-
PDF
- Split View
-
Views
-
Cite
Cite
Justyn R. Maund, Morgan Fraser, Emma Reilly, Mattias Ergon, Seppo Mattila, Whatever happened to the progenitors of supernovae 2008cn, 2009kr and 2009md?, Monthly Notices of the Royal Astronomical Society, Volume 447, Issue 4, 11 March 2015, Pages 3207–3217, https://doi.org/10.1093/mnras/stu2658
- Share Icon Share
Abstract
We present new late-time, high-resolution observations of the sites of supernovae (SNe) 2008cn, 2009kr and 2009md, acquired with the Hubble Space Telescope. In all instances, significant flux from the SNe is still recovered at late times. We show that the previous identification of the progenitor of SN 2008cn was actually a blend of two sources, whose locations are resolved in these new observations. We suggest that the progenitor of SN 2008cn was actually a red supergiant with Minit < 16 M⊙. In the late-time observations of SN 2009kr, we find that the pre-explosion source (previously thought to be a yellow supergiant) is most probably a small compact cluster with mass ∼6000 M⊙. In late-time F814W observations of the site of SN 2009md, we find a single point source with identical brightness to the pre-explosion source, suggesting some caution in assuming that the pre-explosion source was the progenitor.
1 INTRODUCTION
All massive stars, above an initial mass of ∼8 M⊙, are expected to be sufficiently massive to end their lives with an Fe core and undergo a core-collapse-induced supernova (CCSN) explosion. Understanding the nature of the progenitors of CCSNe is crucial for testing both models of stellar and supernova (SN) evolution (for a review see Smartt 2009).
Since the identification of the red supergiant (RSG) progenitor of SN 2003gd in fortuitous pre-explosion images (Smartt et al. 2004), there has been success in identifying the progenitors for nearby CCSNe. The vast majority of identified progenitors have been RSG precursors to Type IIP SNe and, in some cases, possibly yellow supergiant (YSG) stars (Aldering, Humphreys & Richmond 1994; Elias-Rosa et al. 2009, 2010; Fraser et al. 2010; Maund et al. 2011; Van Dyk et al. 2011), in particular associated with hydrogen-poor Type IIb SNe. Conversely, there has been no confirmed success in detecting the progenitor of Type Ibc SNe (Eldridge et al. 2013), although the progenitor of iPTF13bvn remains a candidate for a possible detection (Cao et al. 2013).
Once an SN has faded, a new window on the progenitor is revealed through late-time observations. At the most basic level, late-time observations of the sites of SNe with pre-explosion imaging are crucial for confirming, through their disappearance, that previously identified progenitor candidates were in fact the progenitors (e.g. Maund & Smartt 2009). Such late-time observations permit enhanced levels of analysis, in particular through better astrometry and significantly better photometric accuracy (Maund et al. 2014b). As the pre-explosion observations used for the direct observations of the progenitors of CCSNe have been, principally, fortuitous in nature, the late-time observations provide the opportunity to acquire tailor-made, deep observations of the SN sites and, through the application of image subtraction techniques, improve the photometric accuracy, in particular reducing systematic errors due to flux contamination. Maund & Smartt (2009) and Maund, Reilly & Mattila (2014a) confirmed the disappearance of five progenitors of Type IIP SNe, and using image subtraction and Bayesian spectral energy distribution (SED) fitting techniques were able to derive confident initial masses for these progenitors. Importantly, in the case of SN 1999ev, for which a progenitor candidate had been previously identified by Maund & Smartt (2005), Maund et al. (2014a) showed that an object in late-time observations of the SN site had the same brightness as the pre-explosion source, demonstrating that pre-explosion source could not have been the progenitor. In addition, the progenitors of two Type IIb SNe have also been confirmed to have disappeared (Maund & Smartt 2009; Van Dyk et al. 2013; Ergon et al. 2014).
Here, we report new late-time Hubble Space Telescope (HST) observations of the sites of three Type II SNe, with progenitor candidates identified in fortuitous pre-explosion images: SNe 2008cn, 2009kr and 2009md.
The paper is structured as follows: in Section 2, we present the new late-time HST observations and a re-reduction of the previously analysed pre-explosion observations. We report our results and analysis of the data for each SN in Section 3 and discuss these results in Section 4. We report our conclusions in Section 5.
2 OBSERVATIONS AND DATA REDUCTION
The sites of SNe 2008cn, 2009kr and 2009md were observed prior to explosion with the HST Wide Field Planetary Camera 2 (WFPC2). Details of these observations are presented in Table 1. The pre-explosion observations of these SNe were acquired under programmes GO-6439 and GO-7507 (PI: Zepf) for SN 2008cn, SNAP-10877 (PI: Li) for SN 2009kr and GO-10413 (PI: Gregg) for SN 2009md.
Pre-explosion and late-time HST observations of the sites of SNe 2008cn, 2009kr and 2009md.
Date . | Instrument . | Filter . | Exposure . |
---|---|---|---|
(ut) . | . | . | time(s) . |
SN 2008cn | |||
1996 May 27.5 | WFPC2/WF2 | F555W | 7400a |
1996 Jun 01.4 | WFPC2/WF2 | F555W | 7400a |
1996 Jun 08.3 | WFPC2/WF2 | F555W | 7400a |
1996 Jun 14.1 | WFPC2/WF2 | F555W | 7400a |
1996 Jun 21.0 | WFPC2/WF2 | F555W | 7400a |
1996 Jun 28.8 | WFPC2/WF2 | F555W | 7400a |
1996 Jul 07.4 | WFPC2/WF2 | F555W | 4800b |
1997 Jun 17.5 | WFPC2/WF2 | F814W | 7400a |
WFPC2/WF2 | F555W | 4800b | |
1997 Jul 17.9 | WFPC2/WF2 | F814W | 7400a |
WFPC2/WF2 | F555W | 4800b | |
2011 May 29.0 | ACS/WFC | F435W | 1060 |
ACS/WFC | F555W | 1860 | |
ACS/WFC | F814W | 1585 | |
SN 2009kr | |||
2008 Jan 11.3 | WFPC2/WF3 | F555W | 460 |
WFPC2/WF3 | F814W | 700 | |
2012 Oct 26.3 | ACS/WFC | F435W | 1080 |
ACS/WFC | F555W | 1700 | |
ACS/WFC | F814W | 1536 | |
SN 2009md | |||
2005 Mar 14.3 | WFPC2/WF2 | F606W | 4900c |
WFPC2/WF2 | F814W | 4700c | |
2005 May 20.8 | WFPC2/WF2 | F606W | 5200c |
WFPC2/WF2 | F814W | 5200c | |
2012 Nov 08.1 | ACS/WFC | F435W | 1072 |
ACS/WFC | F555W | 1700 | |
ACS/WFC | F814W | 1536 |
Date . | Instrument . | Filter . | Exposure . |
---|---|---|---|
(ut) . | . | . | time(s) . |
SN 2008cn | |||
1996 May 27.5 | WFPC2/WF2 | F555W | 7400a |
1996 Jun 01.4 | WFPC2/WF2 | F555W | 7400a |
1996 Jun 08.3 | WFPC2/WF2 | F555W | 7400a |
1996 Jun 14.1 | WFPC2/WF2 | F555W | 7400a |
1996 Jun 21.0 | WFPC2/WF2 | F555W | 7400a |
1996 Jun 28.8 | WFPC2/WF2 | F555W | 7400a |
1996 Jul 07.4 | WFPC2/WF2 | F555W | 4800b |
1997 Jun 17.5 | WFPC2/WF2 | F814W | 7400a |
WFPC2/WF2 | F555W | 4800b | |
1997 Jul 17.9 | WFPC2/WF2 | F814W | 7400a |
WFPC2/WF2 | F555W | 4800b | |
2011 May 29.0 | ACS/WFC | F435W | 1060 |
ACS/WFC | F555W | 1860 | |
ACS/WFC | F814W | 1585 | |
SN 2009kr | |||
2008 Jan 11.3 | WFPC2/WF3 | F555W | 460 |
WFPC2/WF3 | F814W | 700 | |
2012 Oct 26.3 | ACS/WFC | F435W | 1080 |
ACS/WFC | F555W | 1700 | |
ACS/WFC | F814W | 1536 | |
SN 2009md | |||
2005 Mar 14.3 | WFPC2/WF2 | F606W | 4900c |
WFPC2/WF2 | F814W | 4700c | |
2005 May 20.8 | WFPC2/WF2 | F606W | 5200c |
WFPC2/WF2 | F814W | 5200c | |
2012 Nov 08.1 | ACS/WFC | F435W | 1072 |
ACS/WFC | F555W | 1700 | |
ACS/WFC | F814W | 1536 |
Notes.a3 × 2 exposures.
