-
PDF
- Split View
-
Views
-
Cite
Cite
Huaying Du, Rong Yong, Jiaqi Zhang, Guang Cai, Ruqin Wang, Jianbo Li, Yuji Wang, Hongsheng Zhang, Xiuying Gao, Ji Huang, OsBAK2/OsSERK2 expression is repressed by OsBZR1 to modulate brassinosteroid response and grain length in rice, Journal of Experimental Botany, Volume 74, Issue 17, 13 September 2023, Pages 4978–4993, https://doi.org/10.1093/jxb/erad196
- Share Icon Share
Abstract
Brassinosteroids (BRs) are a class of polyhydroxylated steroidal phytohormones that are essential for plant growth and development. Rice BRASSINOSTEROID-INSENSITIVE1 (BRI1)-ASSOCIATED RECEPTOR KINASES (OsBAKs) are plasma membrane-localized receptor kinases belonging to the subfamily of leucine-rich repeat receptor kinases. It has been found that in Arabidopsis, BRs induce the formation of a BRI1–BAK1 heterodimer complex and transmit the cascade signal to BRASSINAZOLE RESISTANT1/bri1-EMS-SUPPRESSOR1 (BZR1/BES1) to regulate BR signaling. Here, in rice (Oryza sativa ssp. japonica), we found that OsBZR1 binds directly to the promoter of OsBAK2, but not OsBAK1, and represses the expression of OsBAK2 to form a BR feedback inhibition loop. Additionally, the phosphorylation of OsBZR1 by OsGSK3 reduced its binding to the OsBAK2 promoter. The osbak2 mutant displays a typical BR-deficiency phenotype and negative modulates the accumulation of OsBZR1. Interestingly, the grain length of the osbak2 mutant was increased whereas in the cr-osbak2/cr-osbzr1 double mutant, the reduced grain length of the cr-osbzr1 mutant was restored, implying that the increased grain length of osbak2 may be due to the rice somatic embryogenesis receptor kinase-dependent pathway. Our study reveals a novel mechanism by which OsBAK2 and OsBZR1 engage in a negative feedback loop to maintain rice BR homeostasis, facilitating a deeper understanding of the BR signaling network and grain length regulation in rice.
Introduction
Brassinosteroids (BRs) are a class of widely distributed phytohormones that regulate plant growth. BR deficiency mutants are insensitive to BR treatment and display a severely dwarfed stature, are dark green, and have reduced root length (Clouse et al., 1996). As major plant hormones promoting plant growth, BRs regulate many biological processes, including cell elongation, division, and differentiation, root development, and leaf development (Yang et al., 2011). In the presence of BRs, transmembrane receptor kinase BR INSENSITIVE1 (BRI1) binds BR and trans-phosphorylates its co-receptor, BRI1 ASSOCIATED RECEPTOR KINASE1 (BAK1). BAK1 also trans-phosphorylates BRI1 to increase its kinase activity and transmit the BR signal to a specific substrate (Wang et al., 2008). The active BRI1–BR–BAK1 complex catalyses the phosphorylation of two membrane-localized receptor-like cytoplasmic kinases, BR SIGNALING KINASE (BSK) and CONSTITUTIVE DIFFERENTIAL GROWTH1 (CDG1). BSKs and CDG1 phosphorylate and activate the protein phosphatase bri1-SUPPRESSOR1 (BSU1) (Choe et al., 2002; Kim et al., 2011; Zhang et al., 2016a; Amorim-Silva et al., 2019). BSU1 dephosphorylates and inactivates GSK3/shaggy-like kinase BR INSENSITIVE2 (BIN2), which phosphorylates the transcription factors BRASSINAZOLE-RESISTANT1 (BZR1) and bri1-EMS-SUPPRESSOR1 (BES1) to negatively regulate BR signaling (Kim et al., 2009; Tong et al., 2012; Nolan et al., 2020).
BR regulates nuclear localization and protein accumulation of BZR1 and promotes dephosphorylation of BZR1 by protein phosphatase 2A (PP2A) (Ryu et al., 2007; Wang et al., 2021). The non-phosphorylated form of BZR1 subsequently regulates BR-responsive genes and modulates plant growth (Yin et al., 2005; Qiao et al., 2017). CGTGC/TG, referred to as the BR response element (BRRE), is one of the BZR1 binding sequences. BZR1 and BES1 confer feedback inhibition on BR biosynthesis by binding to the BRRE of genes for BR biosynthetic proteins, including CONSTITUTIVE PHOTOMORPHOGENESIS AND DWARF (CPD), DWARF4 (DWF4), ROTUNDIFOLIA3 (ROT3), and BRASSINOSTEROID-6-OXIDASE (BR6OX).
BZR1 binding ability can be induced by BR signaling (He et al., 2005). BZR1 and BES1 function as both transcriptional activators and repressors. Microarray data have demonstrated that there is significant overlap between BZR1 target genes and BR-responsive genes including BR-repressed genes and BR-induced genes. The BRRE and G-box (CACGTG) elements are more enriched in the BR-repressed targets, while the E-box motif (CATGTG) is more enriched in the BR-induced genes (Sun et al., 2010). In addition to some BR synthetic genes, BZR1 also represses the expression of BRI1, BES1/BZR1 homolog 1 (BEH1), and BEH2, to attenuate BR responses via negative feedback regulation. Almost half of the BES1/BZR1 binding sites are present in gene bodies, even several kilobases downstream of the transcription start site. Additionally, BES1 prefers to bind the −500 to 1500 regions, especially in proximity to the transcription start site (Yu et al., 2011).
In Arabidopsis, there are five homologous somatic embryogenesis receptor kinase (SERK) proteins, named SERK1, SERK2/BRI1-ASSOCIATED KINASE2 (BAK2), SERK3/BRI1-ASSOCIATED KINASE1 (BAK1), SERK4/BAK1-LIKE KINASE1 (BKK1), and SERK5, which participate in plant development and various signaling pathways (Li, 2010; Gou et al., 2012; Fan et al., 2016). The small extracellular domain of SERK proteins contains five leucine-rich repeats (LRRs), one serine–proline–proline (SPP) domain, one single-pass transmembrane domain, and one intracellular kinase domain (Aan den Toorn et al., 2015). SERKs have evolved to allow LRR receptor kinases to transduce information across membranes as adapter proteins without changing conformation. The amino acid residues from the SERK N-terminal capping domain combine with brassinolide (BL) (Hohmann et al., 2018). The interaction of kinase-associated protein phosphatase (KAPP) and SERK3 in a phosphorylation-dependent manner can accelerate SERK3 internalization in intracellular vesicles; KAPP sustained the dephosphorylated form of AtSERK1 and was required for AtSERK1 internalization via the endocytosis pathway (Shah et al., 2002). A previous study showed that accelerated endocytosis and internalization occurs when BRI1 and AtSERK3 heterodimerize with each other, which indicated activated BR signaling. Most of the internalized BRI1 and AtSERK3 proteins were degraded (Russinova et al., 2004). Endocytosis of activated BRI1 regulates BR signaling negatively, resulting in signal attenuation (Martins et al., 2015). SERKs have functions independent of their role in BR signaling in Arabidopsis, and phosphorylation of SERKs is regulated independently for BR signaling, cell death, plant innate immunity, and root development (Schwessinger et al., 2011; Fan et al., 2016).
