-
PDF
- Split View
-
Views
-
Cite
Cite
Matthew Pontefract, Janet E. Drew, Tim J. Harries, René D. Oudmaijer, Hα spectropolarimetry of the Herbig Ae star AB Aurigae, Monthly Notices of the Royal Astronomical Society, Volume 319, Issue 2, December 2000, Pages L19–L23, https://doi.org/10.1111/j.1365-8711.2000.04118.x
- Share Icon Share
Abstract
We present spectropolarimetric observations, obtained at Hα, of the Herbig Ae star AB Aurigae. Changes in linear polarization across the Hα line probe structure in the immediate circumstellar environment of the central star, down to scales of the order of one to a few stellar radii. In the case of AB Aurigae the observed polarimetric signature is complex. After applying a correction for foreground continuum polarization, we find that there is a linear-polarized Hα emission component intrinsic to the source. Rotation of the angle of polarization through the emission-line profile suggests scattering in a rotating circumstellar disc. The magnetic accretor model commonly applied to T Tauri stars shows promise of explaining these data.
1 Introduction
In this paper we report the discovery of a striking polarization phenomenon in the spectrum of the Herbig Ae star AB Aurigae. High spectral resolution spectropolarimetry spanning the P Cygni Hα feature obtained at the William Herschel Telescope has revealed a change in linear polarization that has its origin deep within the much-studied, yet still poorly understood, flows around this star. In particular we believe that we have detected intrinsic polarization of the Hα line.
Herbig Ae/Be (HAeBe) stars, identified by Herbig (1960), have long been considered to be the higher mass counterparts to the pre-main-sequence T Tauri stars. AB Aur, with mν ≃ 7.1, is the brightest in the northern hemisphere, and amongst the brightest in its class. In a number of the transitions apparent in its spectrum, blueshifted absorption is seen alongside strong redshifted emission (Catala et al. 1986), suggesting the presence of at least some outflow. Hα, the transition on which we focus here, is one of these. AB Aur has been the subject of considerable observer attention, resulting in the accumulation of a large body of spectroscopic and polarimetric data spanning numerous wavebands (Catala et al. 1999, and references therein).
One of the more striking attributions to AB Aur, an A0pe star, is the presence of what has been dubbed an extended chromosphere achieving a maximum temperature of ∼17 000 K, as compared with a photospheric temperature of 10 000 K (Catala, Kunasz & Praderie 1984; Catala & Kunasz 1987). Evidence of surprisingly ‘high excitation’ in the vicinity of this star has grown with the recent detection of P Cygni N v 1240 Å (Bouret, Catala & Simon 1997). AB Aur is also noted to be a spectroscopic and polarimetric variable on short time-scales (Beskrovnaya et al. 1995; Catala et al. 1999). Periodicities at ∼34 and ∼45 h have been picked out by Catala et al. (1999) and are tentatively linked to the rotation of the star. Beskrovnaya et al. (1995) find polarimetric variability on even shorter time-scales, with the polarization position angle changing by more than 20° in under 5 h.
By analogy with the favoured model for lower mass T Tauri stars, it may be that some form of magnetically truncated accretion disc and thereafter channelled accretion flow lie behind the complex phenomena seen in AB Aur and other Herbig stars (see the extended discussion of the issues in Hartmann 1999). A likely consequence of the channelled accretion flow, as in a range of classical T Tauri stars (Edwards et al. 1994), would be evidence of inflow in the form of inverse P Cygni line profiles. These are occasionally observed in AB Aur. Also one might expect short-term, possibly erratic, photometric variability. The idea here is that variability would arise as stellar rotation periodically conceals and reveals hotspots that are located where the magnetically channelled flow hits the stellar surface. Only in the circumstance that the magnetic poles are continuously visible would a more or less invariant light curve be likely. However, recent work by Herbst & Shevchenko (1999) suggests that photometric periodicities on the right time-scales are not apparent in HAeBe stars. In this respect, HAeBe stars would appear to be different from T Tauri stars. In the specific case of AB Aur it can be noted that the periodicities detected spectroscopically are not apparent photometrically. Indeed, the optical light output of AB Aur shows really quite modest variation (Δmv being less than ∼0.1 mag, typically: see Herbst & Shevchenko 1999). In the absence of a complete match to a T Tauri-style model, there is room to entertain quite different models for AB Aur. For example, it has been proposed that the phenomenology is powered by the dissipation of stellar interior rotational energy through shear instabilities (Vigneron et al. 1990; Lignières, Catala & Mangeney 1996).