b2 × 2 exposures.
c4 exposures.
Pre-explosion and late-time HST observations of the sites of SNe 2008cn, 2009kr and 2009md.
Date . | Instrument . | Filter . | Exposure . |
---|---|---|---|
(ut) . | . | . | time(s) . |
SN 2008cn | |||
1996 May 27.5 | WFPC2/WF2 | F555W | 7400a |
1996 Jun 01.4 | WFPC2/WF2 | F555W | 7400a |
1996 Jun 08.3 | WFPC2/WF2 | F555W | 7400a |
1996 Jun 14.1 | WFPC2/WF2 | F555W | 7400a |
1996 Jun 21.0 | WFPC2/WF2 | F555W | 7400a |
1996 Jun 28.8 | WFPC2/WF2 | F555W | 7400a |
1996 Jul 07.4 | WFPC2/WF2 | F555W | 4800b |
1997 Jun 17.5 | WFPC2/WF2 | F814W | 7400a |
WFPC2/WF2 | F555W | 4800b | |
1997 Jul 17.9 | WFPC2/WF2 | F814W | 7400a |
WFPC2/WF2 | F555W | 4800b | |
2011 May 29.0 | ACS/WFC | F435W | 1060 |
ACS/WFC | F555W | 1860 | |
ACS/WFC | F814W | 1585 | |
SN 2009kr | |||
2008 Jan 11.3 | WFPC2/WF3 | F555W | 460 |
WFPC2/WF3 | F814W | 700 | |
2012 Oct 26.3 | ACS/WFC | F435W | 1080 |
ACS/WFC | F555W | 1700 | |
ACS/WFC | F814W | 1536 | |
SN 2009md | |||
2005 Mar 14.3 | WFPC2/WF2 | F606W | 4900c |
WFPC2/WF2 | F814W | 4700c | |
2005 May 20.8 | WFPC2/WF2 | F606W | 5200c |
WFPC2/WF2 | F814W | 5200c | |
2012 Nov 08.1 | ACS/WFC | F435W | 1072 |
ACS/WFC | F555W | 1700 | |
ACS/WFC | F814W | 1536 |
Date . | Instrument . | Filter . | Exposure . |
---|---|---|---|
(ut) . | . | . | time(s) . |
SN 2008cn | |||
1996 May 27.5 | WFPC2/WF2 | F555W | 7400a |
1996 Jun 01.4 | WFPC2/WF2 | F555W | 7400a |
1996 Jun 08.3 | WFPC2/WF2 | F555W | 7400a |
1996 Jun 14.1 | WFPC2/WF2 | F555W | 7400a |
1996 Jun 21.0 | WFPC2/WF2 | F555W | 7400a |
1996 Jun 28.8 | WFPC2/WF2 | F555W | 7400a |
1996 Jul 07.4 | WFPC2/WF2 | F555W | 4800b |
1997 Jun 17.5 | WFPC2/WF2 | F814W | 7400a |
WFPC2/WF2 | F555W | 4800b | |
1997 Jul 17.9 | WFPC2/WF2 | F814W | 7400a |
WFPC2/WF2 | F555W | 4800b | |
2011 May 29.0 | ACS/WFC | F435W | 1060 |
ACS/WFC | F555W | 1860 | |
ACS/WFC | F814W | 1585 | |
SN 2009kr | |||
2008 Jan 11.3 | WFPC2/WF3 | F555W | 460 |
WFPC2/WF3 | F814W | 700 | |
2012 Oct 26.3 | ACS/WFC | F435W | 1080 |
ACS/WFC | F555W | 1700 | |
ACS/WFC | F814W | 1536 | |
SN 2009md | |||
2005 Mar 14.3 | WFPC2/WF2 | F606W | 4900c |
WFPC2/WF2 | F814W | 4700c | |
2005 May 20.8 | WFPC2/WF2 | F606W | 5200c |
WFPC2/WF2 | F814W | 5200c | |
2012 Nov 08.1 | ACS/WFC | F435W | 1072 |
ACS/WFC | F555W | 1700 | |
ACS/WFC | F814W | 1536 |
Notes.a3 × 2 exposures.
b2 × 2 exposures.
c4 exposures.
The data were retrieved from the Space Telescope Science Institute archive,1 having been processed with the latest calibrations as part of the On-the-Fly recalibration pipeline. For the purposes of comparisons with late-time observations (see below), the images for each SN, in each filter, were corrected for geometric distortion and cosmic rays, before being combined using the astrodrizzle package2 running in the pyraf environment.3 The positions of SNe 2008cn and 2009md fell on the Wide Field (WF) 2 chip, while the site SN 2009kr was imaged on the WF3 chip prior to explosion.
For all SNe, the pre-explosion WFPC2 observations were drizzled to final pixel scales of 0.1 arcsec, matching the pixel scale of the original individual exposures. The pre-explosion WFPC2 observations of SN 2008cn were composed of nine separate epochs of F555W imaging and two epochs of F814W imaging. The observations were acquired in groups of either three or two pairs of images, each pair allowing for cosmic ray rejection and each pair being acquired at slightly different pointings in a linear pattern. For the purposes of image subtraction (see below), for each epoch of pre-explosion observations of SN 2008cn, master drizzled images were constructed. In the case of SN 2009kr, the two pre-explosion exposures in each filter were conducted at the same pointing, such that the final image could not be drizzled to a finer pixel scale. While the two sets of pre-explosion observations of the site of SN 2009md were composed of four dithered exposures, the spacing was insufficient to achieve a finer final pixel scale for the images on the WF chips. The two sets of pre-explosion observations for SN 2009md were not combined, and were considered separately in our analysis. Photometry of the WFPC2 observations was conducted using the dolphot package (Dolphin 2000), with the specific WFPC2 module.4 Pairs of observations taken at the same pointing were used for the rejection of cosmic rays and were then co-added.
