-
PDF
- Split View
-
Views
-
Cite
Cite
Céline Péroux, Nicolas Bouché, Varsha P. Kulkarni, Donald G. York, Giovanni Vladilo, A SINFONI integral field spectroscopy survey for galaxy counterparts to damped Lyman α systems – III. Three additional detections, Monthly Notices of the Royal Astronomical Society, Volume 419, Issue 4, February 2012, Pages 3060–3073, https://doi.org/10.1111/j.1365-2966.2011.19947.x
- Share Icon Share
ABSTRACT
We report three additional Spectrograph for Integral Field Observations in the Near Infrared (SINFONI) detections of Hα emission line from quasar absorbers, two of which are new identifications. These were targeted among a sample of systems with and metallicities measured from high-resolution spectroscopy. The detected galaxies are at impact parameters ranging from 6 to 12 kpc from the quasar's line of sight. We derive star formation rates (SFRs) of a few M⊙ yr−1 for the two absorbers at zabs∼ 1 and SFR = 17 M⊙ yr−1 for the damped Lyman α system (DLA) at zabs∼ 2. These three detections are found among a sample of 16 DLAs and sub-DLAs (five at zabs∼ 1 and seven at zabs∼ 2). For the remaining undetected galaxies, we derive flux limits corresponding to SFR < 0.1–11.0 M⊙ yr−1 depending on redshift of the absorber and depth of the data. When combined with previous results from our survey for galaxy counterparts to H i-selected absorbers, we find a higher probability of detecting systems with higher metallicity as traced by dust-free [Zn/H] metallicity. We also report a higher detection rate with SINFONI for host galaxies at zabs∼ 1 than for systems at zabs∼ 2. Using the [N ii]/Hα ratio, we can thus compare absorption and emission metallicities in the same high-redshift objects, more than doubling the number of systems for which such measures are possible.
1 INTRODUCTION
Our understanding of the processes taking place in galaxy formation has considerably advanced in the last years but important issues still need to be addressed. In order to obtain a complete picture, we need to study the details of processes though which galaxies convert their gas into stars. One way to tackle these phenomena is to relate the neutral hydrogen gas and the stars in individual galaxies.
The study of the galaxies probed by the absorption that their gas produces in the spectrum of a background quasar has been a prime tool to study the evolution of gas over cosmological time scales (Péroux et al. 2003a, 2005; Prochaska, Herbert-Fort & Wolfe 2005; Noterdaeme et al. 2009). In fact, the physical state and chemical abundances of the gas in the absorbing galaxies can be probed back to ∼10 billion years ago (e.g. Kulkarni et al. 2007, 2010). Nevertheless, studying the stellar content of all these systems turns out to be rather challenging: the galaxies that produce such absorption might be faint, thus requiring deep observations to detect their stellar/interstellar emission; in addition, they sometimes have small angular separations from the bright background quasars, which makes it difficult to disentangle the light of the galaxy from that of the quasar (Chen & Lanzetta 2003; Rao et al. 2003, 2011; Rosenberg & Schneider 2003).
We use the integral field unit (IFU) technique to detect redshifted Hα emission from the host of quasar absorbers. This approach has previously led to the detection of two-third of the galaxy hosts in a sample of 21 Mg ii-selected absorbers at zabs∼ 1 (Bouché et al. 2007). More recently, Bouché et al. (2011) have undertook a similar survey at z∼ 2 but obtain a lower detection rate (four out of 20 Mg ii-selected absorbers). Here, we expand our survey for quasar absorbers (Péroux et al. 2011a,b) and report additional detections at redshifts z∼ 1 and ∼ 2, two of which are new identifications. These three damped Lyman α systems (DLAs)/sub-DLAs have N(H i) and hence absorption metallicities known from high-resolution spectroscopy, and are detected among a sample of 16 quasar absorbers.
The present paper is structured as follows. A summary of the targets selection and observational details is provided in Section 2. In Section 3, we present the detected DLA/sub-DLAs galaxies. The results on physical properties, including detection rate, optimal target selection criteria, star formation estimates and metallicities, are presented in Section 4. Finally, Appendix A contains details of the systems which yield no detection. The dynamical properties (see e.g. Péroux et al. 2011b) of these detections will be published in future papers. Throughout this paper, we assume a cosmology with H0= 71 km s−1 Mpc−1, ΩM= 0.27 and ΩΛ= 0.73.
2 OBSERVATIONS
We have searched for the Hα emissions in 16 intervening quasars absorbers with H i column densities , corresponding to the definition of sub-DLAs (Péroux et al. 2003b) and
, corresponding to the definition of DLAs (Wolfe et al. 1995). All of the prime target absorbers have well constrained N(H i) based on space-based Hubble Space Telescope (HST) observations (Rao, Turnshek & Nestor 2006). Similarly, the objects have been selected so that the metallicity nearly free from dust depletion, as traced by zinc [Zn/H],1 has been studied with high-resolution spectroscopy. Because of the redshift of the absorbers is accurately known from fits of heavy elements, we have also selected absorbers for which the predicted Hα lines fall in regions free from OH sky lines contamination. From these, we selected a sample of 16 absorbers with absorption redshift 0.7 < zabs < 2.6 and appropriate right ascension (RA)/declination (Dec.) for the observing set-up, constituting the ‘main’ targets as listed in Table 1. Five of these targets are at zabs∼ 1 and 11 at zabs∼ 2, putting the Hα emission line to Spectrograph for Integral Field Observations in the Near Infrared (SINFONI)2J- or K-band filters, respectively.
Adopted short quasar namesa . | Discovery quasar names . | Vmag . | zem . | zabs . | ESO period . | Texp(s) ×Nexp . | Band . | AOb . | Seeing (arcsec) . |
---|---|---|---|---|---|---|---|---|---|
Q0001−0159 | UM 196 | 18.0 | 2.810 | 2.0950 | P85 | 900 × 4 + 600 × 4 | K | NGS | 0.6 |
Q0452−1640 | B0449−1645 | 18.0 | 2.679 | 1.0072 | P85 | 600 × 12 | J | No AO | 1.1 |
Q1211+0902 | SDSS J121134.95+090220.9 | 18.5 | 3.292 | 2.5841 | P85 | 600 × 8 | K | No AO | 0.9 |
Q1220−0040 | SDSS J122037.01−004032.4 | 18.6 | 1.411 | 0.9746 | P83 | 600 × 12 | J | No AO | 1.0 |
Q1225+0035 | SDSS J122556.61+003535.1 | 19.0 | 1.226 | 0.7731 | P83 | 600 × 4 | J | No AO | 1.0 |
Q1226+1736 | SDSS J122607.19+173649.8 | 18.1 | 2.925 | 2.5576 | P85 | 600 × 4 | K | NGS | 0.7 |
Q1234+0758 | PKS 1232+082 | 18.4 | 2.570 | 2.3376 | P83 | 600 × 4 | K | No AO | 0.9 |
Q1356−1101 | PKS 1354−107 | 19.2 | 3.006 | 2.5009 | P85 | 600 × 12 | K | NGS | 0.5 |
Q1454+1210 | SDSS J145418.58+121053.8 | 18.6 | 3.256 | 2.2550 | P85 | 900 × 4 + 600 × 8 | K | No AO | 0.7 |
Q1631+1156 | SDSS J163145.17+115603.3 | 18.5 | 1.792 | 0.9008 | P83 | 600 × 8 | J | No AO | 1.1 |
Q2059−0528 | SDSS J205922.42−052842.7 | 19.3 | 2.539 | 2.2100 | P85 | 900 × 8 + 600 × 4 | K | NGS | 0.9 |
Q2102−3553 | B2059−360 | 18.6 | 3.090 | 2.5070 | P85 | 600 × 8 | K | No AO | 1.1 |
Q2222−0946 | SDSS J222256.11−094636.2 | 18.3 | 2.927 | 2.3543 | P85 | 900 × 8 + 600 × 4 | K | NGS | 0.6 |
Q2313−3704 | PKS 2311−373 | 18.5 | 2.476 | 2.1821 | P85 | 600 × 11 | K | No AO | 1.0 |
Q2350−0052 | SDSS J235057.88−005209.8/UM 184 | 19.5 | 3.023 | 2.6147 | P83 | 600 × 8 + 300 × 4 | K | No AO | 0.8 |
Q2352−0028 | SDSS J235253.51−002850.4 | 18.6 | 1.624 | 1.0318 | P85 | 600 × 12 | J | NGS | 0.7 |
Adopted short quasar namesa . | Discovery quasar names . | Vmag . | zem . | zabs . | ESO period . | Texp(s) ×Nexp . | Band . | AOb . | Seeing (arcsec) . |
---|---|---|---|---|---|---|---|---|---|
Q0001−0159 | UM 196 | 18.0 | 2.810 | 2.0950 | P85 | 900 × 4 + 600 × 4 | K | NGS | 0.6 |
Q0452−1640 | B0449−1645 | 18.0 | 2.679 | 1.0072 | P85 | 600 × 12 | J | No AO | 1.1 |
Q1211+0902 | SDSS J121134.95+090220.9 | 18.5 | 3.292 | 2.5841 | P85 | 600 × 8 | K | No AO | 0.9 |
Q1220−0040 | SDSS J122037.01−004032.4 | 18.6 | 1.411 | 0.9746 | P83 | 600 × 12 | J | No AO | 1.0 |
Q1225+0035 | SDSS J122556.61+003535.1 | 19.0 | 1.226 | 0.7731 | P83 | 600 × 4 | J | No AO | 1.0 |
Q1226+1736 | SDSS J122607.19+173649.8 | 18.1 | 2.925 | 2.5576 | P85 | 600 × 4 | K | NGS | 0.7 |
Q1234+0758 | PKS 1232+082 | 18.4 | 2.570 | 2.3376 | P83 | 600 × 4 | K | No AO | 0.9 |
Q1356−1101 | PKS 1354−107 | 19.2 | 3.006 | 2.5009 | P85 | 600 × 12 | K | NGS | 0.5 |
Q1454+1210 | SDSS J145418.58+121053.8 | 18.6 | 3.256 | 2.2550 | P85 | 900 × 4 + 600 × 8 | K | No AO | 0.7 |
Q1631+1156 | SDSS J163145.17+115603.3 | 18.5 | 1.792 | 0.9008 | P83 | 600 × 8 | J | No AO | 1.1 |
Q2059−0528 | SDSS J205922.42−052842.7 | 19.3 | 2.539 | 2.2100 | P85 | 900 × 8 + 600 × 4 | K | NGS | 0.9 |
Q2102−3553 | B2059−360 | 18.6 | 3.090 | 2.5070 | P85 | 600 × 8 | K | No AO | 1.1 |
Q2222−0946 | SDSS J222256.11−094636.2 | 18.3 | 2.927 | 2.3543 | P85 | 900 × 8 + 600 × 4 | K | NGS | 0.6 |
Q2313−3704 | PKS 2311−373 | 18.5 | 2.476 | 2.1821 | P85 | 600 × 11 | K | No AO | 1.0 |
Q2350−0052 | SDSS J235057.88−005209.8/UM 184 | 19.5 | 3.023 | 2.6147 | P83 | 600 × 8 + 300 × 4 | K | No AO | 0.8 |
Q2352−0028 | SDSS J235253.51−002850.4 | 18.6 | 1.624 | 1.0318 | P85 | 600 × 12 | J | NGS | 0.7 |
Note:
The adopted short quasar names are truncated hhmm ± ddmm values for the 2000 coordinates.
No AO: no adaptive optics, natural seeing; NGS: adaptive optics with a natural guide star.
