. Van Eenennaam, Cytological and Molecular Approaches for Ploidy Determination: Results from a Wild Walleye Population, North American Journal of Fisheries Management, Volume 42, Issue 4, August 2022, Pages 849–856, https://doi.org/10.1002/nafm.10771
- Share Icon Share
Abstract
With the increasing use of triploid fish for sport fisheries management, biologists, researchers, and managers working in field environments need practical methods to determine ploidy. Cytological methods (e.g., flow cytometry, Coulter counter) using erythrocytes are the most common for ploidy determination in fishes. However, collecting and storing erythrocytes can be logistically challenging during field work, and donor fish need to be alive or freshly killed. With rapid advances in molecular genetics, biologists, researchers, and managers may be unaware of molecular approaches for ploidy determination that could alleviate the difficulties associated with cytological methods and allow ploidy determination from archived samples (e.g., fin clips, scales, otoliths). In this study, we analyzed the agreement between molecular‐based (using fin tissue) and Coulter counter‐based (using blood) ploidy determinations for Walleyes Stizostedion vitreum—the first assessment of concordance between molecular and cytological methods for determining ploidy in the family Percidae. We found that agreement between these two methods was >98%. The high degree of agreement and greater ease of collecting and storing samples for molecular‐based approaches relative to the traditional cytological ones support the utility of molecular methods for ploidy determination.
Artificially induced triploidy is used in aquaculture worldwide to increase growth efficiency, improve flesh quality, and prevent unwanted reproductive development of cultured organisms (Piferrer et al. 2009; Zhou and Gui 2017). Interest in stocking sterile fish is rising among fisheries managers for situations where natural reproduction is undesirable (Budy et al. 2012; Farrell et al. 2022) and may even be required by state regulation (California Code of Regulations 2021). While there are emerging techniques for producing sterile fish that show great promise (Zohar 2021), inducing triploidy is one of the most common and practical methods for large‐scale production of sterile fish (Maxime 2008), especially in cases where interspecific hybrids are capable of successful reproduction (Bartley et al. 2001). For example, triploid Grass Carp Ctenopharyngodon idella are stocked outside their native range for vegetation control and triploid sport fish like Walleyes Stizostedion vitreum (Farrell et al. 2022), saugeyes (Walleye × Sauger S. canadense; Koch et al. 2018), and Cutthroat Trout Oncorhynchus clarkii (Cassinelli et al. 2019) are stocked to provide angling opportunities where reproductive and genetic containment are necessary to protect native fish stocks. As perfect induction of triploidy is rarely achieved (Fetherman et al. 2015), prestocking ploidy determinations are necessary to ensure that batches of fish with low induction rates are not released onto the landscape. Likewise, since ploidy is rarely visually identifiable (Maxime 2008), those interested in studying the effects of induced triploidy on life‐history traits and population dynamics must determine the ploidy of sampled individuals.
Flow cytometry is a cytological method for determining ploidy and is considered the “gold standard” among practitioners (Fiske et al. 2019; Hubálek and Flajšhans 2021), as it has been deemed the most accurate technique for determining ploidy in fish (Maxime 2008). Flow cytometry quantifies the relative nuclear DNA content of individual cells, typically erythrocytes in fishes, by measuring the fluorescence emitted by stained nuclear DNA (Allen 1983). Coulter counter analysis is another common cytological method for determining ploidy, which measures nuclear volume of erythrocytes as a proxy for nuclear DNA content (Wattendorf 1986) and is nearly as accurate as flow cytometry (Fiske et al. 2019). There are other ploidy determination methods (e.g., karyotyping, blood smears), but these may not be suitable when processing multiple samples, as most of these methods are time consuming (Fiske et al. 2019; Maxime 2008).
Using cytological methods to determine ploidy can be logistically challenging, especially when sampling fish in remote field locations. For example, when using erythrocytes for cytological ploidy determination, blood must be collected prior to postmortem clotting, as clotted blood makes ploidy determination difficult or impossible. Additionally, blood samples can rapidly deteriorate if not handled properly, must be stored with an anticoagulant (e.g., heparin), refrigerated, and analyzed 14–30 d postcollection. Extending the life of blood samples used for ploidy determinations is possible but requires the use of additional specialized methods (Brown et al. 2000; Jenkins and Thomas 2007; Hubálek and Flajšhans 2021). Furthermore, collecting blood samples requires additional equipment (i.e., syringes and needles, heparinized tubes) and skill on the part of the collector, and the procedure can be stressful to the fish. Fisheries managers, biologists, and researchers could benefit from alternative methods for determining ploidy from fish collected in the field.