In the midst of this uncertainty, even the inclination of AB Aur remains somewhat controversial, potentially complicating the interpretation of any observations. On the larger scale of ∼1000 au, Grady et al. (1999) see little flattening of the optical envelope in an HST coronagraphic image. On this basis, they propose an inclination relative to the plane of the sky i ≤ 45°. By contrast, Mannings & Sargent (1997) present a resolved 13CO map which shows flattening consistent with i = 76° (no error specified; the deconvolved semi-major and semi-minor axes being 450 and 110 au at a distance of 160 pc). This estimate is broadly in line with the inclination of about 70° that is favoured by Catala et al. (1999) on the basis of the probable stellar radius, distance, v sin i and rotation period of AB Aur.
So, despite the attention bestowed on AB Aur, we know little about the geometry of its immediate circumstellar environment. There may or may not be an accretion disc present, possibly with a central hole. While there is undoubtedly some outflow at speeds up to several hundred km s−1 (Catala et al. 1986; Bouret et al. 1997), there is also occasional evidence of simultaneous inflow from He i line data (Catala et al. 1999).
The value of spectropolarimetric observations, in such circumstances, is that they can provide an independent means of probing the circumstellar environment on scales of one to a few stellar radii. At a basic level, observations at Hα can allow conclusions to be drawn as to whether ionized material close to the star projects on to the plane of the sky as a circle or must show some asymmetry. In classical Be stars, for example, the Hα line is formed over the large area of an ionized circumstellar disc, while the continuum arises only from the central object. In such a circumstance, there is essentially no in situ polarization of Hα photons, while continuum photons scattered by free electrons in the disc will show a net linear polarization as long as the disc is significantly inclined with respect to the observer. The net outcome in such a case would be a change in polarization across the Hα line that enables separation of the polarization due to electron scattering from that due to interstellar extinction (which affects line and continuum equally). Data of this type played a pivotal role in showing that classical Be stars are embedded in discs (Clarke & McLean 1974; Poeckert 1975; Poeckert & Marlborough 1976).
If Hα photons are also subject to coherent scattering, more complex linear polarization signatures across the line profile are possible (Wood, Brown & Fox 1993) and have indeed been seen (e.g. BI Cru: Harries 1996; HD 87643: Oudmaijer et al. 1998). Where such effects are present, the relatively small scattering opacities involved will ensure that the polarized light component is sensitive to geometry on a much more compact spatial scale than the total intensity profile in a strong line like Hα.
The data that we present below show that AB Aur is another example of an object presenting a complex linear polarization signature across the Hα line. We draw attention to it in this short contribution in order to signal the great potential for new insights and/or tests that Hα spectropolarimetry has to offer the study of young stellar objects.
2 Observations
Optical linear spectropolarimetric data were obtained with the red arm of the ISIS spectrograph mounted at the Cassegrain focus of the William Herschel Telescope, La Palma, on the night of 1999 December 18. A spectral resolution of approximately 60 km s−1 was achieved using a 1200R grating and 1024 × 1024 pixel TEK CCD, giving spectral coverage from 6370–6760 Å. The seeing was poor, being generally worse than 3 arcsec. The instrument was equipped with a half-wave plate rotator which may be set to various angles to obtain the Stokes QU parameters, and a calcite block to separate the light into perpendicularly polarized light waves. A comb dekker allowed simultaneous observations of both the target and sky, such that each CCD frame contains four relevant spectra: the O- and E-rays of both the target and sky respectively (the comb dekker generates several sky spectra in each frame but only those adjacent to the target spectrum were used). Four such frames with the half-wave plate positioned at angles of 0°, 45°, 22°.5 and 67°.5 constitute a complete polarization observation. After each set of four frames, the sky and target dekker slots were exchanged to compensate for any asymmetry in either detector or instrument.
Several polarimetric standards were observed during the run, and the unpolarized targets reveal an intrinsic instrumental polarization of ∼0.2 per cent. Observations of polarized standards were used to calibrate the zero-point rotation angle offset. Observations of a selection of standards throughout the run show this offset to be consistent to within ±1°. Cu–Ar arc lamp spectra exposed at frequent intervals throughout the night provided wavelength calibration data. The AB Aur data set consists of 16 frames (four sets of four wave-plate rotations as described above), each exposed for 150 s through a projected slitwidth of 1.23 arcsec.
The data were subsequently processed using figaro tools. After bias subtraction and flat-fielding, the CCD rows containing the O- and E-spectra of both sky and target were identified. These, along with other imaged data, were supplied to the Ccd2pol polarimetry extraction package, part of the Times Series/Polarimetry Package (tsp), within Figaro. The Stokes parameters were determined and subsequently analysed with the Polmap package. Polmap was also used to remove an instrumental, wavelength-dependent ripple known to be present in such data [see Harries & Howarth (1996) for a full discussion].