Adopted short quasar namesa . | Discovery quasar names . | Vmag . | zem . | zabs . | ESO period . | Texp(s) ×Nexp . | Band . | AOb . | Seeing (arcsec) . |
---|---|---|---|---|---|---|---|---|---|
Q0001−0159 | UM 196 | 18.0 | 2.810 | 2.0950 | P85 | 900 × 4 + 600 × 4 | K | NGS | 0.6 |
Q0452−1640 | B0449−1645 | 18.0 | 2.679 | 1.0072 | P85 | 600 × 12 | J | No AO | 1.1 |
Q1211+0902 | SDSS J121134.95+090220.9 | 18.5 | 3.292 | 2.5841 | P85 | 600 × 8 | K | No AO | 0.9 |
Q1220−0040 | SDSS J122037.01−004032.4 | 18.6 | 1.411 | 0.9746 | P83 | 600 × 12 | J | No AO | 1.0 |
Q1225+0035 | SDSS J122556.61+003535.1 | 19.0 | 1.226 | 0.7731 | P83 | 600 × 4 | J | No AO | 1.0 |
Q1226+1736 | SDSS J122607.19+173649.8 | 18.1 | 2.925 | 2.5576 | P85 | 600 × 4 | K | NGS | 0.7 |
Q1234+0758 | PKS 1232+082 | 18.4 | 2.570 | 2.3376 | P83 | 600 × 4 | K | No AO | 0.9 |
Q1356−1101 | PKS 1354−107 | 19.2 | 3.006 | 2.5009 | P85 | 600 × 12 | K | NGS | 0.5 |
Q1454+1210 | SDSS J145418.58+121053.8 | 18.6 | 3.256 | 2.2550 | P85 | 900 × 4 + 600 × 8 | K | No AO | 0.7 |
Q1631+1156 | SDSS J163145.17+115603.3 | 18.5 | 1.792 | 0.9008 | P83 | 600 × 8 | J | No AO | 1.1 |
Q2059−0528 | SDSS J205922.42−052842.7 | 19.3 | 2.539 | 2.2100 | P85 | 900 × 8 + 600 × 4 | K | NGS | 0.9 |
Q2102−3553 | B2059−360 | 18.6 | 3.090 | 2.5070 | P85 | 600 × 8 | K | No AO | 1.1 |
Q2222−0946 | SDSS J222256.11−094636.2 | 18.3 | 2.927 | 2.3543 | P85 | 900 × 8 + 600 × 4 | K | NGS | 0.6 |
Q2313−3704 | PKS 2311−373 | 18.5 | 2.476 | 2.1821 | P85 | 600 × 11 | K | No AO | 1.0 |
Q2350−0052 | SDSS J235057.88−005209.8/UM 184 | 19.5 | 3.023 | 2.6147 | P83 | 600 × 8 + 300 × 4 | K | No AO | 0.8 |
Q2352−0028 | SDSS J235253.51−002850.4 | 18.6 | 1.624 | 1.0318 | P85 | 600 × 12 | J | NGS | 0.7 |
Adopted short quasar namesa . | Discovery quasar names . | Vmag . | zem . | zabs . | ESO period . | Texp(s) ×Nexp . | Band . | AOb . | Seeing (arcsec) . |
---|---|---|---|---|---|---|---|---|---|
Q0001−0159 | UM 196 | 18.0 | 2.810 | 2.0950 | P85 | 900 × 4 + 600 × 4 | K | NGS | 0.6 |
Q0452−1640 | B0449−1645 | 18.0 | 2.679 | 1.0072 | P85 | 600 × 12 | J | No AO | 1.1 |
Q1211+0902 | SDSS J121134.95+090220.9 | 18.5 | 3.292 | 2.5841 | P85 | 600 × 8 | K | No AO | 0.9 |
Q1220−0040 | SDSS J122037.01−004032.4 | 18.6 | 1.411 | 0.9746 | P83 | 600 × 12 | J | No AO | 1.0 |
Q1225+0035 | SDSS J122556.61+003535.1 | 19.0 | 1.226 | 0.7731 | P83 | 600 × 4 | J | No AO | 1.0 |
Q1226+1736 | SDSS J122607.19+173649.8 | 18.1 | 2.925 | 2.5576 | P85 | 600 × 4 | K | NGS | 0.7 |
Q1234+0758 | PKS 1232+082 | 18.4 | 2.570 | 2.3376 | P83 | 600 × 4 | K | No AO | 0.9 |
Q1356−1101 | PKS 1354−107 | 19.2 | 3.006 | 2.5009 | P85 | 600 × 12 | K | NGS | 0.5 |
Q1454+1210 | SDSS J145418.58+121053.8 | 18.6 | 3.256 | 2.2550 | P85 | 900 × 4 + 600 × 8 | K | No AO | 0.7 |
Q1631+1156 | SDSS J163145.17+115603.3 | 18.5 | 1.792 | 0.9008 | P83 | 600 × 8 | J | No AO | 1.1 |
Q2059−0528 | SDSS J205922.42−052842.7 | 19.3 | 2.539 | 2.2100 | P85 | 900 × 8 + 600 × 4 | K | NGS | 0.9 |
Q2102−3553 | B2059−360 | 18.6 | 3.090 | 2.5070 | P85 | 600 × 8 | K | No AO | 1.1 |
Q2222−0946 | SDSS J222256.11−094636.2 | 18.3 | 2.927 | 2.3543 | P85 | 900 × 8 + 600 × 4 | K | NGS | 0.6 |
Q2313−3704 | PKS 2311−373 | 18.5 | 2.476 | 2.1821 | P85 | 600 × 11 | K | No AO | 1.0 |
Q2350−0052 | SDSS J235057.88−005209.8/UM 184 | 19.5 | 3.023 | 2.6147 | P83 | 600 × 8 + 300 × 4 | K | No AO | 0.8 |
Q2352−0028 | SDSS J235253.51−002850.4 | 18.6 | 1.624 | 1.0318 | P85 | 600 × 12 | J | NGS | 0.7 |
Note:
The adopted short quasar names are truncated hhmm ± ddmm values for the 2000 coordinates.
No AO: no adaptive optics, natural seeing; NGS: adaptive optics with a natural guide star.
A journal of observations summarizing the quasar properties and experimental set-up is also presented in Table 1. The table provides the observing log, the exposure times for each of the objects and the resulting seeing of the combined data. The observations were carried out in Service Mode during two separate observing runs (ESO 83.A-0699 and 85.A-0708) at the European Southern Observatory (ESO) with SINFONI on the 8.2-m YEPUN telescope. The ESO period P83 ran from 2009 April to 2009 September, while the ESO period P85 ran from 2010 April to 2010 September. For fields where the quasar itself is bright enough or in which a bright star is available nearby, we have used them as natural guide stars (NGSs) for adaptive optics (AO) in order to improve the spatial resolution. For the fields with no suitable tip-tilt stars, we have used natural seeing (no AO). The resulting seeing ranges from 0.5 to 1.1 arcsec. We note that most of the observations for the P83 run and some of the P85 run were incomplete and without AO. The J (K) grism provides a spectral resolution of around R∼ 2000 (4000). The resulting field of view (a mosaic of the 8 × 8 arcsec2 SINFONI field of view resulting in a 10 × 10 arcsec2 effective field of view) corresponds to a search radius of ∼41 kpc at z∼ 1 and ∼43 kpc at z∼ 2, thus probing rather large impact parameters between the galaxy host and quasar line of sight. The data reduction and flux calibration were performed using the same procedures as the ones described in Péroux et al. (2011a) with a more recent version of the ESO pipeline (version 1.9.8).
3 DETECTED GALAXY COUNTERPARTS TO QUASAR ABSORBERS
In this section, we report on the detection of three absorbers in our SINFONI survey. The remaining undetected systems observed during P83 and P85 are described in Appendix A. The redshift of the main absorbers targeted is indicated in the title of the subsection, while additional systems reported in the literature but not optimal for SINFONI Hα detections in terms of wavelength coverage and OH contamination are indicated in brackets. In some cases, [O iii] was covered and looked for since [O iii] can be much stronger than Hα in some situations. However, we do not detect any [O iii] emission lines at flux levels comparable to the one listed in Table 2 for Hα. All of our main targets (and some of the non-prime systems) have been observed in absorption at high resolution and we indicate abundance ratios, Mg ii equivalent widths, heavy element velocity widths and number of components whenever available. We also list any previous attempts to image some of these fields.
Summary of the detections and upper limits for the 16 main targets from this work. We also recall previous results from the first epoch of our survey (Péroux et al. 2011a).
Quasar . | zabs . | ![]() | [Zn/H]a . | [Fe/H]a . | [Si/H]a . | F(Hα)b (erg s−1 cm−2) . | Lum(Hα) (erg s−1) . | SFR (M⊙ yr−1) . | Ref . |
---|---|---|---|---|---|---|---|---|---|
Q0001−0159 | 2.0950 | 20.70 ± 0.10 | −0.75 | −1.70 | −0.84 | <4.9 × 10−17 | <16.1 × 1041 | <7.1 | This work |
Q0134+0051 | 0.8420 | 19.93![]() | < −0.36 | −0.91 | … | <1.0 × 10−17 | <0.3 × 1041 | <0.2 | Péroux et al. (2011a) |
Q0302−223 | 1.0094 | 20.36![]() | −0.51 | −1.20 | −0.73 | 7.7 ± 2.7 × 10−17 | 4.1 ± 1.4 × 1041 | 1.8 ± 0.6 | Péroux et al. (2011a) |
Q0452−1640 | 1.0072 | 20.98![]() | −0.96 | −1.34 | … | 14.5 ± 4.3 × 10−17 | 8.0 ± 2.4 × 1041 | 3.5 ± 1.0 | This work |
Q1009−0026 | 0.8426 | 20.20 ![]() | < −0.98 | −1.28 | … | <1.0 × 10−17 | <0.3 × 1041 | <0.2 | Péroux et al. (2011a) |
– | 0.8866 | 19.48 ![]() | +0.25 | −0.37 | < −0.02 | 17.1 ± 6.0 × 10−17 | 6.6 ± 2.3 × 1041 | 2.9 ± 1.0 | Péroux et al. (2011a) |
Q1211+0902 | 2.5841 | 21.40 ± 0.10 | −1.09 | −1.65 | −1.05 | <2.3 × 10−17 | <12.6 × 1041 | <5.6 | This work |
Q1220−0040 | 0.9746 | 20.20![]() | < −1.14 | −1.33 | … | <0.7 × 10−17 | <0.3 × 1041 | <0.1 | This work |
Q1225+0035 | 0.7731 | 21.38![]() | −0.78 | −1.19 | … | <5.3 × 10−17 | <1.5 × 1041 | <0.7 | This work |
Q1226+1736 | 2.5576 | 19.32 ± 0.15 | < −0.36 | −1.84 | −1.59 | <2.9 × 10−17 | <15.7 × 1041 | <7.0 | This work |
Q1228−113 | 2.1929 | 20.60![]() | < −0.22 | … | … | <0.5 × 10−17 | <2.0 × 1041 | <0.9 | Péroux et al. (2011a) |
Q1234+0758 | 2.3376 | 20.80 ± 0.10 | −0.86 | −1.59 | −1.18 | <1.2 × 10−17 | <5.9 × 1041 | <2.4 | This work |
Q1323−0021 | 0.7160 | 20.21![]() | +0.61 | −0.51 | … | <1.0 × 10−17 | <0.2 × 1041 | <0.1 | Péroux et al. (2011a) |
Q1356−1101 | 2.5009 | 20.40 ± 0.49 | < −1.32 | −1.25 | … | <2.3 × 10−17 | <12.1 × 1041 | <5.4 | This work |
Q1454+1210 | 2.2550 | 20.30 ± 0.15 | −1.12 | −1.47 | > −0.40 | <1.2 × 10−17 | <4.8 × 1041 | <2.1 | This work |
Q1631+1156 | 0.9008 | 19.70![]() | −0.18 | −1.06 | … | <1.5 × 10−17 | <0.6 × 1041 | <0.3 | This work |
Q2059−0528 | 2.2100 | 20.80 ± 0.20 | −0.53 | −1.30 | −1.00 | <0.8 × 10−17 | <3.2 × 1041 | <1.4 | This work |
Q2102−3553 | 2.5070 | 20.21 ± 0.10 | < −0.44 | −2.24 | −1.04 | <1.2 × 10−17 | <6.3 × 1041 | <2.8 | This work |
Q2222−0946 | 2.3543 | 20.50 ± 0.15 | −0.46 | −0.99 | −0.51 | 8.7 ± 2.6 × 10−17 | 38.5 ± 1.1 × 1041 | 17.1 ± 5.1 | This work |
Q2313−3704 | 2.1821 | 20.48 ± 0.10 | < −1.29 | −1.70 | −1.52 | <1.1 × 10−17 | <4.1 × 1041 | <1.8 | This work |
Q2350−0052 | 2.6147 | 21.30 ± 0.10 | < −2.10 | −2.23 | −1.97 | <1.5 × 10−17 | <8.6 × 1041 | <3.8 | This work |
Q2352−0028 | 1.0318 | 19.81![]() | < −0.51 | −0.37 | +0.14 | 4.9 ± 2.4 × 10−17 | 2.8 ± 1.4 × 1041 | 1.3 ± 0.6 | This work |
Quasar . | zabs . | ![]() | [Zn/H]a . | [Fe/H]a . | [Si/H]a . | F(Hα)b (erg s−1 cm−2) . | Lum(Hα) (erg s−1) . | SFR (M⊙ yr−1) . | Ref . |
---|---|---|---|---|---|---|---|---|---|
Q0001−0159 | 2.0950 | 20.70 ± 0.10 | −0.75 | −1.70 | −0.84 | <4.9 × 10−17 | <16.1 × 1041 | <7.1 | This work |
Q0134+0051 | 0.8420 | 19.93![]() | < −0.36 | −0.91 | … | <1.0 × 10−17 | <0.3 × 1041 | <0.2 | Péroux et al. (2011a) |
Q0302−223 | 1.0094 | 20.36![]() | −0.51 | −1.20 | −0.73 | 7.7 ± 2.7 × 10−17 | 4.1 ± 1.4 × 1041 | 1.8 ± 0.6 | Péroux et al. (2011a) |
Q0452−1640 | 1.0072 | 20.98![]() | −0.96 | −1.34 | … | 14.5 ± 4.3 × 10−17 | 8.0 ± 2.4 × 1041 | 3.5 ± 1.0 | This work |
Q1009−0026 | 0.8426 | 20.20 ![]() | < −0.98 | −1.28 | … | <1.0 × 10−17 | <0.3 × 1041 | <0.2 | Péroux et al. (2011a) |
– | 0.8866 | 19.48 ![]() | +0.25 | −0.37 | < −0.02 | 17.1 ± 6.0 × 10−17 | 6.6 ± 2.3 × 1041 | 2.9 ± 1.0 | Péroux et al. (2011a) |
Q1211+0902 | 2.5841 | 21.40 ± 0.10 | −1.09 | −1.65 | −1.05 | <2.3 × 10−17 | <12.6 × 1041 | <5.6 | This work |
Q1220−0040 | 0.9746 | 20.20![]() | < −1.14 | −1.33 | … | <0.7 × 10−17 | <0.3 × 1041 | <0.1 | This work |
Q1225+0035 | 0.7731 | 21.38![]() | −0.78 | −1.19 | … | <5.3 × 10−17 | <1.5 × 1041 | <0.7 | This work |
Q1226+1736 | 2.5576 | 19.32 ± 0.15 | < −0.36 | −1.84 | −1.59 | <2.9 × 10−17 | <15.7 × 1041 | <7.0 | This work |
Q1228−113 | 2.1929 | 20.60![]() | < −0.22 | … | … | <0.5 × 10−17 | <2.0 × 1041 | <0.9 | Péroux et al. (2011a) |
Q1234+0758 | 2.3376 | 20.80 ± 0.10 | −0.86 | −1.59 | −1.18 | <1.2 × 10−17 | <5.9 × 1041 | <2.4 | This work |
Q1323−0021 | 0.7160 | 20.21![]() | +0.61 | −0.51 | … | <1.0 × 10−17 | <0.2 × 1041 | <0.1 | Péroux et al. (2011a) |
Q1356−1101 | 2.5009 | 20.40 ± 0.49 | < −1.32 | −1.25 | … | <2.3 × 10−17 | <12.1 × 1041 | <5.4 | This work |
Q1454+1210 | 2.2550 | 20.30 ± 0.15 | −1.12 | −1.47 | > −0.40 | <1.2 × 10−17 | <4.8 × 1041 | <2.1 | This work |
Q1631+1156 | 0.9008 | 19.70![]() | −0.18 | −1.06 | … | <1.5 × 10−17 | <0.6 × 1041 | <0.3 | This work |
Q2059−0528 | 2.2100 | 20.80 ± 0.20 | −0.53 | −1.30 | −1.00 | <0.8 × 10−17 | <3.2 × 1041 | <1.4 | This work |
Q2102−3553 | 2.5070 | 20.21 ± 0.10 | < −0.44 | −2.24 | −1.04 | <1.2 × 10−17 | <6.3 × 1041 | <2.8 | This work |
Q2222−0946 | 2.3543 | 20.50 ± 0.15 | −0.46 | −0.99 | −0.51 | 8.7 ± 2.6 × 10−17 | 38.5 ± 1.1 × 1041 | 17.1 ± 5.1 | This work |
Q2313−3704 | 2.1821 | 20.48 ± 0.10 | < −1.29 | −1.70 | −1.52 | <1.1 × 10−17 | <4.1 × 1041 | <1.8 | This work |
Q2350−0052 | 2.6147 | 21.30 ± 0.10 | < −2.10 | −2.23 | −1.97 | <1.5 × 10−17 | <8.6 × 1041 | <3.8 | This work |
Q2352−0028 | 1.0318 | 19.81![]() | < −0.51 | −0.37 | +0.14 | 4.9 ± 2.4 × 10−17 | 2.8 ± 1.4 × 1041 | 1.3 ± 0.6 | This work |
References for and abundance ratios are provided in Section 3 for the detected systems and in Appendix A for the undetected ones.