Molecular‐based methods of ploidy determination are relatively new and offer an alternative approach that can overcome the difficulties associated with using cytological methods in the field while also providing additional genetic information that can be used for other analyses. Collection and storage of tissue samples for molecular‐based ploidy determination is relatively simple; epithelial tissue from a fin is a preferred source of DNA for extraction (Wandeler et al. 2007) and can be easily and nonlethally collected from a fish using only a clean pair of scissors (Pratt and Fox 2002). Properly stored tissue samples (e.g., stored in ethanol, frozen, or dried overnight at room temperature) have a shelf life measured in years to centuries rather than weeks (LaHood et al. 2008; Hubálek and Flajšhans 2021). While cytological approaches, especially when using erythrocytes, typically require fish in relatively good condition (i.e., alive or recently deceased), tissue samples used for molecular‐based approaches can be used on specimens that have been dead for decades (Wandeler et al. 2007), eliminating the concern of obtaining high‐quality samples from fish rapidly postmortem.
In this study we compared paired Coulter counter‐ and molecular‐based ploidy determinations for individual fish to test agreement between these two methods and to assess the utility of molecular‐based methods for determining ploidy in a percid. Samples (paired blood and fin tissue) for ploidy determination were collected from a population of mixed‐ploidy Walleyes in a southwestern Colorado reservoir within the upper Colorado River basin, where triploid Walleyes are stocked to satisfy local angler demand for high‐quality sport fisheries while minimizing the potential spread (natural and illegal) and establishment of nonnative piscivores into areas deemed important for endangered native fish recovery efforts (Farrell et al. 2022).
Methods
Fish Sampling
Narraguinnep Reservoir is a 215‐ha irrigation supply reservoir in southwest Colorado, USA. Colorado Parks and Wildlife (CPW) stocked diploid Walleyes in Narraguinnep on an irregular basis from as early as 1972 until 2004. In 2008, CPW began stocking triploid Walleyes into Narraguinnep Reservoir, resulting in a mixed population of diploid and triploid Walleyes (Farrell et al. 2022). Fish were collected using gill nets configured for fall Walleye index netting (FWIN; Morgan 2002) during spring in 2019 and spring and fall in 2020. Gill nets were set overnight during spring sampling and were set for 2–4 h during fall sampling. Water temperatures during both sampling periods were <12°C, and limited netting‐related mortality occurred. Fish condition (i.e., alive/dead) was noted prior to processing for tracking sample quality for Coulter counter analyses. Sampling procedures were approved by the Institutional Animal Care and Use Committee (Protocol #18‐7822A) at Colorado State University.
Sagittal otoliths were collected for age determination and cohort assignment. Because CPW began stocking triploid Walleyes in 2008 and Walleyes have been documented to reach >20 years old in this population (Farrell et al. 2022), fish born prior to 2008 could be classified as known diploids. While spontaneous polyploidy is rare in higher teleosts such as order Perciformes (Leggatt and Iwama 2003), fish born prior to 2008 were also screened for ploidy using Coulter counter analyses. Otoliths were sectioned transversely through the core and examined with a compound microscope using reflected light at 40–100× magnification. Ages were assigned, blind to fish length, three times by an experienced reader. When ages disagreed, the median integer age was used as the final age. Ploidy determinations were performed independently by different laboratories that were blind to fish age/cohort assignment.
