-
PDF
- Split View
-
Views
-
Cite
Cite
Susan M Linder, Mandy Miller Koop, Sarah Ozinga, Zachary Goldfarb, Jay L Alberts, A Mobile Device Dual-Task Paradigm for the Assessment of mTBI, Military Medicine, Volume 184, Issue Supplement_1, March-April 2019, Pages 174–180, https://doi.org/10.1093/milmed/usy334
- Share Icon Share
Abstract
Dual-task performance, in which individuals complete two or more activities simultaneously, is impaired following mild traumatic brain injury. The aim of this project was to develop a dual-task paradigm that may be conducive to military utilization in evaluating cognitive-motor function in a standardized and scalable manner by leveraging mobile device technology.
Fifty healthy young adult civilians (18–24 years) completed four balance stances and a number discrimination task under single- and dual-task conditions. Postural stability was quantified using data gathered from iPad’s native accelerometer and gyroscope. Cognitive task difficulty was manipulated by presenting stimuli at 30, 60, or 90 per minute. Performance of cognitive and balance tasks was compared between single- and dual-task trials.
Cognitive performance from single- to dual-task paradigms showed no significant main effect of balance condition or the interaction of condition by frequency. From single- to dual-task conditions, a significant difference in postural control was revealed in only one stance: tandem with eyes closed, in which a slight improvement in postural stability was observed under dual-task conditions.
The optimal dual-task paradigm to evaluate cognitive-motor performance with minimal floor and ceiling effects consists of tandem stance with eyes closed while stimuli are presented at a rate of one per second.
INTRODUCTION
Mild traumatic brain injury (mTBI) results in multi-system impairments, including declines in postural stability, information processing, vestibular function, and cognition.1,2 Traditional clinical approaches to mTBI assessment include balance and neurocognitive function, each examined as a single-task in an isolated manner, but rarely evaluate the interaction of performing two or more of these tasks concurrently in a dual- or multi-task paradigm. Dual-task activities in which cognitive and motor tasks occur simultaneously are more representative of real-world tasks performed by military service members.3–5 Service members in the battlefield are required to continually monitor and process auditory information presented in real-time and to react quickly and accurately in threatening conditions. The inability to effectively measure and therefore detect potential impairments in dual-task conditions following mTBI may lead to the premature clearance for full, unrestricted return to duty that may compromise not only the individual soldier’s safety, but also the safety of their fellow soldiers.
It is readily acknowledged that the assessment of dual-task function is an important aspect of mTBI management in both military and civilian populations.3,6–11 However, a gap in the clinical management of mTBI remains, as the development and implementation of a dual-task paradigm that is valid, sensitive, scalable, and objective, while being ecologically sound in measuring cognitive and motor function has evaded field experts.9 Undertaking a dual-task activity that requires active information processing and a motor response typically results in dual-task costs, in which a decline in performance is experienced in one or both task(s) compared to performance if each task is completed separately.12,13 In the assessment of dual-task function, it is critical to utilize tools of sufficient sensitivity to detect dual-task costs in both cognitive function and postural stability that may go undetected using traditional clinical methods. The current study leverages our previous work by combining a portable, biomechanical approach to measuring postural stability with a new measure of taxing cognitive function in which attentional demands are incrementally challenged.14,15
Clinical measures of postural stability have been shown to lack sensitivity in detecting the subtle, yet important, differences in motor control as a result of mTBI.16,17 Recently, this gap in assessment has been addressed by using data gathered from the accelerometer and gyroscope within mobile devices.15,18–21 The accelerometer and gyroscope data are then processed to provide an objective measure of postural sway that is not subject to ceiling and floor effects present in the subjective scoring of the Balance Error Scoring System.15,18 Utilizing the inertial sensors within the iPad to provide a biomechanical measure of postural stability results in a portable, scalable and affordable approach to assessment of motor control that is conducive for use in the clinical setting, battlefield or athletic field.22
With respect to the measurement of neurocognitive function, various tests have utilized dual-task constructs to simulate real-life scenarios and improve the detection of residual deficits in cognitive and/or motor function following mTBI, measured as dual-task costs.11,23–25 Catena and colleagues reported that adding an attentional task (question and answer) to gait assessment elicited significant declines in gait stability between a cohort of concussed individuals compared to healthy controls, undetected in the single task (gait only) construct.26 Similar findings were reported by Dorman and colleagues, in which a concomitant cognitive task (reciting months of the year backwards) enhanced the detection of postural instability in athletes with concussion compared to postural stability testing in isolation.10 While these and other dual-task paradigms3,4 have enhanced the detection of residual postural instability or gait deficits in sport concussion, none include a cognitive task suitable for military use that requires the active monitoring and processing of information in order to make a correct response.