The 2.5σ upper limits for non-detections are computed for an unresolved source spread over 32 spatial pixels and spectral FWHM = 6 pixels = 9 Å.
Summary of the detections and upper limits for the 16 main targets from this work. We also recall previous results from the first epoch of our survey (Péroux et al. 2011a).
Quasar . | zabs . | ![]() | [Zn/H]a . | [Fe/H]a . | [Si/H]a . | F(Hα)b (erg s−1 cm−2) . | Lum(Hα) (erg s−1) . | SFR (M⊙ yr−1) . | Ref . |
---|---|---|---|---|---|---|---|---|---|
Q0001−0159 | 2.0950 | 20.70 ± 0.10 | −0.75 | −1.70 | −0.84 | <4.9 × 10−17 | <16.1 × 1041 | <7.1 | This work |
Q0134+0051 | 0.8420 | 19.93![]() | < −0.36 | −0.91 | … | <1.0 × 10−17 | <0.3 × 1041 | <0.2 | Péroux et al. (2011a) |
Q0302−223 | 1.0094 | 20.36![]() | −0.51 | −1.20 | −0.73 | 7.7 ± 2.7 × 10−17 | 4.1 ± 1.4 × 1041 | 1.8 ± 0.6 | Péroux et al. (2011a) |
Q0452−1640 | 1.0072 | 20.98![]() | −0.96 | −1.34 | … | 14.5 ± 4.3 × 10−17 | 8.0 ± 2.4 × 1041 | 3.5 ± 1.0 | This work |
Q1009−0026 | 0.8426 | 20.20 ![]() | < −0.98 | −1.28 | … | <1.0 × 10−17 | <0.3 × 1041 | <0.2 | Péroux et al. (2011a) |
– | 0.8866 | 19.48 ![]() | +0.25 | −0.37 | < −0.02 | 17.1 ± 6.0 × 10−17 | 6.6 ± 2.3 × 1041 | 2.9 ± 1.0 | Péroux et al. (2011a) |
Q1211+0902 | 2.5841 | 21.40 ± 0.10 | −1.09 | −1.65 | −1.05 | <2.3 × 10−17 | <12.6 × 1041 | <5.6 | This work |
Q1220−0040 | 0.9746 | 20.20![]() | < −1.14 | −1.33 | … | <0.7 × 10−17 | <0.3 × 1041 | <0.1 | This work |
Q1225+0035 | 0.7731 | 21.38![]() | −0.78 | −1.19 | … | <5.3 × 10−17 | <1.5 × 1041 | <0.7 | This work |
Q1226+1736 | 2.5576 | 19.32 ± 0.15 | < −0.36 | −1.84 | −1.59 | <2.9 × 10−17 | <15.7 × 1041 | <7.0 | This work |
Q1228−113 | 2.1929 | 20.60![]() | < −0.22 | … | … | <0.5 × 10−17 | <2.0 × 1041 | <0.9 | Péroux et al. (2011a) |
Q1234+0758 | 2.3376 | 20.80 ± 0.10 | −0.86 | −1.59 | −1.18 | <1.2 × 10−17 | <5.9 × 1041 | <2.4 | This work |
Q1323−0021 | 0.7160 | 20.21![]() | +0.61 | −0.51 | … | <1.0 × 10−17 | <0.2 × 1041 | <0.1 | Péroux et al. (2011a) |
Q1356−1101 | 2.5009 | 20.40 ± 0.49 | < −1.32 | −1.25 | … | <2.3 × 10−17 | <12.1 × 1041 | <5.4 | This work |
Q1454+1210 | 2.2550 | 20.30 ± 0.15 | −1.12 | −1.47 | > −0.40 | <1.2 × 10−17 | <4.8 × 1041 | <2.1 | This work |
Q1631+1156 | 0.9008 | 19.70![]() | −0.18 | −1.06 | … | <1.5 × 10−17 | <0.6 × 1041 | <0.3 | This work |
Q2059−0528 | 2.2100 | 20.80 ± 0.20 | −0.53 | −1.30 | −1.00 | <0.8 × 10−17 | <3.2 × 1041 | <1.4 | This work |
Q2102−3553 | 2.5070 | 20.21 ± 0.10 | < −0.44 | −2.24 | −1.04 | <1.2 × 10−17 | <6.3 × 1041 | <2.8 | This work |
Q2222−0946 | 2.3543 | 20.50 ± 0.15 | −0.46 | −0.99 | −0.51 | 8.7 ± 2.6 × 10−17 | 38.5 ± 1.1 × 1041 | 17.1 ± 5.1 | This work |
Q2313−3704 | 2.1821 | 20.48 ± 0.10 | < −1.29 | −1.70 | −1.52 | <1.1 × 10−17 | <4.1 × 1041 | <1.8 | This work |
Q2350−0052 | 2.6147 | 21.30 ± 0.10 | < −2.10 | −2.23 | −1.97 | <1.5 × 10−17 | <8.6 × 1041 | <3.8 | This work |
Q2352−0028 | 1.0318 | 19.81![]() | < −0.51 | −0.37 | +0.14 | 4.9 ± 2.4 × 10−17 | 2.8 ± 1.4 × 1041 | 1.3 ± 0.6 | This work |
Quasar . | zabs . | ![]() | [Zn/H]a . | [Fe/H]a . | [Si/H]a . | F(Hα)b (erg s−1 cm−2) . | Lum(Hα) (erg s−1) . | SFR (M⊙ yr−1) . | Ref . |
---|---|---|---|---|---|---|---|---|---|
Q0001−0159 | 2.0950 | 20.70 ± 0.10 | −0.75 | −1.70 | −0.84 | <4.9 × 10−17 | <16.1 × 1041 | <7.1 | This work |
Q0134+0051 | 0.8420 | 19.93![]() | < −0.36 | −0.91 | … | <1.0 × 10−17 | <0.3 × 1041 | <0.2 | Péroux et al. (2011a) |
Q0302−223 | 1.0094 | 20.36![]() | −0.51 | −1.20 | −0.73 | 7.7 ± 2.7 × 10−17 | 4.1 ± 1.4 × 1041 | 1.8 ± 0.6 | Péroux et al. (2011a) |
Q0452−1640 | 1.0072 | 20.98![]() | −0.96 | −1.34 | … | 14.5 ± 4.3 × 10−17 | 8.0 ± 2.4 × 1041 | 3.5 ± 1.0 | This work |
Q1009−0026 | 0.8426 | 20.20 ![]() | < −0.98 | −1.28 | … | <1.0 × 10−17 | <0.3 × 1041 | <0.2 | Péroux et al. (2011a) |
– | 0.8866 | 19.48 ![]() | +0.25 | −0.37 | < −0.02 | 17.1 ± 6.0 × 10−17 | 6.6 ± 2.3 × 1041 | 2.9 ± 1.0 | Péroux et al. (2011a) |
Q1211+0902 | 2.5841 | 21.40 ± 0.10 | −1.09 | −1.65 | −1.05 | <2.3 × 10−17 | <12.6 × 1041 | <5.6 | This work |
Q1220−0040 | 0.9746 | 20.20![]() | < −1.14 | −1.33 | … | <0.7 × 10−17 | <0.3 × 1041 | <0.1 | This work |
Q1225+0035 | 0.7731 | 21.38![]() | −0.78 | −1.19 | … | <5.3 × 10−17 | <1.5 × 1041 | <0.7 | This work |
Q1226+1736 | 2.5576 | 19.32 ± 0.15 | < −0.36 | −1.84 | −1.59 | <2.9 × 10−17 | <15.7 × 1041 | <7.0 | This work |
Q1228−113 | 2.1929 | 20.60![]() | < −0.22 | … | … | <0.5 × 10−17 | <2.0 × 1041 | <0.9 | Péroux et al. (2011a) |
Q1234+0758 | 2.3376 | 20.80 ± 0.10 | −0.86 | −1.59 | −1.18 | <1.2 × 10−17 | <5.9 × 1041 | <2.4 | This work |
Q1323−0021 | 0.7160 | 20.21![]() | +0.61 | −0.51 | … | <1.0 × 10−17 | <0.2 × 1041 | <0.1 | Péroux et al. (2011a) |
Q1356−1101 | 2.5009 | 20.40 ± 0.49 | < −1.32 | −1.25 | … | <2.3 × 10−17 | <12.1 × 1041 | <5.4 | This work |
Q1454+1210 | 2.2550 | 20.30 ± 0.15 | −1.12 | −1.47 | > −0.40 | <1.2 × 10−17 | <4.8 × 1041 | <2.1 | This work |
Q1631+1156 | 0.9008 | 19.70![]() | −0.18 | −1.06 | … | <1.5 × 10−17 | <0.6 × 1041 | <0.3 | This work |
Q2059−0528 | 2.2100 | 20.80 ± 0.20 | −0.53 | −1.30 | −1.00 | <0.8 × 10−17 | <3.2 × 1041 | <1.4 | This work |
Q2102−3553 | 2.5070 | 20.21 ± 0.10 | < −0.44 | −2.24 | −1.04 | <1.2 × 10−17 | <6.3 × 1041 | <2.8 | This work |
Q2222−0946 | 2.3543 | 20.50 ± 0.15 | −0.46 | −0.99 | −0.51 | 8.7 ± 2.6 × 10−17 | 38.5 ± 1.1 × 1041 | 17.1 ± 5.1 | This work |
Q2313−3704 | 2.1821 | 20.48 ± 0.10 | < −1.29 | −1.70 | −1.52 | <1.1 × 10−17 | <4.1 × 1041 | <1.8 | This work |
Q2350−0052 | 2.6147 | 21.30 ± 0.10 | < −2.10 | −2.23 | −1.97 | <1.5 × 10−17 | <8.6 × 1041 | <3.8 | This work |
Q2352−0028 | 1.0318 | 19.81![]() | < −0.51 | −0.37 | +0.14 | 4.9 ± 2.4 × 10−17 | 2.8 ± 1.4 × 1041 | 1.3 ± 0.6 | This work |
References for and abundance ratios are provided in Section 3 for the detected systems and in Appendix A for the undetected ones.
The 2.5σ upper limits for non-detections are computed for an unresolved source spread over 32 spatial pixels and spectral FWHM = 6 pixels = 9 Å.
3.1 Q0452−1640, zabs= 1.0072
This absorber at zabs= 1.0072 had its N(H i) column density measured by Rao et al. (2006) to be . Nestor et al. (2008) reported Zn ii and Cr ii detections in this Ca ii absorber using Intermediate dispersion Spectrograph and Imaging System (ISIS) on William Herschel Telescope (WHT). The quasar was first observed at high resolution by Péroux et al. (2008) who then derived the metallicity of the absorber by fitting 13 components over ∼230 km s−1 to the absorption profile. These authors derive [Mg/H] =−0.66 ± 0.11 from Mg iλ2852, [Cr/H] =−1.14 ± 0.09, [Mn/H] =−1.54 ± 0.11, [Fe/H] =−1.34 ± 0.08, [Zn/H] =−0.96 ± 0.08 and
. The Mg ii doublet for that system falls in an UV–Visual Echelle Spectrograph (UVES) spectral coverage gap. They also report possible detection of Co ii in the same system, [Co/H] =−0.45.