3 Results and the correction to intrinsic polarization
Fig. 1 shows the Hα region of the spectrum, after de-rippling. Two things are immediately apparent: the continuum polarization is very low, and complex changes are seen across the Hα profile itself. To learn something from this and to establish whether the Hα emission merely dilutes the continuum polarization or is itself somewhat polarized, it is necessary to try to separate out foreground polarization contributions from those intrinsic to AB Aur.
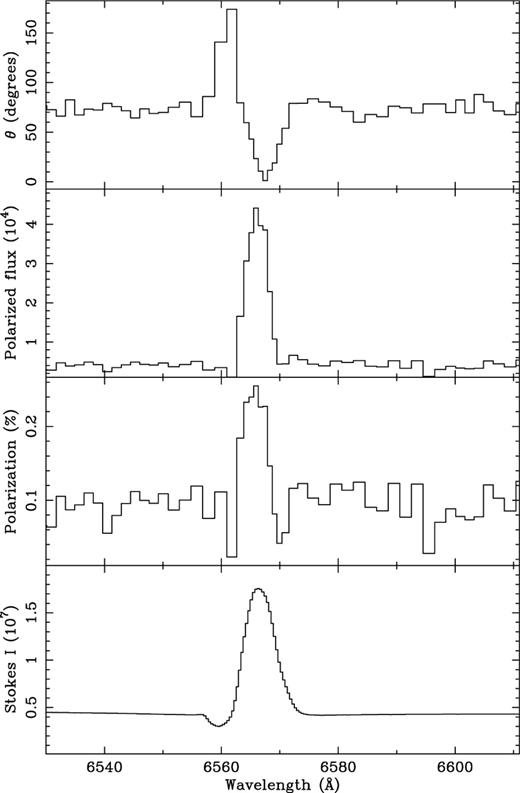
The polarization spectrum around Hα. The spectrum is rebinned to a constant error of 0.03 per cent in polarization magnitude.
The plot of these same data in QU space, shown in Fig. 2, allows us to begin unravelling what is going on. The dense knot of points centred on (−0.07, 0.05) is due to the continuum. Out of this emerges a clear loop that mostly falls in the positive U range – this is associated with the rotation and apparent increase in polarization through the redshifted emission of the Hα line. There is also a more linear excursion out of the continuum knot into the bottom right-hand quadrant that, spectrally, occurs within the Hα blueshifted absorption component. We have determined the position angle (PA) of this excursion to be 160° ± 10°. Adapting an argument from McLean (1979), it is plausible that this PA defines the intrinsic plane of continuum polarization of AB Aur. The basic idea is that the blueshifted absorption in the Hα line preferentially removes direct unscattered light from the continuum source in AB Aur, leaving an enhanced scattered (and polarized) fraction in the residual light at the same PA. By this argument, we should expect the correction for any foreground instrumental, interstellar medium or circumstellar polarization to shift the observed set of (q̄, ū) points uniformly into the bottom right quadrant of QU space such that the new PA of the knot of continuum points matches that of the excursion arising from the blueshifted absorption.
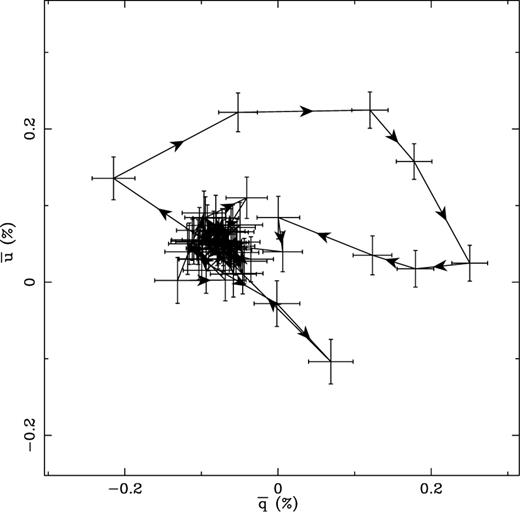
Plot of against
Two departures from the central knot of continuum points are clearly visible: the large loop in the upper portion of the plot is associated with polarization in the line emission, whilst the smaller excursion to the lower right corresponds to the PA flip in the blueshifted Hα absorption (see Fig. 1). Note that the plot axis directions
correspond to sky PAs of 0°, 45°, 90° and 135° respectively (i.e.
where θ is the sky PA).
