-
PDF
- Split View
-
Views
-
Cite
Cite
Yanan He, Wei Li, Jian Lv, Yuebin Jia, Mengcheng Wang, Guangmin Xia, Ectopic expression of a wheat MYB transcription factor gene, TaMYB73, improves salinity stress tolerance in Arabidopsis thaliana, Journal of Experimental Botany, Volume 63, Issue 3, February 2012, Pages 1511–1522, https://doi.org/10.1093/jxb/err389
- Share Icon Share
Abstract
MYB transcription factors (TFs) play pivotal roles in the abiotic stress response in plants, but their characteristics and functions in wheat (Triticum aestivum L.) have not been fully investigated. A novel wheat MYB TF gene, TaMYB73, is reported here based on the observation that its targeting probe showed the highest salinity-inducibility level among all probes annotated as MYB TFs in the cDNA microarray. TaMYB73 is a R2R3 type MYB protein with transactivation activity, and binds with types I, II, and IIG MYB binding motifs. The gene was induced by NaCl, dehydration, and several phytohormones, as well as some stress-, ABA-, and GA-responsive cis-elements present in its promoter region. Its over-expression in Arabidopsis enhanced the tolerance to NaCl as well as to LiCl and KCl, whereas it had no contribution to mannitol tolerance. The over-expression lines had superior germination ability under NaCl and ABA treatments. The expression of many stress signalling genes such as AtCBF3 and AtABF3, as well as downstream responsive genes such as AtRD29A and AtRD29B, was improved in these over-expression lines, and TaMYB73 can bind with promoter sequences of AtCBF3 and AtABF3. Taken together, it is suggested that TaMYB73, a novel MYB transcription factor gene, participates in salinity tolerance based on improved ionic resistance partly via the regulation of stress-responsive genes.
Introduction
Both grain yield and quality of wheat are downgraded by adverse environmental conditions, among which soil salinity, drought, and extreme temperatures cause the greatest economic losses. Thus, improving its level of abiotic stress tolerance has become a key objective in many wheat breeding programmes. Genetic variation for stress tolerance is rather limited in the domesticated gene pool, but a number of wild relatives of wheat have been proved to process notable high-level tolerance (Zamir, 1995); thus one approach to enhance stress tolerance genetically is to create alien gene introgression lines via somatic hybridization. The salinity-tolerant cultivar Shanrong No. 3 (SR3) was obtained in this way; it was selected among the derivatives of a somatic hybrid between the conventional, salinity-sensitive bread wheat cultivar and the highly tolerant wild species Thinopyrum ponticum (Peng et al., 2009). Cytogenetic and molecular analysis showed that a few chromatin fragments were introgressed into the genome of SR3 (Xia et al., 2003; Wang et al., 2005), and dramatic genomic variation arises, suggesting that SR3 is a genetic reservoir for salinity tolerance associated gene mining.
Stress tolerance in plants can be enhanced via both ABA-dependent and ABA-independent pathways (Barrero et al., 2006; Ergen et al., 2009). In the former, AREB/ABF transcription factors are thought to play an important role while, in the latter, DREB/CBF transcription factors are heavily responsible for signal transduction. The Arabidopsis genome contains >1600 transcription factors, equivalent to ∼6% of the total number of genes present, of which the MYB family has more members than any other (Riechmann et al., 2000). MYB proteins are recognized by their DNA-binding domains, comprising one to four imperfect repeats, each of which form a helix-turn-helix structure of ∼50 residues (Dubos et al., 2010). As a prototype, three repeats of c-Myb are referred to as R1, R2, and R3, and repeats from other MYB proteins are named according to their similarity to R1, R2, or R3. Four MYB subfamilies have been recognized according the repeats that they possess: MYB-related (containing a single or partial R1, R2, or R3 domain), R2R3, R1R2R3, and 4R (containing four R1 and/or R2 domains) (Dubos et al., 2010). MYB proteins were found to be implicated in various developmental and physiological processes, especially in abiotic and biotic stress responses (Dubos et al., 2010). For instance, AtMYB2 functions in the ABA-mediated drought-stress response (Abe et al., 2003); Over-expression of AtMYB44 can reduce the rate of water loss and improve drought and salinity tolerance (Jung et al., 2008); Arabidopsis lines engineered to over-express AtMYB96 are more drought-tolerant than the wild type (Seo et al., 2009); AtMYB15 accounts for tolerance to drought and salt stresses (Agarwal et al., 2006; Ding et al., 2009); over-expression of OsMYB4 has been shown to increase the chilling and freezing tolerance of Arabidopsis (Vannini et al., 2004); OsMYB3R-2 contributes to tolerance to cold (Ma et al., 2009) and resistance to multiple abiotic stresses in Arabidopsis (Dai et al., 2007).
In recent years, the identification of wheat MYB TF genes and their roles in abiotic stress tolerance have been attempted. TaMYB10 was proved to be responsible for the coat pigmentation of red wheat grain (Himi et al., 2005). TaWRS2 has been shown to suppress the expression of KNOX, and hence affects lateral organ initiation and meristem differentiation (Morimoto et al., 2009). TaMYB1 is involved in the response to hypoxia (Lee et al., 2007). TaMyb2, TaMyb4, and TaMyb1 were elevated when treated with 30% PEG, but their function(s) have not been fully described to date (Chen et al., 2005). Ectopic expression of TaMYB2A confers enhanced tolerance to multiple abiotic stresses in Arabidopsis (Mao et al., 2011). TaMYBsdu1 has higher expression in salt-tolerant wheat cultivars than in salt-sensitive cultivars under saline and drought conditions, and is predicted to be involved in wheat adaptation to both salt and drought stresses (Rahaie et al., 2010). However, not much is known about MYB proteins in wheat, and more attempts for isolating MYB genes and characterizing their functions are needed.