This absorber is the primary target of our Hα search and is clearly detected in our SINFONI data. Fig. 1 shows the Hα emission map and the spectrum of this galaxy. Table 2 lists the resulting Hα flux, luminosity and star formation rate (SFR) estimate. We report F([N ii] λ6585) =1.8 ± 0.5 × 10−17 erg s−1 cm−2, but S ii is not detected.
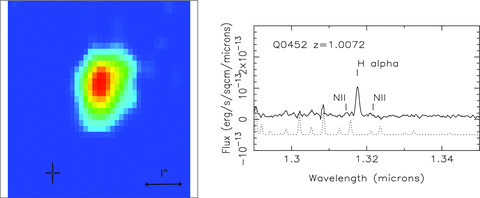
Left-hand panel: the Hα emission map of the targeted absorber in the field of Q0452−1640 at zabs= 1.0072. At this redshift, 1 arcsec = 8.0 kpc. The seeing of the stacked image is 1.1 arcsec. In this, and the following maps, the cross indicates the position of the quasar, north is up and east is to the left. Right-hand panel: the integrated spectrum over 13 pixels of the corresponding absorber galaxy with the redshifted Hα emission line. In this, and the following figures, the units are in erg s−1 cm−2 μm−1. The spectrum is smoothed (5-pixel boxcar). The dotted spectrum at the bottom of the panel is the sky spectrum with arbitrary flux units, scaled for clarity, and indicating the position of the OH sky lines.
3.2 Q2222−0946, zabs= 2.3541 (2.8647)
This is a quasar discovered as part of the Sloan Digital Sky Survey (SDSS) Data Release 3 (Schneider et al. 2005). Herbert-Fort et al. (2006) have studied the targeted absorber in detail. They report [Si/H] =−0.61 ± 0.20. Prochaska et al. (2007) list = 20.50 ± 0.15,
and [Fe/H] =−1.05 ± 0.02. The Mg ii doublet is not covered by these data. More recently, Fynbo et al. (2010) have used a triangulation of X-shooter spectra of that object to both accurately measure the metallicity of the absorber at zabs= 2.3541 fitted by three components over ∼100 km s−1: [Si/H] =−0.51 ± 0.06, [Zn/H] =−0.46 ± 0.07, [Fe/H] =−0.99 ± 0.06, [Ni/H] =−0.85 ± 0.06 and [Mn/H] =−1.23 ± 0.06 and detect Lyα emission from the galaxy host. They also report EW(Mg ii 2796) = 2.7 Å.
They also detect Hα and derive F(Hα) > 2.5 × 10−17 erg s−1 cm−2 corresponding to L(Hα) > 1.1 × 1042 erg s−1 and SFR > 10 M⊙ yr−1. They obtain Lyα/Hα > 3.6 and [O iii]/Hα= 1.6, such ratios are often considered the result of low-abundance gas which is hot (∼15 K instead of 8 K for normal abundances). The [O ii] and Hβ are located on top of bright sky emission lines leading to an upper limit F([O ii]) < 8 × 10−17 erg s−1 cm−2, i.e. SFR < 40 M⊙ yr−1. The authors note however that these fluxes are uncertain because the quasar flux in the X-shooter spectrum seems to be 30 per cent larger compared to the Sloan calibration and thus they artificially decreased the flux level. In addition, their slit does not actually cover the galaxy itself and the flux calibration is therefore subject to important slit loss.
We also detect the Hα emission in our SINFONI data cube and are able to locate the galaxy on the sky (see Table 3 for sky position). Fig. 2 shows the Hα emission map and the spectrum of this galaxy. Table 2 lists the resulting Hα flux, luminosity and SFR estimate. We report F([N ii]) < 0.4 × 10−17 erg s−1 cm−2 from a non-detection. From Hα, we derive a SFR estimate of 17.1 ± 6.0 M⊙ yr−1 consistent with findings from Fynbo et al. (2010) who estimated SFR > 10 M⊙ yr−1.
Properties of the two previously detected and three newly detected DLAs and sub-DLAs in the SINFONI cubes.
Quasar . | Δva (km s−1) . | ΔRA (arcsec) . | ΔDec. (arcsec) . | Projected dist (arcsec) . | Phys dist (kpc) . | ΣSFR (M⊙ yr−1 kpc−2) . | [N ii]/Hα . | 12+log(O/H) . | Reference . |
---|---|---|---|---|---|---|---|---|---|
Q0302−223 | 1 | +0.50 | +3.12 | 3.16 | 25 | 0.13 | <0.34 | <8.6 | Péroux et al. (2011a) |
Q0452−1640 | 10 | −0.125 | +2.00 | 2.00 | 16 | 0.49 | 0.12![]() | 8.4 ± 0.1 | This work |
Q1009−0026 | 125 | −5.01 | +0.37 | 5.01 | 39 | 0.31 | 0.48![]() | 8.7 ± 0.2 | Péroux et al. (2011a) |
Q2222−0946 | 30 | +0.50 | +0.50 | 0.70 | 6 | 1.97 | <0.05 | <8.2 | This work |
Q2352−0028 | 10 | +1.50 | +0.00 | 1.50 | 12 | 0.24 | 0.12![]() | 8.4 ± 0.3 | This work |
Quasar . | Δva (km s−1) . | ΔRA (arcsec) . | ΔDec. (arcsec) . | Projected dist (arcsec) . | Phys dist (kpc) . | ΣSFR (M⊙ yr−1 kpc−2) . | [N ii]/Hα . | 12+log(O/H) . | Reference . |
---|---|---|---|---|---|---|---|---|---|
Q0302−223 | 1 | +0.50 | +3.12 | 3.16 | 25 | 0.13 | <0.34 | <8.6 | Péroux et al. (2011a) |
Q0452−1640 | 10 | −0.125 | +2.00 | 2.00 | 16 | 0.49 | 0.12![]() | 8.4 ± 0.1 | This work |
Q1009−0026 | 125 | −5.01 | +0.37 | 5.01 | 39 | 0.31 | 0.48![]() | 8.7 ± 0.2 | Péroux et al. (2011a) |
Q2222−0946 | 30 | +0.50 | +0.50 | 0.70 | 6 | 1.97 | <0.05 | <8.2 | This work |
Q2352−0028 | 10 | +1.50 | +0.00 | 1.50 | 12 | 0.24 | 0.12![]() | 8.4 ± 0.3 | This work |
Observed velocity shift corresponding to Δz=zabs−zem of the detected galaxies.
Properties of the two previously detected and three newly detected DLAs and sub-DLAs in the SINFONI cubes.
Quasar . | Δva (km s−1) . | ΔRA (arcsec) . | ΔDec. (arcsec) . | Projected dist (arcsec) . | Phys dist (kpc) . | ΣSFR (M⊙ yr−1 kpc−2) . | [N ii]/Hα . | 12+log(O/H) . | Reference . |
---|---|---|---|---|---|---|---|---|---|
Q0302−223 | 1 | +0.50 | +3.12 | 3.16 | 25 | 0.13 | <0.34 | <8.6 | Péroux et al. (2011a) |
Q0452−1640 | 10 | −0.125 | +2.00 | 2.00 | 16 | 0.49 | 0.12![]() | 8.4 ± 0.1 | This work |
Q1009−0026 | 125 | −5.01 | +0.37 | 5.01 | 39 | 0.31 | 0.48![]() | 8.7 ± 0.2 | Péroux et al. (2011a) |
Q2222−0946 | 30 | +0.50 | +0.50 | 0.70 | 6 | 1.97 | <0.05 | <8.2 | This work |
Q2352−0028 | 10 | +1.50 | +0.00 | 1.50 | 12 | 0.24 | 0.12![]() | 8.4 ± 0.3 | This work |
Quasar . | Δva (km s−1) . | ΔRA (arcsec) . | ΔDec. (arcsec) . | Projected dist (arcsec) . | Phys dist (kpc) . | ΣSFR (M⊙ yr−1 kpc−2) . | [N ii]/Hα . | 12+log(O/H) . | Reference . |
---|---|---|---|---|---|---|---|---|---|
Q0302−223 | 1 | +0.50 | +3.12 | 3.16 | 25 | 0.13 | <0.34 | <8.6 | Péroux et al. (2011a) |
Q0452−1640 | 10 | −0.125 | +2.00 | 2.00 | 16 | 0.49 | 0.12![]() | 8.4 ± 0.1 | This work |
Q1009−0026 | 125 | −5.01 | +0.37 | 5.01 | 39 | 0.31 | 0.48![]() | 8.7 ± 0.2 | Péroux et al. (2011a) |
Q2222−0946 | 30 | +0.50 | +0.50 | 0.70 | 6 | 1.97 | <0.05 | <8.2 | This work |
Q2352−0028 | 10 | +1.50 | +0.00 | 1.50 | 12 | 0.24 | 0.12![]() | 8.4 ± 0.3 | This work |
Observed velocity shift corresponding to Δz=zabs−zem of the detected galaxies.
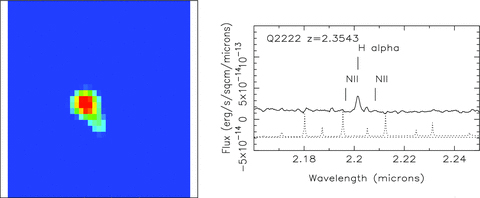
Left-hand panel: the Hα emission map of the targeted absorber in the field of Q2222−0946 at zabs= 2.3541. At this redshift, 1 arcsec = 8.3 kpc. The seeing of the stacked image is 0.6 arcsec. Right-hand panel: the integrated spectrum over 5 pixels of the corresponding absorber galaxy with the redshifted Hα emission line. In that case, the continuum flux level of the galaxy does not go to zero because of the proximity of the quasar.
3.3 Q2352−0028, zabs= 1.0318 (0.8730, 1.2468)
This quasar was first discovered by Foltz et al. (1989) as part of the Large Bright Quasar Survey (LBQS). It is also part of the Early Release of the SDSS (Schneider et al. 2002). Rao et al. (2006) list three sub-DLAs with zabs= 0.8730, 1.0318, 1.2468 (see Appendix A for details on the non-prime targets).
For the zabs= 1.0318, Rao et al. (2006) measure . The corresponding EW(Mg ii 2803) = 1.714 ± 0.110 Å. Meiring et al. (2009) used 11 components to fit the absorber over Δv∼ 220 km s−1 and derive
, [Fe/H] =−0.37 ± 0.13 and [Si/H] =+0.14 ± 0.14.
In our SINFONI observations, we report the detection of the system at zabs= 1.0318. Fig. 3 shows the Hα emission map and the spectrum of this galaxy. Table 2 lists the resulting Hα flux, luminosity and SFR estimate. We report F([N ii]) = 0.6 ± 0.3 × 10−17 erg s−1 cm−2, but S ii is not detected.

Left-hand panel: the Hα emission map of the targeted absorber in the field of Q2352−0028 at zabs= 1.0318. At this redshift, 1 arcsec = 8.3 kpc. The seeing of the stacked image is 0.7 arcsec. Right-hand panel: the integrated spectrum over 5 pixels of the corresponding absorber galaxy with the redshifted Hα emission line.
4 RESULTS
4.1 Detection rate
In the sample presented here, we report the three detections out of 16 absorbers. With the caveat that the sample sizes are still small, we attempt to assess which selection criteria better optimize the Hα emission line detections from both this sample and the earlier results from our survey for galaxy counterparts to DLAs and sub-DLAs.
When combining the data of Péroux et al. (2011a) and the sample presented in this paper, we note that the mean seeing for the combined cubes is 0.9 arcsec and that this seems to play little role in the likelihood of the detections: 25 per cent (two out of eight) of the detections are done in data at >0.9 arcsec, while 21 per cent (three out of 14) are found in cubes with seeing ≤0.9 arcsec. NGS AO data, however, seem to improve slightly the detection rate from 14 per cent for no AO (two out of 14) to 37 per cent (three out of eight), because of slight increase in sensitivity.
Péroux et al. (2011a) reported the detections of one DLA and one sub-DLA out of two DLAs and four sub-DLAs. Here, we have detected two DLAs and one sub-DLA out of 11 DLAs and five sub-DLAs. Combined together, this makes the search for sub-DLAs (22 per cent) comparable to the one for DLAs (23 per cent), while there are suggestions that the former might represent more massive galaxies, with higher SFR (Khare et al. 2007; Kulkarni et al. 2007). We note however that the fraction of sub-DLAs is higher at zabs∼ 1 (seven out of 10) than at zabs∼ 2 (two out of 12), so that it is difficult to disentangle the effect of column density from a difference in redshift.