Late-time observations of the site of SN 2008cn were acquired with the HST Advanced Camera for Surveys (ACS) Wide Field Channel (WFC), as part of programme GO-12262 (PI: Maund). The late-time observations of the sites of SNe 2009kr and 2009md were acquired with the HST ACS WFC as part of programme GO-12559 (PI: Maund). ACS/WFC was selected due to the unavailability of WFPC2, and the similarities between the F555W and F814W filters of the two instruments. The observation strategy was identical for all SNe, with the observations for each filter being composed of four separate exposures using the 1024 × 1024 subarray, read through the ‘B’ amplifier. The exposures were acquired in a box dither pattern, and were combined with astrodrizzle to a final pixel scale of 0.025 arcsec (compared to the original pixel scale of 0.05 arcsec). Photometry of the late-time-drizzled, combined images was conducted using the point-spread function (PSF) fitting photometry routines of daophot (Stetson 1987), with the latest zero-points appropriate for the epochs of the two observations5 and corrections for charge transfer inefficiency (CTI) provided by Chiaberge et al. (2009).
The positions of SNe 2008cn, 2009kr and 2009md on their respective pre-explosion and late-time observations were found in conjunction with high-resolution adaptive optics (AO) post-explosion images, acquired with the European Southern Observatory Very Large Telescope (VLT) Nasmyth Adaptive Optics System and High-Resolution Near-Infrared Camera (NACO) and Gemini-N Near Infrared Imager and Altitude Conjugate Adaptive Optics for the Infrared (NIRI+ALTAIR), soon after the SNe explosions.
SN 2008cn was observed with VLT/NACO (Rousset et al. 2003) using Target of Opportunity observations as a part of programme 081.D-0279 (PI: Mattila) on 2008 June 29. The imaging was carried out in the Ks band with the S27 camera (0.027 arcsec pixel−1) using the AutoJitter imaging sequence. The AO correction was performed using the visual wavefront sensor with the SN (mV ∼ 16.5) itself as a natural guide star. The NACO data were reduced using iraf. The jittered on-source frames were median-combined to form a sky frame, the sky-subtracted images de-dithered making use of the centroid coordinates of the SN, and the de-dithered frames median-combined. For SNe 2009kr and 2009md, the post-explosion observations were previously presented by Fraser et al. (2010) and Fraser et al. (2011), respectively.
We also utilized the isis image subtraction package (Alard & Lupton 1998; Alard 2000), with the deeper late-time images (transformed to match the coordinate system of the pre-explosion observations) as the reference images, to establish the change in brightness between the pre-explosion and late-time sources. Following Maund & Smartt (2009) and Maund et al. (2014a), we utilized the aperture photometry output from isis, with zero-points and aperture corrections derived from reference stars (with measured photometry) in the late-time ACS observations. Systematic uncertainties were determined by conducting multiple iterations of the image subtraction process, with the isis parameters varied. Further corrections for the CTI in photometric system of the pre-explosion WFPC2 images were calculated following the prescription presented by Maund et al. (2014a).
3 RESULTS AND ANALYSIS
3.1 SN 2008cn
SN 2008cn was discovered by R. Martin in the galaxy NGC 4603 on 2008 May 21.5 (Martin, Monard & Africa 2008). Stritzinger & Morrell (2008) subsequently classified the SN as being a Type II SN, with further monitoring by Elias-Rosa et al. (2009) confirming it to be a Type IIP SN. Li et al. (2008) claimed the probable detection of an RSG coincident with the SN position, which Elias-Rosa et al. (2009) later suggested was a YSG with initial mass Minit = 15 ± 2 M⊙. While Elias-Rosa et al. suggested that the pre-explosion source was possibly a composite of early- and late-type supergiants, they ruled out the source being an extended cluster. Elias-Rosa et al. adopted a Cepheid distance of μ = 32.61 ± 0.10 mag (Newman et al. 1999), which we adopt here.
The post-explosion VLT NACO image of SN 2008cn was used to identify the position of the SN in the late-time ACS/WFC F814W image, using 16 common stars. The SN position was identified with a precision of 0.014 arcsec (or 0.56 drizzled ACS/WFC pixels). Small shifts between the late-time F435W and F555W images and the F814W image were determined using cross-correlation techniques. A further geometric transformation between the late-time F814W image and the pre-explosion F555W image, acquired at the first epoch, was calculated using 29 common stars, permitting us to locate the SN position to within 0.033 arcsec (or 0.33 WF pixels). As with the late-time images, cross-correlation techniques were then used to calculate the corresponding shifts between all of the pre-explosion observations (F555W and F814W) with respect to the first epoch F555W image (which was used as the astrometric reference for all pre-explosion WFPC2 images). The position of SN 2008cn in the pre-explosion and late-time observations is presented in Fig. 1 .
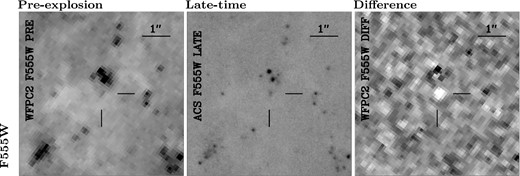
Pre-explosion WFPC2 and late-time ACS F555W observations of the site of SN 2008cn. The difference between the two epochs is shown in the right-hand panel. A positive residual in the difference image corresponds to a source that is brighter in the late-time image. A number of minor negative residuals in the difference image correspond to clusters, for which the image subtraction process (calculated assuming point-like sources) introduced inaccuracies. Each panel has dimensions 6 arcsec × 6 arcsec and aligned such that north is up and east is left.
In the late-time observations, a bright source is significantly recovered in all bands in close proximity to the transformed SN position. The brightness of the late-time source was measured to be mF435W = 26.70 ± 0.14, mF555W = 25.50 ± 0.08 and mF814W = 24.72 ± 0.09 mag. Despite the precision of the transformation between the post-explosion VLT NACO image and the late-time F814W image, we measured apparent offsets between the transformed SN position and the late-time source of 2.3, 1.4 and 0.93 pixels (corresponding to 0.058, 0.035 and 0.023 arcsec), for the F435W, F555W and F814W images, respectively, suggesting that the sources recovered in each of the bands are not the same source. From the daophot photometry, we found the late-time sources to be extended with sharpness values >0.5 (whereas point sources should have values <0.3), with the broadest profile observed in the F555W frame.
In the pre-explosion observations, we find a source in close proximity to the SN position; this object was previously identified by Elias-Rosa et al. (2009) as the possible (or candidate) progenitor. Using dolphot, we measure the average brightness of this source over all epochs of mF555W = 26.56 ± 0.05 and mF814W = 25.23 ± 0.07 mag (the nature of these measurements is discussed in more detail below). The slight shifts in the pointings between the pre-explosion observations at each of the epochs (and hence the shifts in the positions of the pixels with respect to the position of the source) resulted in slight changes in the apparent offset between the source position and the transformed SN position (in the range 0.47–1.83 pixels). Even the minimum observed offset is larger than the 1σ uncertainty on the transformed SN position on the pre-explosion images. As the pixels of the pre-explosion observations are four times larger than those of the late-time images, it is only in conjunction with the late-time observations that the true separation between the SN position and the nearby pre-explosion source becomes apparent.
