-
PDF
- Split View
-
Views
-
Cite
Cite
Lanqin Xia, Youzhi Ma, Yi He, Huw D. Jones, GM wheat development in China: current status and challenges to commercialization, Journal of Experimental Botany, Volume 63, Issue 5, March 2012, Pages 1785–1790, https://doi.org/10.1093/jxb/err342
- Share Icon Share
Abstract
Genetic modification facilitates research into fundamental questions of plant functional genomics and provides a route for developing novel commercial varieties. In 2008, significant financial resources were supplied by the Chinese government for research and development (R&D) into genetic modification of the major crop species. This project was aimed at providing an opportunity for crop improvement while accentuating the development of a safe, precise, and effective wheat genetic transformation system suitable for commercialization. The focus here is on one of the key crops included in this project, wheat, to provide an insight into the main transformation methods currently in use, the target traits of major importance, and the successful applications of wheat genetic improvement in China. Furthermore, the biosafety and regulatory issues of major concern and the strategies to produce ‘clean’ transgenic wheat plants will also be discussed. This commentary is intended to be a helpful insight into the production and commercialization of transgenic wheat in China and to put these activities into a global context.
Introduction
Wheat (Triticum aestivum L.) is one of the major staple food crops grown worldwide on more than 17% of the cultivatable land and produced in a wide range of climatic environments and geographic regions. During the past four decades, wheat has made a significant contribution to the increase in global food production as total production rose steadily through the use of higher yielding, water- and fertilizer-responsive, and disease-resistant varieties supported by a strengthened input system, tailored management practices, and improved marketing (Dixon et al., 2009). In 2010, wheat was the second most important cereal crop after maize, with global production of 653 million metric tonnes. Despite generally increasing global production trends, the 2010 harvest was 21 million tonnes lower than the previous year, mainly due to a severe drought in Australia and a major fire in Russia (www.ers.usda.gov/data/wheat) and reminds us of the fragility of crop harvests that can be dependent on environmental factors largely out of our control. China is the top producer of wheat globally with production reaching 112 million metric tonnes in 2010, even though within China, it is only the third most cultivated crop after maize and rice. Wheat production in China, like that in the rest of the world, is under pressure from changing climates and the increasing financial and environmental costs associated with chemical inputs. Present attempts to raise the yield and qualities of wheat through conventional breeding in China are being confronted by slow progress in the genetic improvement of yield potential and stability. Although conventional breeding programmes have led to continuous improvements in some aspects, outbreaks of drought, and fungal and insect pests causing substantial losses are being reported regularly. For example, nearly 80% of Chinese wheat is planted in areas suffering drought or semi-drought conditions (Huang, 1996). In 2009 and 2010, two-thirds of the 26 million hectares of the Chinese wheat growing area suffered severe drought, especially north-western China and in areas where irrigation was limited or not available. Also in 2010, 62.5% of this area was affected by severe aphid infestations. Thus, there is an urgent need to mitigate the effects of biotic and abiotic stresses on wheat yield through the use of tolerant or resistant varieties which could be financially and environmentally beneficial. One way to produce these resistant varieties is via genetic modification and GM wheat opens up new opportunities to meet these challenges.
Plant biotechnology has made significant advancements during the past decade, and offers the opportunities to make step-change increases in crop yield by avoiding losses due to disease, pests, and adverse abiotic conditions such as drought, salinity, heat, etc, in addition to major advances in novel desirable quality characteristics which are unlikely to be achieved by conventional breeding methods. Remarkably, in 2010, the accumulated global area of GM crops planted during the 15 years from 1996 to 2010 exceeded, for the first time, 1 billion hectares. Engineered traits such as herbicide tolerance and insect resistance have resulted in a significant reduction in herbicide and insecticide use (James, 2010).
China started the research and development (R&D) initiative on GM crops early in 1999, but, initially, only a few crops and target traits were involved. In 2008, the Chinese government invested significant financial support to accelerate this R&D project on GM crops including cotton, maize, rice, wheat, and soybean etc. with the aim of improving a range of target traits. In addition to providing an opportunity for crop improvement through genetic engineering, this project also allowed the development of a biosafe, precise, and effective genetic transformation system suitable for commercialization. Under the support of these projects, Chinese varieties of GM cotton for insect resistance have been developed, commercialized and are now planted in most cotton-growing area in China, resulting in a significant reduction in insecticide use. At the same time, after a long development and regulatory process, GM rice and maize for insect resistance and improved phytase activity were also authorized to be commercially grown (http://www.moa.gov.cn/ztzl/zjyqwgz/zswd/201107/U020110705523604929127.doc) although significant commercial planting of these varieties has not been reported so far.