All of the quasar absorbers presented here or in Péroux et al. (2011a) have [Zn/H] abundance measurements or limits derived from high-resolution spectroscopy as described in Table 2. Treating limits as measures, we can compute the success rates for systems with [Zn/H] above or below the mean metallicity of the sample [Zn/H] =−0.65. We find 30 per cent (four out of 12) chance of detecting absorbers with for 10 per cent (one out of 10) to detect those with
. Excluding limits from the sample, the mean metallicity is then [Zn/H] =−0.50. We find 28 per cent (two out of seven) chance of detecting absorbers with
for 0 per cent (zero out of three) to detect those with
. In other words, using emission lines and looking within 6 arcsec of quasar sightlines, absorbers are seen four of five times when the abundances of Zn or Si are >1/5 solar. Four out of five detections have Hα SFRs above 1.3 M⊙ yr−1, which is a lower limit as the data for Si are incomplete. On the contrary, four systems that meet this abundance minimum do not show detections. Three of these have detection limits near our minimum detections. One of these is Q1323−0021 which has an early-type galaxy within 1 arcsec with yet unconfirmed redshift (Chun et al. 2010; Rao et al. 2011). Therefore, as advocated by others before (Møller et al. 2002), selecting systems with higher metallicity might increase the likelihood to detect Hα emission, which can be easily understood in terms of higher SFR in these systems.
Using the ratio of iron-peak elements which are mildly depleted (Zn) and refractory (Fe), one gets indications of the dust content of quasar absorbers (Pettini et al. 1994). For the undetected systems, we derive a mean (treating limits as measures) for 16 systems, while the five detected systems have
. Hence, we see a hint of a trend whereby less dusty systems are more likely to be detected. Incidentally, we note that the mean alpha-peak to iron-peak elements ratio are
for the nine undetected systems which have [Si/H] measurements, and
for the four corresponding detected ones. We note that some of the individual [Si/Fe] values are high, i.e. [Si/Fe] =+1.20 in the absorber towards Q2102−3553.
However, looking at the detection rates, we find that the factor which is the most discriminatory is the actual redshift (and therefore SINFONI observing band) of the absorber. At face value, we note that 40 per cent (four out of 10, i.e. two detections of five in Péroux et al. 2011a and two of five in this work) of the zabs∼ 1 systems are detected, while only 8 per cent (one out of 12, i.e. zero out of two in Péroux et al. 2011a and one of 11 in this work) of the zabs∼ 2 are found. These results are in line with recent reports from Bouché et al. (2011) indicating that the success rate of detections of Mg ii-selected absorbers at z∼ 2 is much lower than the detection rate at z∼ 1 (Bouché et al. 2007), taking into account the increase in luminosity distance. Bouché et al. (2011) advocate that these results cannot be explained by the galaxies hiding within the quasar image or being outside the SINFONI field of view. In the case of H i-selected absorbers, we note, indeed, that the impact parameters probed by our observational approach can be quite low (down to 6 kpc in the case of Q2222−0946), while the search radius is large compared with expectations from DLA/sub-DLA galaxies (up to 39 kpc in the case of Q1009−0026; see Péroux et al. 2011b, although see Chen et al. 2010, too). Still, in the case of Q0001−0159, van der Werf, Moorwood & Bremer (2000) have reported an unconfirmed galaxy candidate which falls just outside our SINFONI field. Nevertheless, the search radius at zabs∼ 1 and ∼ 2 are comparable (41 and 43 kpc, respectively) and cannot explain alone the difference in detection rates. These differences with redshift led Bouché et al. (2011) to conclude that z∼ 2 Mg ii absorbers reside in haloes smaller than z∼ 1 systems based on redshift evolution arguments. It is important to note that it can well be that some of the targeted galaxies are bright in the continuum but not strong emitters or are dwarf galaxies with low metallicities and low SFR which at z∼ 2 have not yet merged with bigger galaxies.
4.2 Star formation rate
In this section, we provide more details on the three Hα detections presented in this work. We estimate the Hα fluxes by extracting emission lines within a given radius. For the absorber at zabs= 1.0072 towards Q0452−1640, we use a radius of 13 pixels or 3.25 arcsec (seeing = 1.1 arcsec); for the zabs= 2.3543 system toward Q2222−0946 and the zabs= 1.0318 system toward Q2352−0028, we used 5 pixels or 1.25 arcsec to avoid contamination by the quasar nearby (seeing = 0.6 and 0.7 arcsec, respectively). The fluxes are estimated from a Gaussian fit to the emission lines and resulting values are listed in Table 2. Using the Hα luminosity, we then derive the SFR assuming the Kennicutt (1998) flux conversion corrected to a Chabrier (2003) initial mass function (IMF). These estimates are not corrected for dust extinction. Some of the SFR limits reached in that way are among the most sensitive limits obtained to date.
For the DLA with at zabs= 1.0072 towards Q0452−1640, we derive F(Hα) = 14.5 ± 4.3 × 10−17 erg s−1 cm−2. This corresponds to L(Hα) = 8.0 ± 2.4 × 1041 erg s−1 which leads to a SFR estimate of 3.5 ± 1.0 M⊙ yr−1. For the DLA with
= 20.50 ± 0.15 at zabs= 2.3543 towards Q2222−0946, we derive F(Hα) = 8.7 ± 2.6 × 10−17 erg s−1 cm−2. This corresponds to L(Hα) = 38.5 ± 1.1 × 1041 erg s−1 which leads to a SFR estimate of SFR = 17.1 ± 5.1 M⊙ yr−1. We therefore report a higher SFR than Fynbo et al. (2010) who detected this object by triangulation of X-shooter spectra in the field, but we note that their data do not cover the actual galaxy leading to important ‘slit loss’ and that their calibration was artificially decreased by 30 per cent to match the quasar flux in the Sloan spectrum. Therefore, their SFR estimate of >10 M⊙ yr−1 should be viewed as a lower limit and is consistent with our result. This object is the only z∼ 2 detected object in our H i-selected absorbers SINFONI search and one should note that the SFR is considerably higher than the one from other detections at z∼ 1, probably because of the SFR–M* sequence. Finally, for the sub-DLA with
= 19.81
at zabs= 1.0318 towards Q2352−0028, we derive F(Hα) = 4.9 ± 2.4 × 10−17 erg s−1 cm−2. This corresponds to L(Hα) = 2.8 ± 1.4 × 1041 erg s−1 which leads to a SFR estimate of SFR = 1.3 ± 0.6 M⊙ yr−1.
For all the remaining absorbers searched for, we reach stringent star formation limits. The 2.5σ upper limits for non-detections are computed for an unresolved source spread over 32 spatial pixels and spectral FWHM = 6 pixels = 9 Å. These limits are summarized in Table 2. We note that the resulting luminosity limits vary from one field to another, complicating the interpretation of detection rates.
In Fig. 4, we present the derived SFR as a function of both the N(H i) column density and metallicity for the detections and the non-detections presented in this work. The black points are for systems at z∼ 1 and the red ones for systems at z∼ 2. We note that some of the limits at z∼ 2 would not be stringent enough to reach some of the detections at z∼ 1. Within the small number of objects currently observed, we see no clear correlation between the quantities plotted, although we note that the detections were made among the most metal-rich systems. It is possible that the absence of trends is due to a dust bias where at high metallicity only systems of low N(H i) column density are observed, and at high N(H i), only objects of low metallicity are observed (see e.g. Vladilo & Péroux 2005). We note that the detection of the galaxy towards Q2222−0946 (SFR = 17.1 ± 5.1 M⊙ yr−1) is well above the typical detection limits.
![Left-hand panel: SFR as a function of H i column density for the full sample. The black points are for systems at z∼ 1 and the red ones for systems at z∼ 2. There is no clear correlation between these quantities in our sample. Right-hand panel: SFR as a function of metallicity with respect to solar as traced by dust-free [Zn/H]. We note that the detections were made among the most metal-rich systems.](https://oup.silverchair-cdn.com/oup/backfile/Content_public/Journal/mnras/419/4/10.1111_j.1365-2966.2011.19947.x/2/m_mnras0419-3060-f4.jpeg?Expires=1750197330&Signature=mBmzPupovJD7NBtene~FK8w55151iMwr40TgFgMQh60CwG~h1Mc8wUlawMFQS4TY4mczE0c8X9GGC93J5AicZgvN-DAKp9QhnnghRCxjsN04n0LUb7vh7WQlc~IM7792BX6mBA3Bnl85y1VUcXejmKfrj-XDaD5CFB5TT4Mxlh3EgVg0drIyte5x3QMDkdDksEED6zFJe3SxkwgKtYk6gKRsa8bagBw-nLsGIhh82c8EydWyKtncnTrRMf~AhNqmMUy89Scokhxh0m6LenCJfG7Up9cf85nSFHtNkkvRUDDdvxXOvnLParecZRClnZ5VXc1Fcu0TgsyYfHEJMrB48w__&Key-Pair-Id=APKAIE5G5CRDK6RD3PGA)
Left-hand panel: SFR as a function of H i column density for the full sample. The black points are for systems at z∼ 1 and the red ones for systems at z∼ 2. There is no clear correlation between these quantities in our sample. Right-hand panel: SFR as a function of metallicity with respect to solar as traced by dust-free [Zn/H]. We note that the detections were made among the most metal-rich systems.
4.3 Star formation rate per unit area
Recently, Wolfe et al. (2003a) have proposed a technique that measures SFRs in DLAs inferring the heating rate by equating it to the cooling rate measured by the strength of C ii* λ1335.7 absorption. In this way, they compute the SFR per unit area for a sample of almost 30 DLAs in which the dust-to-gas ratio has been inferred from element depletion patterns in two different models: one in which the line of sight passes through cold neutral medium (CNM; T∼ 80 K) and warm neutral medium (WNM; T∼ 8000 K) – the so-called CNM model – and another one where the line of sight passes only through WNM gas – the WNM model. They obtain SFR per unit area equals 10−2.2 M⊙ yr−1 kpc−2 (similar to the one measured in the Milky Way interstellar medium) for the CNM solution and 10−1.3 M⊙ yr−1 kpc−2 for the WNM solution. Since the heating rate is proportional to the product of the dust-to-gas ratio, the grain photoelectric heating efficiency and the SFR per unit area, the WNM solution lead to higher estimate of the SFR per unit area. One of the systems in that sample (Q1234+0758) is part of the current SINFONI survey but it remains undetected in Hα. Nevertheless, for the five detections of our survey, both the SFR and size of Hα emitting regions are measured providing a robust estimate of the SFR per unit area in the galaxies. These values are tabulated in Table 3 and shown in red in Fig. 5 as a function of absorber's redshift. These show values which are more in line with results from the WNM model but one should bear in mind that our detection limits might be above values expected from CNM model. Alternatively, we can also compute the SFR per unit area assuming a model in which the absorbers are passive probes of the radiation field at some distance taken to be the impact parameter from the central core of star formation detected in Hα (Rafelski, Wolfe & Chen 2011). These results are shown in green in Fig. 5 and can be viewed as lower limits to a comparison with results from C ii* estimates. They show values which are in agreement with results from the CNM model.
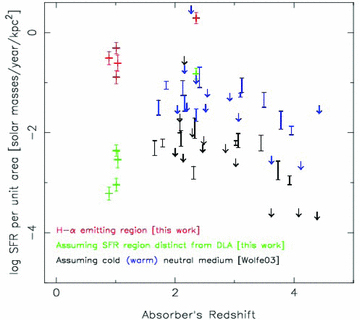
SFR per unit area in quasar absorbers as a function of redshift. The black and blue points are inferred from the two cooling rates measured by the strength of C ii* λ1335.7 absorption (Wolfe, Prochaska & Gawiser 2003a). Predictions vary according to whether a CNM (in black) or a WNM (in blue) is assumed. These results can be directly compared with our measurements of ΣSFR in the five detections presented here in red for the Hα emitting regions and in green when we assume absorbers are passive probes of the radiation field at the impact parameter distance from the detected central core of star formation traced by Hα.
In addition, in Fig. 6, we present these measures as a function of N(H i) column density and metallicity traced by [Si/H] and compare it with the sample of Wolfe et al. (2003b) assuming CNM as favoured by the authors. Our data probe lower column densities and because of our selection criterion, higher metallicities.
![Left-hand panel: SFR per unit area in quasar absorbers as a function of H i column density. Right-hand panel: SFR per unit area in quasar absorbers as a function of metallicity with respect to solar as traced by [Si/H]. The black points are inferred from C ii* measurements assuming a CNM phase which is favoured by Wolfe, Gawiser & Prochaska (2003b). Our measures of the same quantities from the SINFONI observations are presented in red for the Hα emitting regions and in green when we assume absorbers are passive probes of the radiation field at the impact parameter distance from the detected central core of star formation traced by Hα.](https://oup.silverchair-cdn.com/oup/backfile/Content_public/Journal/mnras/419/4/10.1111_j.1365-2966.2011.19947.x/2/m_mnras0419-3060-f6.jpeg?Expires=1750197330&Signature=uTMbvM3gM8pVNS34u~TorArDlntwQw2FO1movTWdbwGaFnJYEwZaajohPYGIfHKDcrpfZ0TZFbyKanhJzSjbSGokYRe0IthMKSy0GxBlYhlWlp5LaTMz8yInwcZsgt51k0ODnGEn~34Gbi5cIckKjh7yfzV7DFXjdYxh~rCfy1Cvs8u2BvaikRUTtebD7nVwCZtECbgQc0ntCcS~kABQEJYSMGV3-U50COiObuK56zcKYfSouRKeJlslRoTDoHFRUk-TBbcK~XyxaSJfjZCjY4TOkqhrIf~GVHZVsyMTvvlmuMWz73wqiP3ahrz6Ck2R2VBpfIFw~op2H-GpNTRoxA__&Key-Pair-Id=APKAIE5G5CRDK6RD3PGA)
Left-hand panel: SFR per unit area in quasar absorbers as a function of H i column density. Right-hand panel: SFR per unit area in quasar absorbers as a function of metallicity with respect to solar as traced by [Si/H]. The black points are inferred from C ii* measurements assuming a CNM phase which is favoured by Wolfe, Gawiser & Prochaska (2003b). Our measures of the same quantities from the SINFONI observations are presented in red for the Hα emitting regions and in green when we assume absorbers are passive probes of the radiation field at the impact parameter distance from the detected central core of star formation traced by Hα.