In order to identify a MYB gene that possibly plays an important role in the abiotic stress response from wheat, a transcriptomic analysis of the wheat introgression line SR3 was performed using the wheat gene microarray, in which a set of probes annotated as MYB TF genes appeared to be either over- or under-expressed under salt stress (Wang et al., 2008). In this study, a new R2R3 type MYB gene, TaMYB73, for SR3 was cloned, whose referring probes showed the most salinity-induced pattern among all the MYB probes. It was found that TaMYB73 over-expression in Arabidopsis enhanced tolerance to salinity, while it had no positive effect on coping with osmotic stresses, suggesting that TaMYB73 may play special roles in response to ionic stresses.
Materials and methods
Wheat growing conditions and treatment
The wheat introgression line SR3 with high salt and drought tolerance was used as the wheat material in this work. Seedlings of SR3 were grown hydroponically in half-strength Hoagland’s solution under a 16/8 h light/dark, 22/20 °C, 60% humidity regime to the three-leaf stage and were then treated in half-strength Hoagland’s solution with or without 200 mM NaCl, 200 μM abscisic acid (ABA), 200 μM salicylic acid (SA), 200 μM 1-aminocyclopropane-1-carboxylic acid (ACC), 200 μM gibberellic acid (GA) or 200 μM jasmonic acid (JA) for 0–24 h. Dehydration stress was imposed by removing the seedlings from liquid culture and leaving them on a sheet of Whatman No. 5 filter paper in the dark at room temperature for various periods of time. All samples were selected at the same time to avoid the influence of photoperiod on gene expression. Mock analysis of NaCl and dehydration was carried out by leaving the seedlings in half-strength Hoagland’s medium, and that of phytohormone treatment by transferring the seedlings into half-strength Hoagland medium and adding an equivalent volume of the corresponding solution for solving these phytohormones. All of the treatments were repeated three times.
Cloning and sequence analysis
Based on the data of transcriptomic analysis of SR3 seedlings with or without salinity stress that was conducted using our customized 60-mer oligo-DNA microarray containing 15 172 probes (the probes harbouring 15 170 genes were designed according to the EST sequences either from the SR3 cDNA library or from the public wheat EST database), the expression pattern of probes annotated as wheat MYB genes (TaMYBs) under salinity/drought stress were fully analysed. Among them, one fragment, TaMYB73, was induced to the greatest extent under stress. To obtain the full-length cDNA of TaMYB73, its 5' segment was amplified from the SR3 cDNA library using the 3' terminal specific reverse primer, Ta.5405-As, in combination with the NT3 primer targeting the pBluescript (+) vector sequence at the 5' end of the inserts, and its 3' segment was amplified using the 5'-terminal specific forward primer, Ta.5405-S, and the NT7 primer targeting vector sequence at the 3' end of the inserts. Two segments were sequenced and assembled into a full-length cDNA. According to the assembled sequence, the TaMYB73 open reading frame (ORF) was cloned for further analysis. Its phylogeny was constructed from the peptide sequences of TaMYB73 and various heterologous MYB proteins using a Neighbor–Joining method within CLUSTAL X and MEGA3 software (Kumar et al., 2004; Larkin et al., 2007). SNAT domains of TaMYB73 were aligned with those of other MYBs using CLUSTAL X software (Larkin et al., 2007).
Promoter isolation and analysis
The promoter sequence was isolated using the site-finding PCR method (Tan et al., 2005) (the primers are listed in Supplementary Table S1 at JXB online). Promoter cis-elements were identified using the algorithm developed by Higo et al. (1999) and are available at www.dna.affrc.go.jp/PLACE/index.html.
RT-PCR and real-time PCR
Total RNA was extracted from both Arabidopsis and wheat seedlings with the Trizol reagent (Takara) and treated with RNase-free DNase (Promega). The cDNA first strand was synthesized using an M-MLV kit (Invitrogen), following the manufacturer's protocol. TaActin (AB181991) and AtActin (GI:145338402) were used as internal reference genes for assessing expression levels in wheat and Arabidopsis, respectively, and primers for these two genes are both specific to amplify a single gene. All RT-PCR experiments described in this section were reproduced at least three times using independent cDNA preparations. LightCycler® FastStart DNA Master SYBR Green I (Roche) was used for real-time PCR. Each 10 μl real-time PCR was subjected to a cycling regime of 94 °C for 10 s, 60 °C for 10 s, and 72 °C for 10 s. After the PCR program, data were quantified using the comparative CT method (2−ΔΔCT method) based on CT values (Livak and Schmittgen, 2001). Each RT-PCR was repeated four times, and each real-time PCR was run in triplicate. Primer sequences for RT-PCR and real-time PCR were listed in Supplementary Table S1 at JXB online.
Subcellular localization and transactivation assay of TaMYB73
The TaMYB73 sequence, lacking its stop codon, was PCR cloned (see Supplementary Table S1 at JXB online) from SR3 cDNA and ligated into the XbaI and BamHI sites of the 326-GFP vector (Lee et al., 2003). The positive construct or the 326-GFP vector alone was transformed into onion epidermal cells (Lee et al., 2003) and Arabidopsis mesophyll protoplasts (Yoo et al., 2007), respectively. After a period of 16 h at 22 °C in the dark, these cells were analysed by bright field and fluorescence microscopy. The full-length TaMYB73 ORF and a series of truncated ORF fragments were amplified using specific primers with EcoRI and PstI restriction digestion sites that flanked both terminals of each full-length/truncated sequence (see Supplementary Table S1 at JXB online), and these amplified sequences were inserted into the EcoRI and PstI sites of the pGBKT7 vector (Clontech). Each of the positive plasmids and an insert-less vector were transformed separately into the yeast AH109 strain (Clontech), according to the supplier’s protocol. Yeast colonies growing in SD-Trp medium were transferred to either SD-Trp-His-Ade or fresh SD-Trp medium after 2 d of culture at 30 °C. The β-galactosidase assay was conducted using O-nitrophenyl-β-D-galactopyranoside (ONPG) as the substrate following the manufacturer’s specifications (Clontech).