Despite its global importance, wheat was the last major cereal to be genetically transformed due to its recalcitrance in tissue culture and genotype-dependence on foreign DNA-transfer by Agrobacterium (Jones, 2005; Bhalla, 2006). The commercialization of transgenic wheat has been problematic because of concerns from farmers, trade organizations, and even governments about market acceptance (Fox, 2009). However, since 2009, agribusiness has reconsidered the opportunities for transgenic wheat, anticipating the commercialization of GM wheat within a decade. For example, Monsanto indicated their renewed interest in the GM wheat business by acquiring the seed businesses WestBred in the USA in 2009 and a 20% stake in InterGrain Australia in 2010. Also in 2010, Syngenta announced a strategic alliance with CIMMYT (Centro Internacional de Mejoramiento de Maíz y Trigo) based in Mexico and, in the same year, Bayer CropScience of Germany formed an alliance with the Commonwealth Scientific and Industrial Research Organization (CSIRO) in Australia, promising to bring solutions to wheat growers as early as 2015 in improvement of drought, yield, and phosphorus absorption.
In recent years, Chinese scientists have demonstrated significant advances in wheat transformation technology development and genetic engineering for the improvement of traits such as drought tolerance, resistance to pests and diseases, specific aspects of grain quality etc. A few projects have already led to field-trial applications. A short commentary on GM wheat development in China is presented here, addressing the current status, future perspectives, and challenges to commercialization.
Wheat genetic engineering in China
In common with widely-used methods elsewhere, wheat transformation in China utilized mainly micro particle bombardment which is relatively genotype-independent and is able to introduce DNA directly into various tissues for transient gene expression studies (Rogers, 1991; Altpeter et al., 1996; Vasil, 2007). Now this method is routine in a lot of laboratories in China and the transformation efficiency can reach as high as 10%. However, since it was first reported by Cheng et al. (1997), there is a general perception that Agrobacterium-mediated transformation of wheat is a better option, particular when regulatory approval for field trials is required, because it has several advantages including the ability to transfer large segments of DNA with minimal rearrangement, a lower transgene copy number with few events of gene silencing and, in cereals, the predisposition to integrate into transcriptional active regions of the genome and improved stability of expression over generations (Hiei et al., 1997; Hansen and Wright, 1999; Dai et al., 2001; Wu et al., 2003; Jones et al., 2005; He et al. 2010).
There has been significant progress in methodology development in Agrobacterium-mediated transformation of wheat in Chinese laboratories with the transformation efficiency ranging from 0.5–6.3%, but it is still confined mainly to a few responsive varieties (He et al., 2010). Current publications on methodology development of Agrobacterium-mediated wheat transformation in China are listed in Table 1. In most cases, the starting materials were immature embryos or embryogenic callus, but in the Chinese Academy of Agricultural Sciences (CAAS) there is a growing interest in developing transformation protocols and methods using mature seeds rather than the immature embryo as the starting explants.