Blood samples were collected with syringes via cardiac puncture immediately following euthanasia (Duman et al. 2019), stored in tubes coated with lithium heparin kept at 4°C until Coulter counter ploidy determinations could be performed by the Genomic Variation Laboratory at the University of California Davis. Coulter counter analyses were conducted 3–7 d postcollection. A Z2 Coulter Particle Count and Size Analyzer (Beckman Coulter Inc., Brea, California) was used for cytological ploidy determination (Fiske et al. 2019). One microliter of blood was pipetted from the lithium heparin tubes and placed into a 25‐mL cuvette containing 10 mL of Isoton II diluent and three drops of Zapoglobin II lytic reagent to measure the volume of the erythrocyte nuclei. Previous research indicated that nuclear volumes exhibit less variance than whole erythrocyte volumes and are more appropriate for ploidy determination (Fiske et al. 2019). Approximately 20,000 erythrocyte nuclei were measured for each sample, and the modal value of the nuclear volume was recorded (Fiske et al. 2019).
A 3‐cm2 fin clip was collected for genetic analysis from the lower lobe of the caudal fin using scissors and forceps that were sanitized with 70% ethanol (note: smaller tissue samples [e.g., <3 mm2] are likely still effective for genetic ploidy determination). Fin clips were dried at ambient temperature and stored on Whatman paper until genetic analyses could be completed by the Idaho Department of Fish and Game's Eagle Fish Genetics Laboratory. Samples were genotyped for a panel of 151 loci following the Genotyping‐in‐Thousands by sequencing method of amplicon sequencing (Campbell et al. 2015). These loci were a subsample of those described by Bootsma et al. (2020), which were previously found to be polymorphic within the first 79 bp in either this population of Walleyes or those present in Idaho (Idaho Department of Fish and Game, unpublished data). Reads were aligned to reference amplicon sequences using Bowtie2 (Langmead and Salzberg 2012) with parameters “‐‐rdg 0,5 ‐‐rfg 0,5 ‐‐score‐min L,0,‐0.76” to help account for high variability within the targeted loci. Read counts for each allele were extracted using microTyper (https://github.com/delomast/microTyper). To compare models of diploidy and triploidy, a critical log‐likelihood ratio (LLR) was calculated using the read counts for each single nucleotide polymorphism (SNP) allele with the method described by Delomas (2019) and implemented in the tripsAndDipR R package (https://github.com/delomast/tripsAndDipR). For loci with multiple SNPs (i.e., microhaplotypes), a maximum of one SNP per locus was used. In these cases, the SNP closest to the forward primer that passed the binomial test described by Delomas (2019) was selected. We genotyped 231 samples to use as a training set to establish LLRs used for determining ploidy from molecular data following the method of Delomas (2019). The LLRs of the training samples were manually assessed to determine critical values for discerning diploids from triploids. The critical value for diploidy was set at −5, as this value excluded all triploid training samples and aligned with critical values identified from similar panels in salmonids (Delomas 2019). The critical value for triploidy was set at 200, as this value excluded all diploid training samples. Fin tissue samples were categorized as either triploid (LLR ≥ 200), diploid (LLR ≤ −5) or ambiguous (200 > LLR > −5). We then used 118 samples, where Coulter counter‐based ploidy determinations were withheld from the genetics laboratory, as a test set to evaluate agreement between the two methods.
Statistical Analyses
All statistical analyses were performed using R 4.0.3 (R Development Core Team 2020). We used Cohen's kappa to assess agreement between the two ploidy determination methods (Cohen 1960). Cohen's kappa adjusts for chance agreement (van Stralen et al. 2012); values ≤0.2 indicate poor agreement, and values ≥0.9 indicate excellent agreement (Landis and Koch 1977; Byrt 1996). Cohen's kappa values were calculated using the irr package in R (Gamer et al. 2019). Figures were prepared using the package ggplot2 (Wickham 2016).
Results
Walleyes in this study ranged in size from 179 to 680 mm TL (Table S1 available in the Supplementary Material in the online version of this article). Diploids from the 1998 to 2020 cohorts and triploids from the 2011 to 2018 cohorts were present in the training and test sets. Because triploid Walleye stocking at Narraguinnep Reservoir began in 2008 and no triploids were assigned to cohorts born prior to 2011, diploid Walleyes belonging to the 1998–2007 cohorts were treated as individuals of known ploidy, which comprised 54 individuals in the training set and 7 individuals in the test set.