Yeast two-hybrid and one-hybrid assays
Among TaMYB73’s homologous proteins, AtMYB15 from Arabidopsis was found to bind to MYB binding cis-elements in the AtCBF3 promoter and to interact with AtICE1 (Zhu et al., 2007). In order to know whether TaMYB73 can bind to the promoter of AtCBF3 and interact with AtICE1 as well, a yeast one-hybrid assay and a yeast two-hybrid assay were conducted respectively. For the yeast one-hybrid assay, MYB binding motif sequences were synthesized; different fragments containing different MYB binding cis-elements of promoter sequences of Arabidopsis AtCBF3 and AtABF3 were cloned using primer pairs with restriction digestion sites that flank each of the fragments with genomic DNA as the template (see Supplementary Table S1 at JXB online). These sequences were ligated into the EcoRI and SacI sites of the pHIS2 vector (Clontech). The pGADT7 with full-length TaMYB73 ORF and pHIS2 either with or without the MYB binding motif sequences/AtCBF3 or AtABF3 promoter fragments were co-transformed into the yeast strain Y187 (Clontech), and transformants were selected by exposure to SD-Trp-Leu medium at 30 °C for 2 d, after which surviving colonies were transferred to SD-Trp-Leu-His medium containing either 10 mM or 30 mM 3-AT for 2 d. For the yeast two-hybrid assay, a full-length TaMYB73 ORF was PCR cloned (see Supplementary Table S1 at JXB online) into the pGADT7 vector (Clontech); the full-length AtICE1 ORF was PCR cloned from Arabidopsis cDNAs and ligated into pGBKT7 vector (Clontech). These vectors were transformed into the yeast AH109 strain (Clontech) for yeast two-hybrid analysis. Transformants were selected by growing on a SD-Trp-Leu medium at 30 °C. Surviving colonies were transferred to SD-Trp-Leu and SD-Trp-Leu-His-Ade medium for a further 2 d before observation.
Generation of transgenic Arabidopsis plants
TaMYB73 was introduced into the BamHI and SalI restriction endonuclease sites of the pSTART vector to construct a TaMYB73 over-expressor driven by the cauliflower mosaic virus (CaMV) 35S promoter (De Amicis et al., 2007) using the primers listed in Supplementary Table S1 at JXB online, and then transformed into Arabidopsis Col-0 by the Agrobacterium tumefaciens-mediated floral dip method (Clough and Bent, 1998). Transformed Arabidopsis plants were grown in soil under a 16/8 h light/dark, 22/20°C, 60% relative humidity regime. Transformed lines were selected by growing them on one-half Murashige and Skoog (MS) agar plates containing 60 μg ml−1 kanamycin under the same growth regime, and homozygous T3 progeny, bred from a T2 population segregating into three kanamycin-resistant to one kanamycin-sensitive, were used for the phenotypic analysis. Arabidopsis Col-0 transformed with the empty pSTART vector was used as the control.
Response to applied stress of transgenic Arabidopsis
Seeds harvested from both homozygous transgenic Arabidopsis carrying TaMYB73 (OE lines) and the pSTART empty vector (VC line) were surface-sterilized by a 5 min immersion in 70% ethanol, followed by a 10 min treatment with 5% w/v NaOCl and finally rinsed several times in sterile water. They were then germinated for 72 h in the dark at 22 °C by placing on sterile, solidified half-strength MS medium. Uniformly germinated seeds were then transferred to half-strength MS plates with various concentrations of NaCl, LiCl, KCl, and mannitol and the plates were placed vertically and kept under a 16/8 h light/dark, 22/20 °C, 60% relative humidity regime for one week. Two-week-old seedlings of VC and OE lines grown in soils were watered with 100 mM NaCl every 2 d for 2 weeks.
Germination assay of transgenic Arabidopsis
VC and OE seeds were surface-sterilized as above, laid on solidified half-strength MS medium containing various concentrations of ABA (0–2 μM) or NaCl (0–200 mM), and held at 4 °C for 2 d in the dark before being transferred to 22 °C under a 16/8 h light/dark regime. The germination rate of seedlings producing open cotyledons was expressed as a percentage of the total number of seeds plated.
