-
PDF
- Split View
-
Views
-
Cite
Cite
Zhimiao Li, William M. Palmer, Antony P. Martin, Rongqing Wang, Frederick Rainsford, Ye Jin, John W. Patrick, Yuejian Yang, Yong-Ling Ruan, High invertase activity in tomato reproductive organs correlates with enhanced sucrose import into, and heat tolerance of, young fruit, Journal of Experimental Botany, Volume 63, Issue 3, February 2012, Pages 1155–1166, https://doi.org/10.1093/jxb/err329
- Share Icon Share
Abstract
Heat stress can cause severe crop yield losses by impairing reproductive development. However, the underlying mechanisms are poorly understood. We examined patterns of carbon allocation and activities of sucrose cleavage enzymes in heat-tolerant (HT) and -sensitive (HS) tomato (Solanum lycopersicum L.) lines subjected to normal (control) and heat stress temperatures. At the control temperature of 25/20 °C (day/night) the HT line exhibited higher cell wall invertase (CWIN) activity in flowers and young fruits and partitioned more sucrose to fruits but less to vegetative tissues as compared to the HS line, independent of leaf photosynthetic capacity. Upon 2-, 4-, or 24-h exposure to day or night temperatures of 5 °C or more above 25/20 °C, cell wall (CWIN) and vacuolar invertases (VIN), but not sucrose synthase (SuSy), activities in young fruit of the HT line were significantly higher than those of the HS line. The HT line had a higher level of transcript of a CWIN gene, Lin7, in 5-day fruit than the HS line under control and heat stress temperatures. Interestingly, heat induced transcription of an invertase inhibitor gene, INVINH1, but reduced its protein abundance. Transcript levels of LePLDa1, encoding phospholipase D, which degrades cell membranes, was less in the HT line than in the HS line after exposure to heat stress. The data indicate that high invertase activity of, and increased sucrose import into, young tomato fruit could contribute to their heat tolerance through increasing sink strength and sugar signalling activities, possibly regulating a programmed cell death pathway.
Introduction
Heat stress has become a more frequent and serious problem to crop productivity in recent years, probably due to climate change associated with global warming (Sato et al., 2000; Hedhly et al., 2008). In tomato, fruit number, fruit weight, and seed number per fruit were markedly decreased at daily mean temperatures of 29 °C compared with those at 25 °C (Peet et al., 1998). Severe heat stress (45 °C, 20 min) imposed on mature-green tomato fruit induced programmed cell death (PCD) as indicated by DNA fragmentation, release of cytochrome c, and activation of specific caspase-like enzymes (Qu et al., 2009). It is well recognized that plant reproductive organs are generally more sensitive to heat stress than vegetative organs (Ruan et al., 2010; Zinn et al., 2010). For instance, studies have shown detrimental effects of heat stress on developing pollen grains (Pressman et al., 2002, 2006; Firon et al., 2006; Frank et al., 2009). By contrast, however, little is known about the influence of heat stress on fruit and seed set, a critical phase for realizing yield potential (Ruan et al., 2010).
Sucrose is the principal end product of photosynthesis which is translocated from source leaves to sink organs through the phloem. Sucrose, and its hexose cleavage products, could regulate plant development and response to stresses through carbon allocation and sugar signalling (Roitsh and González, 2004). Sucrose synthase (SuSy; EC 2.4.1.13) and invertase (INV; EC 3.2.1.26) are the two enzymes that cleave sucrose into hexoses. The former contributes to maintaining sink strength and synthesizing storage products during organ maturation; the latter plays diverse roles in development and signalling (Koch, 2004; Weber et al., 2005; Ruan, 2007; Pugh et al., 2010; Ruan et al., 2010). Based on their pH optima and subcellular localization, INVs are categorized into three groups: cytoplasmic invertase (CIN), vacuolar invertase (VIN), and cell wall invertase (CWIN). Apart from their critical roles in development (Jin et al., 2009; Wang et al., 2010), both VIN and CWIN may act as major players in response to biotic and abiotic stresses (Sturm and Chrispeels, 1990; Roitsch et al., 2003; McLaughlin and Boyer, 2004; Essmann et al., 2008). For example, under drought, VIN and CWIN activity became very low in maize ovaries as compared with well-hydrated controls, leading to severe ovary abortion in water-deficient plants by triggering expression of genes responsible for PCD (Boyer and McLaughlin, 2007).