Current status of methodology development for wheat Agrobacterium-mediated transformation in China
Wheat genotype | Explant | Transformation efficiency | Selection marker gene | Reporter gene | References |
Jinan177 | Pre-cultured immature embryo, immature embryo | 3.7–5.9% | NptII | Xia et al., 1999 | |
Bobwhite | Pre-cultured immature embryo | 0.82% | NptII | NptII | Ye et al., 2001 |
Nongda170, Nongda146 | Immature embryo,embryogenesis callus | 2.1% | bar | GUS | Wang et al., 2002 |
Taishan021, Lumai21, Huixianghong, 971942, 980293 | Floret of wheat | 2–3.2% | NptII | NptII | He et al., 2003 |
Xinchun 9, PM97034 Bobwhite | Immature embryo | 1.1–3.3% | NptII | GUS | Ye et al., 2005 |
EM12 | Mature embryo | 0–2.5% | bar | GUS | Ding et al., 2009 |
Stewart | Immature embryo | 2.8–6.3% | bar | GUS | He et al., 2010 |
Wheat genotype | Explant | Transformation efficiency | Selection marker gene | Reporter gene | References |
Jinan177 | Pre-cultured immature embryo, immature embryo | 3.7–5.9% | NptII | Xia et al., 1999 | |
Bobwhite | Pre-cultured immature embryo | 0.82% | NptII | NptII | Ye et al., 2001 |
Nongda170, Nongda146 | Immature embryo,embryogenesis callus | 2.1% | bar | GUS | Wang et al., 2002 |
Taishan021, Lumai21, Huixianghong, 971942, 980293 | Floret of wheat | 2–3.2% | NptII | NptII | He et al., 2003 |
Xinchun 9, PM97034 Bobwhite | Immature embryo | 1.1–3.3% | NptII | GUS | Ye et al., 2005 |
EM12 | Mature embryo | 0–2.5% | bar | GUS | Ding et al., 2009 |
Stewart | Immature embryo | 2.8–6.3% | bar | GUS | He et al., 2010 |
Current status of methodology development for wheat Agrobacterium-mediated transformation in China
Wheat genotype | Explant | Transformation efficiency | Selection marker gene | Reporter gene | References |
Jinan177 | Pre-cultured immature embryo, immature embryo | 3.7–5.9% | NptII | Xia et al., 1999 | |
Bobwhite | Pre-cultured immature embryo | 0.82% | NptII | NptII | Ye et al., 2001 |
Nongda170, Nongda146 | Immature embryo,embryogenesis callus | 2.1% | bar | GUS | Wang et al., 2002 |
Taishan021, Lumai21, Huixianghong, 971942, 980293 | Floret of wheat | 2–3.2% | NptII | NptII | He et al., 2003 |
Xinchun 9, PM97034 Bobwhite | Immature embryo | 1.1–3.3% | NptII | GUS | Ye et al., 2005 |
EM12 | Mature embryo | 0–2.5% | bar | GUS | Ding et al., 2009 |
Stewart | Immature embryo | 2.8–6.3% | bar | GUS | He et al., 2010 |
Wheat genotype | Explant | Transformation efficiency | Selection marker gene | Reporter gene | References |
Jinan177 | Pre-cultured immature embryo, immature embryo | 3.7–5.9% | NptII | Xia et al., 1999 | |
Bobwhite | Pre-cultured immature embryo | 0.82% | NptII | NptII | Ye et al., 2001 |
Nongda170, Nongda146 | Immature embryo,embryogenesis callus | 2.1% | bar | GUS | Wang et al., 2002 |
Taishan021, Lumai21, Huixianghong, 971942, 980293 | Floret of wheat | 2–3.2% | NptII | NptII | He et al., 2003 |
Xinchun 9, PM97034 Bobwhite | Immature embryo | 1.1–3.3% | NptII | GUS | Ye et al., 2005 |
EM12 | Mature embryo | 0–2.5% | bar | GUS | Ding et al., 2009 |
Stewart | Immature embryo | 2.8–6.3% | bar | GUS | He et al., 2010 |
A scan of the current literature published both in international and Chinese peer-reviewed journals showed that GM wheat research has contributed to the improvement of a wide variety of agronomic traits including resistance to biotic and abiotic stresses, improved bread-making and nutritional qualities, increased yield, etc., with almost 40% of the papers focused on disease resistance, 25% on quality improvement followed by abiotic stresses, pest resistance, and yield (Fig. 1) (Yu et al., 2010). From 1994 to 2009, USA alone released 433 field trials of transgenic wheat, among which 44.6% were herbicide resistance, 26.8, 15.0, and 12.2% were related to disease resistance, quality, and yield improvement, respectively (The USDA APHIS data, accessed through The Information System for Biotechnology (ISB) website: http://www.nbiap.vt.edu).