Results
Analysis of TaMYB73 gDNA, cDNA, and upstream sequences
In order to identify a MYB transcription factor gene that may be closely involved in the salinity response in wheat, probes were screened targeting a set of unigenes with annotation as MYB transcriptional factors in the wheat gene microarray in our previous transcriptomic analysis on SR3 (Wang et al., 2008). According to these unigenes, more than 40 MYB genes were cloned (data not shown). Of them, the gene (TaMYB73) harboured three probes, which were induced to the greatest extent in salinity-stressed SR3 (Table 1). In roots, transcript abundance rose by about 6-fold within 0.5 h of the imposition of stress, while at 24 h, the transcript level was still higher than that in non-stressed plants (Table 1). The 1200 bp cDNA sequence of TaMYB73 comprised a 149 bp 5'-UTR, a 765 bp ORF, and a 286 bp 3'-UTR (Fig. 1a). A comparison with the genomic sequence showed that no intron was present (data not shown). The 254 residue predicted polypeptide included two conserved SANT domains at the N terminus (Fig. 1b). Phylogenetic analysis indicated that it clustered with R2R3 type MYB proteins of different plants (Fig. 1c), of which AtMYB15 has been confirmed as an important transcriptional factor involved in the salt- and drought-response signal transduction pathways in Arabidopsis (Agarwal et al., 2006; Ding et al., 2009). The TaMYB73 R2 and R3 SANT domains were conserved when compared with other plant MYB proteins (Fig. 1d). In the 847 bp upstream sequence of TaMYB73, ABA-responsive cis-elements, ACGT and ABRE, gibberellin-responsive cis-elements, GARE and MYC, as well as light-responsive G and E boxes were identified (see Supplementary Table S2 at JXB online).
Probe | N0.5 ratio | N24 ratio | ||||||||
Name | 1 | 2 | 3 | Mean | P value | 1 | 2 | 3 | Mean | P value |
ta_02121 | 2.41 | 2.24 | 2.85 | 2.50 | 2.63e-3 | 3.36 | 3.42 | 3.61 | 3.36 | 2.47e-4 |
ta_02124 | 1.84 | 1.59 | 2.10 | 1.84 | 3.18e-3 | 2.24 | 2.03 | 2.18 | 2.24 | 4.29e-4 |
ta_13116 | 2.43 | 2.31 | 2.64 | 2.46 | 7.89e-4 | 2.39 | 2.25 | 2.37 | 2.39 | 1.67e-4 |
Probe | N0.5 ratio | N24 ratio | ||||||||
Name | 1 | 2 | 3 | Mean | P value | 1 | 2 | 3 | Mean | P value |
ta_02121 | 2.41 | 2.24 | 2.85 | 2.50 | 2.63e-3 | 3.36 | 3.42 | 3.61 | 3.36 | 2.47e-4 |
ta_02124 | 1.84 | 1.59 | 2.10 | 1.84 | 3.18e-3 | 2.24 | 2.03 | 2.18 | 2.24 | 4.29e-4 |
ta_13116 | 2.43 | 2.31 | 2.64 | 2.46 | 7.89e-4 | 2.39 | 2.25 | 2.37 | 2.39 | 1.67e-4 |
Expression of TaMYB73 in the roots of SR3 in the presence and absence of 200 mM NaCl, as determined by hybridization patterns with three probes lying within the TaMYB73 sequence. N0.5 ratio: log2 (transcript level 0.5 h after the imposition of salinity stress/transcript level in the absence of salinity stress). N24 ratio: log2 (transcript level 24 h after the imposition of salinity stress/transcript level in the absence of salinity stress). 1, 2, 3: three independent biological replicates. P values calculated from these three replicates.
Probe | N0.5 ratio | N24 ratio | ||||||||
Name | 1 | 2 | 3 | Mean | P value | 1 | 2 | 3 | Mean | P value |
ta_02121 | 2.41 | 2.24 | 2.85 | 2.50 | 2.63e-3 | 3.36 | 3.42 | 3.61 | 3.36 | 2.47e-4 |
ta_02124 | 1.84 | 1.59 | 2.10 | 1.84 | 3.18e-3 | 2.24 | 2.03 | 2.18 | 2.24 | 4.29e-4 |
ta_13116 | 2.43 | 2.31 | 2.64 | 2.46 | 7.89e-4 | 2.39 | 2.25 | 2.37 | 2.39 | 1.67e-4 |
Probe | N0.5 ratio | N24 ratio | ||||||||
Name | 1 | 2 | 3 | Mean | P value | 1 | 2 | 3 | Mean | P value |
ta_02121 | 2.41 | 2.24 | 2.85 | 2.50 | 2.63e-3 | 3.36 | 3.42 | 3.61 | 3.36 | 2.47e-4 |
ta_02124 | 1.84 | 1.59 | 2.10 | 1.84 | 3.18e-3 | 2.24 | 2.03 | 2.18 | 2.24 | 4.29e-4 |
ta_13116 | 2.43 | 2.31 | 2.64 | 2.46 | 7.89e-4 | 2.39 | 2.25 | 2.37 | 2.39 | 1.67e-4 |
Expression of TaMYB73 in the roots of SR3 in the presence and absence of 200 mM NaCl, as determined by hybridization patterns with three probes lying within the TaMYB73 sequence. N0.5 ratio: log2 (transcript level 0.5 h after the imposition of salinity stress/transcript level in the absence of salinity stress). N24 ratio: log2 (transcript level 24 h after the imposition of salinity stress/transcript level in the absence of salinity stress). 1, 2, 3: three independent biological replicates. P values calculated from these three replicates.