The CWIN gene family in tomato consists of four distinct members: Lin5, Lin6, Lin7, and Lin8 (Godt and Roistch, 1997). Lin5 and Lin7 are tandem repeats localized on tomato chromosome 9, while Lin8 and Lin6 are similar but localized on chromosome 10 (Fridman and Zamir, 2003). Gene expression profiling indicates that Lin5 and Lin7 are specifically expressed in reproductive organs but with different patterns: Lin5 was mainly expressed in ovaries and fruits whereas Lin7 was expressed in stamens and pollen (Fridman and Zamir, 2003). Lin8 was found to express in vegetative organs (root and leaf), while Lin6 was expressed mainly in vegetative tissues but weakly in young fruits (Fridman and Zamir, 2003). In addition to Lin5, TIV1, and SuSy1 genes coding for a tomato VIN and SuSy, respectively, are expressed strongly in tomato fruit (Godt and Roistch, 1997). Tomato fruit is therefore enriched in sucrose cleavage enzymes and hence represents an ideal system to examine their roles in fruit development and in response to heat stress.
Given that sucrose import and INV expression and activity are highly sensitive to abiotic stresses and that reduction of CWIN and VIN activities has been identified as a key early event causing ovary abortion under drought in maize (McLaughlin and Boyer, 2004), we hypothesized that a high sucrose import rate and INV activity in tomato reproductive organs may contribute to heat tolerance in young fruit. The hypothesis was tested by conducting a genotypic comparison of biomass partitioning to and sucrose import rate by INV and SuSy activities, and their gene expression patterns in developing fruit of a heat-sensitive (HS) and a heat-tolerant (HT) line under optimal and heat stress conditions. The analyses revealed that, compared with the HS line, the HT line displayed a higher sucrose import rate into, and CWIN activity of, 5-day young fruits, independent of leaf photosynthetic capacity, at the control temperature. After heat treatment, activities of both CWIN and VIN, but not SuSy, were significantly higher in young fruits of the HT line compared to those in the HS line. Low CWIN and VIN activities in the HS line were associated with a high expression of LePLDa1, encoding phospholipase D, which degrades cell membranes. The data suggest that high invertase activity of, and increased sucrose import into, young tomato fruit could contribute to their heat tolerance through increasing sink strength and sugar signalling activities possibly regulating a PCD pathway.
Materials and methods
Plant material and heat stress conditions
The HT and HS lines were selected from 100 tomato genotypes based on their fruit-set performance under heat stress conditions in a field trial carried out in Hangzhou, China, with latitude 30° 15′ N and longitude 120° 10′ E, in the summer of 2009. HT and HS lines were grown in the same plot under irrigated field conditions ensuring that plants did not suffer from water stress. Trusses 1–3 mainly developed in June with a daily mean temperature of 26 °C (moderate temperature), while trusses 4–6 mainly developed in July with daily mean temperature of 29 °C (high temperature). Flower and fruit numbers of each truss were recorded and fruit-set percentages computed. Two genotypes showing the highest and lowest fruit-set percentages were designated respectively as the HT and the HS lines examined in this study. The HT line, CLN 2413R, was released from the World Vegetable Center, Tainan, Taiwan. The HS line, T9246, is an inbred line with its ancestry in The Netherlands.
For heat stress experiments the HT and HS lines were grown in a temperature-controlled greenhouse at the University of Newcastle, Australia. Plants were grown under a control (normal) day/night temperature regime of 25 °C (6 am to 8 pm)/20 °C (8 pm to 6 am) with natural light illumination. Mild or strong heat stress respectively were 24-h temperature treatments of 30/25 °C (14-h day at 30/10-h night at 25 °C) or 36/28 °C (14-h day at 36/10-h night at 28 °C) at 4 days after anthesis. For short-term heat treatments, tomato plants grown under the control temperature regime of 25/20 °C were exposed to 30 °C during the daytime for 2 h (9–11 am) or 25 °C at night for 4 h (8–12 pm) at 5 days after anthesis. In all cases, plants were well irrigated with equal volumes of water delivered through an automatic watering system to ensure pots were held at or close to field capacity. Heat-treated plants exhibited no signs of water stress. Flowers were hand-pollinated and tagged on the day of anthesis (day 0). Flower and fruit samples were harvested into liquid nitrogen and stored at −80 °C for enzyme assay and RNA isolation.
Photosynthesis measurements
Maximum photosynthetic efficiencies of photosystem II (PSII; Fv/Fm) and net photosynthetic rates (PN) were measured on young fully expanded leaves supporting the growth of young fruit using a Li-6400 CO2 analyzer (LI-COR Incorporation, Lincoln, NE, USA) according to Wingler et al. (2004). The instrument was set at saturating light of 1000 μmol m−2 s−1 and a CO2 concentration of 380 ppm. Each monitored leaf was dark-adapted for 20 min before clamping it into the leaf chamber. The control temperature was maintained at 25 °C, and raised to 30 °C for photosynthesis measurements under mild heat stress.
Measurement of plant biomass
To ensure comparability at maturity between the two lines, both of which exhibit an indeterminate growth pattern, only fruits from trusses 1–3 were developed to full maturity, and the remaining trusses were pruned off. By fruit maturity, about 50 day after pollination, plants were harvested and dissected into fruits, stem, leaves, and roots. Fresh weights of each plant fraction were recorded. After drying at 80 °C for 48 h to constant weight each fraction was re-weighed to provide estimates of biomass.