Many components participating in rice BR signaling have been identified, most of which are functionally identical to those in Arabidopsis (Tong and Chu, 2018), including OsBRI1, OsBAK1, OsBSK3, OsGSK1/2/3, and OsBZR1 (Park et al., 2011; Tong et al., 2012; Zhao et al., 2013; Zhang et al., 2016a; Tian et al., 2021). However, unlike BSU1, qGL3/OsPPKL1 negatively regulates BR signaling. The qGL3 mutant displays an increased sensitivity to exogenous BL treatment compared with the wild type and has a longer grain size (Gao et al., 2019). More extensive investigation of the qGL3/OsPPKL1-mediated regulatory network with data from phosphoproteomic and interactomic screening revealed qGL3/OsPPKL1-dependent components in rice, including OsGF14b, OsAK3, OsVIL1, OsMAPK4, OsCCL, OsRCF3, OsCPK24, OsJAZ6, and OsUBC15. Mutation of their genes altered plant height and grain size, and sensitivity to BR treatment also greatly changed (Gao et al., 2022b). OsGF14b works as a negative regulator in BR signaling, and OsPPKL1 mediates an increase in the phosphorylation state of OsGF14b. The interaction between OsGF14b and OsBZR1 promotes the cytoplasmic localization of OsBZR1 and reduces the transcriptional activation of OsBZR1 (Gao et al., 2022a). OsAK3 interacts with OsPPKL1, and mutation of OsAK3 caused reduced sensitivity to exogenous BR treatment, resulting in small grain size and dwarf plants (Zhang et al., 2021).
Four homologous SERKs were identified in rice, namely OsBAK1 (OsSERK1), OsBAK2 (OsSERK2), OsSERK3, and OsSERK4. OsBAK1 and OsBAK2 are homologs of AtSERK1 and AtSERK2, respectively. OsBAK1 regulates lamina joint, plant height, and grain size to modify rice architecture, and overexpression of OsBAK1 gave the strongest phenotypic recovery of the BR-deficient mutant bri1-5 compared with OsBAK2, OsSERK3, and OsSERK4. Additionally, these SERK proteins are involved in rice BR signaling and multiple plant immunity responses independent of BR (Li et al., 2009). OsBAK2 is a multidomain integral membrane protein, and the intracellular portion of the protein consists of a juxta-membrane and kinase domain (McAndrew et al., 2014). OsBAK1 is 87% identical to OsBAK2 and 66–87% identical to other SERK family kinases in its entire amino acid sequence. The expression of OsBAK2 in all organs has similar levels (Ito et al., 2005). Rice SERKs are involved in BR signaling, and plants overexpressing OsBAK1 are semi-dwarfed and hypersensitive to 24-epiBL (Yuan et al., 2017). OsBAK2 can interact with OsBRI1 and is critical for BR-regulated development (Dong et al., 2020). Moreover, OsBAK2 plays an important role in immune signaling pathways including three immune-related protein kinases: Xa21, Xa3, and OsFL2. OsBAK2–Xa21 interaction is critical for resistance to Xanthomonas oryzae pv. oryzae, but does not depend exclusively on four specific tyrosine residues of the kinase domain in Xa21 (Caddell et al., 2018).
In this study, we obtained a rice BR-deficient mutant, osbak2, which is insensitive to BR and highly sensitive to BRZ. The mutant showed decreased lamina joint bending and a dwarf phenotype. OsBAK2 regulates BR signaling positively through OsBRI1 but regulates grain length negatively. Here we confirmed that OsBAK2 is a target gene of OsBZR1. OsBZR1 represses OsBAK2 expression via a BR feedback loop. Our results reveal a novel molecular mechanism in which OsBAK2 not only acts as an OsBRI1 co-receptor to transduce the BR-mediated signal but also attenuates the BR response in a feedback inhibition loop.
Materials and methods
Plant materials and growth conditions
Rice (Oryza sativa ssp. japonica) cultivars Zhonghua11 (ZH11) and Nanjing9108 (NJ9108) were used to generate transgenic plants. To construct OE-OsBAK2 or OE-OsBZR1 transgenic plants, the whole coding sequence (CDS) was cloned into pCAMBIA1300s plasmid containing the 35S promoter. The T-DNA insertion mutant osbak2 (PFG_3A-16371.R) was obtained from the rice genome database (http://signal.salk.edu/cgi-bin/RiceGE). Rice (Oryza sativa ssp. japonica) cultivar Dongjin (DJ) was used as wild type. The plants were grown in the field at natural long-day conditions in Nanjing, China, or in a growth chamber at 28 °C for 14 h light (light intensity 120 µmol m−2 s−1) and 24 °C for 10 h dark.
BL and BRZ treatment assay
For the BR-induced lamina joint bending assay, seeds were grown for 10 d in a dark chamber at 28 °C. Then seedling segments of 1–2 cm contained the first or second lamina joint bend were excised and incubated in different concentrations of 28-homoBL (Macklin, China) for 3 d in the dark.
To test the influenced of transcript level and protein expression level between wild type and transgenic lines with 28-homoBL treatment, 2-week-old seedlings cultured in nutrient solution were treated with 1 μM BL for 0, 6, and 12 h. Samples were collected immediately and frozen in liquid nitrogen for RNA and protein extraction. Commercial anti-OsBZR1 (AbP80051-A-SE, BPI) and rice heat shock protein (AbM51099-31-PU, BPI) were used for western blot. Detection of OsGSK3 protein used laboratory prepared polyclonal antibodies (Gao et al., 2019). To assess the inhibition of high BL concentration on the elongation of the coleoptile and root length, seeds were grown in darkness for 5 d on 0.3% agar medium containing 0, 0.1, 1, 5, 10, and 50 μM BL after germination. For BRZ treatment, seeds were sown in nutrient solution containing 0, 5, and 20 μM BRZ, and data were statistically analysed after 10 d.
Scanning electron microscopy analysis
Different lines of mature rice seeds were washed by ultrasonic cleaner, put in an electric heat-blast drying oven at 85 °C for 2 d, and the dried samples were sputter-coated with platinum. The outer epidermal cells of the lemma were observed by scanning electron microscopy (Hitachi, Regulus 8100). Cells of the middle part were observed and imaged for counting cell number and measuring cell length and width by ImageJ software.
Quantitative real-time PCR analysis
Total RNA was extracted from 2-week-old rice seedlings using the High Purity Total RNA Rapid Extraction Kit (Tiangen) and treated with DNase I. Complementary (cDNA) was generated with HiScript III RT SuperMix for qPCR (+gDNA wiper) (Vazyme). RT-qPCR was performed with SYBR Green PCR master mix (Vazyme). The actin gene Os03g0718100 and ubiquitin fusion protein (UBQ) Os03g0234200 were used as internal controls to normalize the quantitative real-time PCR (RT-qPCR) data, respectively. The gene expression levels were calculated with the 2-△△Ct method (Zhang et al., 2021). Student’s t-test was used to assess statistical significance of differences between wild type and transgenic lines. Each analysis was repeated three times. The primer used are listed at Supplementary Table S1.
Yeast one-hybrid assay
Each fragment of promoter sequences (LacZ-M1, LacZ-M2, LacZ-M3, LacZ-OsBAK1) was ligated into the pLacZi vector (reporter) and the coding sequence of OsBZR1 was cloned into the Pb42AD vector (effector). They were co-transformed into yeast strain EGY48 and plated on minimal synthetic defined (SD) base supplemented with the −Ura/−Trp dropout (DO) mix using X-gal for screening.