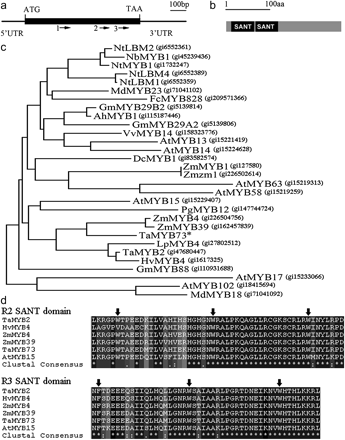
Structure, phylogeny, and domain alignment of TaMYB73. (a) TaMYB73 cDNA sequence, arrows 1, 2, and 3 represent the positions of cDNA microarray probes ta_13116, ta_02124, and ta_02121 in TaMYB73. (b) Predicted protein structure of TaMYB73. SANT: SANT SWI3, ADA2, N-CoR, and TFIIIB DNA-binding domain. (c) Phylogeny of TaMYB73 (starred) was constructed based on peptide sequences using a Neighbor–Joining method. (d) Alignment of R2 and R3 SANT domains. Identical amino acid residues are starred and conserved amino acid residues of SANT domain motifs are indicated by arrows. Ah, Arachis hypogaea; At, Arabidopsis thaliana; Dc, Daucus carota; Fc, Fagus crenata; Gm, Glycine max; Hv, Hordeum vulgare; Lp, Lolium perenne; Md, Malus×domestica; Nb, Nicotiana benthamiana; Nt, Nicotiana tabacum; Pg, Picea glauca; Ta, Triticum aestivum; Vv, Vitis vinifera; Zm, Zea mays.
TaMYB73 localizes in nuclei and has transactivation activity
The transient expression assay using onion epidermis cells and Arabidopsis mesophyll protoplasts both showed that TaMYB73 was localized in the nucleus (Fig. 2a). The transactivation assay indicated that all transformants grew well on the transformant selective SD/Trp plates (Fig. 2b), whereas only transformants of pGBKT7 that was fused with full-length TaMYB73 ORF (covering 1–254 aa) and truncated TaMYB73 ORF fragments expressing C terminal amino acid sequences (covering 65–254 aa, 121–254 aa, and 179–254 aa, respectively) grew on the transactivation selective SD/Trp-/Ade-/His plates (Fig. 2b), showing that these transformants activated the transcription of reporter genes Ade and His in the genome of the yeast AH109 strain. Consistently, β-galactosidase activity analysis indicated that the β-galactosidase units of the transformant with pGBKT7-TaMYB73 (covering 1–254 aa) were higher by about 2-fold than that of pGBKT7, and those of the pGBKT7s fused with truncated fragments (covering 65–254 aa, 121–254 aa, and 179–254 aa, respectively) were also significantly improved (Fig. 2c), demonstrating that the full-length and truncated TaMYB73 fragments containing C terminal 179–254 residues also activated the reporter gene LacZ in the genome of AH109, so that these transformants had higher β-galactosidase activity to catalyse more substrate OPNG. These two assays both indicated that TaMYB73 has transactivation activity, which is attributed to the C terminal 179–254 residues. In addition, the TaMYB73 protein can bind types I, II, and IIG MYB binding motifs (Fig. 2d).
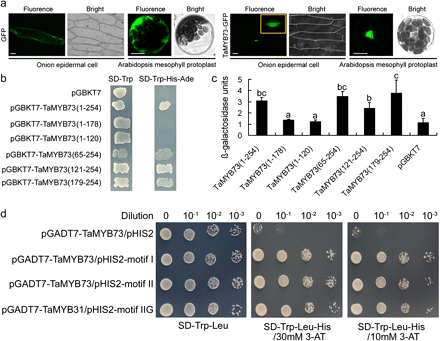
Subcellular localization, transactivation assay, and binding motifs of TaMYB73. (a) Transiently expressed TaMYB73 in onion epidermis cells and Arabidopsis mesophyll protoplasts shows its subcellular localization. GFP, green fluorescent protein. Bars, 100 μm. (b) Transactivation assay of TaMYB73. Either the full length or a truncated ORF of TaMYB73 was fused with pGBKT7, and transformed yeasts were selected on both SD-Trp and SD-Trp-His-Ade media, respectively. (c) β-galactosidase activity assay. The mean value is from three independent measurements, and error bars indicate the standard variation. pGBKT7 was used as a negative control. Columns without the same letter are significantly different using one-way ANOVA. (b, c) The numbers in brackets show the region of the TaMYB73 present, numbered from the N terminus. (d) DNA binding activity of TaMYB73 in a yeast one-hybrid system. Motifs I, II, and IIG mean three types of MYB binding motifs.
TaMYB73 is induced by various abiotic stresses
RT-PCR profiling indicated that TaMYB73 expression was increased in SR3 roots between 0.5 h and 24 h after exposure to 200 mM NaCl, while in the leaf, its transcript level was somewhat lower than in the non-stressed controls (Fig. 3a). Under dehydration stress, an increase in the TaMYB73 transcript level was observed within 0.5 h of the imposition of the treatment (Fig. 3b). The expression of TaMYB73 was induced during the whole course (0.5–24 h) of exogenous JA treatment (Fig. 3c). However, the response to exogenous supply of other phytohormones (GA, ABA, ACC, and SA) was an increase in TaMYB73 expression within 0.5 h, but the transcript level returned to its background level thereafter.
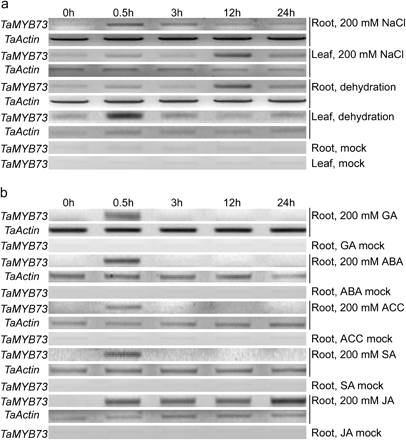
Expression of TaMYB73 in SR3, as assessed by RT-PCR. (a) Three-leaf stage seedlings exposed to 200 mM NaCl and dehydration. (b) Response of three-leaf stage seedlings to exogenously supplied phytohormone. GA, gibberellic acid; ABA, abscisic acid; ACC, 1-aminocyclopropane-1-carboxylic acid; SA, salicylic acid; JA, jasmonic acid. Three repetitions were performed for each PCR analysis.