Two assessment batteries have been developed for use in return-to-duty decision-making: The Assessment of Military Multitasking Performance (AMMP) and the Military Functional Assessment Program (MFAP).27 The AAMP employs five functional multi-tasks and three dual-tasks designed to simulate functional tasks relevant to military service members and to inform return-to-duty decision-making following mTBI.9 Reliability and feasibility testing of the original version of the AMMP resulted in the modification and reduction of tasks to a final iteration of three dual- and three multi-tasks.7 The final six tasks employ complex, functional, military-specific multi-tasking endeavors, each scored using a unique scoring system by trained test administrators. The modified AMMP takes approximately 1 hour and 45 min to complete.7 The MFAP is administered over 5–6 days and involves a complex and comprehensive series of military tasks including administering basic life support, egressing from a simulated vehicle rollover, tactical simulation training, and land navigation skills, among others.18 Portions of the MFAP are administered using simulated scenarios while others employ a virtual reality environment.6 Collectively, the MFAP and AMMP are excellent examples of assessments that utilize military-specific tasks. However, their limitations include lengthy test duration, specialized academic training requirement, and need for costly equipment. These factors limit the potential to implement a standardized and scalable approach to evaluating dual-task performance in military personnel.
In contrast to the AMMP and MFAP, the aim of our study was to develop a portable and automated scoring approach to dual-task evaluation. We sought to combine biomechanics to quantify postural stability and an information processing task that resembles, but does not explicitly replicate, the type of audio processing tasks that are often present in military operations. The postural stability and cognitive assessments were developed using the iPad platform, exploiting its native inertial measurement units (IMUs) and computing capacity, thus minimizing the need for cumbersome set up, extensive external hardware, or specially trained personnel for scoring. Our approach was to employ motor and cognitive tasks that could be incrementally adjusted in difficulty to determine the optimal combination to provide discriminatory value with respect to the identification of dual-task cognitive or motor costs. We hypothesized that the intermediate level of difficulty for the cognitive task would result in the greatest dual-task losses when comparing single- to dual-task performance across all four balance stances.
METHODS
Participants
Fifty healthy, physically active young adults, 18–24 years of age with no active neurologic or musculoskeletal condition resulting in impaired postural stability participated in the study. Institutional Review Board approval was obtained from The Cleveland Clinic. The United States Army Medical Research and Material Command’s Human Research Protection Office also approved the study, and informed consent was obtained from all participants. All testing occurred in the Neural Control Lab at the Cleveland Clinic and participants were compensated $25. Participant demographics are detailed in Table I.