Electrophoretic mobility shift assay
An electrophoretic mobility shift assay (EMSA) was performed using recombinant His–OsBZR1 protein purified from Escherichia coli strain BL21 (DE3). Glutathione S-transferase (GST)–OsGSK3 purified protein was incubated with His–SUMO–OsBZR1 in kinase buffer with or without ATP at 30 °C for 60 min, and 20 μM bikinin was added to the phosphorylation reaction system as required. The biotin-labeled double-stranded oligonucleotide probes containing G-box or BRRE sequences of OsBAK2 promoter were generated by a company (Genewiz, Suzhou, China). Detection of biotin-labeled DNA was performed using a chemiluminescence EMSA kit (Beyotime, Shanghai, China) based on the manufacturer’s instructions, with corresponding unlabeled regions used as competitors. Probe sequences are listed in Supplementary Table S1.
Transient transcription dual-luciferase assay
For the transient expression of OsBZR1’s target gene, the OsBAK2 and OsBAK2 promoter region was cloned into pGreenII 0800-LUC vector as a reporter, and the coding sequence of OsBZR1 was cloned into PAN580 as an effector. Renilla luciferase (REN) driven by the 35S promoter was used as an internal control. The effector and reporter were co-transfected into rice protoplasts. The transfected cells were treated with or without 1 μM BL and incubated for 16 h before detection. Luciferase activity was measured using a dual-LUC reporter assay kit (Promega). The LUC/REN ratio was calculated and the relative ratio was used as the final measurement.
Chromatin immunoprecipitation coupled with qPCR analysis
For chromatin immunoprecipitation (ChIP)-qPCR assays, the coding sequence of OsBZR1 was cloned into the green fluorescent protein (GFP) tag in pCAMBIA1300s to generate pCAMBIA1300s-GFP-OsBZR1 transgenic plants and a pCAMBIA1300s-GFP transgenic line as a control. ChIP assays were performed as previously reported (Zheng et al., 2019). 2-week-old transgenic rice seedlings were submerged in 1% formaldehyde to cross-link genomic DNA and protein under a vacuum. The chromatin complex was sonicated to shear DNA to a mean size of 200–500 bp. Immunoprecipitation was performed with anti-GFP antibody. The amount of each DNA fragment was determined by RT-qPCR and the values in pCAMBIA1300s-GFP were set as 1. Primers used for qPCR amplification are listed in Supplementary Table S1.
Accession numbers
Sequence data from this article can be found in the GenBank/EMBL database libraries under the following accession numbers: OsBAK2, Os04g38480; OsBZR1, Os07g39220; OsGSK3, Os02g14130; OsD2, Os01g10040; OsD11, Os04g39430; OsDWF4, Os03g12660; CYP734A2, Os02g11020; CYP734A4, Os06g39800; CYP734A6, Os01g29150; OsBAK1, Os08g07760; OsSERK3, Os06g12120; OsSERK4, Os02g18320.
Results
Morphological analysis of osbak2 mutant
A previous study has shown that the plant height and grain size of the osbak1 (08SG2) mutant were reduced compared with wild type (Yuan et al., 2017). To study a gene homologous to OsBAK1, we obtained an osbak2 mutant from Korea rice mutant library (Supplementary Fig. S1A, B) (Jeong et al., 2006; Yi and An, 2013). Interestingly, we found that the osbak2 mutant had reduced plant height, similar to the BR-deficient mutants d61-1 and dlt (Fig. 1A, C) (Tong et al., 2009). The grain length was obviously increased while the width showed no significant variation compared with the wild type, DJ (Fig. 1B, C). Since osbak2 produced large grains, we examined the outer epidermal cell size of DJ and osbak2 spikelet hulls. The number of outer epidermal cells in osbak2 was more than that of DJ while the cell length and width in the central part of lemma were reduced (Fig. 1D, E). OsBAK2 regulates grain size mainly through cell proliferation.
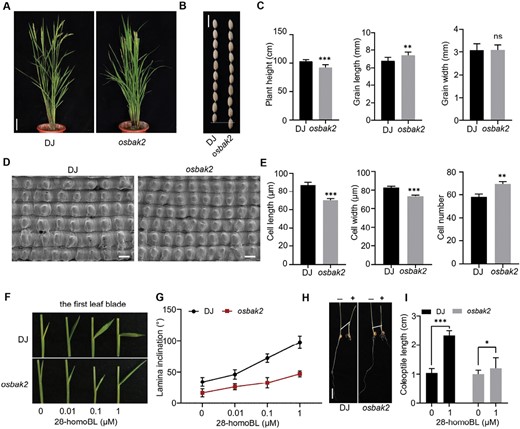
Characteristics of osbak2 mutant. (A) Gross morphology of wild type DJ and osbak2 mutant at the reproductive stage. Scale bar, 10 cm. (B) Grain size of DJ and osbak2 mutant. Scale bar, 1 cm. (C) Statistical data for plant height, grain length, and grain width of DJ and osbak2. Data are means ±SD (n=12 for plant height, n=30 for grain length and width). P-values from Student’s t-test of osbak2 against DJ are indicated (**P<0.01, ***P<0.001; ns, not significant). (D) Scanning electron microscopy analysis of glume outer surfaces of mature seeds. Scale bars, 60 μm. (E) Statistical analysis of cell length, cell width, and cell number in the outer epidermal cells. Data are given as means ±SD (n=4). Student’s t-test was used to calculate P-values (**P<0.01, ***P<0.001). (F) Response of lamina joint bending in the first leaf blade to different concentrations of 28-homoBL. (G) Statistical analysis of leaf inclination in (F); data are given as means ±SD (n=15). (H) Comparison of coleoptile elongation between DJ and osbak2 mutant in the presence of 1 μM 28-homoBL; white line represents the degree of elongation. Scale bar, 1 cm. (I) Statistical analysis of coleoptile elongation under BL treatment. Data are given as means ±SD (n=20). Student’s t-test was used to calculate P-values (*P<0.05, ***P<0.001).
Two-week-old seedlings displayed erect leaves and reduced second internode length (Supplementary Fig. S2A–D). Exogenous BL treatment increases coleoptile length and lamina inclination (Zhang et al., 2016b). A lamina inclination assay showed that, compared with DJ, both the first and the second leaf blades of osbak2 were insensitive to different concentrations of 28-homoBL, an active form of BR (Fig. 1F, G; Supplementary Fig. S2E, F) (Sirhindi et al., 2009; Gao et al., 2022a). Furthermore, coleoptile growth after treating with 1 μM BL showed that exogenous BL increased coleoptile length of DJ but not osbak2 (Fig. 1H, I). A RT-qPCR assay indicated that the transcript expression levels of three BR biosynthesis genes, OsDWF4, OsD2, and OsD11 were higher in osbak2 (Supplementary Fig. S3A). Notably, the expression of these BR-biosynthesis genes was decreased after BL treatment in DJ but increased in osbak2 (Supplementary Fig. S3B–D). Several BR catabolic enzymes degrade BRs, including CYP734A2, CYP734A4, CYP734A5, and CYP734A6 (Sakamoto et al., 2011). The relative expression of CYP734A2, CYP734A4, and CYP734A6 was significantly down-regulated in osbak2 compared with DJ (Supplementary Fig. S3E–G). The expression level of CYP734As was increased rapidly by BL treatment in DJ and increased less in osbak2.