The differences between the positions of the sources recovered in the pre-explosion and late-time observations are presented in Fig. 2. Using a linear interpolation scheme, contours were calculated for the pre-explosion image to illustrate the approximate flux weighting between the central pixel containing the bulk of the pre-explosion source flux and the surrounding pixels (analogous to identifying the position of a source through centroiding). While some caution is required in the interpretation of Fig. 2, due to the interpolation of counts between pixels, the figure illustrates the relative offsets between the late-time source and pre-explosion source in certain filters. It is evident that the late-time F435W source is coincident with the source as observed in the pre-explosion F555W image, but is offset from the transformed SN position. The position of the extended late-time F555W source is approximately mid-way between the transformed SN position and the pre-explosion observations, although the separation between the two positions is less than the full width at half-maximum (FWHM) of 1.9 pixels in the drizzled pre-explosion image. Due to their proximity and the relatively low S/N at which the late-time F555W source was detected, it was not possible to accurately partition the flux between the two positions using daophot. The pre-explosion and late-time F814W sources appear coincident with the transformed SN position.
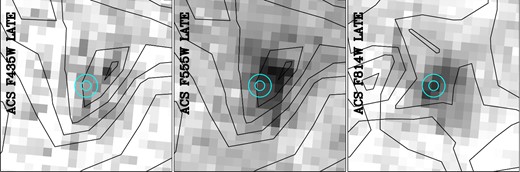
Detailed comparison of the site of SN 2008cn in the late-time (grey-scale) and pre-explosion (contours) images. In the middle and right-hand panels, the pre-explosion and late-time F555W and F814W observations are paired together, and in the left-hand panel, the late-time F435W image is overlaid with contours corresponding to the pre-explosion F555W image. The pre-explosion image contours were calculated at 10 count intervals using an linear interpolation scheme. Each panel has dimensions 0.5 arcsec × 0.5 arcsec and is centred on the transformed SN position, with the corresponding circles indicating the positional uncertainties on the SN position in the late-time (small circle) and pre-explosion (large circle) observations.
We propose that the pre-explosion F814W photometry is dominated by an object at the SN position (the progenitor), while the pre-explosion F555W source is significantly offset from the SN position. This implies that the sources identified in the pre-explosion F555W and the pre-explosion F814W images are not the same object. The apparent offset of the F555W pre-explosion source from the transformed SN position (determined from the late-time ACS observations) corresponds to ∼10pc.
In their analysis of the pre-explosion WFPC2 observations of SN 2008cn, Elias-Rosa et al. (2009) suggested a possible sinusoidal variation in the F555W brightness over the available pre-explosion epochs. We analysed the pre-explosion WFPC2 observations at each epoch, conducting dolphot photometry on each pair of images at each epoch (i.e. such that at each epoch there were two or three independent photometric measurements). The results of this photometry (for each pair of images and also an average for each epoch) on the sequence of pre-explosion F555W images are presented in Fig. 3. We do not recover the sinusoidal variation reported by Elias-Rosa et al., instead seeing a scatter of measurements consistent with the photometric uncertainties. The average brightness over all the pre-explosion photometric measurements are presented above as our estimate of the pre-explosion brightness of the source.
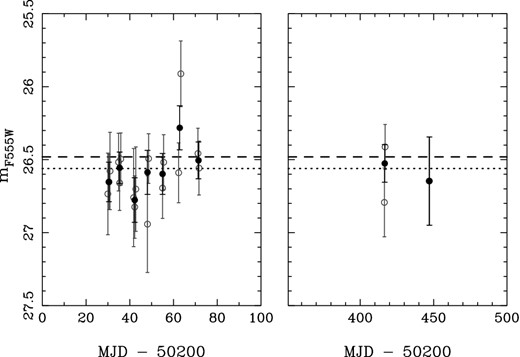
The WFPC2 F555W light curve of the pre-explosion source associated with SN 2008cn for the two groups of pre-explosion observations listed in Table 1. Open points and error bars in grey are the direct photometric measurements (conducted using dolphot) for each pair of exposures with the same pointing acquired at the same epoch (with shifts of −0.5, 0, +0.5 d used to separate measurements for clarity in instances where three pairs of exposure were acquired, or −0.5, +0.5 d when two pairs were available). Filled points indicate the weighted average F555W photometry at each epoch. The dotted horizontal line indicates the average of all the dolphot measurements, while the dashed grey line is the weighted average photometry derived using the late-time F555W image and image subtraction techniques.
In addition, for each pair of pre-explosion images, we also constructed combined frames using the iraf task crrej (running as part of stsdas), and used these with the isis image subtraction package (see Fig. 1) and the late-time F555W and F814W images to acquire photometry of the pre-explosion source (with respect to the flux deficit in the difference image and our photometry of the late-time source). In general, the isisF555W photometry was found to agree with the results from the direct photometry on the pre-explosion images using dolphot, producing a final brightness of mF555W = 26.48 ± 0.08, slightly brighter than the average value derived from direct photometry of the pre-explosion images, quoted above. Similarly, using isis, we derived mF814W = 25.13 ± 0.09.
Given the distance reported for NGC 4603 (see above), we can estimate an initial mass for the progenitor. In Fig. 4, we show the luminosities calculated for MARCS model SEDs (Gustafsson et al. 2008) for RSGs with log g = 0.00 and solar metallicity, assuming E(B − V) = 0.35 ± 0.04 (Elias-Rosa et al. 2009). Assuming that the temperature of the progenitor is log T > 3.5 (i.e. it is not a luminous super asymptotic giant branch star), we find the mass of the progenitor to be Minit ∼ 16 M⊙, if all of the pre-explosion F814W flux arises from the progenitor. If the pre-explosion F814W flux is a blend of the progenitor flux with that of a nearby source this mass estimate corresponds to an upper mass limit (see Fig. 4). This mass limit is consistent with one of the progenitor scenarios proposed by Elias-Rosa et al. (2009), who suggested that if the pre-explosion source was a blend it could possibly contain an RSG with Minit ∼ 15 M⊙.
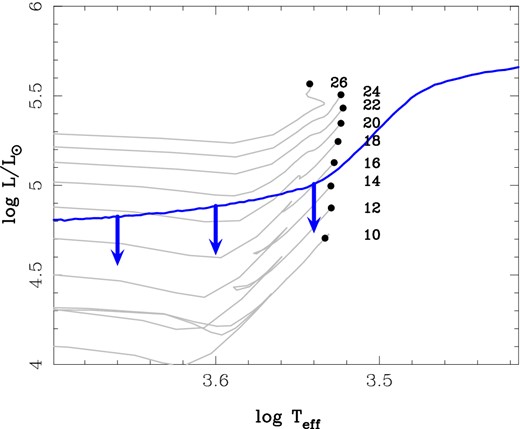
The luminosity constraint for the progenitor of SN 2008cn derived from the pre-explosion F814W photometry. The upper luminosity limit on the progenitor is shown in blue, assuming a distance modulus of μ = 32.61 ± 0.10 for NGC 4603.