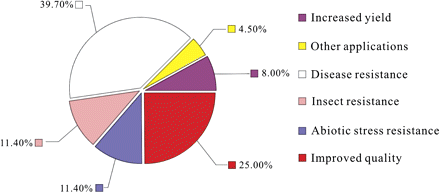
Currently reported wheat traits improved by genetic modification. A literature search using the terms ‘wheat and transgenic or transformed or transformation’ was performed and the publications identified were classified according to trait investigated. Figure adapted from Yu et al (2010) by kind permission of the Chinese Electronic Periodical Services.
In this aspect, China lagged far behind the USA, from 1997 to 2009, only 62 cases of transgenic wheat entered into the regulatory process including 21 for disease resistance, with other traits, including pest resistance, drought resistance, quality improvement, and others having 9, 18, 10, and 4 applications, respectively. Of these, 44 cases were in the bio-evaluation phase in the greenhouse and, while14 were authorized for environmental release, only four were actually trialled in the field and possessed traits such as disease resistance to yellow mosaic virus, drought and pre-harvest sprouting resistance, and dough strength for quality improvement. The insertion (via biolistics) of the antisense sequence of the yellow mosaic virus coat protein by wheat researchers in CAAS resulted in good field performance of resistance to yellow mosaic virus (Ma, 2009). Furthermore, by the end of 2010, a total of 105 gene sequences or technologies used for wheat genetic engineering had patents pending in China. Among them, 73 were related to abiotic stresses such as drought, cold, heat, etc, 12 were disease-resistance genes which mainly involved key genes in signalling pathways, 12 were genes related to wheat dough strength and grain texture, four were insect resistance genes such as the plant lectin genes gna, pta, and the EβF synthase genes, and five were involved in the improvement of genetic transformation technologies per se (www.cnpatent.com). Furthermore, among the 33 papers on GM wheat published by Chinese researchers so far, 19 concerned disease resistance, including five on resistance to wheat and barley yellow mosaic virus, seven on powdery mildew resistance, four on blight/scab resistance, two on sharp eyespot, and one for leaf rust resistance, whereas eight publications related to quality improvement such as improved dough strength, with two on grain texture and three on increased lysine and carotene content. The remaining eight papers related to abiotic stresses with five for drought resistance, one for salt tolerance, and two for wheat pre-harvest sprouting resistance (Yu et al., 2010).
As far as we can ascertain, most of the 62 cases of wheat transgenics submitted to the GMO regulatory authorities in China were generated by the biolistics method. Although each GM event is risk-assessed individually regardless of the mode of transformation, for experiments in contained environments and for small-scale research trials to demonstrate gene function where no material will enter the food or feed chains, less emphasis is placed on the precise molecular insertion patterns and biolistic methods are deemed appropriate. However, the full commercialization of selected gene constructions would require the generation of new events with simple, low copy number, ‘clean’ insertions with little or no redundant sequences or marker genes, and demonstrated stability of the insert over several generations. In addition, a thorough assessment would need to be carried out on the potential risks posed by the plant to human health and the environment.
Challenges
Following the recent activities of companies such as Monsanto, Syngenta, and Bayer CropScience, GM wheat appears to be back on the agribusiness agenda, at least in the major biotech crop-growing regions of the world. Although China has made some progress in GM wheat in recent years, it faces the challenge of increasing wheat yield stability to feed its large population under a changing climate and increasing pressure on agricultural land. Technical hurdles also remain in aspects such as the establishment of a high throughput wheat transformation platform, the development of elite wheat varieties without deleterious selection marker genes, and the introduction of a minimal backbone or no bacterial DNA sequences, enacting responsible, rigorous, and feasible regulatory systems and the issues involving intellectual property rights and social and market acceptance of GM wheat products.