To further investigate the role of OsBAK2 in BR signaling, a high concentration BL sensitivity assay was performed with DJ and osbak2. With increasing BL concentrations, the coleoptile elongation and inhibition of root length in osbak2 were not significant compared with DJ. Notably, the coleoptile elongation of DJ was obviously inhibited under 50 μM BL treatment conditions. Compared with DJ, the degree of coleoptile elongation inhibition in osbak2 was significantly reduced (Fig. 2A, C). The result suggested that osbak2 exhibited insensitivity to high BL concentrations. Exogenous brassinazole (BRZ; a BR biosynthesis inhibitor) treatment showed that the first internode length and seedling length of osbak2 were reduced significantly more than that of ZH11 under 5 μM and 20 μM BRZ conditions, suggesting that osbak2 is more sensitive to BRZ (Fig. 2B, D). These results indicated that osbak2 is insensitive to BL, and BR signaling is suppressed in osbak2 mutant.

OsBAK2 is involved in the rice BR signaling. (A) Phenotypes of coleoptile elongation and root length in DJ and osbak2 under different treatment concentrations of 28-homoBL (0, 0.1, 1, 5, 10, and 50 μM) for 5 d. White line represents the degree of elongation. Scale bar, 1 cm. (B) Germinated seeds of DJ and osbak2 sown in nutrient solutions with different concentrations of BRZ (0, 5, 20 μM) for 10 d. White arrows represent the first internode position; scale bars, 2 cm. (C) Statistical analysis of BL response of relative coleoptile elongation and root length compared with the respective mock values in (A). Data are means ±SD (n=15). Student’s t-test was used to calculate P-values (**P<0.01, ***P<0.001) to compare the relative coleoptile elongation and root length, respectively, at the same BL concentration treatment between DJ and osbak2. (D) Statistical analysis of BRZ response for relative internode length and seedling elongation under different concentrations of BRZ, compared with the respective mock values in (B). Data are means ±SD (n=15). Different letters (P<0.05, one-way ANOVA) compare DJ and osbak2.
OsBAK2 is a direct target gene of OsBZR1
OsBZR1 acts as both a transcriptional activator and a transcriptional repressor as determined by the sequence of the binding motif, and both G-box (CACGTG) and BRRE (CGTGC/TG) are putative binding motifs for OsBZR1 (Sun et al., 2010). We found that the OsBAK2 promoter contains BRRE (−1664 bp upstream of the predicted translation start site) and G-box (−1584 bp upstream of the predicted translation start site) cis-elements (http://plantregmap.gao-lab.org/) (Fig. 3A). The abovementioned findings prompted us to confirm whether OsBZR1 could bind to the promoter of OsBAK2. Then a yeast one-hybrid (Y1H) assay was performed and it was observed that OsBZR1 bound to the OsBAK2 promoter with the region of the sequence containing BRRE and the G-box while no binding signal was found between OsBZR1 and the promoter of OsBAK1 (Fig. 3B). OsBZR1-GFP transgenic plants were generated for ChIP-qPCR. The result revealed that the significant enrichment of the OsBAK2 promoter fragment containing the BRRE and G-box motifs (S2, −1704 to −1522, upstream of the predicted translation start site) (Fig. 3C). However, there is neither enrichment in the region of S1 and S3 (−2460 to −2215, −578 to −433, upstream of the predicted translation start site) nor in the region of the 5ʹ untranslated region (UTR) compared with the pCAMBIA1300s-GFP transgenic plant (Fig. 3C). These data suggested that OsBAK2 is a direct target gene of OsBZR1.

OsBZR1 binds directly to the OsBAK2 promoter. (A) Schematic diagram of BRRE and G-box motif in OsBAK2 promoter. The BRRE motif and the G-box motif are marked with brown and blue, respectively. M1 to M3 indicate the different regions of the OsBAK2 promoter; the M1 fragment contains the BRRE motif, the M2 fragment contains the G-box motif, and the M3 fragment contains both BRRE and G-box motifs. (B) Yeast one-hybrid assays showed OsBZR1 binds to the promoter of OsBAK2 but not OsBAK1. The empty vector pB4AD and pLacZi were used as negative control. (C) ChIP-qPCR showing the binding of OsBZR1 to the BRRE motif and G-box motif in the promoter of OsBAK2 in vivo using anti-GFP antibody. Four regions (S1, S2, S3, 5ʹUTR) were investigated and the region S2 contained the BRRE and G-box motifs. Data are means ±SD (n=3), Student’s t-test was used to calculate P-values (**P<0.01; ns, not significant).
The repression by OsBZR1 of OsBAK2 expression is affected by OsGSK3
To further reveal how OsBZR1 regulates the expression of OsBAK2, transient transactivation assays were performed in rice protoplasts. It was found that 35Spro:OsBZR1 as an effector can significantly suppress the expression of the Luciferase (LUC) reporter gene, driven by the OsBAK2 promoter containing BRRE and G-box motifs. It was observed that the relative LUC activity of the OsBAK1 promoter remained unchanged (Fig. 4A, B). Additionally, exogenous BL remarkably enhanced the transcriptional repression in OsBAK2 promoter by OsBZR1, which was consistent with the positive role of BL on OsBZR1 protein accumulation. As a negative regulator of BR signaling in rice, OsGSK3 phosphorylated OsBZR1 to repress its activity, and mutation of OsGSK3 enhanced BR signaling (Gao et al., 2019). Interestingly, the repression of OsBAK2–LUC reporter activity was decreased significantly when co-expressed with 35Spro:OsBZR1 and OsGSK3-GFP in rice protoplasts, demonstrating that the transcriptional repression of OsBAK2 by OsBZR1 was abrogated in the presence of OsGSK3 (Fig. 4B). To further confirm the regulation of the OsBAK2 by OsBZR1 and OsGSK3, we performed an EMSA using purified recombinant OsBZR1 protein. There is a BRRE and a G-box cis-element in the OsBZR1 binding fragments of the OsBAK2 promoter; DNA probes containing BRRE or G-box sequences were generated with biotin (Fig. 4C). Both probes can be detected by binding strongly His–SUMO–OsBZR1 and the binding could be competed out by unlabeled probe, while unlabeled mutant probes in which the BRRE motif or G-box motif was replaced by AAAAAA could not abolish the binding of the probes to His-SUMO-OsBZR1 (Fig. 4D, E). In parallel, we used these probes to investigate whether the phosphorylation of OsBZR1 by OsGSK3 has an effect on the binding ability; His–SUMO–OsBZR1 and GST–OsGSK3 were co-incubated with ATP. As shown, when His–SUMO–OsBZR1 and GST–OsGSK3 were co-incubated, the binding affinity of OsBZR1 to the probes containing BRRE and G-box was significantly reduced. However, the application of bikinin (ATP-competitive inhibitor of GSK3 kinases) restored the binding intensity of OsBZR1 to DNA probes containing BRRE or the G-box motif (Fig. 4D, E). All of the above results support the view that OsBZR1 regulates OsBAK2 expression as a transcriptional repressor, and OsGSK3 prevented the binding of OsBZR1 to both the BRRE and G-box motif of the OsBAK2 promoter by phosphorylating OsBZR1.