3.2 SN 2009kr
SN 2009kr was discovered by K. Itagaki at 2009 Nov 6.7, in the galaxy NGC 1832 (Nakano, Yusa & Kadota 2009a). Early spectroscopy (2009 Nov 9), revealed weak Balmer lines and a blue continuum, consistent with a young Type-II SN (Steele, Cobb & Filippenko 2009a). Further subclassification, based on the photometric evolution of the SN, was ambiguous with Elias-Rosa et al. (2010) and Arcavi et al. (2012) concluding SN 2009kr was a Type IIL SN, while Fraser et al. (2010) reported a short light-curve plateau consistent with a Type IIP SN. Using natural seeing post-explosion images, Li et al. (2009) identified a possible progenitor in HST WFPC2 F555W and F814W images. The brightness and relatively blue colour suggested that the progenitor was either a single massive YSG or a compact cluster. Later analyses of the same WFPC2 data by Elias-Rosa et al. (2010) and Fraser et al. (2010) concluded the progenitor was a YSG, with initial masses reported as 18–24 M⊙ and |$15^{+5}_{-4}\,\mathrm{M}_{{\odot }}$|, respectively. Most recently, Kuncarayakti et al. (2013) used integral field spectroscopy of a cluster adjacent to the position of SN 2009kr to determine an oxygen abundance of 8.61, corresponding to 0.89 Z⊙ (assuming a solar oxygen abundance of 8.66; Asplund et al. 2004). We adopt the distance to NGC 1832 of μ = 32.09 ± 0.15, used by Fraser et al. (2010), and adopt a solar metallicity.
The position of SN 2009kr in the pre-explosion and late-time observations is shown on Fig. 5. The exact location was identified on the pre-explosion and late-time images to within 0.040 and 0.019 arcsec, respectively, with reference to a post-explosion VLT NACO observation previously presented by Fraser et al. (2010). In the pre-explosion images, we identify the same star identified as the progenitor by Fraser et al. (2010) and Elias-Rosa et al. (2010), and we measure the brightness of this source to be mF555W = 24.51 ± 0.11 and mF814W = 23.40 ± 0.08 mag. In both filters, the pre-explosion source is consistent with a single point source.
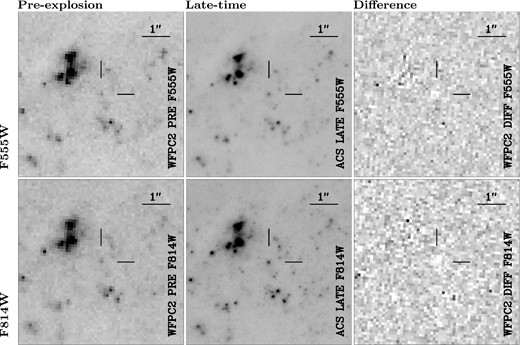
Pre-explosion WFPC2 and late-time ACS F555W and F814W observations of the site of SN 2009kr. The difference between the two epochs, for both filters, is shown in the right-hand panels of each row. Positive residuals in the difference image correspond to sources that are brighter in the late-time image.
In the late-time images, a source is clearly recovered at the SN position at all wavelengths. The offset between the transformed SN position and the position of the late-time source is only 0.011 pixels, less than the uncertainty on the geometric transformation. The results from the PSF fitting photometry suggest that the source is slightly extended with an FWHM approximately 40 per cent larger than point sources observed in the same image, and with χ2 = 1.8 and a sharpness value =0.6. We used the ishape package (Larsen 1999) to probe the spatial properties of the late-time source, assuming the underlying profile of the source could be approximated by a Moffat function with order 2.5. At all wavelengths, the convolved Moffat profile provided a better fit to the source than a convolved delta function, with effective radii corresponding to 3.3, 4.8 and 2.5 pc in the F435W, F555W and F814W images, respectively. Some caution is required in the interpretation of these radii, however, as the sensitivity of ishape is necessarily dependent on the signal-to-noise ratio (S/N) of the source being analysed. Because of the apparent extended nature of the late-time source in both the ishape and daophot analyses, the correct determination of the brightness of this source required the subtraction of nearby stars (within a radius of 40 pixels) before aperture photometry of the isolated source was conducted. Aperture photometry of the late-time source at the SN position was conducted with an aperture size of 0.5 arcsec, which was corrected to an infinite aperture using the values of Sirianni et al. 2005, yielding mF435W = 24.36 ± 0.04, mF555W = 23.96 ± 0.07 and mF814W = 23.08 ± 0.06 mag.
In the two filters shared between the pre-explosion and late-time observations, F555W and F814W, the late-time source is brighter than the pre-explosion source, observed as a bright residual in the difference images (see Fig. 5). The excess brightness may arise from the still fading SN, which would suggest some caution is required in interpreting the ishape analysis as the late-time source might be a combination of a point source PSF (the SN) and a broad, resolved component (a cluster). Although the residuals observed in the difference images have relatively low S/N, due to the small difference between the pre-explosion and late-time photometry, the centroids of the position of the residuals, for both filters, are coincident (within the uncertainties of the geometric transformation) with the transformed position of the SN and, consequently, the position of the progenitor candidate in the pre-explosion and late-time images.
The difference between the pre-explosion and late-time photometry was determined to correspond to mF555W = 25.36 ± 0.06 and mF814W = 24.52 ± 0.07 mag, which may correspond to late-time flux originating from the fading SN. Fraser et al. (2010) and Elias-Rosa et al. (2010) showed the early evolution of the light curve, over the first ∼100 d since discovery. Although initially showing a shallow decline from maximum light, the light curve exhibited a dramatic decrease in brightness at ∼85 d, reaching V ≈ 19.4. As the late-time observations of SN 2009kr were acquired 1084-d post-discovery, if the excess brightness were due to the fading SN these would correspond to very shallow decline rates of ∼0.006 and ∼0.007 mag d−1; compared to the V- and I-band light curves presented by Elias-Rosa et al. (2010).
Given the similarity in brightness between the pre-explosion and late-time photometry and the apparent extended nature of the source, we propose that the pre-explosion source was most likely a cluster, rather than a single star, that persists in the late-time images (with some additional flux from the fading SN).
As the currently available late-time observations of SN 2009kr suggest that the previously identified progenitor candidate is a compact cluster, we considered the pre-explosion photometry (without the additional contaminating flux) against starburst99 models (Leitherer et al. 1999), assuming RV = 3.1, solar metallicity and a single, initial burst of star formation. Although the degree of flux contamination, at late-times, can be derived for the F555W and F814W observations, through comparison with the pre-explosion observations, the late-time F435W can only be used to provide an upper limit on the probable cluster brightness at that wavelength. The portion of the age-reddening parameter space constrained by the pre-explosion and late-time observations was computed using our own Bayesian SED-fitting analysis routines (Maund, in preparation) and is shown in Fig. 6. Due to limited constraints on the SED provided by the F435W upper limit, it is not possible to determine a unique solution and there is some degeneracy between the reddening and age that can be roughly categorized into two distinct groups: (1) low reddening (0 < E(B − V) < 0.7) with an ‘old’ stellar population (t > 7.8 Myr) and (2) a younger population (t < 7.8 Myr) with high reddening (E(B − V) > 0.7). As can be seen from the bottom panel of Fig. 6, the degeneracy arises due to the need, for young SEDs, to compensate for the steep blue slope of the intrinsic SED with large amounts of reddening to accommodate the relatively shallow F555W–F814W colour.