Establishment of a precise, marker-free, and high throughput wheat transformation platform is essential
The potential for employing Agrobacterium-mediated transformation methods to achieve candidate commercial lines has been well documented, although additional tests are needed to demonstrate that no vector backbone had been inserted, especially the sequence near the left border of T-DNA (Hanson et al., 1999; Shibata and Liu, 2000; Rommens, 2004; Wu et al., 2006). To address this, the development of suitable vectors for Agrobacterium-mediated transformation is essential. The pCLEAN vectors developed by Dr Phillip Vain’s group at the John Innes Centre, UK, could serve as a model to eliminate both the redundant sequences and the selectable marker genes. The pCLEAN vectors are based on the widely used pGreen/pSoup system (Hellens et al., 2000), and have been designed to enable the delivery of multiple transgenes from distinct T-DNAs and/or vector backbone sequences while minimizing the insertion of superfluous DNA sequences into the plant nuclear genome as well as facilitating the production of marker-free plants. Compared with other vectors currently in use, pCLEAN vectors contain a minimal T-DNA (102 nucleotides) consisting of direct border repeats surrounding a 52-nucleotide-long multiple cloning site, an optimized left-border sequence, a double left-border sequence, restriction sites outside the borders, and two independent T-DNAs. In addition, selectable and/or reporter genes have been inserted into the vector backbone sequence to allow either the counter-screening of backbone transfer or its exploitation for the production of marker-free plants. Analysis of stably transformed tobacco and rice plants demonstrated that the pCLEAN dual binary vector system enabled the efficient delivery of a range of DNA fragments from single to multiple T-DNAs without vector backbone sequences and selection markers into the nuclear genome of mono- and dicotyledonous species (Thole et al., 2007). Researchers in CAAS have further developed the pCLEAN vector series to give a more robust gateway system and with additional left borders. Testing the efficacy of this vector system in wheat is underway. Successful application of the pCLEAN vector series in wheat could establish a versatile platform that can contribute to the further development of precise wheat transformation technologies.
Furthermore, in addition to the utilization of immature embryos as starting material, considerable efforts have also been devoted to the development and optimization of Agrobacterium-mediated transformation of both mature embryos and of germ line cells in the shoot meristem with some success (Zhao et al., 2006; Ding et al., 2009). The use of mature embryos as starting material presents a low-cost and time-saving alternative to explants from immature plants, especially for most Chinese laboratories which lack easy access to the contained environment facilities to grow wheat donor plants continually all year round. However, these protocols need to be further refined to be reproducible and efficient, and this is a goal for the next few years.
The selection marker genes currently utilized in wheat genetic transformation in China
To date, most of the commercialized GM crop varieties contain a selection marker in addition to the gene of interest. The plant selectable marker genes currently used in Chinese laboratories are: bar gene for glufosinate or PPT resistance, 5-enolpyruvylshikimate-3-phosphate synthesase gene (epsps) for glyphosate resistance, and the phosphomannose isomerase gene (pmi) for mannose selection (Zhou et al., 1995; Hu et al., 2003; Gadaleta et al. 2006). For epsps and pmi genes, use of these genes in commercializing GM wheat will be complicated by licensing issues since these two genes are covered by international patents with Monsanto and Syngenta, respectively. Although some novel epsps genes were isolated in Professor Min Lin’s laboratory at the Institute of Biotechnology Research, CAAS and in Professor Zhicheng Shen’s laboratory in Zhejiang University in China who successfully demonstrated their efficacy in a range of GM crops including cotton, maize, and rice, the utilization of these novel epsps genes in wheat genetic transformation is underway both in our laboratory and in Professor Jialin Yu’s laboratory in the China Agriculture University.
It is also worth highlighting here that the Chinese National Biosafety Committee of Agricultural GMOs (CNBCAG) has given guidance that the only acceptable selectable marker genes in commercial GM wheat would be epsps and pmi. Even the bar gene which is useful for conferring herbicide resistance was ruled out of any field applications. However, this gene has been widely used as a selection marker for the transformation of wheat and to provide tolerance to the non-selective, broad-spectrum, post-emergent, contact herbicides commonly known as Basta, Bialaphos, Herbiace, and Glufosinate in commercial GM crops such as soybean and canola which are currently widely grown in USA or other countries (Vasil et al., 1993; Weeks et al., 1993; Jones et al., 2005; He et al., 2010; James, 2010). Therefore, it would be valuable for the policy makers to re-evaluate the situation for the successful application of this gene in wheat.