OsBZR1 represses OsBAK2 at the transcriptional level and the repression is attenuated by phosphorylation of OsGSK3. (A) Schematic diagram of the effector and reporter used in the transient assay in rice protoplasts. The coding sequence of OsBZR1 driven by the 35S promoter acts as the effector, and the promoter containing the BRRE motif or the G-box motif fused with LUC act as reporter. (B) Transient transcription dual-luciferase assay in rice protoplasts showed that OsBZR1 repressed the activity of OsBAK2 promoter but not OsBAK1. In transfected cells treatment with 1 μM BL enhanced the repression of OsBAK2 promoter activity by OsBZR1, and the repression was prevented by the co-expression of OsGSK3–GFP. M1 to M3 indicate the different regions of the OsBAK2 promoter; the M1 fragment contains the BRRE motif, the M2 fragment contains the G-box motif, and the M3 fragment contains both BRRE and G-box motifs. Statistical analysis was represented as the activity of LUC/REN ratio. Data are means ±SD (n=20). Student’s t-test was used to calculate P-values (**P<0.01, ***P<0.001; ns, not significant). (C) The positions and identified DNA sequences of the wild type or mutant (Mu) probes used for EMSA. (D, E) EMSA showing the specific binding of OsBZR1 to the BRRE motif and the G-box motif of OsBAK2, respectively. The hot probe was a biotin-labeled DNA sequence of the OsBAK2 promoter containing the target motif. Unlabeled P1 and P2 were used as competitor probes at 100-fold that of the biotin labeled probes. For lanes 5 and 6 of (D, E), phosphorylated His–SUMO–OsBZR1 was incubated with the probe mentioned above, and 20 μM bikinin was added in lane 6 to inhibit GSK3 kinase activity. Arrow indicates the specific OsBZR1 binding.
Genetic analysis of OsBZR1 and OsBAK2 in BR signaling and grain length regulation
To analyse the genetic relationship between OsBZR1 and OsBAK2, we firstly created an OsBAK2 knockout mutant with CRISPR/Cas9 in ZH11 background (Supplementary Fig. S4), and obtained two OsBAK2-edited lines, denoted cr-osbak2-L1 and cr-osbak2-L2, which caused extremely reduced plant height and larger grain length (Fig. 5A–D). An OsBAK2-overexpressing line (OE-OsBAK2) was generated in ZH11 background. Consistent with osbak2, the coleoptile elongation and root length inhibition analysis showed that cr-osbak2 was insensitive to increasing BL concentration, and OE-OsBAK2 presents a sensitive phenotype compared with ZH11. However, the coleoptile elongation was significantly inhibited in OE-OsBAK2 under 50 μM BL treatment compared with ZH11 (Supplementary Fig. S5A, B). Compared with ZH11, cr-osbak2 exhibited strong reduction of first internode length and seedling length under BRZ treatment, but the growth inhibition was weaker in OE-OsBAK2 than in ZH11 (Supplementary Fig. S5C, D). The results indicated that OE-OsBAK2 responds to BL more strongly than ZH11, while the response to BRZ was significantly weaker than for ZH11 and cr-osbak2.

Morphological analysis of OsBAK2 and OsBZR1 transgenic plants. (A) The gross morphology of cr-osbak2, OE-OsBAK2, cr-osbak2/cr-osbzr1, cr-osbzr1, and OE-OsBZR1 compared with wild type ZH11 at the reproductive stage. Scale bar, 10 cm. (B) Grain phenotype of ZH11, cr-osbak2, OE-OsBAK2, cr-osbak2/cr-osbzr1, cr-osbzr1, and OE-OsBZR1. Scale bar, 1 cm. (C) Statistical data for plant height in (A) are means ±SD (n=12). Different letters (P<0.05, one-way ANOVA) compare plant height between ZH11 and transgenic lines. (D) Statistical data for grain length in (B) are means ±SD (n=30). Different letters (P<0.05, one-way ANOVA) compare grain length between ZH11 and transgenic lines.
The cr-osbzr1 line was available in our lab and displayed decreased grain length (Gao et al., 2022a). Next, we obtained cr-osbak2/cr-osbzr1 double mutant lines with the CRISPR/Cas9 system. The phenotypes of cr-osbak2/osbzr1 plants are similar to cr-osbak2 in plant height, and the grain length of the double mutant was slightly longer than that of ZH11, much shorter than that of the cr-osbak2 mutant, but longer than that of the cr-osbzr1 mutant (Fig. 5A–D). The double mutant could rescue the decreased grain length of cr-osbzr1. An OsBZR1-overexpressing line (OE-OsBZR1) was also generated in ZH11 background, and both OE-OsBAK2 and OE-OsBZR1 showed reduced plant height, and in contrast to the slightly enlarged grain length of OE-OsBZR1, OE-OsBAK2 showed a decreased grain length (Fig. 5A–D). We also generated OsBAK2-edited lines using CRISPR/Cas9 in Nanjing9108 (NJ9108) background. cr-osbak2-NJ9108 displayed reduced plant height and large grain size compared with NJ9108, similar to osbak2 mutant (Supplementary Fig. S6A–C).
In addition, 2-week-old seedlings of cr-osbak2 displayed a reduced lamina joint while OE-OsBAK2 restored the leaf angle to that of ZH11, which indicated that OsBAK2 enhanced the BR-related phenotype. Similar to cr-osbak2, cr-osbzr1 displayed decreased leaf angle, but OE-OsBZR1 showed no obvious change compared with WT (Supplementary Fig. S7A, B). We also found that the lamina joint bending of the double mutant cr-osbak2/cr-osbzr1 still showed a phenotype with significantly erect leaves (Supplementary Fig. S7A, B). For the second internode length, all the transgenic lines exhibit a significantly shortened internode except OE-OsBZR1 (Supplementary Fig. S7C, D).
To further investigate whether the relationship between OsBZR1 and OsBAK2 was related to BR signaling. We performed a BL treatment assay with different concentrations (0, 0.1, 1 μM) to investigate the lamina joint bending and coleoptile length induced by 28-homoBL. As expected, the first and the second lamina joint of cr-osbak2 seedlings showed no alteration under 28-homoBL incubation, and cr-osbak2 had a lower elongation of coleoptile length than ZH11, suggesting that OsBAK2-edited lines were less sensitive to 28-homoBL (Fig. 6A–D). However, OE-OsBAK2 was hypersensitive to BL with a larger leaf angle and longer coleoptile (Fig. 6A–D). cr-osbzr1 also displayed reduced BR sensitivity while OE-OsBZR1 was phenotypically similar to OE-OsBAK2, including significantly increased leaf angle and evidently coleoptile elongation. In addition, the leaf blade and coleoptile growth of the cr-osbak2/cr-osbzr1 double mutant showed a reduction in BR sensitivity compared with ZH11 (Fig. 6A–D). These results confirmed that OsBAK2 acts collaboratively with OsBZR1 in BR signaling. Based on the results of the BL sensitivity of these OsBAK2 and OsBZR1 mutants, we speculated that, similar to BR signaling in Arabidopsis, OsBAK2 acts with OsBZR1 in regulating the BR response.

BL sensitivity assays of OsBAK2 and OsBZR1 transgenic plants. (A) Lamina bending evaluated as the angle of the first and second leaf after different concentration gradients of 28-homoBL treatment. (B) Statistical analysis of BL response for the relative increase of leaf inclination in cr-osbak2, cr-osbak2/cr-osbzr1, cr-osbzr1, OE-OsBAK2, and OE-OsBZR1, compared with the respective mock values. Data are means ±SD (n=15). Different letters (P<0.05, one-way ANOVA) compare relative angle of lamina inclination at the same BL concentration treatment between ZH11 and transgenic lines. (C) Coleoptile elongation of ZH11, cr-osbak2, cr-osbak2/cr-osbzr1, cr-osbzr1, OE-OsBAK2, and OE-OsBZR1 in the absence or presence of 1 μM 28-homoBL. The white line represents the degree of elongation. Scale bar, 1 cm. (D) Statistical analysis of BL response for coleoptile length in (C). Data are means ±SD (n=20). Different letters (P<0.05, one-way ANOVA) compare coleoptile elongation at 1 μM 28-homoBL treatment between ZH11 and transgenic lines.