Gender | |
Male | 25 (50%) |
Female | 25 (50%) |
Age (years) | |
Mean SD | 20.2 ± 1.5 |
Median (quartiles) | 20 (19, 21) |
Athletic exposure | |
Competitive | 10 (20%) |
Recreational | 31 (62%) |
None | 9 (18%) |
Dominant foot | |
Right | 44 (88%) |
Left | 6 (12%) |
Gender | |
Male | 25 (50%) |
Female | 25 (50%) |
Age (years) | |
Mean SD | 20.2 ± 1.5 |
Median (quartiles) | 20 (19, 21) |
Athletic exposure | |
Competitive | 10 (20%) |
Recreational | 31 (62%) |
None | 9 (18%) |
Dominant foot | |
Right | 44 (88%) |
Left | 6 (12%) |
Gender | |
Male | 25 (50%) |
Female | 25 (50%) |
Age (years) | |
Mean SD | 20.2 ± 1.5 |
Median (quartiles) | 20 (19, 21) |
Athletic exposure | |
Competitive | 10 (20%) |
Recreational | 31 (62%) |
None | 9 (18%) |
Dominant foot | |
Right | 44 (88%) |
Left | 6 (12%) |
Gender | |
Male | 25 (50%) |
Female | 25 (50%) |
Age (years) | |
Mean SD | 20.2 ± 1.5 |
Median (quartiles) | 20 (19, 21) |
Athletic exposure | |
Competitive | 10 (20%) |
Recreational | 31 (62%) |
None | 9 (18%) |
Dominant foot | |
Right | 44 (88%) |
Left | 6 (12%) |
Data Collection
Cognitive and balance modules were custom developed within the Cleveland Clinic Neural Control Laboratory using the native iPhone operating system (iOS) software in Objective C as part of a battery of Cleveland Clinic research mobile applications.22 The testing protocol was administered as follows: (1) single-task postural stability, (2) single-task cognitive, and (3) dual-task (postural stability and cognitive). A depiction of the experimental setup is provided in Figure 1. All raw cognitive and balance data were stored locally on the iPad in JavaScript Object Notation, extracted following data collection, and converted to comma-separated values for off-line analysis using custom MATLAB programs. Participant demographics were collected using REDCap electronic data capture tools hosted at the Cleveland Clinic.28
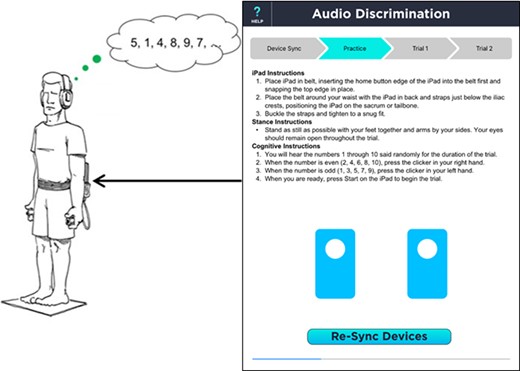
Depiction of dual-task experimental setup with iPad affixed to participant’s sacrum, collecting biomechanical data with its native IMU sensors while the cognitive number discrimination task is administered.
Postural Stability Assessment
A custom iPad application previously developed and validated in our lab15,18,29 was used to provide a biomechanical assessment of postural stability. Development, methodology, and validation of the inertial sensors native to the iPad have been outlined in detail in our previous publications.18,20 Briefly, the iPad was affixed at the level of the iliac crests to the individual’s sacrum as an approximation of center of mass (COM) using a custom-made belt. Data from the iPad’s accelerometer and gyroscope (sampled at 100 Hz) measuring linear acceleration and angular velocity, respectively, were used to compute postural stability metrics including 95% ellipsoid volumes and a normalized measure termed the Cleveland Clinic-Postural Stability Index (CC-PSI).15,18–21,29 The CC-PSI uses the normalized path length of acceleration converted into standardized z-scores to represent postural sway for each individual as a percentile relative to the normative population.21,29 The CC-PSI was computed under single-task balance and dual-task cognitive-balance conditions for 60-second trials. For single-task balance assessment, participants completed two trials of each of the following stances, the order of which was randomized across participants: (1) double limb, eyes open; (2) double limb, eyes closed; (3) tandem, eyes open; and (4) tandem, eyes closed. For balance assessment under dual-task conditions, participants completed one 60-second trial of each of the four stances identified above under three levels of cognitive load, for a total of 12 trials. During all trials (single- and dual-task), participants wore socks, positioned their arms relaxed at their sides, and were instructed to maintain a steady posture for the duration of the 60-second trial.