![Top panel: age-reddening solutions for the pre-explosion and late-time observations of the probable compact cluster host of SN 2009kr, through comparison with starburst99 models and assuming RV = 3.1. The inner and outer contours contain 68 and 95 per cent of the total probability. The horizontal dashed line indicates the lifetime [3.2 × 107yr; for Padova isochrones (Girardi et al. 2000), used in the calculation of the starburst99 models] of a star with initial mass Minit = 8 M⊙ (i.e. the expected maximum lifetime for a star to end its life through a CCSN explosion). Bottom panel: selected example SED solutions to fits for the cluster SED, for age and reddening (according to the legend in top-right corner of the panel), drawn from the top panel.](https://oup.silverchair-cdn.com/oup/backfile/Content_public/Journal/mnras/447/4/10.1093/mnras/stu2658/3/m_stu2658fig6.jpeg?Expires=1750231925&Signature=yJaqTuGavdcxKDunEG3PzMbD3UK0cCqJJTB-8XACpA4zE1d03xwcVkcyEVCLrzKdCtQN2FenbOmjv~Zsc-9JxJxoX5HAB~4yoNI-WYHO85HUN1pJSIjeic7t-o~kd8cSOv-keF1gP9~adIl667iK0m4kSsNM7su-nb8y~65eA5RwcxDpSXNVXZuUhLiSOdylG6DhaQ3r2X7eomMDH2wXRT674FKNZCaCeRN62hrirIhZv2~SZErz7VmFJAgqpiAHTBNCzWLxbVnBGcae6pCyDrcQnIDu2JD-OjSNfhMfxDTcB82qwYIr5XXVu7WElNPVu5JDLHO4jl8jYIIiEQ8NZQ__&Key-Pair-Id=APKAIE5G5CRDK6RD3PGA)
Top panel: age-reddening solutions for the pre-explosion and late-time observations of the probable compact cluster host of SN 2009kr, through comparison with starburst99 models and assuming RV = 3.1. The inner and outer contours contain 68 and 95 per cent of the total probability. The horizontal dashed line indicates the lifetime [3.2 × 107yr; for Padova isochrones (Girardi et al. 2000), used in the calculation of the starburst99 models] of a star with initial mass Minit = 8 M⊙ (i.e. the expected maximum lifetime for a star to end its life through a CCSN explosion). Bottom panel: selected example SED solutions to fits for the cluster SED, for age and reddening (according to the legend in top-right corner of the panel), drawn from the top panel.
The group of young, high reddening solutions are inconsistent with the low degree of reddening inferred towards SN 2009kr and, therefore by extension, to the presumed cluster host. The age of this objects is, therefore, constrained to be ≳7.8 Myr, corresponding to a progenitor with initial mass MZAMS ≲ 20–25 M⊙, while older solutions would suggest a lower mass (e.g. t = 16 Myr corresponds to MZAMS ≈ 12 M⊙). These solutions imply a cluster luminosity of ∼106.0L⊙ which, given the starburst99 models were computed with an initial cluster mass of 106 M⊙, imply a cluster with mass ∼6000 M⊙.
3.3 SN 2009md
SN 2009md was discovered by S. Nakano on 2009 Dec 4.8, in the galaxy NGC 3389 (Nakano, Yusa & Kadota 2009b), which was subsequently classified as a Type IIP SN by Sollerman et al. (2009) and Steele, Kandrashoff & Filippenko (2009b). Fraser et al. (2011) found SN 2009md to be consistent with a low-luminosity Type IIP SN and reported the identification of an RSG progenitor in pre-explosion WFPC2 images, concluding that the progenitor was a star with initial mass |$7^{+5}_{-1}\,\mathrm{M}_{{\odot }}$| exhibiting low reddening. Spiro et al. (2014) showed that SN 2009md had similar photometric and spectroscopic properties to another Type IIP SN, 2008bk. Here, we use the same distance (μ = 31.63 ± 0.55) and metallicity (solar metallicity) as was used by Fraser et al. (2011).
The pre-explosion and late-time observations of the position of SN 2009md are shown in Fig. 7. The position of SN 2009md was identified on the pre-explosion and late-time images with precision 0.026 and 0.022 arcsec, respectively, with reference to the post-explosion Gemini NIRI+ALTAIR observation presented by Fraser et al. (2011). In the pre-explosion images, we identify the same source as previously found by Fraser et al. at the transformed SN position. Using dolphot, we measured the brightness of this source to be mF606W = 26.76 ± 0.16 and mF814W = 24.92 ± 0.10 mag, on 2005 Mar 14, and mF606W = 26.73 ± 0.23 and mF814W = 24.87 ± 0.11 mag, on 2005 May 20. Our F606W photometry is ∼0.6 mag brighter than measured by Fraser et al. (2011) using hstphot (Dolphin 2000).
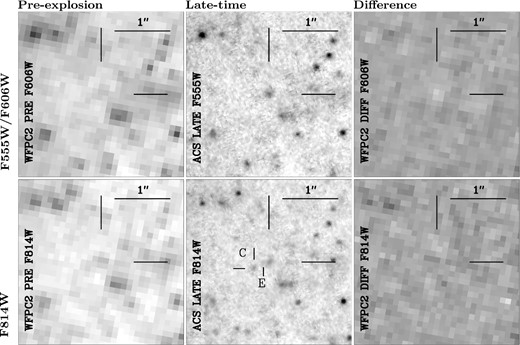
Pre-explosion WFPC2 F606W and F814W and late-time ACS F555W and F814W observations of the site of SN 2009md. The difference between the two epochs, for both filters, is shown in the right-hand panels of each row. Positive residuals in the difference image correspond to sources that are brighter in the late-time image. A nearby star, previously identified by Fraser et al. (2011), is labelled ‘C’
In the late-time ACS observations, a source is still recovered close to the transformed SN position, with a measured offset 0.94 pixel, corresponding to 0.023 arcsec (which is slightly larger than the uncertainty of the geometric transformation). We measured the brightness of the late-time source to be mF435W = 25.99 ± 0.10, mF555W = 25.32 ± 0.05 and mF814W = 24.99 ± 0.09 mag. The source is observed to be point like in the late-time images. The spatial extent of the late-time source, in all three images, was analysed using ishape (as was done for late-time source observed at the position of SN 2009kr, see above). The extended profiles, using a Moffat function with order 2.5, did not yield a significantly better solution over the delta function convolved with the PSF. We conclude that the late-time source is consistent with a point source.
In the late-time F814W image, a source (labelled E in Fig. 7, following the scheme of Fraser et al. 2011) located 0.11 arcsec east of the SN position is clearly resolved; this may have contributed to the pre-explosion F814W photometry, with mF814W = 26.38 ± 0.29 mag.
In the difference images between the pre-explosion and late-time images, the disparity between the pre-explosion F606W and late-time F555W photometry is readily apparent, with a bright positive residual recovered at the transformed SN position. We propose that this residual is from the still bright SN. This interpretation is somewhat complicated by an additional contribution from a colour term arising from the difference between the pre-explosion and late-time filters. We used synthetic photometry of ATLAS9 synthetic SEDs (Castelli & Kurucz 2004) covering the temperature range 3500 < T < 50 000 K (with gravities appropriate for supergiants at each temperature), to determine the relationship between F606W − F814W and F555W − F606W. Given the pre-explosion colour F606W − F814W = 1.86, we would expect a corresponding colour of 0.6 < F555W − F606W < 0.8. The disparity between the pre-explosion F606W and the late-time F555W photometry is not, therefore, merely a colour effect and represents significant, real excess flux in the late-time observation. Conversely, there is no clear residual in the F814W difference image, which reflects the approximately similar photometry derived directly and independently from the pre-explosion and late-time F814W images. A z-test with the pre-explosion and late-time F814W photometry fails to reject the null hypothesis that the two sets of measurements are the same (p = 0.39).