OsBAK2 promotes BR signal transduction in an OsBZR1-mediated manner
As described above, OsBAK2 deficiency resulted in several BR-related phenotypes including reduced BR sensitivity. To further explore the molecular mechanism of how OsBAK2 participates in the BR pathway, we measured the transcript expression level of several BR biosynthetic genes by RT-qPCR, including OsDWF4, OsD2, and OsD11. The expression of these biosynthetic genes was up-regulated in OsBAK2-edited transgenic lines to partially restore the defect in BR signaling, but repressed in OE-OsBAK2 transgenic lines compared with ZH11 (Fig. 7A). As expected, we found that the transcript level of BR biosynthetic genes was obviously increased in cr-osbzr1 and down-regulated in OE-OsBZR1 compared with ZH11. OsDWF4, OsD2, and OsD11 had increased expression levels in cr-osbak2/osbzr1 that were similar to cr-osbak2 and cr-osbzr1. Together with the result that OsBAK2 mutants were insensitive to BR treatment, we found that defective OsBAK2 and OsBZR1 weaken the BR signaling, thereby promoting BR synthesis through feedback regulation (Fig. 7A). Furthermore, the inhibition of the expression of OsBAK2 by 28-homoBL treatment was rescued in cr-osbzr1 (Fig. 7B). Previous study showed the transcript expression of OsBZR1 was greatly repressed by 1 μM BL treatment (Zhang et al., 2012). Transcript expression of OsBZR1 in cr-osbak2 transgenic lines was highly increased and that in OE-OsBAK2 was lower than in ZH11 (Fig. 7C). The transcript expression of OsGSK3, which negatively regulates the BR pathway, was induced in both cr-osbak2, cr-osbzr1, and OE-OsBAK2 compared with ZH11 whereas no significant differences were observed in OE-OsBZR1 (Fig. 7D). These results suggest that the BR response is partially inhibited in OsBAK2-edited lines, similar to in OsBZR1-edited lines, and enhanced in OE-OsBAK2 plants.

OsBAK2 affects the expression of BR-related genes and accumulation of OsGSK3 and OsBZR1. (A) The relative expression level of BR biosynthetic genes, including OsDWF4, OsD2, and OsD11, in ZH11, cr-osbak2, OE-OsBAK2, cr-osbak2/cr-osbzr1, cr-osbzr1, and OE-OsBZR1. The expression of ZH11 was defined as ‘1’. Data are means ±SD (n=3). Student’s t-test was used to calculate P-values (*P<0.05, **P<0.01, ***P<0.001; ns, not significant). (B) The relative expression level of OsBAK2 in cr-osbzr1 and OE-OsBZR1 in response to exogenous 1 μM 28-homoBL for 6 h compared with ZH11, determined by RT-qPCR. The expression level of OsBAK2 in ZH11 without BL treatment was set as ‘1’, and the expression level in dimethyl sulfoxide (DMSO) treatment for each line was used as control. Data are shown as means ±SD (n=3). Student’s t-test was used to calculate P-values (**P<0.01, ***P<0.001; ns, not significant). (C) The relative expression level of OsBZR1 in cr-osbak2 and OE-OsBAK2 treated with 1 μM 28-homoBL for 6 h compared with ZH11. The expression level of OsBZR1 in ZH11 without BL was set as ‘1’, and the expression level in DMSO treatment for each line was used as control. Data are shown as means ±SD (n=3). Student’s t-test was used to calculate P-values (**P<0.01, ***P<0.001; ns, not significant). (D) Relative expression of BR-related gene OsGSK3 in cr-osbak2, OE-OsBAK2, cr-osbak2/cr-osbzr1, cr-osbzr1, and OE-OsBZR1 treated with 1 μM 28-homoBL for 6 h compared with ZH11. The expression level of OsGSK3 in ZH11 without BL was set as ‘1’, and the expression level in DMSO treatment for each line was used as control. Data are shown as means ±SD (n=3). Student’s t-test was used to calculate P-values (**P<0.01, ***P<0.001; ns, not significant). (E) Quantification of OsBZR1 protein levels in OsBAK2 mutant transgenic lines extracted from 2-week-old seedlings. The band intensity was calculated by ImageJ and normalized. The relative protein level of OsBZR1 in each wild type was set as ‘1’, and OsBZR1 was detected with anti-OsBZR1 antibody. Rice heat shock protein (HSP) was used for normalization as an internal control and detected with anti-HSP antibody. (F) Western blot analysis of ZH11, cr-osbak2, and OE-OsBAK2 treated with 1 μM 28-homoBL for different times as shown to detect the abundance of OsBZR1 protein (top) and BR related protein OsGSK3 (middle). The respective relative protein level of OsBZR1 and OsGSK3 in ZH11 was set as ‘1’. Rice HSP was used as an internal control. The band intensity was measured using ImageJ and normalized.
We examined the alteration of OsBZR1 protein accumulation in these transgenic plants by western blot using anti-OsBZR1 antibody. Compared with wild type ZH11, OsBZR1 protein level was impaired in cr-osbak2 and cr-osbak2-NJ9108 (Fig. 7E). The osbak2 mutant also showed an extremely reduced protein level of OsBZR1 compared with DJ (Fig. 7E). These results indicate that OsBAK2 positively modulates OsBZR1 protein level. Furthermore, western blot analysis showed that the OsBZR1 protein level was accumulated in the presence of exogenous BL with a 0 h–6 h–12 h gradient treatment in these transgenic lines including ZH11, cr-osbak2, and OE-OsBAK2. cr-osbak2 had an extremely significant accumulation of the protein, from 0.48 to 1.61 after 12 h BL treatment (Fig. 7F). We also determined the protein level of OsGSK3, which was enhanced in OsBAK2-edited lines and significantly decreased in OsBAK2-OE transgenic plants compared with ZH11 (Fig. 7F). Our analysis suggests that the enhancement of BR signaling results in the attenuation of OsGSK3 protein expression. We showed that the protein level of OsGSK3 is suppressed gradually by exogenous BL in all these lines, ZH11, cr-osbak2, and OE-OsBAK2 (Fig. 7F). It is noticeable that compared with ZH11 and cr-osbak2, OsGSK3 protein level were greatly reduced in OE-OsBAK2. Taking these results together, they demonstrate that OsBAK2 contributes to BR signaling partially in an OsBZR1-mediated manner.
The expression pattern of OsBAK2 is distinct from other SERK proteins in rice
To expand our knowledge of how OsBZR1 regulates OsBAK2, 2-week-old seedlings and the flag leaves of rice with completed filling were used for analysis. Interestingly, the transcriptional level of OsBZR1 was repressed after rice filling, and meanwhile, the transcript expression level of OsBAK2 was substantially activated at maturity (Fig. 8A). Similarly, we also observed the same expression patterns of OsBZR1 and OsBAK2 between the seedling and the mature stage in DJ (Fig. 8A). Consistent with this repression model, the OsBZR1 protein levels were greatly reduced at the mature stage compared with the seedling stage in ZH11 and DJ (Fig. 8B). Since OsBZR1 represses the transcription of OsBAK2, substantial activation of OsBAK2 was found in the OsBZR1-repressed mature stage of rice. We simultaneously assessed whether the promoter of other SERK family protein kinases contained an OsBZR1 binding motif. It was found that no OsBZR1 binding motif was predicted in the promoter of OsBAK1 (Os08g07760), OsSERK3 (Os06g12120), and OsSERK4 (Os02g18320). The transcript expression of OsBAK1, OsSERK3, and OsSERK4 was also not significantly different in OE-OsBZR1 transgenic plants compared with ZH11 (Fig. 8C). Notably, rice microarray analysis indicated that the expression of OsBAK2 was distinct from that of OsBAK1 in various tissues and developmental stages, including seedling, leaf, shoot apical meristem, diverse stages of panicle (P1–P4), and seed (Fig. 8D) (Jain et al., 2007). The data also showed the expression of OsBAK2 was lower than the expression of OsBAK1, OsSERK3, and OsSERK4 during every stage of panicle development (Fig. 8D). We speculate that the longer grain size of the osbak2 mutant is due to the low expression of OsBAK2 in the panicle, as distinct from that of other rice SERK genes, which affects gametophyte and pollen development.