Cognitive Assessment
A number discrimination task was developed in which a series of random stimuli between 1 and 10 were presented to participants via headphones over a 60-second trial. Participants held a response input device in each hand and were instructed to press the button held in the right hand when an even number was presented and activate the left button when an odd number was presented. The right and left response input devices were connected to a microcontroller (Arduino Pro Mini 3.3 v), which sent a signal to the iPad when the button on the device was pressed via a direct current output to the microphone input of the iPad device. Each device provided a specific pulse-width modulation output which allowed the identification of which button was depressed. Sampling rate for the response input devices was 100 Hz and synchronized with collection of IMU data.
All participants completed a 30-second practice trial of the number discrimination task prior to the formal assessment to ensure comprehension of task requirements. Task difficulty was manipulated by presenting stimuli at three rates: 30, 60, or 90 stimuli per minute. Participants were instructed to respond as quickly and accurately as possible to the presented stimuli. For single task cognitive assessment, participants performed two 60-second trials, while seated in a chair, at each of the three stimulus presentation rates. A paired randomization scheme was used when administering single-task cognitive testing across participants (i.e., Task difficulty was randomized, however both trials of the same stimulus presentation rate were completed consecutively). During assessment of cognitive function under dual-task conditions, participants completed one 60-second trial of each difficulty level (30, 60, or 90 stimuli per minute) simultaneously under the four postural stability stances outlined above. A total of 12 dual-task trials were completed (three levels of cognitive difficulty for each of the four postural stability stances). Participants were instructed to maintain a steady posture while responding as quickly and accurately as possible to the number discrimination task. Cognitive performance under single- and dual-task conditions was measured by response accuracy.
Statistical Analysis
To assess cognitive dual-task loss, a robust two-way ANOVA with white correction due to non-Gaussian distribution (p > 0.05, Kolmogorov–Smirnov test (R Core Team 2015), was utilized to determine if balance condition (five levels: single task, double-leg eyes open and closed, tandem stance eyes open and closed) or frequency of stimulus presentation (three levels: 90/min, 60/min, and 30/min), and/or their interaction had a significant effect on cognitive performance (outcome measure: percent correct in number recognition). When necessary, post-hoc linear mixed effect models30 were used to determine significant difference within each main effect (balance condition and frequency). To determine balance dual-task loss within each stance condition, a Friedman’s Test (R Core Team, 2015) due to a non-Gaussian distribution (p > 0.05, Kolmogorov–Smirnov test (R Core Team 2015)), was utilized to determine the effect of frequency within each stance condition: double limb, eyes open; double limb, eyes closed; tandem, eyes open; and tandem, eyes closed. For each stance, a model with CC-PSI as the outcome variable and one factor, frequency (four levels: single task (ST) 30, 60, or 90 stimuli per minute) was utilized. To control for Type I errors across all four balance conditions, a Holm–Bonferroni correction method was applied to all the p values. The target alpha level was set at 0.05 for all analyses.
RESULTS
In terms of cognitive performance, the accuracy rate during the single task assessment decreased as a function of frequency of stimulus presentation with median values of 97%, 87%, and 43% accuracy for the 30, 60, and 90 stimuli per minute rates, respectively. This finding held true for all conditions as cognitive performance decreased significantly across all single and dual-task conditions as a function of frequency (F = 849 DF = 2, p < 0.001), with median values of 93.5%, 83.2%, and 46.4% for the 30, 60, and 90 stimuli per minute rates, respectively (p < 0.05 for all comparisons) (Fig. 2). Cognitive performance between single- to dual-task paradigms showed no significant main effect of balance condition (F = 0.6003, DF = 4, p = 0.66) or the interaction of condition × frequency (F = 0.4245, DF = 8, p = 0.91). Median and interquartile ranges for performance at all three levels under single- and dual-task conditions are presented in Figure 2 and Table II.