First, is there any independent evidence that favours an intrinsic PA of 160° for AB Aur? Yes, there is. Both Marsh et al. (1995) and Mannings & Sargent (1997) have reported long-wavelength imaging data that suggest a relatively highly inclined disc at a PA of about 80°. This is encouraging in that this is close to perpendicular to the PA of 160° implied by the blueshifted absorption, and hence is consistent with what would be expected if light from the central continuum source in AB Aur scattered off a circumstellar disc at a PA of ∼80°.
Secondly, is it possible to estimate the correction that ought to be applied to our data in order to remove the effects of foreground polarization? From the observed position of the continuum knot in Fig. 2 (at θ ≃ 70°) and the expectation of an intrinsic angle of 160°, it is apparent that the dominant term to be removed is itself at θ ≃ 70° (i.e. nearly perpendicular on the sky to the intrinsic direction) and of a magnitude that is at least as great as the intrinsic component. On two occasions Vrba has argued from observations of nearby field stars that the net interstellar plus circumstellar polarization direction is at a PA of ∼70° [Vrba (1975) specifies 68°; Hillenbrand et al. (1992) modify this to 76°]. This is in perfect agreement with our expectations. Furthermore, the data in Hillenbrand et al. (1992) suggest that the magnitude of this component is quite high, at 1.3 per cent. This is not out of line with the colour excess of AB Aur: the standard relation (e.g. Spitzer 1978) between E(B − V) (0.14 for AB Aur: Herbst & Shevchenko 1999) and linear polarization percentage implies a maximum of about 1.3 per cent. Whatever the foreground polarization is, it can be assumed not to be significantly time-variable since the optical light variations of AB Aur are very modest [Herbst & Shevchenko (1999) found the typical V magnitude variation to be only ±0.1 mag]. Significantly, a recent polarimetric observation of AB Aur (Ashok et al. 1999) revealed a continuum polarization of 0.8 per cent at θ = 70°, when the source appeared, spectroscopically, to be in a minimum-activity state. If we suppose the intrinsic polarization to have vanished in this minimum state, we could interpret the observed polarization at this time as being all due to foreground. More correctly, this observation of 0.8 per cent polarization is a lower bound on the magnitude of the foreground term, since there may have been residual, approximately orthogonal, intrinsic polarization at the time.
Fig. 3 shows how our spectropolarimetric data are transformed if it is assumed that a foreground polarization of 0.8 per cent at a PA of 70° has to be removed. This includes no correction for instrumental polarization. This is not a problem since it is, by comparison, a small correction at about the 0.1 per cent level. It is more important to note that the true correction could be bigger than the one that we have applied, such that the intrinsic continuum polarization might be as high as ∼1.3 per cent, rather than ∼0.8 per cent as shown. The simple shift in QU space that we have applied does not, of course, modify the progressive rotation seen in the redshifted Hα emission (i.e. the ‘loop’ so obvious in Fig. 2 is unchanged by the translation), but it does reduce the amplitude of the angle variation, since now the (corrected) continuum polarization is much larger.
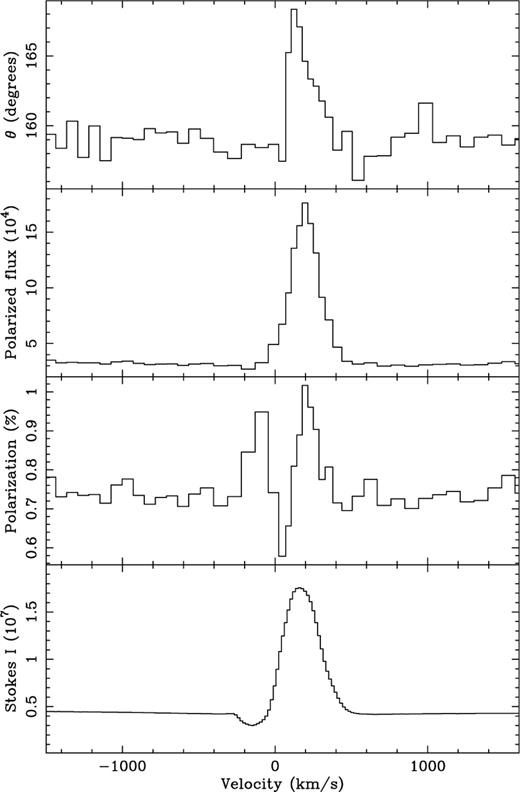
The polarization spectrum after removing our estimate of the minimum polarization owing to foreground interstellar and circumstellar sources. It is argued in Section 3 that this component is likely to be at least 0.8 per cent at a PA of ∼70°. Also, for reasons outlined in Section 3, the correction is required to ‘restore’ an intrinsic PA of ∼160°.