4.4 Velocity shift and impact parameter
Thanks to the Hα maps, we are able to accurately report the sky position and impact parameters between the quasar's line of sight and the centre of the detected galaxies. Table 3 summarizes these properties. Once again, one can see that IFU observational approach allows us to probe impact parameters which can be quite small (down to 6 kpc in the case of the absorber towards Q2222−0946). The three detections reported here have indeed impact parameters lower than the one found in Péroux et al. (2011b). In addition, we compute the observed velocity shift between the absorber and the detected galaxies, Δv with an accuracy of about 30 km s−1. In some cases, the large values observed suggest that some of the absorption features observed might be due to material outflowing from the galaxy itself. If true, the kinematic observations would allow us to make an estimate of the rate of the outflowing material. In the case of Q2222−0946, Fynbo et al. (2010), who rely on a X-shooter spectrum for both the emission and absorption redshift determination, derive from the Hα, an emission redshift z= 2.3537, resulting in Δv= 35 ± 10 km s−1 (Fynbo, private communication). Our estimate is in good agreement with this value. It is interesting to note however that their emission redshift determination based on [O iii] z= 2.35406 is in better agreement with the redshift of low-ionization absorption lines.
In Fig. 7, we plot SFR, log N(H i) and metallicity, as a function of both the velocity shift, Δv, between the galaxy and the absorbing material seen along the quasar's line of sight and the impact parameter. In spite of the very small size of the sample, the range of impact parameter is relatively large, spanning from few kpc to more than 125 kpc. So, in principle, we may probe the outskirts and surroundings of the main galaxy. The effect the most expected in this figure (Rao et al. 2011) would be a decrease of N(H i) with impact parameter and there is indeed a hint of that effect (Spearman's rank correlation coefficient rs=− 0.56), to be confirmed by a larger data set. Similarly, we see no clear hint of decreasing SFR with impact parameter, although the highest SFR is at the smallest impact parameter. The metallicity [Zn/H] is more strongly correlated with Δv (rs= 0.81) and the impact parameter (rs= 0.70). Assuming some of the material is outflowing, Δv, in combination with the known orientation of galaxy from SINFONI observation, will allow us to interpret the high-resolution absorption profile kinematics for these objects.
![Top panels: SFR, log of H i column density and metallicity with respect to solar as traced by dust-free [Zn/H] as a function of the velocity shift between the emitting galaxy and the absorbing material seen along the quasar's line of sight for the five systems detected in our SINFONI survey. The black points are for systems at z∼ 1 and the red one for the system at z∼ 2. Bottom panels: same quantities as a function of impact parameter.](https://oup.silverchair-cdn.com/oup/backfile/Content_public/Journal/mnras/419/4/10.1111_j.1365-2966.2011.19947.x/2/m_mnras0419-3060-f7.jpeg?Expires=1750197330&Signature=IPkOWCVh2Korhc3jqqT7lAIYC3p9XjePRxxSFRPqVmje2CupD2RTkAx8uUHGXaJ7Wh6LjjTxa--cD~cUHcU0wQ~F~Af1GfRSAH~2~yuLra3K-suI6SY4AP7ip8kRuJvAt-2-8nxKkIf58uRNqunPsjiqrJU2BHtx~lNBEB7FxC6PNacK1YzivjoA6yM5cQsoVujtTm~uSvKhTA6jvnOSfMn~MXfVmV0hezUNCvWdu3Eg3pAZC4U4zQQ6AgXQJmmWS6y4VAhpFkx~8yvFW0nZMM39z5x5thJJLohKfIadG5cZ6msidtuM3WX6St5j87x644hJ9FWm4-M8Lq7eeb0cSg__&Key-Pair-Id=APKAIE5G5CRDK6RD3PGA)
Top panels: SFR, log of H i column density and metallicity with respect to solar as traced by dust-free [Zn/H] as a function of the velocity shift between the emitting galaxy and the absorbing material seen along the quasar's line of sight for the five systems detected in our SINFONI survey. The black points are for systems at z∼ 1 and the red one for the system at z∼ 2. Bottom panels: same quantities as a function of impact parameter.
4.5 Metallicity
Using the N2 parameter (Pettini & Pagel 2004) based on [N ii] λ6585/Hα ratio, we can derive an estimate of the emission metallicity. Such observations of the absorption and emission metallicities in the same high-redshift object allow us to trace the two different gas phases (neutral versus ionized gas). For the DLA towards Q0452−1640, we find 12 + log(O/H) = 8.4 ± 0.1, i.e. slightly less than solar3 compared to the one-tenth solar absorption metallicity reported in absorption ([Zn/H] =−0.96 ± 0.08). For the DLA towards Q2222−0946, we find 12 + log(O/H)<8.2 from a non-detection, i.e. below solar compared with the one-third absorption metallicity ([Zn/H] =−0.46 ± 0.07). Finally, for the sub-DLA towards Q2352−0028, 12 + log(O/H) = 8.4 ± 0.3, i.e. slightly less than solar compared to the less than one-third solar absorption metallicity reported in absorption (). These values are summarized in Table 3.
Following our investigations in Paper I, we compare the metallicity with respect to solar as a function of impact parameters. Table 4 lists the absorption and emission abundances for the quasar absorbers for which both measures are available. Fig. 8 illustrates the observed gradients derived from these observations. The two metallicities arising from the same galaxy are linked by a line. The data from our survey have more than doubled the number of systems for which such measures are possible. Out of the eight galaxy counterparts to quasar absorber, two have abundances at the impact parameter which are slightly higher than in the centre of the galaxy, although the statistical significance of the results is limited given the large error estimates in the emission metallicity measurements (Table 4). These results could be due to a patchy metals distribution across the galaxies, but we note that ‘inverted gradients’ have been recently reported in the literature as a signature of primordial gas infall on to galaxies (Cresci et al. 2010). Based on a larger sample of metallicity gradients from the MASSIV survey (Contini et al. 2012), Queyrel et al. (2012) suggest that the gas infall might be due to either interactions or cold gas accretion. We also note that using lensed galaxies to study metallicity gradients allows us to probe smaller scales (Jones et al. 2010).
Metallicity with respect to solar measured in absorption at given impact parameter and in emission.
Quasar . | Phys dist . | Absorption abundance . | Ion . | Emission metallicitya . | Gradients . | Reference . |
---|---|---|---|---|---|---|
(kpc) . | (X/H) . | X . | 12+log(O/H) . | (dex kpc−1) . | ||
HS 1543+5921 | 0.4 | −0.41 ± 0.06 | S | −0.54 ± 0.20 | +0.32 ± 0.21 | Bowen et al. (2005) |
Q1009−0026 | 39 | +0.25 ± 0.06 | Zn | +0.04 ± 0.80 | +0.01 ± 0.80 | This work |
AO0235+164 | 7 | −1.80 ± 0.40 | Fe | −0.24 ± 0.15 | −0.22 ± 0.43 | Chen, Kennicutt & Rauch (2005) |
Q0302−223 | 25 | −0.51 ± 0.12 | Zn | < −0.06 | > −0.02 | This work |
PKS 0439−433 | 7 | −0.72 ± 0.12 | Fe | +0.45 ± 0.15 | −0.17 ± 0.19 | Chen et al. (2005) |
Q0827+243 | 36 | −1.01 ± 0.11 | Fe | > +0.06 | < −0.03 | Chen et al. (2005) |
Q0452−1640 | 16 | −0.96 ± 0.08 | Zn | −0.26 ± 0.01 | −0.04 ± 0.08 | This work |
Q2222−0946 | 6 | −0.46 ± 0.07 | Zn | < −0.46 | > −0.00 | This work |
Q2352−0028 | 12 | < −0.51 | Zn | −0.26 ± 0.03 | < −0.02 | This work |
Quasar . | Phys dist . | Absorption abundance . | Ion . | Emission metallicitya . | Gradients . | Reference . |
---|---|---|---|---|---|---|
(kpc) . | (X/H) . | X . | 12+log(O/H) . | (dex kpc−1) . | ||
HS 1543+5921 | 0.4 | −0.41 ± 0.06 | S | −0.54 ± 0.20 | +0.32 ± 0.21 | Bowen et al. (2005) |
Q1009−0026 | 39 | +0.25 ± 0.06 | Zn | +0.04 ± 0.80 | +0.01 ± 0.80 | This work |
AO0235+164 | 7 | −1.80 ± 0.40 | Fe | −0.24 ± 0.15 | −0.22 ± 0.43 | Chen, Kennicutt & Rauch (2005) |
Q0302−223 | 25 | −0.51 ± 0.12 | Zn | < −0.06 | > −0.02 | This work |
PKS 0439−433 | 7 | −0.72 ± 0.12 | Fe | +0.45 ± 0.15 | −0.17 ± 0.19 | Chen et al. (2005) |
Q0827+243 | 36 | −1.01 ± 0.11 | Fe | > +0.06 | < −0.03 | Chen et al. (2005) |
Q0452−1640 | 16 | −0.96 ± 0.08 | Zn | −0.26 ± 0.01 | −0.04 ± 0.08 | This work |
Q2222−0946 | 6 | −0.46 ± 0.07 | Zn | < −0.46 | > −0.00 | This work |
Q2352−0028 | 12 | < −0.51 | Zn | −0.26 ± 0.03 | < −0.02 | This work |
The emission metallicities are derived from R23 (Pagel et al. 1979) except for objects studied in this work where we used N2 (Pettini & Pagel 2004).
Metallicity with respect to solar measured in absorption at given impact parameter and in emission.
Quasar . | Phys dist . | Absorption abundance . | Ion . | Emission metallicitya . | Gradients . | Reference . |
---|---|---|---|---|---|---|
(kpc) . | (X/H) . | X . | 12+log(O/H) . | (dex kpc−1) . | ||
HS 1543+5921 | 0.4 | −0.41 ± 0.06 | S | −0.54 ± 0.20 | +0.32 ± 0.21 | Bowen et al. (2005) |
Q1009−0026 | 39 | +0.25 ± 0.06 | Zn | +0.04 ± 0.80 | +0.01 ± 0.80 | This work |
AO0235+164 | 7 | −1.80 ± 0.40 | Fe | −0.24 ± 0.15 | −0.22 ± 0.43 | Chen, Kennicutt & Rauch (2005) |
Q0302−223 | 25 | −0.51 ± 0.12 | Zn | < −0.06 | > −0.02 | This work |
PKS 0439−433 | 7 | −0.72 ± 0.12 | Fe | +0.45 ± 0.15 | −0.17 ± 0.19 | Chen et al. (2005) |
Q0827+243 | 36 | −1.01 ± 0.11 | Fe | > +0.06 | < −0.03 | Chen et al. (2005) |
Q0452−1640 | 16 | −0.96 ± 0.08 | Zn | −0.26 ± 0.01 | −0.04 ± 0.08 | This work |
Q2222−0946 | 6 | −0.46 ± 0.07 | Zn | < −0.46 | > −0.00 | This work |
Q2352−0028 | 12 | < −0.51 | Zn | −0.26 ± 0.03 | < −0.02 | This work |
Quasar . | Phys dist . | Absorption abundance . | Ion . | Emission metallicitya . | Gradients . | Reference . |
---|---|---|---|---|---|---|
(kpc) . | (X/H) . | X . | 12+log(O/H) . | (dex kpc−1) . | ||
HS 1543+5921 | 0.4 | −0.41 ± 0.06 | S | −0.54 ± 0.20 | +0.32 ± 0.21 | Bowen et al. (2005) |
Q1009−0026 | 39 | +0.25 ± 0.06 | Zn | +0.04 ± 0.80 | +0.01 ± 0.80 | This work |
AO0235+164 | 7 | −1.80 ± 0.40 | Fe | −0.24 ± 0.15 | −0.22 ± 0.43 | Chen, Kennicutt & Rauch (2005) |
Q0302−223 | 25 | −0.51 ± 0.12 | Zn | < −0.06 | > −0.02 | This work |
PKS 0439−433 | 7 | −0.72 ± 0.12 | Fe | +0.45 ± 0.15 | −0.17 ± 0.19 | Chen et al. (2005) |
Q0827+243 | 36 | −1.01 ± 0.11 | Fe | > +0.06 | < −0.03 | Chen et al. (2005) |
Q0452−1640 | 16 | −0.96 ± 0.08 | Zn | −0.26 ± 0.01 | −0.04 ± 0.08 | This work |
Q2222−0946 | 6 | −0.46 ± 0.07 | Zn | < −0.46 | > −0.00 | This work |
Q2352−0028 | 12 | < −0.51 | Zn | −0.26 ± 0.03 | < −0.02 | This work |
The emission metallicities are derived from R23 (Pagel et al. 1979) except for objects studied in this work where we used N2 (Pettini & Pagel 2004).