Conditions . | Median (First & Third Quartile) Percent Correct . | |||||
---|---|---|---|---|---|---|
30/min . | 60/min . | 90/min . | ||||
Single task | 96.7 | [90,97] | 86.7 | [77,92] | 43.3 | [36,53] |
Double-leg stance, eyes open | 100.0 | [97,100] | 89.2 | [82,95] | 43.9 | [33,56] |
Double-leg stance, eyes closed | 96.6 | [97,100] | 91.7 | [79,95] | 44.4 | [38,55] |
Tandem stance, eyes open | 100.0 | [97,100] | 86.7 | [77,93] | 42.8 | [38,56] |
Tandem stance, eyes closed | 96.6 | [93,100] | 85.0 | [78,930] | 46.7 | [40,58] |
Conditions . | Median (First & Third Quartile) Percent Correct . | |||||
---|---|---|---|---|---|---|
30/min . | 60/min . | 90/min . | ||||
Single task | 96.7 | [90,97] | 86.7 | [77,92] | 43.3 | [36,53] |
Double-leg stance, eyes open | 100.0 | [97,100] | 89.2 | [82,95] | 43.9 | [33,56] |
Double-leg stance, eyes closed | 96.6 | [97,100] | 91.7 | [79,95] | 44.4 | [38,55] |
Tandem stance, eyes open | 100.0 | [97,100] | 86.7 | [77,93] | 42.8 | [38,56] |
Tandem stance, eyes closed | 96.6 | [93,100] | 85.0 | [78,930] | 46.7 | [40,58] |
Conditions . | Median (First & Third Quartile) Percent Correct . | |||||
---|---|---|---|---|---|---|
30/min . | 60/min . | 90/min . | ||||
Single task | 96.7 | [90,97] | 86.7 | [77,92] | 43.3 | [36,53] |
Double-leg stance, eyes open | 100.0 | [97,100] | 89.2 | [82,95] | 43.9 | [33,56] |
Double-leg stance, eyes closed | 96.6 | [97,100] | 91.7 | [79,95] | 44.4 | [38,55] |
Tandem stance, eyes open | 100.0 | [97,100] | 86.7 | [77,93] | 42.8 | [38,56] |
Tandem stance, eyes closed | 96.6 | [93,100] | 85.0 | [78,930] | 46.7 | [40,58] |
Conditions . | Median (First & Third Quartile) Percent Correct . | |||||
---|---|---|---|---|---|---|
30/min . | 60/min . | 90/min . | ||||
Single task | 96.7 | [90,97] | 86.7 | [77,92] | 43.3 | [36,53] |
Double-leg stance, eyes open | 100.0 | [97,100] | 89.2 | [82,95] | 43.9 | [33,56] |
Double-leg stance, eyes closed | 96.6 | [97,100] | 91.7 | [79,95] | 44.4 | [38,55] |
Tandem stance, eyes open | 100.0 | [97,100] | 86.7 | [77,93] | 42.8 | [38,56] |
Tandem stance, eyes closed | 96.6 | [93,100] | 85.0 | [78,930] | 46.7 | [40,58] |
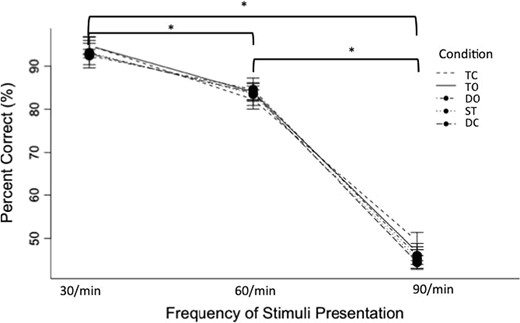
Cognitive dual-task losses were not detected within each frequency condition between single and dual-task conditions. However across all single and dual-tasks conditions, cognitive performances during the most difficult cognitive task were significantly worse compared to the intermediate and easiest levels (p < 0.05 for all comparisons, corrected), and performance was significantly worse in the intermediate difficulty level compared to the easiest (p < 0.05 for all comparisons, corrected). TC: Tandem stance with eyes closed; TO: tandem stance with eyes open; DO: double limb stance with eyes open; DC: double limb stance with eyes closed; ST: single task.