TaMYB73 transgenic Arabidopsis shows enhanced salinity tolerance
In total, seven homozygous transgenic lines over-expressing TaMYB73 (OE) were obtained, and their TaMYB73 expression levels (transcription abundance of TaMYB73 to that of the internal reference gene AtActin) showed differences using real-time PCR. The vector control line (VC) had no detectable TaMYB73 transcript; by comparison with the transgenic line with the lowest expression level (6-13) as the standard (the relative expression level was 1), three other OE lines (3-21, 4-21, and 6-10) had the highest relative expression levels (the ratio of their expression levels to the that of the standard), and were selected for further analysis (Fig. 4a). When cultured in soil in the greenhouse, the plant phenotype, as well as developmental and reproductive ability such as flowering period and seed production, showed no significant difference among TaMYB73 OE and VC lines (upper panel in Fig. 4c; data not shown). When grown on solidified medium, the root length of OE plants was similar to that of VC plants (Fig. 4b). However, in the presence of 50 mM NaCl, leaf growth in VC plants was retarded, and root elongation was poorer than that in OE plants (Fig. 4b). Root growth remained superior in OE plants as the NaCl concentration was raised to 100, 150, and 200 mM (Fig. 4b). Similarly, OE lines grown in soils irrigated with 100 mM NaCl showed stronger development capacity than the VC line (Fig. 4c). The germination rate of OE seeds was higher than that of VC seeds in the presence of various concentrations of NaCl (Fig. 4d). A similar result was also found with treatment with different concentrations of ABA and, in the presence of 2 μM ABA, the germination of VC seeds was abolished, but some OE seeds continued to germinate (Fig. 4e).
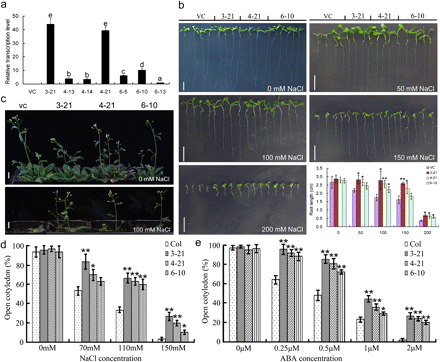
Stress response of Arabidopsis transgenic plants over-expressing TaMYB73. (a) Real-time PCR assay of selected over-expression lines. Over-expression (OE) line 6-13 had the lowest expression level (transcription abundance of TaMYB73 to that of internal reference gene AtActin), so the relative expression levels of OE lines were calculated as the ratio of their expression levels to that of line 6-13. The vector control (VC) line had no TaMYB73 transcript and was labelled as ND (not detectable). (b, c) Phenotypic response to salinity stress. (b) Three-day-old seedlings were transferred to a medium containing various concentrations of NaCl for 7 d before the images shown were taken. (c) Two-week-old seedlings were grown in soils and irrigated with 100 mM NaCl every 2 d for 2 weeks. (d, e) The effect of salinity stress and exogenously supplied ABA on the germination of Arabidopsis transgenic plants over-expressing TaMYB73. Bars, 1 cm. (a) Columns without the same letter are significantly different using one-way ANOVA. (b, d, e) The significance of difference between indices of VC and each OE was calculated using Student’s t test (*P <0.05; **P <0.01).
TaMYB73 transgenic Arabidopsis is specifically tolerant to ionic stress
When stressed by 5–15 mM LiCl, the OE lines appeared to be more tolerant than VC line (Fig. 5b, c). Roots of the OE seedlings also grew better than those of VC seedlings in the presence of 100 mM KCl (Fig. 5d). However, no obvious difference between VC and OE seedlings could be observed under 100–300 mM mannitol stress (Fig. 5e, f).
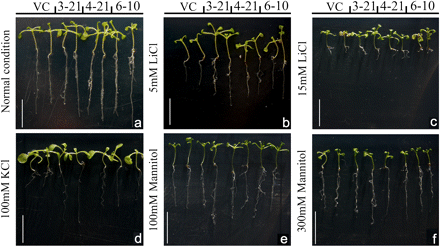
Response of Arabidopsis transgenic plants over-expressing TaMYB73 to stress imposed by the presence of LiCl, KCl, and mannitol. Three-day-old seedlings were transferred to media containing various concentrations of stress agent, and then grown for 7 d before the images shown were taken. VC, Empty vector transgenic line; 3-21, 4-21, and 6-10, over-expression (OE) transgenic lines. Bars, 1 cm. The photographs represent one of the same results from three independent experiments. (This figure is available in colour at JXB online.)
TaMYB73 promotes transcription of a range of stress response genes in Arabidopsis
Under the control conditions, AtABF3, AtMYB2, and AtRD29B participating in the ABA-dependent pathway were all induced in OE lines; ABA-independent genes AtRAB18 and AtCBF3 were induced in OE lines, but AtDREB2A and RD29A showed inconsistent changes among the three OE lines (Fig. 6). Except for AtDREB2A, all of these genes had higher transcriptional levels in the OE lines than in the VC line under salt stress. This increase in the OE lines was minor in comparison with the VC line under both control and saline stress conditions except for AtABF3 in two OE lines (3-21 and 4-21), the increased levels in the OE lines under control conditions were significantly lower than the induction level by salt stress in the VC line (Fig. 6). It should be noted that there was a strong variation of expression among three OE lines for AtRAB18, AtCBF3, AtRD29A, AtABF3 and AtRD29B (Fig. 6), indicating that the induction strength of their expression can be correlated to the transcription abundance of transgenic TaMYB73.