The mean modal nuclear volumes of erythrocytes estimated by Coulter counter for Walleyes in both the training and test sets were 12.4 μm3 (SD = 0.733 μm3) for diploids and 17.6 μm3 (SD = 0.952 μm3) for triploids (Figure 1A). Mean erythrocyte volume for triploid Walleyes was approximately 1.42× larger than their diploid conspecifics. Two Walleyes in the test set had nuclear volumes of 24.3 (age = 5) and 24.8 μm3 (age = 8), much larger than expected for a triploid Walleye (Figure 1A). Because the nuclear volume of erythrocytes for these two individuals was approximately 1.40× greater than measured in triploids, they were classified as putative tetraploids, as nuclear volume increases by 50% for each unit increase in ploidy level (Benfey 1999).
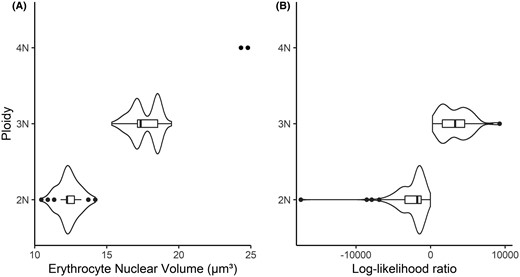
(A) Modal nuclear volume of Walleye erythrocytes measured with a Coulter counter. (B) Log‐likelihood ratios from genetics using read counts for each SNP allele with the method described by Delomas (2019). Training and test data sets included n = 349. 2N = diploid; 3N = triploid; 4N = tetraploid.
Calculated LLRs from molecular data had distinct distributions for triploid and diploid Walleyes. Log‐likelihood ratios were not normally distributed (Figure 1B); median LLRs were −1,795 (interquartile range = −3,426 to −1,261) for diploids and 3,297 (interquartile range = 1,591–4,587) for triploids.
After applying the critical LLR values established from the training set to the test set, we found 98.3% agreement between the molecular and cytological ploidy determinations. These separate methods disagreed for only two individuals (Table 1). These two individuals were those deemed tetraploid via the Coulter counter method (modal erythrocyte nuclear volumes: 24.3 and 24.8 μm3) but triploid via genetics (LLRs: 4,399 and 4,691). No triploids were misclassified as diploid, nor were any diploids misclassified as triploids. All individuals of known ploidy (i.e., diploids from cohorts born in 2007 and prior) were correctly assigned as diploids by both methods in both the training (n = 54) and test sets (n = 7). Cohen's kappa for the comparison of these two methods was 0.965 (95% CI = 0.917–1.00) for the test set, which indicated excellent agreement (Landis and Koch 1977; Byrt 1996).
Confusion matrix comparing the ploidy assignments by cytological (Coulter counter) and molecular (genetics) methods of ploidy determination in the test set (n = 118).
Molecular ploidy calls | |||
Cytological ploidy calls | 2N | 3N | 4N |
2N | 73 | 0 | 0 |
3N | 0 | 43 | 0 |
4N | 0 | 2 | 0 |
Molecular ploidy calls | |||
Cytological ploidy calls | 2N | 3N | 4N |
2N | 73 | 0 | 0 |
3N | 0 | 43 | 0 |
4N | 0 | 2 | 0 |
Confusion matrix comparing the ploidy assignments by cytological (Coulter counter) and molecular (genetics) methods of ploidy determination in the test set (n = 118).
Molecular ploidy calls | |||
Cytological ploidy calls | 2N | 3N | 4N |
2N | 73 | 0 | 0 |
3N | 0 | 43 | 0 |
4N | 0 | 2 | 0 |
Molecular ploidy calls | |||
Cytological ploidy calls | 2N | 3N | 4N |
2N | 73 | 0 | 0 |
3N | 0 | 43 | 0 |
4N | 0 | 2 | 0 |
Discussion
To our knowledge, this is the first study to examine agreement between cytological and molecular ploidy determination methods for a percid and to report distributions of erythrocyte nuclear volumes for diploid and triploid Walleyes. Molecular techniques have been successfully used to determine ploidy in White Sturgeon Acipenser transmontanus (Delomas et al. 2021), Atlantic Salmon Salmo salar (Glover et al. 2015; Jacq 2021), Brook Trout Salvelinus fontinalis, and Chinook Salmon Oncorhynchus tshawytscha (Delomas 2019). Our study further supports that molecular ploidy determination techniques work extremely well and give results indistinguishable from cytological methods. We found 98.3% agreement between molecular and cytological ploidy determinations. The two individuals with noncongruent ploidy assignments were called tetraploid by Coulter counter and triploid by amplicon sequencing. The amplicon sequencing analysis used here assumed all samples were either diploid or triploid, and so misclassification of a true tetraploid is expected. Alternative methods exist that can be used in situations where ploidies other than diploid and triploid are present (Gompert and Mock 2017; Weiß et al. 2018; Delomas et al. 2021), although a sufficient sample of known polyploids other than triploids would be needed to establish criteria for differentiating higher ploidy levels.