Enzyme activity assay
Soluble and insoluble proteins were extracted from approximately 200 mg fresh weight of each sample for assay of CIN, VIN, CWIN, and SuSy activities as described by Tomlinson et al. (2004). Protein quantification was carried out by Bradford assay (Bradford, 1976). Enzyme activities were expressed on a protein basis (Jin et al., 2009; Wang et al., 2010). Expression of enzyme activity on this basis translates to fruit biomass as fruit protein contents were the same when expressed on a dry-weight basis from the HT and HS lines exposed to normal and heat stress temperatures (Supplementary Fig. S1).
Measurement of sucrose import rate into young fruits
Rates of phloem import of sucrose into fruits were estimated according to McCurdy et al. (2010). Briefly, each 5–6-day-old fruit replicate was excised by severing its pedicel with a razor blade while continuously irrigating with 20 mM ethylenediaminetetra-acetic acid (EDTA) buffered to pH 7.0 (100 mM Tris/HCl) to prevent callose formation in sieve pores and hence blockage of phloem sap exudation. Each pedicel was then submerged in a 0.5 ml tube containing 0.75% agarose containing 20 mM EDTA, pH 7.0. Each tube was sealed with parafilm to each severed pedicel and phloem sap was collected for 8 h from 8 am. Sap collection was terminated at 4 pm by snap-freezing each tube in liquid nitrogen.
Samples were thawed at 4 °C, transferred into a fresh 1.5 ml tube, and made up to 1 ml with distilled water before collecting the liquid by centrifugation at 16 000 g for 1 min. Sugars were extracted from supernatants followed by derivatization and quantification using gas chromatography/mass spectrometry (GC/MS) as described in Roessner et al. (2006). The excised fruits were dried for 72 h at 80 °C to determine their biomass.
Semi-quantitative reverse transcriptase (RT)-PCR analyses
Total RNA was extracted by using the RNeasy Plant Mini Kit (Qiagen, Melbourne, Australia) according to the manufacturer’s instructions. Following treatment with DNase I, 1 μl of RNA was transcribed to cDNA using oligo(dT)20 primer and SuperScript III RT (Invitrogen, Melbourne, Australia). First-strand cDNA (100 ng) was used as a template in RT-PCR performed on three biological replicates. Reproductive organ-expressed tomato INV and related genes (Lin5, Lin6, Lin7, TIV1, and INVINH1), two small heat-shock protein (sHSP) genes (LeHSP 17.4-CII and LeHSP 17.6-CII; see Giorno et al., 2010), and one senescence marker gene (LePLDa1, coding for phospholipase D; see McLaughlin and Boyer, 2004) were targeted for analysis. Primer sequences and PCR conditions are listed in Supplementary Table S1.
Western blot analysis
Cell wall proteins were isolated from 5-day fruit for SDS-PAGE. Western blot analysis was conducted as described by Bate et al. (2004) using a polyclonal antiserum raised against the CWIN inhibitor, INVINH1 (Jin et al., 2009), or a polyclonal antiserum against a synthetic peptide of CETLNAWSMDACKMN derived from the C-terminus of Lin5. The anti-Lin5 and anti-INVINH1 antisera detected predicted sized polypeptides at 64 and 16.4 kDa, respectively, with a degree of non-specific reaction with other peptides, typical of cell wall glycosylated proteins. Protein signals were visualized using anti-rabbit IgG conjugated with horseradish peroxidase.
Microarray hybridization assays
Array hybridizations were carried out using three biological replicates of RNA samples extracted from 5-day fruits of the HT and HS lines that were exposed to either control temperature at 25/20 °C or 24-h heat stress at 36/28 °C (day/night). The Affymetrix GeneChip Tomato Genome Array used consists of over 10 000 tomato probe sets (Frank et al., 2009). Probe preparation, chip hybridization, and primary data analysis were performed by CapitalBio Corporation (Beijing, China) according to the relevant Affymetrix GeneChip Expression Analysis Technical Manual (no. 701021, Rev. 5).
Twelve RNA samples (HT or HS lines at the control temperature or after heat stress with three biological replicates of each treatment) were labelled and hybridized to separate arrays. For statistical analysis these hybridizations were arranged into four groups, which included three biological replicates each. They were designated as Groups 1 and 3: the HT line after 24-h heat stress and at the control temperature, respectively; Groups 2 and 4: the HS line after 24-h heat stress and at the control temperature, respectively. This arrangement enabled detection of the effect of heat stress treatment (Group 1 versus Group 3, and Group 2 versus Group 4), the effect of genotypes (Group 1 versus Group 2, and Group 3 versus Group 4), as well as all possible interactions between the two effects, such as synergism or antagonism.