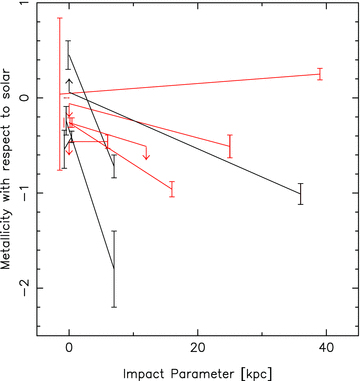
Metallicity with respect to solar measured in emission at zero impact parameters and in absorption at given impact parameters in kpc. The two metallicities arising from the same galaxy are linked by a line. The data from our survey (shown in red) have more than doubled the number of systems for which such measures are possible. The arrows indicate upper and lower limits on the measure of the metallicities. The errors on the emission metallicities are artificially offset from zero impact parameter for clarity.
5 CONCLUSION
In this study, we report three additional SINFONI detections of DLA/sub-DLAs, two of which are new identifications. Together with our earlier results of Péroux et al. (2011b), we therefore have detected five absorbers with , in comparison with 20 such detections at all redshifts reported over several years of dedicated searches (Chen et al. 2003; Rao et al. 2003; Rosenberg & Schneider 2003; Fynbo et al. 2010, 2011). With the limitation of the small number statistics, we find that selecting systems with higher metallicity might increase the likelihood to detect Hα emission. In line with the results of a similar study of Mg ii absorbers obtained by Bouché et al. (2011), we find that the detection rate is significantly lower at zabs∼ 2 than at zabs∼ 1.
For the five absorbers which are detected, we are able to measure SFR, SFR per unit area, impact parameter to the quasar line of sight, velocity shift between absorption and emission redshift and emission metallicities. We derive SFR of a few M⊙ yr−1 at zabs∼ 1 and SFR = 17 M⊙ yr−1 for the DLA at zabs∼ 2. Our estimates of the SFR per unit area are compared with results from Wolfe et al. (2003a) who inferred these from C ii* absorption. Our observations show values which are more in line with results from their WNM model than the cold one. The detected galaxies are at impact parameters ranging from 6 to 12 kpc from the quasar's line of sight. We note again that the IFU technique allows detections at small impact parameter to the quasar. Indeed, one of the detection reported here is at zabs∼ 2 and was detectable in spite of being only 0.7 arcsec away from the quasar. As found by others (Rao et al. 2011), we see a decrease of N(H i) with impact parameter. Finally, our observations of the absorption and emission metallicities in the same high-redshift object allow us to trace the two different gas phase (neutral versus ionized gas). The data from our survey have more than doubled the number of systems for which such measures are possible. Out of the eight galaxy counterparts to quasar absorbers, two might have ‘inverted gradients’, where the abundance at the impact parameter is higher than in the centre of the galaxy.
All of these absorbers have high-resolution quasar spectra and in future papers, we will be able to undertake detailed studies of the absorption/emission metallicities (i.e. Kacprzak et al. 2011) and kinematics of these objects. The growing amount of data which is currently being assembled will allow us to undertake direct comparison on the nature of DLAs and sub-DLAs with expectations from dedicated cosmological models (Tescari et al. 2009; Cen 2010; Hong et al. 2010).
Footnotes
We use the usual definition [ X/H ] = log (X/H) − log (X/H)⊙.
See the following URL for more information: http://www.eso.org/sci/facilities/paranal/instruments/sinfoni/
The solar abundance is 12 + log(O/H)⊙= 8.66 (Asplund et al. 2004).
ACKNOWLEDGMENTS
We would like to thank the Paranal and Garching staff at ESO for performing the observations in Service Mode and the instrument team for making a reliable instrument. CP thanks Johan Fynbo, Max Pettini, Thierry Contini, Chris Howk and Art Wolfe for useful discussions and Thomas Ott for developing and distributing the QFitsView software. VPK acknowledges partial support from the US National Science Foundation grants AST-0908890 (PI: Kulkarni). This work has benefited from support of the ‘Agence Nationale de la Recherche’ with reference ANR-08-BLAN-0316-01.
REFERENCES
Appendix
APPENDIX A: NOTE ON INDIVIDUAL FIELDS WITH NO DETECTIONS
A1 Q0001−0159, zabs= 2.0950 (0.871, 2.1539)
Smith, Cohen & Bradley (1986) first reported two absorbers along this line of sight at zabs= 2.0950 and 2.1539.
For the first system, Prochaska & Wolfe (1999) derive from HIRES spectroscopy: = 20.70 ± 0.10, [Al/H] =−1.518 ± 0.106, [Si/H] =−0.842 ± 0.102, [Cr/H] =−1.632 ± 0.135, [Ni/H] =−1.808 ± 0.114, [Fe/H] =−1.703 ± 0.103 and [Zn/H] =−0.755 ± 0.104.
For the other system, Prochaska & Wolfe (1999) derive from HIRES spectroscopy: = 20.30 ± 0.10, [Al/H] =−1.615 ± 0.101, [Si/H] =−1.534 ± 0.101, [Cr/H] < −1.034,
, [Fe/H] =−1.915 ± 0.105 and
.
Meyer & Roth (1990) also identify a Mg ii and Fe ii system at zabs= 0.871.
Bunker et al. (1999) used long-slit K-band spectroscopic on United Kingdom Infrared Telescope (UKIRT) to try and detect the galaxies responsible for these two absorbers. They did not find any leading to upper limits of F(Hα) < 10.5 × 10−17 erg s−1 cm−2, i.e. SFR < 7.23 h−2 M⊙ yr−1 at zabs= 2.0950 and F(Hα) < 10.0 × 10−17 erg s−1 cm−2, i.e. SFR < 7.33 h−2 M⊙ yr−1 at zabs= 2.1539.
However, van der Werf et al. (2000) report a galaxy candidate consistent with z= 2.1539 from IR narrow-band flux at Hα with K broad-band continuum measurement. The object detected 7.7 arcsec south-west of the quasar has F(Hα) = 1.8 × 10−16 erg s−1 cm−2 corresponding to SFR = 23 h−2 M⊙ yr−1. Unfortunately, this region is just outside our SINFONI field. Colbert & Malkan (2002) also used Near Infrared Camera and Multi-Object Spectrometer (NICMOS) to image this field and reach H magnitudes of 20.9 at zabs= 2.0950 and 21.0 at zabs= 2.1539 without detecting any galaxy candidates.
Therefore, we do not detect either the galaxy responsible for the system at zabs= 2.1539 or the main target at zabs= 2.0950 in our SINFONI data. For the latter, we derive F(Hα) < 4.5 × 10−17 erg s−1 cm−2, i.e. SFR < 11 M⊙ yr−1. Finally, the Hα emission line of the Mg ii system at zabs= 0.871 is not covered by our K-band data.
A2 Q1211+0902, zabs= 2.5841 (1.2650, 2.33, 3.1919)
This high-redshift quasar was first reported by Hazard, McMahon & Morton (1987) but is also part of the Data Release 3 of the SDSS (Richards et al. 2006). From an early Anglo-Australian Telescope (AAT) spectrum, the former authors suspected the presence of a strong N(H i) absorber at zabs= 2.5841. The N(H i) column density was measured to be = 21.40 ± 0.10 by Ledoux et al. (2006). The Zn ii metallicity of that system was first studied by Pettini & Bowen (1997), the C iv profile associated was studied in detail by Fox et al. (2007), while Prochaska et al. (2007) used ESI/HIRES Keck Telescope spectra to measure the abundances of several lines in this system. They derive
, [Si/H] =−1.05 ± 0.11, [Cr/H] =−1.48 ± 0.15, [Fe/H] =−1.65 ± 0.13, [N/H] =−2.22 ± 0.12 and [Zn/H] =−1.09 ± 0.12. As usual in quasar absorbers, the [N/H] values are low (Pettini et al. 2002). The strong lines are spread over ∼600 km s−1. The Mg ii lines are not covered by these spectra. Petitjean et al. (2006) have reported the upper limit of the molecular hydrogen content of that absorber to be log f < −5.69. The abundance pattern in this system is interesting, since both Si and Fe are more depleted with respect to Zn, suggesting the presence of dust, yet no H2 was detected.
Lanzetta et al. (1991) reported from a Las Campanas 2.5-m-telescope spectrum, an additional absorber at zabs= 2.33 which was believed to be below the canonical DLA definition. Narayanan et al. (2008) have also found an EW(Mg ii 2796) = 0.083 ± 0.07 Å Mg ii system at zabs= 1.2650. Finally, Ryabinkov, Kaminker & Varshalovich (2007) mention an additional C iv and Si ii absorption feature at zabs= 3.1919.
Lowenthal et al. (1995) have taken Fabry–Perot observations of that field but do not detect the galaxy counterpart to the main absorber at zabs= 2.5841, providing an upper limit F(Lyα) < 3 × 1017 erg s−1 cm−2 at 3σ, corresponding to SFR < 1 M⊙ yr−1. Colbert & Malkan (2002) subsequently used NICMOS imaging in this field but do not report detection of the galaxy responsible for the quasar absorber. Similarly, we do not detect the Hα line in our SINFONI data. We derive F(Hα) < 2.3 × 1017 erg s−1 cm−2, corresponding to SFR < 5.6 M⊙ yr−1.
For the non-prime targets, only the zabs= 2.33 system is covered by our SINFONI data but it remains undetected.
A3 Q1220−0040, zabs= 0.9746
This quasar is part of the Early Release of the SDSS (Schneider et al. 2002). An absorber at zabs= 0.9746 was reported by Rao et al. (2006). They measure = 20.20
with EW(Mg ii 2803) = 1.793 ± 0.125 Å. Meiring et al. (2008) subsequently estimate the abundances in this absorber using the MIKE spectrograph. They use nine components over Δv∼ 300 km s−1 to derive
and [Fe/H] =−1.33 ± 0.07.
The galaxy responsible for this absorber is not detected in our SINFONI data.
A4 Q1225+0035, zabs= 0.7731
This quasar is part of the Early Release of the SDSS (Schneider et al. 2002). An absorber at zabs= 0.7731 was reported by Rao et al. (2006). They measure = 21.38
with EW(Mg ii 2803) = 1.447 ± 0.150 Å. Meiring et al. (2006) subsequently estimate the abundances in this absorber using the Multiple Mirror Telescope (MMT). They use one-component fit to derive [Fe/H] =−1.19 ± 0.015 and [Cr/H] =−1.08 ± 0.22. In addition, Nestor et al. (2008) derive [Zn/H] =−0.78 ± 0.14.
Recently, based on J, H and K imaging, Rao et al. (2011) report a galaxy candidate in that field at impact parameter of 8.2 arcsec (60.8 kpc). The identification is based on proximity to the quasar, but no photometric redshift could be established. This object falls outside our SINFONI field of view and the galaxy responsible for this absorber is not detected in our data.
A5 Q1226+1736, zabs= 2.5576 (2.4658)
This quasar was first discovered as part of the LBQS. Pettini et al. (1994) used a WHT spectrum to measure = 21.52 ± 0.10 at zabs= 2.4658 and to study the Zn ii metallicity of this absorber. Petitjean, Srianand & Ledoux (2000) report an H2 fraction log f < −6.92. Prochaska et al. (2001) have reported the metallicity of that absorber based on Keck/HIRES observations, they derive
,
, [Si/H] =−1.59 ± 0.10, [Cr/H] =−1.68 ± 0.10, [Fe/H] =−1.84 ± 0.10, [Ni/H] =−1.80 ± 0.10 and [Zn/H] =−1.62, suggesting little dust in this system, but 1/60th solar abundance attributable to nucleosynthetic element production. The profiles for this system are spread over 100 km s−1 (Prochaska & Wolfe 2002).
Dessauges-Zavadsky et al. (2003) have also reported a sub-DLA towards this quasar at zabs= 2.5576 with = 19.32 ± 0.15. They fitted the absorber with 11 components over ∼200 km s−1 and derived [O/H]<−0.77, [Si/H] =−0.50 ± 0.15, [Al/H] =−0.61 ± 0.15, [Fe/H] =−0.84 ± 0.15,
and
, indicating modest nucleosynthesis products with no indication of dust. The low [O/Si] ratio might indicate that the system is ionized, not neutral, in O and H or that the system is Si rich.
Warren et al. (2001) did not find candidates in that field in their NICMOS search down to HAB= 25.0. Similarly, we do not detect the Hα lines of any of these absorbers in the shallow SINFONI data available to us.
A6 Q1234+0758, zabs= 2.3376
This quasar was discovered by Foltz et al. (1987) and subsequently observed for its absorber at zabs= 2.3376 by several authors. Notably, the HD molecule was reported in that system by Varshalovich et al. (2001) to have N(HD) = 1–4 × 1014 and the H2 molecule was found by Ge, Bechtold & Kulkarni (2001) to have N(H2) = 3 × 10 depending on the precise value assumed for the Doppler parameter. Ge et al. (2001) also derived [Zn/H] =−0.86 ± 0.15. This system has been later reviewed by Srianand et al. (2005) who derive log N(H2) = 19.57 ± 0.12. The system has then been studied at higher resolution with UVES by Centurión et al. (2003) who fitted six components over Δv∼ 140 km s−1:
= 20.80 ± 0.10, [N/H] =−2.10 ± 0.13, [Si/H] =−1.18 ± 0.13, [S/H] =−1.17 ± 0.14 and [Fe/H] =−1.59 ± 0.13. These values again indicate modest nucleosynthesis production and modest dust depletion.
The galaxy responsible for this absorber is not detected in our SINFONI data.