Given the time elapsed between the SN explosion and the acquisition of the late-time observations (2.94 yr), the likelihood of observing a progenitor and, subsequently at a randomly selected time, an SN (with the progenitor now missing) with the same brightness would be expected to be small. On the other hand, there is evidence from the late-time F555W photometry that there is still significant SN flux, such that it is difficult to rule out that the pre-explosion and late-time F814W brightnesses are just coincidence, rather than reflecting the continued presence of the object previously identified as the progenitor. Given the low reddening estimated towards SN 2009md (E(B − V) ∼ 0.1; Fraser et al. 2011), the late-time F555W brightness is unlikely to be due to a light echo, and may perhaps be due to ongoing interaction with a circumstellar medium.
We may consider three possible scenarios.
The pre-explosion source was a single star that exploded, and by chance the late-time F814W observation has measured the SN at epoch when it has the same brightness.
The pre-explosion source was a host cluster, that is recovered at late times in the F814W observation.
The pre-explosion source was unrelated to the SN and, by chance, was aligned with the position of the SN.
Under the assumption that the pre-explosion source was the progenitor, we considered the pre-explosion F606W and F814W photometry with respect to solar metallicity MARCS models (with log g = 0.0), with varying degrees of reddening, following a Galactic RV = 3.1 Cardelli, Clayton & Mathis (1989) reddening law. Previously Fraser et al. (2011) concluded that the total reddening towards SN 2009md was low and adopted |$E(B-V)=0.1^{+0.1}_{-0.05}$| (which was also used by Spiro et al.), which suggests that the pre-explosion colour is consistent with an RSG with T = 3550 ± 50 K and log (L/L⊙) = 4.55 ± 0.23. In comparison with STARS stellar evolution models (Eldridge & Tout 2004; Smartt et al. 2009), this suggests an initial mass for the progenitor in the range 8.5–13 M⊙.
If the pre-explosion source was a host cluster, that still persists after the SN explosion, the pre-explosion colours would correspond to clusters requiring significant reddening (E(B − V) ∼ 1.3–1.6 for clusters in the age range 11.7–100 Myr) arising from dust at significant distances from the SN; yielding cluster luminosities of ∼105.9 L⊙. As given above for the single star progenitor scenario, a single-mass star at the lowest initial limit to explode as a Type IIP SN (8 M⊙) would be expected to be a significant contributor to the cluster flux and, hence, would still be expected an appreciable effect on the colours of such a faint cluster before explosion and, through its absence, after explosion. Furthermore, there is no evidence for the late-time source being extended. At the distance of NGC 4603, the FWHM of the PSF in the late-time F555W image (≈3.6 pixel corresponds to 9 pc, suggesting a cluster would have to be significantly smaller than this scale (under good S/N conditions, ishape can probe physical scales down to ∼10 per cent of the FWHM; Larsen 1999). In this scenario, the region hosting SN 2009md would be required to contain extreme amounts of dust (for example, similar to the amount of dust required to redden the cluster M82-L; Lançon et al. 2008; Mattila et al. 2013). Given the reddening reported towards SN 2009md (Fraser et al. 2011), we find the scenario of the pre-explosion source being a host cluster to be, therefore, extremely unlikely.
An approximate measure of the probability of a chance alignment between the SN position and an unrelated star can be made by determining the number of sources in the field with brightness greater than or equal to the pre-explosion source. Given the number of such sources in the late-time F814W image, we crudely estimate the probability of a source with such a brightness occurring within 1 pixel of the SN position, by chance, to be p ∼ 0.001. This estimate does not take into account the local density of stars in the immediate vicinity of the SN position. We note, however, that inspection of the late-time F814W image (see Fig. 7) shows that the location of SN 2009md is not particularly crowded, and that the source at the SN position is sufficiently distant from any other objects with comparable brightness. We therefore discard chance alignment of an unrelated star with the SN position as a viable explanation for the pre-explosion and late-time HST observations of the position of SN 2009md.
Given the brightness at which SN 2009md was observed at late-times, these new HST observations cannot provide an additional window on the interpretation of the pre-explosion observations. The late-time observations, specifically the identical pre-explosion and late-time F814W, would suggest caution in assuming that the pre-explosion source was in fact the progenitor, since its disappearance after explosion cannot be confirmed. There are, however, significant difficulties with other possible scenarios that might be invoked to explain these observations.
4 DISCUSSION
As previously demonstrated by Maund & Smartt (2009), Gal-Yam & Leonard (2009), Maund et al. (2014a), Maund et al. (2014b) and Van Dyk et al. (2013), the confirmation of a progenitor's disappearance is crucial to establishing the authenticity of the original progenitor identification and the associated analysis. The analyses presented by Maund et al. (2014b,a) have shown how tailor made late-time observations, acquired under better conditions than the pre-explosion observations, can provide both enhanced astrometry and photometry of the progenitor than possible from the pre-explosion observations alone.
For each of the three SN considered here a major complication in the analysis of the late-time observations is the presence of significant flux remaining at the SN position. The cases of SN 2009kr and 2009md echo the findings of Maund et al. (2014a) in the case of SN 1999ev; for which a late-time F555W observation of the object at the SN location yielded the same brightness as measured from pre-explosion observations (acquired 15 years before).
In the cases presented here, despite the late-time brightness of each of the SNe, the late-time observations can clarify a number of key issues: (1) the precision of the progenitor identification through differential astrometry (e.g. 2008cn); and (2) the spatial extent of the object at the SN position (2009kr). These aspects can only be probed with deep late-time, high spatial resolution imaging. In the case of SN 2009md, the nature of the late-time source is ambiguous and casts doubt on whether the pre-explosion source was indeed the progenitor. From the late-time observations of SN 2009md, however, we have been able to establish that the late-time source is unlikely to incorporate a cluster (as the source appears point-like) that may have hosted the progenitor. We contrast the case of SN 2009md with that of SN 2009kr, where the spatial extent of the late-time source and the similarity of the pre-explosion and late-time brightnesses clearly indicates that the pre-explosion source identified for SN 2009kr was a cluster.
Clusters have been observed at the positions of SN in the cases of SN 2004dj (Maíz-Apellániz et al. 2004; Vinkó et al. 2009) and 2004am (Mattila et al. 2013), for which ages were derived under the assumption that the total light arose from a large coeval stellar population. Previously, possible cluster scenarios for SNe 2008cn and 2009kr were discussed by Elias-Rosa et al. (2009), Elias-Rosa et al. (2010) and Fraser et al. (2010), but were ultimately ruled out on luminosity criteria, specifically the requirement that MV < −8.6 (Bastian et al. 2005). It should be noted, however, that this limit as presented by Bastian et al. (2005) is only indicative of the brightness for which point-like sources are more likely to be compact clusters than individual, luminous stars. Elias-Rosa et al. (2010) also consider the pre-explosion source at the position of SN 2009kr to violate the Lowest Luminosity Limit (LLL) for a compact cluster, following Cerviño & Luridiana (2004). We note, however, that the LLL in itself does not preclude an object from being a cluster, if it is fainter than a given limit; rather it indicates where the SED of a cluster maybe significantly biased by individual stars.