Data analysis was performed using CapitalBio Molecule Annotation System, version 3.0. The differentially expressed genes were identified as those with q values ≤0.05, fold change ≥2, simultaneously. The putative function of each gene was predicted by the Affymetrix annotation and by searching the National Center for Biotechnology Information database.
Results
The HT line displays a higher capacity of fruit-set under heat stress
To screen for tomato HT and HS lines, a field trial was conducted in the summer of 2009 from June to August. Trusses 1–3 flowered in June under moderate temperatures with a daily mean temperature of 26 °C, while trusses 4–6 developed in July under a higher daily mean temperature of 29 °C.
Two lines with highest and lowest fruit-set capacity were chosen for detailed analyses and designated as the HT and HS lines, respectively. The HT line developed more flowers from trusses 1–3 than the HS line (Fig. 1A). At a later development stage (trusses 4–6), flower numbers appeared to be similar between the two lines except for truss 5 (Fig. 1A). Under moderate temperature, the HT line set more fruits than the HS line for trusses 1–3 (Fig. 1B). Genotypic differences in fruit number became more marked in truss 3 onwards when plants experienced warm-to-hot weather conditions. Here, despite similar flower numbers from truss 3 and above (Fig. 1A), fruit numbers of the HS line were reduced to 25% of the HT line in truss 3 and zero in truss 4 (Fig. 1B) due to fruit abortion or stunted growth. Consequently, these trusses exhibited significantly lower fruit-set percentages in the HS than HT line (Fig. 1C).
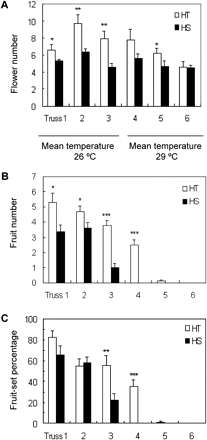
Comparison of flower (A) and fruit (B) numbers and fruit-set percentages (C) between field-grown HT and HS lines. Fruits from trusses 1–3 were set in June 2009 with a daily mean temperature of 26 °C (moderate temperature), while fruits from trusses 4–6 were set in July with daily mean temperature of 29 °C (high temperature). Each value is the mean ± SE from 10 biological replicates. An asterisk indicates a significant difference between the two lines at a given truss position (Student’s t test, *P < 0.05; **P < 0.01, ***P < 0.001).
The HT line partitions more carbohydrates to young fruits and less to vegetative organs
Fruit- and seed-set are regulated by sucrose import and utilization (Ruan et al., 2010). Sucrose import may be influenced by leaf photosynthesis (Boyer and McLaughlin, 2007). We therefore first examined photosynthetic capacity of young fully expanded leaves of HT and HS lines. The analyses revealed no difference in maximum photosynthetic efficiency (Fv/Fm) between the two lines at control temperature of 25 °C or under mild heat stress of 30 °C (Supplementary Fig. S2A). Net photosynthetic rates (PN) also were similar between the two lines at the control temperature (25 °C). However, under mild heat stress (30 °C) the HT line exhibited about a 20% higher PN compared with the HS line (Supplementary Fig. S2B). However, total area of fully expanded leaves of the HT line was 3029.2 ± 78.9 cm2 per plant and was about half of that of the HS line (5892.0 ± 306.6 cm2 per plant). Thus, whole-plant photosynthesis of the HS line exceeded that of the HT line even under mild heat stress. Hence a superior photosynthetic performance does not account the difference in heat tolerance exhibited between the two genotypes (see Boyer and McLaughlin, 2007).
To gain a clue as to whether strong early fruit development in HT line (Fig. 1) relates to higher ability of fruit to import sucrose, we measured sucrose import rates into young fruits. Fig. 2 shows a 38% higher rate of sucrose import into 5–6-day fruit of the HT line than of the HS line (Fig. 2A), accounting for a 26% greater dry-weight gain (Fig. 2B) and approximately 25 and 20% higher fruit fresh and dry weight (Fig. 2C), respectively, than the HS line.
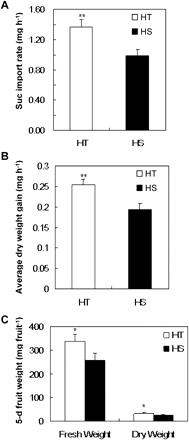
Rates of sucrose (suc) import into young fruits and the corresponding fruit biomass gain. (A) Sucrose import rates from pedicels into 5–6-day fruits of HT and HS lines. (B) Average dry-weight gains of 5–6-day fruits of HT and HS lines. (C) Five-day fruit fresh and dry weights. Each value is the mean ± SE from 10 biological replicates. An asterisk indicates a significant difference (Student’s t test, *P < 0.05; **P < 0.01).