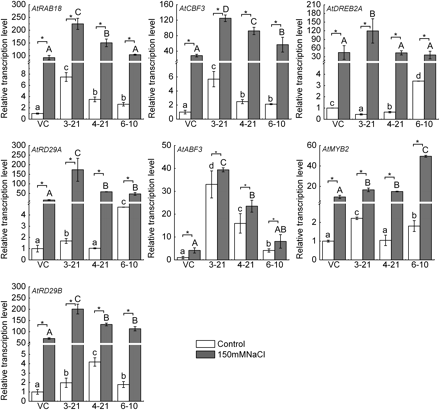
Real-time PCR analysis of the expression patterns of Arabidopsis transgenic plants over-expressing TaMYB73 in the presence or absence of 150 mM NaCl. Relative amounts were calculated and normalized with respect to the relative gene expression of Arabidopsis Col-0 transformed with the pSTART vector without treatment. White bars indicate transcript levels in the absence of salinity stress, black bars in the presence of salinity stress. AtActin was used as the reference gene. The data represent the mean of three replicates. VC, empty vector transgenic line; 3-21, 4-21, and 6-10, over-expression (OE) transgenic lines. The graphs represent one of the same results from three independent experiments. The significance of difference of indices between the control and salt-stressed conditions in VC or each OE line was calculated using Student’s t test (*P <0.05; ND, no significant difference). Columns without the same lowercase and capital letter are significantly different under the control and salt-stressed conditions, respectively, using one-way ANOVA.
TaMYB73 binds to promoters of AtABF3 and AtCBF3
A yeast one-hybrid assay was conducted to detect whether or not TaMYB73 is a putative transcription factor directly to regulate expression of AtABF3 and AtCBF3. The result showed that TaMYB73 can bind the upstream sequences of AtCBF3 and AtABF3 containing different MYB binding sequences such as MYBCORE and MYBCOREATCYB1 (Fig. 7a; see Supplementary Table S2 at JXB online), indicating that AtABF3 and AtCBF3 are possibly direct targets of TaMYB73. This is consistent with AtMYB15 (Agarwal et al., 2006), directly regulating the expression of these two genes (Zhu et al., 2007). Apart from this similarity, the yeast two-hybrid assay showed that, unlike AtMYB15 which interacts with AtICE1 to accomplish its direct regulation on AtABF3 and AtCBF3 (Zhu et al., 2007), TaMYB73 did not interact with AtICE1 (Fig. 7b).
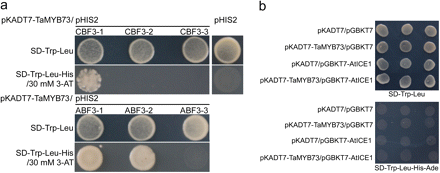
Binding ability of TaMYB73 with AtICE1 as well as promoters of AtABF3 and AtCBF3. (a) DNA binding activity of TaMYB73 in a yeast one-hybrid system. -1, -2, and -3 mean different fragments of the promoter sequences of AtCBF3 and AtABF3. (b) Interaction with AtICE1 in the yeast two-hybrid system. (This figure is available in colour at JXB online.)
Discussion
TaMYB73 encodes a novel salinity response transcription factor
The MYB superfamily is the largest transcription factor family in plants. Sublocalization, β-galactosidase and transactivation assays, and yeast one-hybrid analyses indicate that TaMYB73 is a transcription factor (Figs 2, 7a). TaMYB73 clusters with R2R3 type MYB proteins of other plants (Fig. 1c), indicating that TaMYB73 is a R2R3 type MYB transcription factor. TaMYB73 can be identified on the basis of their conserved SANT domains, which are typically located in the N terminal region and critical for DNA binding activity, while SANT domains of different MYB proteins have their specificities for distinct types of binding motifs. As R2R3 type MYBs, AtMYB15 binds preferentially to types II and IIG, rather than type I (Agarwal et al., 2006), whereas TaMYB73 binds equally well to types I, II, and IIG (Fig. 2d). This indicates that TaMYB73 is a novel MYB TF gene of plants.
Various plant transcription factors are up- or down-regulated in response to abiotic stress (Yamaguchi-Shinozaki and Shinozaki, 2006), and the response is typically quite rapid (Tran et al., 2004; Liu et al., 2007). An increase in TaMYB73 expression in SR3 roots occurred within 0.5 h of the imposition of either dehydration or salinity stress (Fig. 3a, b), which was consistent with the report that Tamyb2 was rapidly induced under osmotic stress for 1 h (Chen et al., 2005), showing the important roles of MYB transcription factors in rapid response to abiotic stresses in plants. By contrast, in the SR3 leaf, the TaMYB73 transcript level was slightly reduced at 0.5 h after the imposition of salinity stress (Fig. 3a). The Arabidopsis MYB transcription factor AtMYB44 behaves in a very similar fashion (Jung et al., 2008). Because salt first enters the plant via the root (not via the leaf), any response is bound to occur earlier in the root than in the leaf. Similarly for moisture stress: the leaf can conserve its moisture by stomatal closure while this mechanism is not available to the root (Berthomieu et al., 2003). Therefore, the different temporal response of the leaf and root to stress suggests that TaMYB73 expression simply reflects the extent of localized stress—the greater the stress, the more the gene is expressed. Apart from its rapid response to abiotic stress, TaMYB73 is also induced by the exogenous application of the phytohormones ABA, JA, ACC, GA, and SA (Fig. 3b, c). Of these phytohormones, the effect of ABA and GA is partially attributed to the presence of the ABA- and GA-responsive cis-elements ACGT, ABRE, GARE, and MYC in the promoter sequence of TaMYB73 (see Supplementary Table S2 at JXB online). More interestingly, unlike other phytohormones, the induction effect of JA is constitutive during the whole course of treatment. JA participates in biotic and abiotic stresses in plants, and has been found to exhibit antagonistic effects against ABA (Anderson et al., 2004; Moons et al., 1997), and to induce reactive oxygen species (ROS), nitric oxide, calcium influx, and mitogen-activated protein kinase (MAPK) and some salt-responsive genes such as RD26 (Fujita et al., 2006). Thus, it appears that this transcription factor can be activated by a number of different signals, so that it represents a node integrating several signalling pathways. Similarly, the expression of the Arabidopsis MYB transcription factor gene AtMYB102 has been documented to integrate signals derived from both wounding and osmotic stress (Denekamp and Smeekens, 2003).