The expression pattern of OsBAK2 is distinct from other SERK proteins in rice. (A) The relative expression level of OsBZR1 and OsBAK2 at seedling stage and maturity stage of two wild type cultivars, ZH11 and DJ. The respective expression level of OsBZR1 and OsBAK2 at seedling stage was set as ‘1’. Data are shown as means ±SD (n=3). Student’s t-test was used to calculate P-values (***P<0.001). (B) Protein accumulation of OsBZR1 in ZH11 and DJ detected by western blot using anti-OsBZR1 antibody. Rice HSP was used as an internal control, and the relative abundance of OsBZR1 at seedling stage was set as ‘1’. ImageJ was used to measure the relative band intensity. ‘S’ represents seedling stage; ‘M’ represents maturity stage. (C) The relative expression level of OsBAK1, OsBAK2, OsSERK3, and OsSERK4 in OE-OsBZR1. The expression level of ZH11 was set as ‘1’. Data are shown as means ±SD (n=3). Student’s t-test was used to calculate P-values (***P<0.001; ns, not significant). (D) Expression data for rice SERK genes with rice tissues and various developmental stages from microarray analysis, including seedling root, leaf, shoot apical meristem (SAM), various stages of panicle (P1–P4), and seed (http://bar.utoronto.ca/efprice/cgi-bin/efpWeb.cgi). (E) A putative working model for the regulation of OsBZR1 and OsBAK2 in rice BR signaling. In the presence of BRs, the BR negative regulator OsGSK3 is degraded via the 26S proteasome pathway thereby failing to phosphorylate OsBZR1. The dephosphorylated form of OsBZR1 is accumulated in nucleus, forming a feedback loop and inhibiting the expression of OsBAK2 to balance BR signaling. More importantly, OsBAK2 expression functions negatively for grain size, coordinating with other rice SERK genes in regulating grain size.
Discussion
OsBAK2 is a crucial component in rice BR signaling
In this article, we provide several pieces of evidence to support the view that OsBAK2 is a critical component of BR signaling and thereby promotes plant development. First, the T-DNA insertion mutant osbak2 displayed dwarf plant height and erect leaves compared with DJ (Fig. 1A, B; Supplementary Fig. S2A, B). In contrast, OsBAK2 overexpression transgenic plants showed an enhanced BR-signaling phenotype, as the leaf angle restored was to that of the ZH11 phenotype (Supplementary Fig. S7A, B). Second, the T-DNA insertion mutant osbak2 was insensitive to BL treatment in a lamina joint bending assay and coleoptile elongation, while OsBAK2 overexpression plants exhibited more sensitivity to BL with larger leaf angle and more coleoptile elongation compared with ZH11 (Figs 1F–I, 6). The coleoptile elongation and inhibition of root length in osbak2 and OsBAK2-edited lines were insensitive to various concentrations of BL, but OE-OsBAK2 had an increased sensitivity to exogenous BL (Fig. 2; Supplementary Fig. S5). The exogenous BRZ treatment assay showed that BRZ strongly inhibited the growth of osbak2 and cr-osbak2 at the same BRZ concentration, but seedling length and the first internode length were significantly less reduced in OE-OsBAK2 than in ZH11 (Fig. 2; Supplementary Fig. S5). Compared with the wild type, OsBAK2 loss-of-function mutants exhibited insensitivity to BL and high sensitivity to BRZ. Third, transcription expression level of BR biosynthetic genes OsDWF4, OsD2 and OsD11 was greatly up-regulated in osbak2 mutant compared with DJ, and the transcript expression was decreased with BL treatment in DJ but obviously increased in osbak2 mutant (Supplementary Fig. S3A–D). In addition, the transcript levels of BR biosynthetic genes were higher in OsBAK2-edited lines but lower in OE-OsBAK2 lines than those in ZH11 (Fig. 7A). Meanwhile, the expression levels of BR catabolic genes CYP734A2, CYP734A4, CYP734A6 were down-regulated in osbak2 compared with DJ (Supplementary Fig. S3E–G). Fourth, OsBZR1 protein accumulation in defective OsBAK2 mutants was extremely reduced compared with ZH11 (Fig. 7E). In combination, these results suggested that OsBAK2 plays a positive role in the BR signaling pathway and BR-mediated plant development.
OsBAK2 and OsBZR1 engage in a feedback loop regulation
The ChIP-chip and expression microarray data demonstrated that BZR1 represses some BR biosynthetic genes and BRI1 through negative feedback regulation (Sun et al., 2010). Identification of BES1 target genes in the Arabidopsis genome indicated that a large proportion of them are BR-regulated genes participating in multiple biological processes. BES1 not only represses BR signaling component BRI1 but also represses its homologous genes BEH1 and BEH2. Based on ChIP-chip data of bes1-D seedlings, BAK2 is a target gene that is down-regulated in bes1-D (Yu et al., 2011). In this study, we noticed that the OsBAK2 promoter contains OsBZR1 binding cis-element BRRE and G-box, and is repressed by OsBZR1 as a direct target gene. We performed a yeast one-hybrid assay and ChIP-qPCR using GFP-OsBZR1 transgenic plants to confirm this (Fig. 3). More importantly, we indicated that the relative LUC activity of the OsBAK2 promoter was repressed by OsBZR1 with transient transactivation assays in rice protoplasts, and in parallel, exogenous BL treatment strengthened this suppression. However, co-incubation with OsGSK3 decreased the repression (Fig. 4B). EMSA was performed to prove the results that purified protein OsBZR1 binds to both the BRRE and the G-box sequences in the OsBAK2 promoter (Fig. 4D, E). Moreover, the phosphorylation of OsBZR1 by OsGSK3 greatly reduced its ability to bind to two probes containing the OsBAK2 promoter sequence (Fig. 4D, E). Previous studies showed that the BRRE and G-box elements are more enriched in BR-repressed targets, and the E-box motif is more enriched in BR-induced genes (Sun et al., 2010). Combined with the RT-qPCR results, we speculate that OsBZR1 could bind both the BRRE and G-box motifs to inhibit the transcription of OsBAK2 (Fig. 7B). These studies provide a novel molecular model in which OsBZR1 represses OsBAK2 expression thereby attenuating BR responses in a feedback inhibition loop, but the binding ability can be prevented by OsGSK3 phosphorylation.