It is unlikely that tetraploids exist in the Narraguinnep Walleye population and more likely that the Coulter counter misclassified these triploids as tetraploids. Previous attempts to produce tetraploid finfish in species without multiple naturally occurring cytotypes have largely yielded tetraploid embryos that are inviable beyond the larval stages (Arai and Fujimoto 2018), with viable tetraploids being reported for only a few species (Chourrout et al. 1986; Nam et al. 2001). Induction of tetraploidy in Walleye has been demonstrated, but no tetraploids survived past the larval stage (Malison et al. 2001). Furthermore, it is unlikely that tetraploids were accidentally created during the induction process. Colorado Parks and Wildlife uses hydrostatic pressure shocks to induce triploidy in Walleyes by preventing the extrusion of the second polar body (Piferrer et al. 2009). The time of pressure initiation CPW uses for induction of triploidy is 8 min postfertilization (Fetherman et al. 2015). Malison et al. (2001) used hydrostatic pressure shocks to induce tetraploidy in Walleyes with a time of pressure initiation of 192 min postfertilization, which suggests that tetraploidy is unlikely to be inadvertently induced in Walleyes during triploid production. It is possible that the blood samples for the two fish classified as tetraploid by the Coulter counter were poorly handled in the field and clotting or cellular swelling occurred between collection and processing, causing the erythrocyte nuclei increase in size and appear as tetraploids (Krasznai and Goda 2021). Also, temperature fluctuations can shift estimated nuclear volumes (Schreier et al. 2021). However unlikely, the potential existence of adult tetraploid Walleyes suggested by the results of this study warrants confirmation and further investigation.
The choice of a ploidy determination method is situationally dependent as each comes with its own advantages and disadvantages. For example, cytological methods can cheaply and accurately measure percent triploidy in batches of larval fish (Jenkins et al. 2017). Also, cytological approaches generally do not require species‐specific development, whereas molecular methods require development of a genotyping panel for a given species if a suitable panel does not already exist. In cases where ploidy information is needed immediately (e.g., to inform stocking or production decisions), Coulter counter analysis can provide ploidy determination results more rapidly than molecular methods (Wattendorf 1986; Fiske et al. 2019). One sample takes only minutes when using the Coulter counter method but can take several hours when using a molecular approach.
However, field researchers and biologists often have other considerations, and a longer delay between sample collection and ploidy determination is acceptable. Fin clips can easily be stored (e.g., in a scale envelope or on Whatman paper), preserved by being dried at ambient temperature overnight and collected and analyzed at a time convenient for the researcher/biologist. The increased shelf life for molecular‐based ploidy determination can allow the researcher/biologist more time to collect more data on individuals (e.g., age) and save money by affording them the opportunity to be more selective with samples sent for ploidy determination. Tissue samples that were collected for other studies where ploidy determination was not the main goal could be used for retroactive analyses. Furthermore, samples can be archived for future studies of populations of mixed ploidy. Obtaining blood samples of satisfactory quality for cytological ploidy determinations requires fish in good condition (i.e., prior to postmortem blood clotting), which can preclude the use of certain fish collection techniques. For example, standardized gillnetting techniques like FWIN or North American netting can be used to estimate fish density but require soak times of 16–24 h (Giacomini et al. 2020). Such long soak times can preclude the use of cytological approaches to determine ploidy, as fish can be dead for far too long to obtain quality blood samples. Molecular ploidy determination methods enable the use of these types of approaches for monitoring and researching mixed diploid–triploid populations.