TaMYB73 plays a role in the response to salinity
MYB proteins are involved in the response to stress in plants which, in turn, facilitate the ability to cope with these stresses. For example, salt- and drought-induced TaMYB2A promotes tolerance to these stresses in Arabidopsis (Mao et al., 2011). Here, the over-expression of TaMYB73 increased the tolerance of Arabidopsis to NaCl stress (Fig. 4b, c). It is concluded that the response to salt and drought stress is performed via both ABA-dependent and ABA-independent pathways. In transgenic Arabidopsis expressing TaMYB73, the ABA-independent pathway genes AtRAB18 and AtCBF3, as well as the ABA-dependent genes AtABF3 and AtRD29B, had more transcripts under both the control and salt-stressed conditions (Fig. 6). All of these genes have been reported to be induced under salt stress, and they also play positive roles in salt tolerance (Yamaguchi-Shinozaki and Shinozaki, 2006). For example, the over-expression of AtABF3 in rice improves drought tolerance without stunting growth (Oh et al., 2005). The combined results of induced transcription of TaMYB73 within 0.5 h of the ABA treatment (Fig. 3c) and the binding of TaMYB73 to the promoter sequences of AtCBF3 and AtABF3 (Fig. 7a) indicate that TaMYB73 is a component modulating both the ABA-dependent and ABA-independent pathways. Moreover, although TaMYB73 and AtMYB15 both bind to promoters of AtCBF3 and AtABF3 (Agarwal et al., 2006), TaMYB73 does not interact with AtICE1 (Fig. 7b), a crucial interacting protein that interacts with AtMYB15 to decrease, but not increase, the expression of these two genes (Zhu et al., 2007), demonstrating the common and specific characteristics among different MYB proteins. Furthermore, the ABA-independent pathway gene AtDREB2A remained constant between the VC and OE lines under salt stress (Fig. 6), whereas over-expression of other MYB factors have been documented to promote the expression of AtDREB2A rather than AtCBF3 (Dai et al., 2007; Liao et al., 2008). This shows the novel role of TaMYB73 in the response to salinity stress.
The salinity tolerance enhancement is possibly achieved by coping with ionic stress
Interestingly, OE lines had an increased tolerance to high concentrations of LiCl and KCl, yet showed no osmotic tolerance (mannitol treatment) (Fig. 5). The ionic-stress-specific response was also found in Arabidopsis sos4 and nks1 mutants: the former is hypersensitive to NaCl, LiCl, and KCl (Shi et al., 2002), and the latter is hypersensitive to NaCl and KCl (Choi et al., 2011). Salinity is thought to trigger programmed cell death (PCD) in plants largely through ionic (rather than osmotic) stress (Huh et al., 2002), suggesting that TaMYB73 functions to prevent salt-induced PCD. Given that salt stress comprises ionic and osmotic stresses (Munns and Tester, 2008), the salinity tolerance conferred by TaMYB73 appears to be mainly based on an improved ionic tolerance rather than osmotic tolerance. However, this seems to conflict with the higher transcription levels of the above detected marker genes in OE lines, for these genes are reported to be induced by drought and osmotic stress and their over-expression enhances the tolerance to osmotic and drought stresses. The possible cause is that most of these genes were increased in OE lines under both the control and saline stress conditions whereas the increase under the control conditions is only minor compared with the levels determined following salt treatment (Fig. 6), and therefore TaMYB73 is most likely to be a secondary player in the regulation of these stress-responsive genes in ABA-dependent and ABA-independent pathways, or this protein might undergo a post-translational modification under stress conditions which increase its potency as a transcription factor. Alternatively, it is proposed that the principal regulatory mechanism underlying the specific role of TaMYB73 in ionic stress may be beyond ABA-dependent and ABA-independent pathways. It has been found that the perception of the salt (ionic) stress signal as well as ionic absorption and intracellular compartmentation is fulfilled through both the SOS pathway (Yamaguchi-Shinozaki and Shinozaki, 2006) and the SOS-independent pathway (e.g. ER-associated endomembrane system) (Choi et al., 2011); however, in which pathway TaMYB73 participates is a question yet to be answered.
Taken together, the novel characteristic of TaMYB73 implies the complex roles of MYB transcription factors in salt response as well as their difference between Arabidopsis and wheat. TaMYB73’s specific role in tolerance to ionic stress provides an entrance to elucidate the difference in mechanisms underlying tolerance to salinity and osmotic stresses.
This work was supported by the National Basic Research 973 Program of China (2009CB118300 and 2012CB114200), the funds of the Major Program of the Natural Science Foundation of China (No. 30530480), and the National Transgenic Project (Grants 2009ZX08009-082B and 2008ZX08002-002). GenBank accession number JN969051.
References
Author notes
These authors contributed equally to this work.
Comments