The CC-PSI values from single- to dual-task paradigms were analyzed using a Friedman Chi-squared model, which, after a Holm–Bonferroni adjustment for multiple comparisons, revealed significant differences in only one of the four stances: tandem with eyes closed (p = 0.02), in which an improvement in postural stability was observed under dual-task conditions. Post-hoc analysis was conducted to determine which level(s) of difficulty for the cognitive task resulted in significant differences in postural stability. Following a Holm–Bonferroni adjustment for multiple comparisons, only the intermediate level (60 stimuli/minute) resulted in significant changes in the CC-PSI (p < 0.05). Median values and interquartile ranges of the difference in CC-PSI score between single task and each dual-task condition are presented in Table III.
Median Values and Interquartile Ranges for CC-PSI Dual-Task Differences Between Single Task (ST) and Dual-Task Conditions at Each Cognitive Difficulty Level (30/min, 60/min, 90/min) Across All Stance Conditions
Conditions . | CC-PSI Dual-Task Differences . | Friedman’s p-Value . | |||||
---|---|---|---|---|---|---|---|
30/min – Single Task . | 60/min – Single Task . | 90/min – Single Task . | |||||
Double-leg stance, eyes open | −3.8 | [−21.1,12.4] | −3.4 | [−13.0,15.1] | 7.5 | [−17.4,21.8] | 0.37 |
Double-leg stance, eyes closed | 0 | [−17.9,13.7] | 2.4 | [−6.8,20.2] | 4.5 | [−5.2,19.2] | 0.11 |
Tandem stance, eyes open | 2.5 | [−11.7,16.9] | 2.9 | [−10.7,22.4] | 6.4 | [−17.8,21.8] | 0.17 |
Tandem stance, eyes closed | 1.8 | [−8.5,15.2] | 10.9 | [−1.1,26.8]* | 7.0 | [−3.2,33.6] | 0.02 |
Conditions . | CC-PSI Dual-Task Differences . | Friedman’s p-Value . | |||||
---|---|---|---|---|---|---|---|
30/min – Single Task . | 60/min – Single Task . | 90/min – Single Task . | |||||
Double-leg stance, eyes open | −3.8 | [−21.1,12.4] | −3.4 | [−13.0,15.1] | 7.5 | [−17.4,21.8] | 0.37 |
Double-leg stance, eyes closed | 0 | [−17.9,13.7] | 2.4 | [−6.8,20.2] | 4.5 | [−5.2,19.2] | 0.11 |
Tandem stance, eyes open | 2.5 | [−11.7,16.9] | 2.9 | [−10.7,22.4] | 6.4 | [−17.8,21.8] | 0.17 |
Tandem stance, eyes closed | 1.8 | [−8.5,15.2] | 10.9 | [−1.1,26.8]* | 7.0 | [−3.2,33.6] | 0.02 |
The intermediate level (60/min) Tandem stance- eyes closed (TC) was the only dual-task condition in which the CC-PSI was significantly improved compared to the ST condition (p = 0.02, corrected).