The development of transgene stacking technology in wheat transformation
So far, most GM wheat lines developed by Chinese researchers focused on single gene traits that, in some cases, have been pyramided by backcrossing. The drawback of this approach is that, as the number of transgenic loci increase, breeding becomes more difficult because increasingly larger populations are needed to find individuals with the desired assortment of transgenic loci along with the non-transgenic elite traits. Crop varieties possessing stacked events are becoming an important commercial trend (Ow, 2001; Moon et al. 2011) with future stacked products comprising both agronomic input traits for pest resistance, tolerance to herbicides and drought, plus output traits such as high omega-3 oil in soybean or enhanced pro-Vitamin A in Golden Rice (James, 2010). In the long run, if many genes, especially the genes involved in metabolite pathways, need to be manipulated into wheat at the same time, intelligent gene stacking strategies utilizing large T-DNAs possessing several expression cassettes need to be employed. To date, several laboratories in China are majoring in this research such as Professor David Ow’s laboratory at the South China Botanical Garden, Chinese Academy of Sciences (CAS) and laboratories in the Chinese Academy of Agricultural Sciences (CAAS).
The regulatory system and social acceptance of GM crops in China
Prior to commercialization, all new GM crop varieties must go through a safety assessment process. China established a strict regulatory system for the risk assessment of GM plants in 1996, and worked alongside the Organization of Economic Cooperation of Development (OECD) and United Nations Environment Programme (UNEP) to make continuous improvements to this regulatory process. Guidelines for biosafety assessment are now largely harmonized with those in the EU and USA. Generally, GM crops in China have to pass through five phases of evaluation involving a proof of concept in the laboratory, the bio-evaluation in the greenhouse, release to the confined environment (small-scale field test), and large-scale test in an open field and, finally, authorization for field applications (Agricultural Genetically Modified Organism, Biosafety Evaluation and Management Measures, http://2010jiuban.agri.gov.cn/). Each step has its strict requirements following the same criteria as those of other countries. For example, before applying for environmental release, the applicant needs to provide the data on the gene function, genetic and expression stability of the insert, and of the precise position of integration of transgenes in the wheat genome, evidence of a change in allergy or toxicity of the newly created variety, and potential for out-crossing/pollination with other organism or related species (http://2010jiuban.agri.gov.cn/). It is expected that this process will take at least 10 years for GM wheat to go from the laboratory to field production. Therefore, although Chinese wheat researchers are working to develop GM wheat with improved traits as rapidly as possible, so far, no GM wheat has been approved for commercialization, and the first release of GM wheat for improved yellow mosaic resistance is expected as early as 2015 depending on social and public acceptance (Ma, 2009).
In general, the adoption of GM crops by Chinese farmers has been rapid, for example, since it was commercialized in 1998, the area planted with insect-resistant GM cotton has increased year on year and reached 3.6 million hectares in 2010, representing 75% of the total cotton-planting area in China (http://www.moa.gov.cn/ztzl/zjyqwgz/zswd/201107/U020110705523604929127.doc). Furthermore, in 2010 alone, China imported 54 million tons of soybeans from the USA, Argentina, and Brazil (Chinese National Agro-tech Extension and Service Centre, http://en.natesc.gov.cn/sites/en/), where most of their soybean cultivars were herbicide-resistant GMOs. Over 75% of the soybean products such as Tofu, soybean juice, soy sauce, edible oil etc. consumed by people in China as part of their normal diet are made of imported GM soybeans. The fact that foods containing GMOs are labelled in supermarkets indicate an increasing public awareness of GM products in China and the authors can find no evidence of a decline in consumption of soy-containing products. Furthermore, following the biosafety certificates issued to the phytic acid gene transgenic maize ‘BVLA430101’ developed by Professor Yunliu Fan’s laboratory at the Institute of Biotechnology Research, CAAS and transgenic insect-resistant rice, ‘Hua Hui 1’ and hybrid ‘Bt Shanyou 63’ generated by Professor Qifa Zhang’s laboratory in Huazhong Agricultural University, the increasing hectarage and acceptance of GM crops appears to be gaining momentum in China, providing a broad and stable foundation for future growth of GM wheat both in China and the rest of the world.
Some of work mentioned in this paper is supported by the Research Initiatives on Development of Transgenic Wheat with Improved Traits (2008ZX08010-003, 2008ZX08002-001) funded by the Chinese Ministry of Agriculture and National Nature Science Foundation (31171618). Research performed at Rothamsted Research was funded by the Biotechnology and Biological Sciences Research Council (BBSRC). Our thanks also go to Dr Phillip Vain at the John Innes Centre for kindly providing the pCLEAN basic vector series.
Comments