Researchers and managers can also obtain more information for a similar price by using molecular methods to determine ploidy. Cost does vary from lab to lab, but in our case, genetic analyses cost US$10.05/sample, while Coulter analyses cost $10.32/sample. Data obtained via cytological approaches are limited to estimations of DNA content, cellular and nuclear volumes, and ploidy. Alternatively, genetics data obtained for ploidy determination can be used for assessing population structure (Garza et al. 2014), parentage‐based tagging (Steele et al. 2013), estimating effective population size (Wang et al. 2016), estimating abundance using close‐kin mark–recapture (Bravington et al. 2016), or any of the myriad applications of genetics in fisheries (Carvalho and Pitcher 1995). Additionally, molecular approaches would be able to discriminate hybrids. For example, Montana Fish, Wildlife and Parks has stocked triploid Walleyes in Bighorn Reservoir (spanning the Montana–Wyoming border) since 2009 to reduce potential hybridization and introgression with native Saugers (Dalbey et al. 2016; S. Blackburn, Montana Fish, Wildlife and Parks, personal communication), and a molecular approach to ploidy determination would be able to identify triploid Walleyes and help discriminate between hybrid and pure Saugers. Moreover, tissues collected for DNA extraction can be used for other, nongenetic analyses. For example, fin clips have long been used in mark–recapture studies (VanDeValk et al. 2007), contaminant biomonitoring (Heltsley et al. 2005; Cerveny et al. 2016), and stable isotope analyses (Sanderson et al. 2009). Furthermore, it may be possible to use molecular‐based ploidy determination techniques for retrospective analyses of archived samples for which ploidy determination was not intended (Cuveliers et al. 2009; Price et al. 2019).
While molecular‐based methods are appealing from several standpoints, those collecting samples in the field must be aware that cross‐contamination is a greater concern than for cytological methods. While DNA sequencing is less sensitive to cross contamination than other molecular techniques, like environmental DNA (Rodgers 2017), it can occur and render samples useless. Practitioners should ensure that instruments are sufficiently cleaned between each sample that is collected.
Emerging concerns over stocking nonnative sportfish or native fish of different lineages than those in recipient systems in some western states and provinces has increased interest in the use of triploids as an alternative stocking strategy to mitigate potential problems associated with releasing diploid conspecifics onto the landscape, especially where there are concerns of their predatory (Farrell et al. 2022), competitive (Budy et al. 2012), or genetic (Koenig et al. 2011) impacts on native species. In addition to their use by Montana Fish, Wildlife and Parks in Bighorn Reservoir, triploid Walleyes are currently being used by the Utah Division of Wildlife Resources (UDWR) and CPW to provide alternative sport fisheries while mitigating the potential for Walleyes to establish themselves in the Colorado River and undermine endangered native fish recovery efforts, as required by a cooperative agreement among CPW, UDWR, Wyoming Game and Fish, and the U.S. Fish and Wildlife Service (USFWS 1996). This cooperative agreement prohibits the stocking of nonnative, nonsalmonid sport fish in the upper Colorado River basin, with a notable exception that grants state fisheries agencies the ability to stock sterile triploids (USFWS 1996). While the primary intention of stocking of triploid sport fish is to reduce the impact of natural dispersal, providing anglers opportunities to catch these sport fish may also reduce the extent of illegal stocking (Johnson et al. 2009). With growing interest in using triploid sport fish, other species may be triploidized and studies examining species‐specific impacts of triploidization on life‐history and population dynamics will be needed for fisheries management purposes. As such, managers, biologists, and researchers should be aware of alternative approaches to ploidy determination that may better suit their needs.
Acknowledgments
Funding for this study was provided by CPW. We thank Dr. Dan Schill, Dr. George Schisler, John Alves, Jeff Spohn, and Lori Martin for financial support and Jim White, Ryan Lane, Tyler Kersey, and several other CPW regional personnel for logistical support. Noah Angell, Adam Hundley, Lillian Legg, Michael Miller, Bill Pate, Joe Pitti, and Jesse Stokes assisted with fish collection in the field. Alisha Goodbla and Emily Funk assisted with and performed Coulter counter analyses. Kelly Heindel assisted with laboratory work. Montezuma Valley Irrigation Company provided access to the reservoir. We thank the anonymous reviewers whose constructive comments greatly improved this article. There is no conflict of interest declared in this article.