Median Values and Interquartile Ranges for CC-PSI Dual-Task Differences Between Single Task (ST) and Dual-Task Conditions at Each Cognitive Difficulty Level (30/min, 60/min, 90/min) Across All Stance Conditions
Conditions . | CC-PSI Dual-Task Differences . | Friedman’s p-Value . | |||||
---|---|---|---|---|---|---|---|
30/min – Single Task . | 60/min – Single Task . | 90/min – Single Task . | |||||
Double-leg stance, eyes open | −3.8 | [−21.1,12.4] | −3.4 | [−13.0,15.1] | 7.5 | [−17.4,21.8] | 0.37 |
Double-leg stance, eyes closed | 0 | [−17.9,13.7] | 2.4 | [−6.8,20.2] | 4.5 | [−5.2,19.2] | 0.11 |
Tandem stance, eyes open | 2.5 | [−11.7,16.9] | 2.9 | [−10.7,22.4] | 6.4 | [−17.8,21.8] | 0.17 |
Tandem stance, eyes closed | 1.8 | [−8.5,15.2] | 10.9 | [−1.1,26.8]* | 7.0 | [−3.2,33.6] | 0.02 |
Conditions . | CC-PSI Dual-Task Differences . | Friedman’s p-Value . | |||||
---|---|---|---|---|---|---|---|
30/min – Single Task . | 60/min – Single Task . | 90/min – Single Task . | |||||
Double-leg stance, eyes open | −3.8 | [−21.1,12.4] | −3.4 | [−13.0,15.1] | 7.5 | [−17.4,21.8] | 0.37 |
Double-leg stance, eyes closed | 0 | [−17.9,13.7] | 2.4 | [−6.8,20.2] | 4.5 | [−5.2,19.2] | 0.11 |
Tandem stance, eyes open | 2.5 | [−11.7,16.9] | 2.9 | [−10.7,22.4] | 6.4 | [−17.8,21.8] | 0.17 |
Tandem stance, eyes closed | 1.8 | [−8.5,15.2] | 10.9 | [−1.1,26.8]* | 7.0 | [−3.2,33.6] | 0.02 |
The intermediate level (60/min) Tandem stance- eyes closed (TC) was the only dual-task condition in which the CC-PSI was significantly improved compared to the ST condition (p = 0.02, corrected).
DISCUSSION
The importance of using multi-dimensional assessments to characterize dual-task performance has been recommended, yet none have been widely adopted.6,9,31,32 Numerous challenges exist in the development of a dual-task paradigm that has a level of ecological validity, practical for widespread clinical utilization while simultaneously providing an objective and quantitative assessment of cognitive and motor performance. Two assessment batteries have been proposed as tools that could facilitate return-to-duty decision-making: MFAP27 and AMMP.7 Both test batteries consist of military-relevant activities that require dual-tasking, however, both have limitations related to administration (e.g., time consuming, expensive and trained personnel) and scoring (primarily subjective and expert opinion) which limit their scalability and adoption. The MFAP is an excellent compilation of military-specific tasks that are commonly performed.27 However, there are fundamental problems that prevent widespread utilization and standardization. Namely, the time required to complete the MFAP was between five to six days and the virtual reality tools are cost prohibitive and are not widely available. The AMMP, initially developed in 20139 and refined over the next several years7, employs complex functional tasks that tax multiple domains of cognitive, sensory, and physical function. Our aim was not to replicate, replace, or mimic the MFAP or AMMP. In fact, the MFAP, AMMP and the developed dual-task paradigm described in this manuscript serve unique functions in the potential management of service members with mTBI, and should be considered complementary in clinical use. The newly developed system could be used prior to completing the MFAP or AMMP and potentially as a way to identify specific areas of function or performance that are deficient, hence guiding therapeutic approaches.
The dual-task paradigm developed in this project is unique as it provides a biomechanical quantification of postural stability and cognitive performance simultaneously and automatically. The motor and cognitive tasks employed in this paradigm are not specific military activities performed routinely by service members. However, they represent motor and cognitive processes that are used in the performance of many military activities. Maintaining a steady posture is critical in target engagement. The cognitive task employed was developed to replicate the type of information processing and response demands that the modern soldier must be proficient in, to accommodate real-time information they are provided in battle and training to replicate the ever-changing battlefield scenarios. The validation process allowed for multiple levels of difficulty to be investigated for both the motor and cognitive tasks, in our efforts to produce a tool with optimal sensitivity to demonstrate changes in performance from single- to dual-task testing. Advancements in the technological specifications to mobile devices such as the iPad have enabled scientists to explore their use in unique applications. The quality of the IMU’s embedded in the iPad, combined with its computational capabilities, has allowed us to transform the mobile device from an expensive electronic notebook to a tool conducive for biomechanical data collection. As such, the dual-task paradigm developed on the iPad provides a portable option to assess postural stability using a biomechanical approach in addition to cognitive function.