To determine how sucrose import into fruit affects biomass accumulation at the whole-plant level at maturity, fresh and dry weights of vegetative organs were measured along with those of fruits. Both fresh weight and dry weight of HT fruits were significantly higher than those of the HS line (Fig. 3A). In contrast, biomass (dry weight) of stem and leaf (Fig. 3B) and root (Fig. 3C) fractions of the HT line were significantly lower than those of the HS line. These findings indicate the HT line partitioned more biomass to their reproductive organs and less to their vegetative organs. Mature fruits accounted for 44 and 26% of the total biomass in the HT and HS line, respectively, with their vegetative organs constituting the remaining portion (Fig. 3E). Total plant fresh or dry weight showed only slight, albeit significant, differences between the two lines (Fig. 3D).
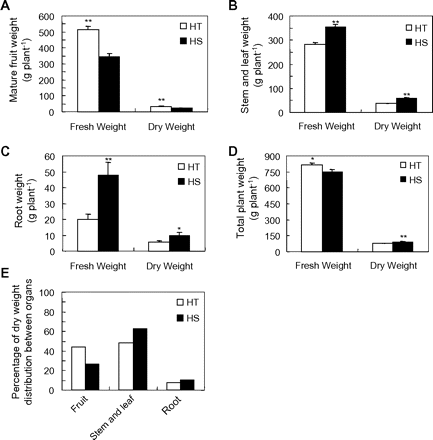
Whole-plant biomass partitioning in HT and HS lines. Fresh and dry (biomass) weights were measured for fruits (A), stems plus leaves (B), and roots (C) at fruit maturity from HT and HS lines. (D) Whole-plant weight from HT and HS lines. (E) Percentage of biomass in fruit and vegetative organs at maturity. Each value is the mean ± SE from eight biological replicates. An asterisk indicates a significant difference (Student’s t test, *P < 0.05; **P < 0.01).
The HT line exhibits higher CWIN activity in flower and young fruit and increases in fruit VIN, CIN, and SuSy activities after heat stress
To explore the biochemical basis of greater capacity of HT line for fruit-set (Fig. 1), we measured activities of sucrose-degrading enzymes in 5-day fruit. Developed under 25/20 °C, fruit CWIN activity from the HT line was about 4-fold higher than that from the HS line, while activities of CIN and VIN showed no difference between the two lines (Fig. 4A). In contrast, SuSy activity was higher in the HS line (Fig. 4A). Short-term heat treatment of raising day or night temperature by 5 °C for either 2 or 4 h resulted in fruit CWIN and VIN activities being significantly higher in the HT line compared to the HS line (Fig. 4B and C). A similar pattern of genotypic difference was found after a 24-h heat treatment (Fig. 4D). Further analyses revealed that following a 2-h heat treatment, activities of VIN, CIN, and SuSy increased while that of CWIN was maintained in HT line fruit (Fig. 4E). In contrast, in HS line fruit VIN, CIN, and SuSy activities were not altered and CWIN activity decreased (Fig. 4F). A similar pattern of genotypic differences was observed after a 24-h heat treatment (Fig. 4G and H).
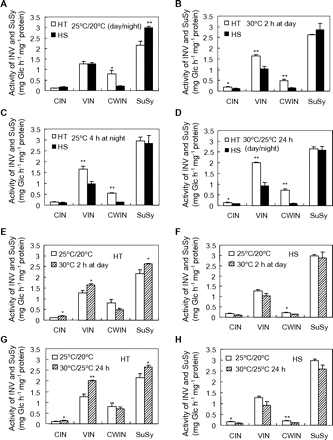
INV and SuSy activities of 5-day fruit from HT and HS lines at the control temperature or after mild heat stress (30/25 °C, day/night). (A) INV and SuSy activity of 5-day fruit from HT and HS lines at the control temperature of 25/20 °C (day/night). (B) INV and SuSy activity of 5-day fruit from HT and HS lines after 30 °C treatment for 2 h during daytime. (C) INV and SuSy activity of 5-day fruit from HT and HS lines after 25 °C treatment for 4 h at night. (D) INV and SuSy activity of 5-day fruit from HT and HS lines after 30/25 °C (day/night) mild heat stress for 24 h. (E, F) Pattern of INV and SuSy activity of 5-day fruit of the (E) HT line and (F) HS line exposed to the control temperature (25/20 °C, day/night) compared with a 2-h short-term mild heat stress (30 °C, daytime) immediately before sampling fruit. (G, H) Pattern of INV and SuSy activity of 5-day fruit of the (G) HT line and (H) HS line exposed to the control temperature (25/20 °C, day/night) compared with a 24-h long-term mild heat stress (30/25 °C, day/night) immediately before sampling fruit. Each value is the mean ± SE from three biological replicates. An asterisk indicates a significant difference (Student’s t test, *P < 0.05; **P < 0.01).