Roles of OsBAK2 and OsBZR1 in modulating BR signaling and grain length
In previous studies, cr-osbzr1 transgenic plants displayed a BR-deficient phenotype. To analyse the genetic relationship between OsBAK2 and OsBZR1, we generated cr-osbak2/osbzr1 plants using CRISPR/Cas9 technology. cr-osbak2 and cr-osbzr1 showed erect leaves and shortened second internode, which were similar to cr-osbak2/osbzr1 (Supplementary Fig. S7). Furthermore, the leaf blade and coleoptile length of cr-osbak2/osbzr1 after exogenous BL treatment exhibited the same insensitivity compared with both cr-osbak2 and cr-osbzr1 transgenic lines (Fig. 6). We also found that cr-osbak2/cr-osbzr1 plants had a greatly higher transcript level of OsDWF4, OsD2, and OsD11, suggesting that defective OsBAK2 and OsBZR1 results in a BR-insensitivity phenotype (Fig. 7A). Western blot analysis demonstrated that OsBZR1 protein level was significantly increased in OE-OsBAK2, and the protein accumulation was gradually increased after a time course of BL treatment. The results suggested that OsBAK2 positively modulates OsBZR1 protein accumulation (Fig. 7E, F). Collectively, we provide insights into mechanisms for the involvement of OsBAK2 in an OsBZR1-mediated BR pathway. OsBZR1 expression negatively regulates OsBAK2 expression; the two genes are not in a linear but rather a circular relationship.
In grain length regulation, the T-DNA mutants osbak2 and cr-osbak2 displayed significantly larger grain length compared with wild type (Figs 1B, 5B), although osbak1 (08SG2) mutant showed reduced grain length and grain width (Yuan et al., 2017). The grain length of the double mutant was similar to that of cr-osbak2 with longer grain size compared with that of ZH11, partially restoring the short grain phenotype of cr-osbzr1, while OE-OsBAK2 displayed a decreased grain length (Fig. 5B, D). The results implied that OsBAK2 and OsBZR1 act simultaneously to regulate grain length, and manipulation of OsBAK2 can be used in rice breeding to optimize grain length and provides new insights into the molecular basis of grain length regulation.
Previous studies have shown that SERK1 and SERK2 (BAK2) proteins play redundant roles in tapetum and pollen development; the serk1/serk2 double mutant showed a male-sterile phenotype (Colcombet et al., 2005). The SERK family have a partially overlapping function in BR signaling; the double mutants between SERK3 (BAK1) and other SERKs showed decreased BR sensitivity after exogenous BR treatment generated only in serk1/serk3. 35S:bes1-D rescues the BR-deficient phenotype of serk1/serk3 but not serk1/serk2 and serk3/serk4. SERK genes involved in BRI1-mediated BR signaling, pathogen response, and other independent signaling pathway are only partially redundant and preserve conserved functions (Albrecht et al., 2008). SERK genes contribute unequally to stomatal patterning, and SERK2 plays a more important role than SERK1 and SERK4 (Meng et al., 2015). The different SERKs also make a different contribution in plant innate immunity; SERK1 and SERK2 form ligand-induced complexes with FLS2, but the affinity is much lower than that of SERK3 (Roux et al., 2011). In contrast to SERK3 and SERK4, SERK2 plays a lesser role in BR signaling. Exogenous BRs cannot enhance the interaction between BRI1 and SERK2, but the phosphorylation level of SERK2 is increased (Gou et al., 2012). Based on the rice expression database, the expression levels of OsBAK2 in numerous tissues and developmental stages are quite different from those of OsBAK1. In particular, OsBAK2 expression was lower than other SERKs during various stages of panicle development, indicating a possible role in gametophyte development and pollen function (Fig. 8D). According to the binding site prediction results (http://plantregmap.gao-lab.org/), no OsBZR1 binding sites, including BRRE motif, G-box motif, and E-box motif, were predicted in the promoter of OsBAK1, OsSERK3, and OsSERK4. Transcript and protein expression of OsBZR1 level are suppressed sharply at maturity, and we assumed OsBZR1 might be involved in the early events of rice development to promote plant growth (Fig. 8A, B). Since both OsBAK2 and OsBZR1 function positively in BR signaling, grain length of cr-osbzr1 was shorter than that of ZH11, but cr-osbak2 and osbak2 mutants showed larger grain length than wild type (Figs 1B, 5B). We speculate that the lack of OsBAK2 function repressed the accumulation of OsBZR1, resulting in a negative feedback effect on BR synthesis genes in BR signaling, finally leading to a longer grain length. Moreover, we also suppose that OsBAK2 positively modulates BR signaling, whereas OsBZR1 represses expression of OsBAK2 to form a regulatory loop in BR homeostasis, and both OsBZR1 and OsBAK2 are involved in the regulation of grain size. Deficiency of OsBAK2 leads to the down-regulation of BR levels, but other SERK genes may function to balance BR signaling and modify the grain size. Further studies on additional mechanisms of rice grain size formation mediated by OsBAK2 and other rice SERK genes are necessary.
Based on our results, we provide a novel model to illustrate how OsBAK2 participates in BR signal transduction in rice (Fig. 8E). As a positive regulator of BR signaling, OsBAK2 forms a heterodimer with OsBRI1 after perceiving BRs at the plasma membrane to reciprocally phosphorylate and transmit the BR signal to transcription factor OsBZR1. When the BR signal is enhanced, OsBZR1 represses OsBAK2 in rice by binding to the BRRE and G-box motif in OsBAK2 promoter to give negative feedback and inhibit BR transduction. This repression is especially pronounced at the seedling stage, where OsBAK2 inhibition by OsBZR1 decreased sharply after rice filling, resulting in a significant increase in OsBAK2 expression levels. However, under conditions of weak BR signal, the repression could be inhibited with the phosphorylation of OsBZR1 by activated OsGSK3. In parallel, OsBAK2 negatively regulates grain length. Our results support an innovative mechanism of a BR–OsBAK2–OsBZR1–OsBAK2 pathway in the regulation of rice growth and development, enhanced understanding of the regulation of grain length greatly. This has significant implications for the genetic improvement of crops.
Supplementary data
The following supplementary data are available at JXB online.
Fig. S1. Confirmation of T-DNA insertion mutant osbak2.
Fig. S2. Phenotypes of osbak2 mutant in response to BR.
Fig. S3. Transcript level alterations of BR biosynthesis and catabolic genes in osbak2 mutant under BR treatment.
Fig. S4. Diagram of the structure of cr-osbak2 generated by CRISPR/Cas9 system.
Fig. S5. BL and BRZ sensitivity assays of OsBAK2 transgenic plants.
Fig. S6. Phenotypic analysis of OsBAK2 generated by CRISPR/Cas9 in NJ9108 background.
Fig. S7. Leaf blade and the second internode length of OsBAK2 and OsBZR1 transgenic plants.
Table. S1. Primers used in this study.
Acknowledgements
We thank Prof. Wenli Zhang from Nanjing Agricultural University for the ChIP-qPCR analysis.
Author contributions
JH, HD, and XG designed the research, interpreted data, and wrote the manuscript. HD performed most of experiments and data analysis. RY, GC, JZ, RW, JL, and YW, helped to perform the experiments. JH, XG, and HZ supervised the work.
Conflict of interest
No conflict of interest declared.
Funding
This work was supported by the Natural Science Foundation of China (32071918, 32000227), Jiangsu Province Government (BE2022336), the Seed Industry Revitalization Project of Jiangsu Province (JBGS [2021]009), Cyrus Tang Innovation Center for Crop Seed Industry, and the Jiangsu Collaborative Innovation Center for Modern Crop Production.
Data availability
Data that support the findings of this study are available in the supplementary data that accompanies this article.
Comments