Our number discrimination task administered as a single task revealed floor effects at the most difficult level, with participants performing at a median accuracy rate of 43%, and ceiling effects at the easiest level, with participants performing at a median of 97% accuracy. In fact, at the most difficult stimulus presentation interval of 90 stimuli per minute, participants’ performance was no better than had they theoretically responded with the same hand each time. A floor effect as such represents a psychometric weakness in the test and confounds clinical interpretation upon re-testing or in using the single task version in any comparative manner (ie: to subsequently compare dual-task performance to single-task performance). Significantly worse than 43% performance during the dual-task test would have been necessary to demonstrate dual-task costs in the cognitive domain, yet responding without effort toward a correct response is not likely to yield a worse score than participants achieved while providing effort. Thus, the 90 stimuli per minute rate was not a viable level in this construct. In contrast, the easiest presentation rate yielded a response accuracy rate close to 100%, where changes in performance from single- to dual-task once again could not be discerned. This high level of performance also reveals a psychometric weakness, as changes in performance could not be captured when baseline performance was near perfect.
Neuroscientists have described a task prioritization concept, in which under dual-task conditions, individuals subconsciously choose to focus on one domain (cognitive or motor) versus the other.12,13,33,34 Dual-task losses are therefore observed in the domain subconsciously relegated as the lesser of the two priorities. In cognitive-motor dual-task paradigms, the prioritization of the postural stability task over the cognitive task is referred to as a “posture-first” strategy, while the opposite is called a “posture-second” strategy. For example, it has been reported that in a cognitive-balance dual-task paradigm, younger adults prioritize the cognitive task and experience increased postural instability while older adults employ a “posture-first” strategy and experience decrement in the cognitive/attentional task.13,35,36 However, which strategy is employed is dependent upon numerous factors including the exact construct of the dual-task paradigm, the degree of difficulty, format of instructions, and age, to name a few.12,13,34 These inconsistencies regarding task prioritization emphasize the need to utilize tasks that do not exhibit floor or ceiling effects, and are thus able to detect dual-task costs in both the cognitive and motor domain, indicative of “posture-first” or “posture-second” strategies.
Interestingly, in the developed dual-task paradigm, our cohort of healthy young adults actually demonstrated improved postural stability under dual-task conditions (during tandem stance, eyes closed), without degradation of cognitive performance. These findings are not unique, as Jehu and colleagues reported improved postural stability when healthy young adults were instructed to focus attention on a reaction time task during a dual-task paradigm employing reaction time and postural stability.36 While our participants were not explicitly instructed to focus on one task over the other, it is plausible that the demand of the number discrimination task elicited concomitant improvement in postural stabilization. Yu and colleagues described this as a “supraposture-focus strategy,” in which a reduction in postural sway is achieved when automatic postural control strategies are adopted by healthy young adults under dual-task conditions.13
Based on the changes in postural stability and cognitive performance under dual-task conditions compared to single task efforts, the optimal dual-task paradigm consists of a stimuli presentation rate of 60 stimuli per minute administered with participants standing in tandem stance with eyes closed. Normative service member and civilian data are currently being generated for this condition and can be used to augment return-to-duty decision-making through the objective quantification of cognitive-motor performance following mTBI. The utilization of the iPad and technically simple response devices makes this paradigm ideally suited for widespread clinical utilization. The reliance on objective measures of postural stability and cognitive performance has the potential to improve clinical care of those not suitable to return-to-duty as those data can be used to guide and direct behavioral or physical interventions.
Previous Presentations
Poster presentation at the 2017 Military Health System Research Symposium, August, 2017. Poster MSRS-17–1631 titled “Development of dual-task paradigm for the evaluation of mTBI in service members.” Presenting author: Jay L Alberts.
Funding
This study was supported by the U.S. Army Medical Research and Material Command: W81XWH-14-1-0532. This supplement was sponsored by the Office of the Secretary of Defense for Health Affairs.
References
Author notes
Drs Alberts, Linder, Koop, and Ozinga have filed intellectual property related to the Cleveland Clinic-Postural Stability Index balance metric.