The difference in fruit INV activities observed between HT and HS lines following mild heat treatments (Fig. 4) prompted us to examine the effect of severe heat stress on enzyme activities. Fig. 5A shows that after a strong heat stress of 36/28 °C (day/night) for 24 h, fruit VIN and CWIN activities of the HT line were significantly higher than those of the HS line. A similar response was elicited by a mild heat stress treatment (Fig. 4D).
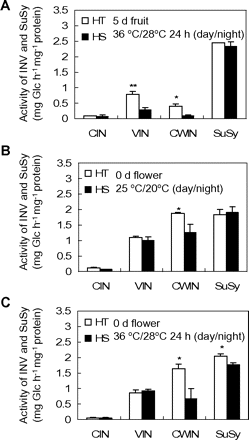
INV and SuSy activities of flowers and 5-day fruits from HT and HS lines at the control temperature or after 24 h of strong heat stress (36/28 °C, day/night). (A) INV and SuSy activity of 5-day fruit from HT and HS lines after 24 h of strong heat stress (36/28 °C, day/night). (B) INV and SuSy activity of 0-day flower from HT and HS lines at the control temperature of 25/20 °C (day/night). (C) INV and SuSy activity of 0-day flower from HT and HS lines after 24 h of strong heat stress (36/28 °C, day/night). Each value is the mean ± SE from three biological replicates. An asterisk indicates a significant difference (Student’s t test, *P < 0.05; **P < 0.01).
Enzyme assays of floral tissues at anthesis also revealed higher CWIN activities in the HT relative to the HS line (Fig. 5B). Upon a 24-h severe heat stress (see above), CWIN and SuSy activities of HT floral tissues were significantly higher than those of the HS line (Fig. 5C).
A CWIN gene, Lin7, is highly expressed in HT but weakly expressed in HS young fruit
The above analyses indicate that CWIN may play a role in heat tolerance in flowers and young fruits (Figs 4 and 5). To explore the molecular basis of high CWIN activity in reproductive organs of the HT line, semi-quantitative RT-PCR analyses were conducted to estimate transcript levels of INV genes known to express in flowers or young fruits (see Introduction). Given similar responses of fruit INV and SuSy activities to 24- and 2-h heat treatments (Fig. 4), gene expression analyses were conducted after a 24-h heat treatment alone in which activity changes were most marked. Fig. 6 shows transcript abundance of Lin5 in 5-day fruit was similar between the two lines under these conditions. Similar results were obtained at the protein level as detected by Western blotting (Fig. 7A). By contrast, Lin7, a direct tandem repeat of Lin5 on chromosome 9 (Fridman and Zamir, 2003) exhibited a higher expression in HT compared to HS young fruit under optimal and heat stress regimes (Fig. 6). Importantly, expression of Lin7 appeared to increase after mild or strong heat stress only in the HT line (Fig. 6). This finding was further verified by Affymetrix GeneChip Tomato Genome Array analyses, which shows that Lin7 transcript levels in 5-day young fruit from the HT line were 3.43- and 5.45-fold higher than those in HS line fruit under normal and after 24 h heat stress (36/28 °C), respectively (Supplementary Table S2).
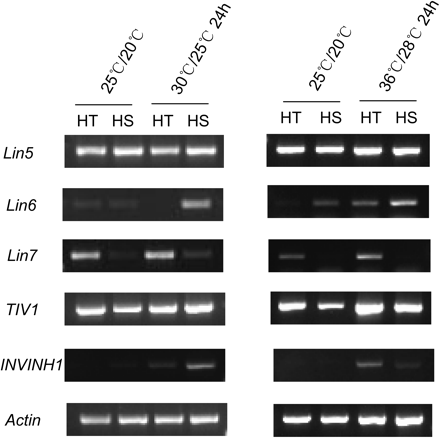
RT-PCR analysis of the expression profiles of INV genes from 5-day fruits of HT and HS lines at the control temperature (25/20 °C, day/night) and after 24-h mild (30/25 °C, day/night) or strong (36/28 °C, day/night) heat stress. For mild heat stress, all genes were amplified by 30 cycles. For strong heat stress, Lin5 and TIV1 were amplified by 25 cycles; Lin6, Lin7, and INVINH1 were amplified by 30 cycles. The reference gene, Actin, was amplified by 28 cycles.
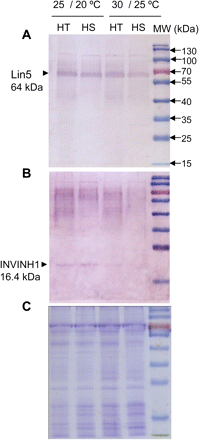
Western blot analyses of 5-day fruit from HT and HS lines at the control temperature (25/20 °C, day/night) and after 24-h mild heat stress (30/25 °C, day/night). (A) Protein gel blot analysis of CWIN, Lin5. Arrowhead indicates position of Lin5 at 64 kDa. (B) Protein gel blot analysis of invertase inhibitor, INVINH1. Note, a band at the predicted size of 16.4 kDa for INVINH1 (arrowhead) was evident at the control temperature for both lines but became hardly detectable at mild heat stress. (C) Coomassie blue stain of SDS/PAGE gel of cell wall protein of 5-day fruit from the HT and HS lines. The far-right lane is a molecular-mass-marker lane.