A7 Q1356−1101, zabs= 2.5009 (2.9668)
This quasar was part of the Parkes radio survey and its absorber was first reported by Ellison et al. (2001) as part of the Complete Optical and Radio Absorption Line System (CORALS) survey which aimed at looking for absorbers in radio-selected quasars. These authors derived a column density of = 20.40 ± 0.49. Akerman et al. (2005) measure the metallicity by fitting at zabs= 2.5009:
, [Cr/H] =−1.18 and [Fe/H] =−1.25. Noterdaeme et al. (2008) derive a molecular fraction log f < −5.18 and report a Δv= 71 km s−1 for the metal lines.
Ellison et al. (2001) also reported an absorber associated with the quasar itself at zabs= 2.9668 with = 20.78 ± 0.47. Akerman et al. (2005) analysed this absorber and derive
, [Fe/H] =−1.54 and [Si/H] =−1.31. Noterdaeme et al. (2008) derive a molecular fraction log f < −6.75 and report a Δv= 30 km s−1 for the metal lines.
This latter object is not covered by our SINFONI data, while the former galaxy responsible for the prime absorber at zabs= 2.5009 is not detected.
A8 Q1454+1210, zabs= 2.2550 (2.4690, 3.1717)
The absorption-line systems towards this quasar discovered by Hazard et al. (1986), have first been studied by Wolfe et al. (1986). The DLA at zabs= 2.4690 was reported by Lanzetta et al. (1991) as well as two other absorption systems at zabs= 2.2550 and 3.1717.
Petitjean et al. (2000) studied the metal content of the DLA at zabs= 2.4690 with = 20.39 ± 0.10. They found [Fe/H] =−2.54 ± 0.12 and [Zn/H] =−1.95 ± 0.16. Noterdaeme et al. (2008) state [Si/H] =−2.27 ± 0.13 over Δv= 35 km s−1 and log f < −4.50.
Dessauges-Zavadsky et al. (2003) have made a detailed study of the zabs= 2.2550 system and measure . These authors fitted five components spread over ∼130 km s−1. They obtained
, [Fe/H] =−1.47 ± 0.17,
, [Zn/H] =−1.12 ± 0.19 and [Cr/H] =−1.11 ± 0.20. We note that in this system, as for the absorber at zabs= 2.6147 towards Q2350−0052, [Si/H] is higher than [Zn/H], which is unusual. The Mg ii doublet is also covered but is so highly saturated at the UVES resolution so that no useful lower limit can be deduced.
Dessauges-Zavadsky et al. (2003) also include a detailed study of the zabs= 3.1717 system for which the N(H i) is not covered. Petitjean et al. (2000) estimated the hydrogen column density of this system from the equivalent width measurement by Bechtold (1994) and give = 19.70. Dessauges-Zavadsky et al. (2003) fitted two components spread over ∼25 km s−1. They obtained
, [C/H] =−1.92 ± 0.26, [Si/H] =−1.62 ± 0.15, [Al/H] =−1.88 ± 0.15, [Fe/H] =−1.87 ± 0.16,
and [Cr/H] <−0.74, the last two values from non-detections. These indicate low-nucleosynthesis products without any indication of dust. The Mg ii doublet is not covered by the UVES spectrum.
Warren et al. (2001) searched for the galaxy counterpart to the main DLA at zabs= 2.4690 but do not find candidates in that field in their NICMOS search down to HAB= 25.0. Christensen et al. (2007) also studied that field to search the three absorbers with IFU Potsdam Multi-Aperture Spectrophotometer (PMAS) but report candidates that have not been subsequently followed-up for confirmation. Similarly, our SINFONI observations show no indication of a Hα emission at that redshift, nor at zabs= 2.2550. Note that our data do not cover the Hα emission line of the zabs= 3.1717 absorber.
A9 Q1631+1156, zabs= 0.9008 (0.5316, 1.3786)
Junkkarinen, Hewitt & Burbidge (1991) reported a Mg ii absorber at zabs= 0.5316 and another one zabs= 0.9008. Aldcroft, Bechtold & Elvis (1994) mention a C iv absorber at zabs= 1.3786 and confirm the Mg ii and Fe ii lines at the position reported by Junkkarinen et al. (1991) with EW(Mg ii 2803) = 1.35 ± 0.07 Å and EW(Mg ii 2803) = 0.69 ± 0.09 Å (see also Rao et al. 2006). The H i column density of the zabs= 0.5316 was measured by Rao et al. (2003) to have from HST/Space Telescope Imaging Spectrograph (STIS) observations, while the one at zabs= 0.9008 has
. Rao et al. (2003) also obtained UBRJK images of the field and identified a disc galaxy at 3.2 arcsec away from the background quasar. They found that the observed spectral energy distribution (SED) of the galaxy may be described by a young galaxy at zem= 0.5316 that had recently undergone a single burst of star formation. Follow-up spectroscopy confirms that this galaxy is at zem= 0.529 (Chen & Lanzetta 2003) and the corresponding projected distance is ρ= 14.1 h−1 kpc. The redshifted Hα line of this absorber is not covered by our SINFONI data, as is also the case for the zabs= 1.3786 system.
For the system at zabs= 0.9008, Ellison (2006) report [Zn/H] =−0.18 and [Fe/H] =−0.86. Meiring et al. (2008) used higher resolution data from the MIKE spectrograph to study this system and derive [Fe/H] =−1.06 ± 0.06 from fitting six components and from non-detection of the lines. Rao et al. (2003) also searched for the zabs= 0.9008 system in their images but do not detect it. They derive a surface brightness limit of μK > 21.6 mag per arcsec−2. These authors also consider that the quasar point spread function (PSF) was subtracted reasonably cleanly, since the PSF was well defined by several stars brighter than the quasar in each frame. The galaxy responsible for this absorber was the main target of our SINFONI search but its Hα line remains undetected.
A10 Q2059−0528, zabs= 2.2100
This is another quasar discovered as part of the SDSS survey and reported in its Data Release 1 (Schneider et al. 2003). Herbert-Fort et al. (2006) have studied this system at zabs= 2.210 in detail and report [Si/H] =−1.00 ± 0.30. Prochaska et al. (2007) list = 20.80 ± 0.20 and [Zn/H] =−0.53 ± 0.11, [Fe/H] =−1.30 ± 0.11. The Mg ii doublet is not covered by these data.
The galaxy responsible for this absorber is not detected in our SINFONI data.
A11 Q2102−3553, zabs= 2.5070 (1.2430, 1.3999, 3.0826)
This quasar was discovered by Warren, Hewett & Osmer (1991). Dessauges-Zavadsky et al. (2003) studied its intervening sub-DLA system at zabs= 2.5070 from UVES spectroscopy and derive = 20.21 ± 0.10. The metal lines are well fitted by two components over Δv= 20 km s−1: [O/H] =−1.50 ± 0.24, [C/H] =−1.86 ± 0.22, [Si/H] =−1.04 ± 0.15, [N/H] =−3.47 ± 0.21, [Fe/H] =−2.24 ± 0.10,
0.44 ± 0.14 and [Cr/H] =−0.83 ± 0.16. The Mg ii doublet is not covered by this UVES spectrum.
Narayanan et al. (2007) report two weak Mg ii doublets: one at zabs= 1.2430 with EW(Mg ii 2796) = 0.015 ± 0.001 Å and EW(Mg ii 2803) = 0.010 ± 0.002 Å as well as another at zabs= 1.3999 with EW(Mg ii 2796) = 0.109 ± 0.002 Å and EW(Mg ii 2803) = 0.061 ± 0.003 Å.
Finally, Petitjean, Ledoux & Srianand (2008) studied the molecular content of the DLA at zabs= 3.0826 and derive = 20.98 ± 0.08. They also report three-component fits leading to [Fe/H] =−1.97 ± 0.08, [O/H] =−1.58 ± 0.09, [N/H] =−2.86 ± 0.08, [S/H] =−1.76 ± 0.09 and [Si/H] =−1.66 ± 0.09. Again, this indicates low nucleosynthetic production and little dust. The Mg ii doublet is not covered by this UVES spectrum.
Pettini et al. (1995) first reported the detection of the Lyα emission in the trough of the absorber at zabs= 3.0826 and derive F(Lyα) = 7 × 10−17 erg s−1 cm−2 corresponding to a luminosity L(Lyα) = 5 × 1042 erg s−1 with a shift of +470 km s−1. Leibundgut & Robertson (1999) confirm this Lyα feature described by two components separated by 5 Å and found the shift to be of the order +490 km s−1. Nevertheless, the Hα line of that prime target is not detected in our SINFONI data leading to F(Hα) < 1.2 × 10−17 erg s−1 cm−2, corresponding to SFR < 2.8 M⊙ yr−1. These results indicate a ratio Lyα/Hα > 6, i.e. consistent with the Lyα case B recombination rate.
The other absorbers are not covered by our observations.
A12 Q2222−0946, zabs= 2.3541 (2.8647)
A C iv absorber is reported at zabs= 2.8647 from these data (York et al., private communication) but the emission lines of the C iv absorber at zabs= 2.8647 are not covered by our observations.
A13 Q2313−3704, zabs= 2.1821 (0.3399)
Again, this quasar was part of the Parkes radio survey and its absorber was first reported by Ellison et al. (2001) as part of the CORALS survey. These authors derived a column density of = 20.48 ± 0.10. Akerman et al. (2005) measure the metallicity by fitting at zabs= 2.1821:
1.29,
, [Fe/H] =−1.70 and [Si/H] =−1.52. Noterdaeme et al. (2008) derive a molecular fraction log f < −5.00 and report a Δv= 77 km s−1 for the metal lines.
Mshar et al. (2007) report another Mg ii absorber with associated Fe ii at zabs= 0.3399. They report N(Fe ii) > 14.41 and N(Mg ii) > 14.07.
The galaxy responsible for the main absorber at zabs= 2.1821 is not detected in our SINFONI data, while the Mg ii system at zabs= 0.3399 is not covered by our data at the Hα position.
A14 Q2350−0052, zabs= 2.6147 (0.8629, 1.0793, 2.0470, 2.4263, 2.9298)
There are two DLAs at zabs= 2.4263 and 2.6147 toward Q2350−0052. These DLAs were discovered as strong Lyα absorptions in a low spectral-resolution (∼5 Å) spectrum by Sargent, Steidel & Boksenberg (1989).
Prochaska et al. (2001) studied the system at zabs= 2.4263 with HIRES spectra and derive = 20.50 ± 0.10, [Al/H] >−1.051, [Si/H] =−0.695 ± 0.102,
, [Fe/H] =−1.386 ± 0.101 and [Ni/H] =−1.400 ± 0.144. Noterdaeme et al. (2007) further analysed the absorber with UVES spectra and using 14 components derive a molecular hydrogen fraction log f=− 1.69. The Si and Fe imply a system with one-fifth solar metallicity in the gas phase and grain depletion. Depleted gas phase elements normally have a pattern with Al much more depleted than Si, so in this regard, the [Al/H] is anomalous, but the presence of dust would also explain the H2.
For the system at zabs= 2.6147, Prochaska et al. (2001) derive = 21.30 ± 0.10,
, [Si/H] =−1.968 ± 0.123, [Cr/H] =−2.296 ± 0.117, [Fe/H] =−2.227 ± 0.133, [Ni/H] =−2.357 ± 0.124 and
2.099. No molecular hydrogen is detected at this redshift down to log f < −7.2 according to Noterdaeme et al. (2007).
Lanzetta et al. (1991) also report an absorber with unknown N(H i) at zabs= 2.0470. An additional Lyman Limit System was reported at zabs= 2.9298 (Stengler-Larrea et al. 1995) with associated C iv features (Lu & Savage 1993). Finally, Khare, York & Green (1989) report Mg ii absorbers at zabs= 0.8629 and 1.0793.
Bunker et al. (1999) use long-slit K-band spectroscopic on UKIRT to try to detect the galaxies responsible for these two absorbers. They did not find any Hα emission line leading to upper limits of F(Hα) < 7.53 × 10−17 erg s−1 cm−2, i.e. SFR < 7.18 h−2 M⊙ yr−1 at zabs= 2.4263 and F(Hα) < 10.5 × 10−17 erg s−1 cm−2, i.e. SFR < 11.9 h−2 M⊙ yr−1 at zabs= 2.6147. Similarly, we do not detect any of these two absorbers in our SINFONI data at limits that are lower than those set by Bunker et al. (1999). For zabs= 2.6147, we derive F(Hα) < 1.5 × 10−17 erg s−1 cm−2, i.e. SFR < 3.8 M⊙ yr−1.
For the non-prime targets, only the zabs= 2.0470 and zabs= 2.4263 are covered by our SINFONI data but they remain undetected.
A15 Q2352−0028, zabs= 1.0318 (0.8730, 1.2468)
For the zabs= 0.8730, Rao et al. (2006) measure = 19.18
. The corresponding EW(Mg ii 2803) = 0.816 ± 0.075 Å. Meiring et al. (2009) used 10 components to fit the absorber over Δv∼ 225 km s−1 and derive
and [Fe/H] =−1.17 ± 0.09.
For the zabs= 1.2468, Rao et al. (2006) measure . The corresponding EW(Mg ii 2803) = 2.261 ± 0.120 Å. Meiring et al. (2009) used 15 components to fit the absorber over Δv∼ 410 km s−1 and derive
and [Fe/H] =−0.86 ± 0.24.
In our SINFONI observations, we do not detect the galaxy responsible for the absorber at zabs= 0.8730 and the absorber at zabs= 1.2468 is not covered by our data.
Author notes
Based on observations collected during programmes ESO 83.A-0699 and 85.A-0708 at the European Southern Observatory with SINFONI on the 8.2-m YEPUN telescope operated at the Paranal Observatory, Chile.