4 Discussion
The most striking feature of the linear polarization changes across Hα, intrinsic to AB Aur (Fig. 3), is that the Hα emission is polarized: the polarized flux spectrum reveals a very clear, somewhat narrowed emission feature compared with the intensity spectrum. This, in itself, is a result outside the ordinary. As described in the Introduction, the usual expectation is that Hα photons arise mainly from a volume that is comparable to or larger than that occupied by the scatterers imposing polarization on the continuum. In AB Aur we most likely have the converse: the Hα emission is mainly produced in a region interior to the scatterers. This conclusion is extremely robust against alternative corrections for foreground polarization. For example, if it were assumed instead that the peak of the Hα emission were close to totally unpolarized intrinsically, as is often assumed, the corresponding foreground correction would only succeed in cancelling the very centre of the emission line in the polarized flux spectrum.
A further attribute of the polarization changes across Hα is the obvious loop in the QU plane (Fig. 2). The PA rotation that this implies has long been recognized as evidence of the breaking of left–right symmetry in the velocity field of the scattering region (see McLean 1979). In many astrophysical systems, including AB Aur, it is reasonable to associate this phenomenon with a rotating circum-object disc. The presence of this QU loop and associated PA rotation stands in interesting contrast to the much more linear distribution of QU points and relative PA constancy seen in the case of the B[e] star HD 87643 (Oudmaijer et al. 1998): in terms of the wavelength dependence of the percentage polarization and the character of the Hα intensity profile, the two cases would seem to be rather similar; yet, in AB Aur, a circumstellar rotating disc is presumably a more prominent component, as revealed by the PA rotation.
The magnetic accretor model commonly applied to T Tauri stars shows some promise of explaining the Hα polarization properties that we have discovered. This model would allow for a significant production of Hα photons in the shocked accretion flow, close to the stellar surface, which could then be scattered in and just beyond the inner edge of the disrupted accretion disc. The continuum polarization would be created in essentially the same way except, of course, that the continuum source is the whole star. A potentially interesting context within this model for our spectropolarimetric observation is provided by the appearance of the He i 6678-Å line which happens to fall within the spectral range of the data obtained. This line profile is shown as Fig. 4. Redshifted absorption is clearly present. Catala et al. (1999) have shown that a similar signature is seen only in the stronger He i 5876-Å line for quite limited intervals. They suggest that this infall signature reappears on a period of ∼43 h which might be the rotation period of the star. A question for the future is whether the Hα spectropolarimetric signature might also vary on this period – it should, if the magnetic accretor model is appropriate. The polarimetric data of Beskrovnaya et al. (1995) have already revealed changes on a time-scale of hours.
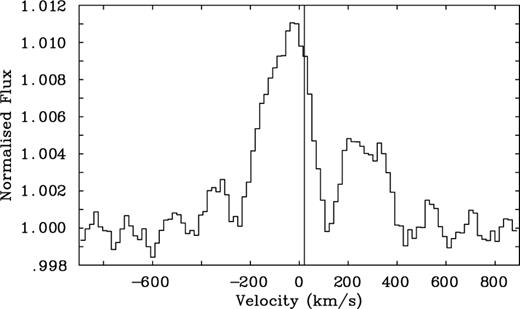
The He i 6678.15-Å line. The vertical bar lies at the systemic velocity of 21 km s−1. Note the redshifted absorption.
To arrive at quantitative constraints on AB Aur, simulation of the spectropolarimetric behaviour of this star is required. We are just beginning to do this, using the Monte Carlo technique presented in Harries (2000). If the magnetic accretor model is the right one, we can ask how big the central hole in the accretion disc is likely to be and ask, in the spirit of the semi-analytic work of Wood et al. (1993), whether the pattern and magnitude of PA rotation across Hα fit in with the expectations of this model. A further intriguing issue to resolve is whether polarization owing to electron scattering fits with the existing observation. The findings of Wood & Brown (1994), based on calculations incorporating electron thermal broadening, urge caution in this respect. Observationally, it would be interesting to discover whether classical T Tauri stars, objects which are widely believed to be magnetic accretors, show spectropolarimetric signatures similar to AB Aur. We already have data on further HAeBe stars, and will know soon from this larger sample if AB Aur is typical or exceptional.
In short, these Hα spectropolarimetric data on AB Aur open up a new line of attack on the problem of characterizing the near-stellar environments of this and other young stellar objects.
Acknowledgments
The William Herschel Telescope is operated on the island of La Palma by the Isaac Newton Group in the Spanish Observatorio del Roque de los Muchachos of the Instituto de Astrofísica de Canarias.
References