CWIN activity is subject to post-translational repression by an invertase inhibitor, INVINH1, in tomato (Jin et al., 2009). RT-PCR analysis showed that the INVINH1 mRNA was hardly detectable in 5-day young fruit under optimal conditions but was induced by heat stress (Fig. 6). Affymetrix GeneChip Tomato Genome Array also revealed a two-fold increase in INVINH1 mRNA level in HT young fruit after heat stress (36/28 °C) (Supplementary Table S2). Its protein level was, however, markedly reduced after heat stress in both lines (Fig. 7B).
The other two members of tomato CWIN gene family, Lin6 and Lin8 also were examined for their expression in young fruit. As previously reported (Fridman and Zamir, 2003), transcript levels of Lin8 were undetectable in young fruit. Lin6 showed low and similar mRNA levels between the HT and HS line at the control temperature and enhanced expression for both lines after severe heat stress at 36/28 °C (Fig. 6). TIV1, a gene coding for tomato VIN, exhibited a slightly higher expression level in the HT line, and appeared up-regulated after heat stress. Affymetrix Genome Array study revealed that TIV1 transcript from the HT line was approximately 1.4-fold higher than that from the HS line after 24-h heat stress (Supplementary Table S2).
To explore how CWIN may confer heat tolerance to young tomato fruit, we examined expression of some candidate genes known to be involved in response to heat or other abiotic stresses. To this end, HSPs are well known to play important roles in plant thermotolerance (Wang et al., 2004). Two sHSP genes in tomato, LeHsp 17.4-CII and LeHsp 17.6-CII, were suggested to be involved in heat tolerance of tomato anthers (Giorno et al., 2010). Fig. 8 shows that transcripts of these two genes were significantly up-regulated after a 24-h heat stress at 36/28 °C, in both HT and HS lines. Affymetrix GeneChip Tomato Genome Array analyses confirmed that the 24-h heat stress increased LeHsp 17.4-CII mRNA level by 3.17-fold in the HT line and LeHsp 17.6-CII mRNA level by 6.16- and 3.90-fold in HT and HS lines, respectively (Supplementary Table S2).
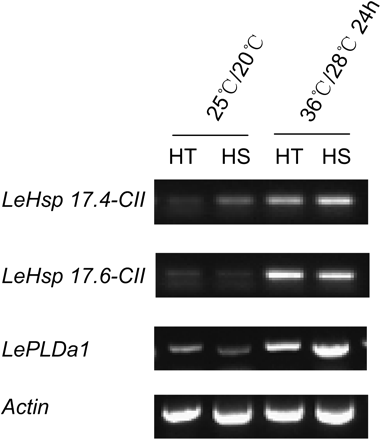
RT-PCR analysis of expression levels of two sHSP genes and one senescence gene (LePLDa1) of 5-day fruit from HT and HS lines exposed to the control temperature (25/20 °C, day/night) and after 24-h strong heat stress (36/28 °C, day/night). The sHSP genes, LeHsp 17.4-CII and LeHsp 17.6-CII, were amplified by 30 cycles and the senescence gene, LePLDa1, was amplified by 25 cycles. The reference gene, Actin, was amplified at 30 cycles.
Drought-induced reduction of INV in maize ovaries is followed by expression of PCD-related genes including PLD1, encoding phospholipase, which degrades cell membrane (Boyer and McLaughlin, 2007). This finding inspired us to explore the potential genotypic difference in PLD1 expression in young fruit under heat stress. We searched the National Center for Biotechnology Information database and found a tomato phospholipase homologue LePLDa1 (GenBank accession no. AY013252). The LePLDa1 transcript was significantly up-regulated in young fruits of both genotypes by heat, with the HS line showing the greater response (Fig. 8).