In our analysis of the pre-explosion colours, we have assumed that the host cluster of SN 2009kr is best represented by a starburst99 model; however, such models assume a continuous sample of stars drawn from the initial mass function (IMF). As shown by Vinkó et al. (2009), stochastic sampling of the IMF, when the number of stars is not above the effective ‘infinite star limit’ (represented by such simple stellar population models), can cause the colours of a cluster to deviate from the predicted colours for a given age. For example, assuming a Salpeter (1955) IMF (α = 2.35), with stars drawn in from the mass range 0.1 ≤ M ≤ 25 M⊙, those stars expected to end their lives as CCSNe would only constitute 0.2 per cent of the stars in the cluster by number. For a cluster with small mass, such as the mass determined for the putative host cluster of SN 2009kr, the cluster luminosity may be dominated by a handful of bright stars, of which the progenitor would be expected to be one. The absence of the progenitor would, therefore, be expected to have a significant impact on the cluster's SED. Unfortunately, given the late-time brightness of SN 2009kr, these late-time observations are not able to probe the effect of sampling and the individual contribution from the progenitor.
The absolute brightness of the pre-explosion source for SN 2009kr corresponds to MV ∼ −8.8, which just satisfies the Bastian et al. (2005) criterion. The cluster hypothesis can be further evaluated using the spatial extent of the pre-explosion source; however, from the pre-explosion images alone, there is limited evidence due to the poor sampling of the PSF, in conjunction with the relatively large distances to these SNe. It is clear from our superior late-time observations, with improved sampling by a factor of 4 (along with increased depth), that the cluster origin hypothesis for these three SNe can be tested more robustly. Conversely, in the case of SN 2009md, there is no evidence that the pre-explosion source or the source recovered in late-time observations is extended.
Kuncarayakti et al. (2013) acquired integral field spectroscopy of the site of SN 2009kr and, in addition to deriving a metallicity appropriate for the region, determined an age of 3.26 Myr, which they applied to the progenitor. From our analysis of the pre-explosion and late-time observations of the site of SN 2009kr, we suggest an age appropriate for the cluster at the SN position to be >7.8 Myr. The target of the Kuncarayakti et al. spectroscopy was a large star cluster, offset from the SN position to north-east and is clearly visible on Fig. 5. As revealed in our high-resolution HST observations, this cluster is in fact a complex group of dense knots of clusters and stars. This might suggest that the age of Kuncarayakti et al. (2013) might be complicated by multiple stellar population in the large cluster of knots. While an association between the putative host cluster of the progenitor of SN 2009kr with this larger cluster is not clear, the host cluster could represent an earlier epoch of star formation in that location of NGC 1832.
SNe 2008cn and 2009kr played a pivotal role in establishing that, for some Type II SNe, YSGs were observed at the progenitors, such as for SNe 2004et, 2008ax and 2011dh; of six cases, however, only the progenitors of SNe 2008ax and 2011dh have been shown to be bona fide YSGs (and have also been shown to have subsequently disappeared). Crockett et al. (2011) showed, through using late-time observations, that the identification of a YSG as the progenitor of the Type IIP SN 2004et was erroneous, with multiple sources blended together in the pre-explosion observations. Although the available light curve for SN 2008cn is limited (Elias-Rosa et al. 2009), it is consistent with being a Type IIP SN. The reanalyses of the pre-explosion observations of SNe 2004et and 2008cn have shown that YSGs are not an additional channel for the production of Type IIP SN. Georgy (2012) invoked enhanced mass-loss from the progenitor to yield a YSG progenitor coupled with a subsequent H-poor SN (e.g. Type IIL/IIb SNe). While SN 2009kr showed a light-curve decline similar to Type IIL SNe, our late-time observations suggests that the yellow nature of the pre-explosion source is not likely to be correlated with the behaviour of the SN.
While the exact nature of the pre-explosion source at the position of SN 2009md cannot be confirmed with these late-time observations, the available data may suggest that this source was an individual RSG. The nature of this RSG and the resulting SN has implications for the understanding of how certain types of progenitors give rise to SNe with given properties. Spiro et al. (2014) demonstrated that SN 2009md is a similar low-luminosity SN to the Type IIP SN 2008bk; with both SNe having similar luminosities on the plateaus, similar plateau durations and similar characteristic velocities (Van Dyk et al. 2012; Spiro et al. 2014). Maund et al. (2014b) showed that, given eight detections and two upper limits covering optical and near-infrared wavelengths, the progenitor of SN 2008bk was constrained to be a highly reddened RSG, with log (L/L⊙) = 4.84, leading Maund et al. to conclude it had an initial mass ∼13 M⊙.
Assuming that the pre-explosion source is the progenitor of SN 2009md, we find that the radius (500 R⊙) is similar to that determined for SN 2008bk (470 R⊙); however, the temperature of the progenitor of SN 2009md would correspondingly be significantly cooler than measured for SN 2008bk (4330 K), leading to the 0.3-dex luminosity difference between the two progenitors. The temperature determined for the progenitor of SN 2008bk is consistent with the new RSG temperature scale defined by Davies et al. (2013), with respect to MARCS SEDs. We note, however, that the Davies et al. sample does not probe luminosity levels comparable to that determined for the progenitor of SN 2009md, such that the difference in temperatures may correspond to a real change in luminosity at constant radius over initial masses in the range 8–13 M⊙.
5 CONCLUSIONS
From our analysis of new late-time observations of the sites of three Type II SNe, we find that the previous conclusions concerning these progenitors based on pre-explosion observations alone are unreliable; however, in each of these three cases, the interpretation of the late-time observations themselves has been complicated by the presence of significant flux which we interpret as arising from the still fading SN. In none of the three cases have we observed the disappearance of the object previously identified as the progenitor. For these three SNe we find.
SN 2008cn.The progenitor candidate, previously identified in pre-explosion F555W and F814W images, is shown to be composed of two separate sources; of which, only the pre-explosion F814W source is coincident with the SN position. This is in agreement with one of the scenarios proposed by Elias-Rosa et al. (2009).
SN 2009kr. The pre-explosion source previously identified as the progenitor appears to be still present and, due to it appearing physically extended, we conclude the source is a compact cluster.
SN 2009md. The pre-explosion source appears to be still present in the late-time F814W image, with the same brightness as measured from pre-explosion observations.
We find that previous reports of YSGs as the progenitors of Type IIP SNe, which were based on limited, lower resolution pre-explosion images, were most likely mistaken. The only remaining cases of bona fide YSG progenitors are those associated with Type IIb SNe, and there are still questions about the nature of the progenitors of the ‘intermediate’ Type IIL SNe. Despite sharing similar properties with the other low-luminosity SN 2008bk, SN 2009md arose from a significantly fainter and cooler RSG.
The research of JRM is funded through a Royal Society University Research Fellowship. The research of MF was partly supported by the European Union FP7 programme through ERC grant number 320360.
Based on observations made with the NASA/ESA Hubble Space Telescope, which is operated by the Association of Universities for Research in Astronomy, Inc., under NASA contract NAS 5-26555. These observations are associated with program GO-12262.
Royal Society Research Fellow.