Discussion
CWIN and VIN may contribute to heat tolerance of tomato flowers and young fruit
This study provides genotypic evidence that high CWIN and VIN activities are likely to play important roles in heat tolerance in tomato reproductive organs. First, CWIN was the only sucrose cleavage enzyme in flowers and 5-day young fruits that exhibited higher activity in the HT line compared to the HS line under control temperature conditions (Figs 4A and 5B). Second, CWIN and VIN activities were significantly higher in HT young fruits after short- (2- or 4-h) or long-term (24-h) heat stress (Fig. 4B, C, and D). This outcome resulted from maintaining and increasing CWIN and VIN activities respectively in the HT line (Fig. 4E and G). These responses were absent from the HS line (Fig. 4F and H). In fact, CWIN activity was reduced in HS young fruits upon heat treatments (Fig. 4F and H) similar to that found in anthers of tomato flower buds (Pressman et al., 2006). Third, after severe heat stress, CWIN and VIN activities remained significantly higher in HT young fruit, with the former being higher in flowers as well (Fig. 5A and C). The above analyses also suggest that CWIN may be involved in heat tolerance of both young fruits and flowers whereas VIN appears to only contribute to fruit heat tolerance. Relevant to this view, reductions in CWIN and VIN activities in reproductive organs is considered to be responsible for grain abortion under drought in maize (McLaughlin and Boyer 2004) and wheat (Ji et al., 2010).
It is noted that CIN activity also was induced in young fruits of the HT line alone by heat stress (Fig. 4E and G). However, its activity was very low compared to that of CWIN and VIN (Figs 4 and 5) rendering it unlikely to be a major contributor to heat tolerance. High activity of SuSy was observed in flowers and young fruits (Figs 4 and 5). However, no differences were found in fruit between HT and HS lines after heat treatments (Fig. 4B, C, and D), indicating that SuSy is unlikely to be a major factor responsible for genotypic difference in fruit-set under heat stress (Fig. 1C). However, heat-induced increases in SuSy activity were noticed in young fruits (Fig. 4E and G) and flowers (Fig. 5C) of the HT line. Thus, its potential contribution to heat-tolerance of reproductive organs cannot be ruled out.
High sucrose import rate and CWIN and VIN activity may contribute to tomato fruit heat tolerance through sugar signalling regulating PCD pathway
The major phenotypic difference between HT and HS lines is that the former produced more flowers and they exhibited a much higher capacity to set fruit, particularly in trusses 3 and 4, when plants began to experience hot weather conditions (Fig. 1). Fruit and seed set are highly vulnerable to reductions in sucrose supply under heat or drought (Barnabás et al., 2008,;Ruan et al., 2010). In maize, for example, 14-h heat stress before silking reduced grain yield but increased biomass of vegetative tissues (Suwa et al., 2010). Thus, an ability to partition photoassimilate (predominately sucrose) to reproductive organs would appear to strengthen tolerance to abiotic stresses including heat. Consistent with this idea, the HT line exhibited higher biomass ratio of fruit to vegetative organs compared to the HS line (Fig. 3). The feature is most likely due to a higher phloem import rate of sucrose to young fruits in the HT line (Fig. 2).
Phloem import rates of sucrose into sinks could be influenced by supply from photosynthetic leaves, strength in sink tissues or hydraulic conductivities of phloem pathways interconnecting source and sink. In this context, the two genotypes exhibited the same maximum photosynthetic efficiency (Fv/Fm) and net photosynthetic rate (PN) at the control temperature (Supplementary Fig. S2A and Supplementary Data). Since total leaf area of the HS line is almost double that of the HT line (see Results), it follows that the amount of sucrose produced in the HS line would be much higher than the HT line. Therefore, the observed lower rate of phloem import into young fruit in the HS line (Fig. 2A) is due to factors other than source supply from photosynthesis.
Noticeably, the HT line exhibited significantly lower biomass partitioned to vegetative tissues including leaves, stems and roots as compared to the HS line (Fig. 3). Therefore, it is likely that more sucrose would be available for partitioning to fruit in the HT lines consistent with a higher phloem import rate of sucrose in pedicels when their fruits were excised (Fig. 2A). Thus the lower phloem import rate into HS fruit (Fig. 2A), despite higher amounts of sucrose produced in their leaves (see above), is probably due to stronger competition by vegetative tissues in this line (Fig. 3B and C). In addition, or alternatively, vascular development in fruit peduncle of the HS line may lag behind that of the HT line (see Goren et al., 2011). This would contribute to a lowered phloem conductance accounting for a lower phloem import rate of sucrose by young HS fruit (Fig. 2A).
sHSPs belong to a superfamily of chaperones that function to prevent protein misfolding or denaturation during development and under stress (Kotak et al., 2007; Tripp et al., 2009). In tomato, two members of sHSP, LeHsp 17.4-CII and LeHsp 17.6-CII, together with HsfA2, were found to contribute to heat tolerance of tomato anthers (Giorno et al., 2010). In agreement with their role in heat tolerance, expression of these two sHSP genes was markedly up-regulated in young fruit after strong heat stress (Fig. 8; Supplementary Table S2). The high abundance of sHSP may help protect CWIN and VIN stability under heat stress. This issue may be particularly relevant here since both CWIN and VIN need to be transported to their respective cellular destinations through secretory pathways (Ruan et al., 2010) where correct folding and packaging supported by sHSPs would be critical (Kotak et al., 2007).
Significant induction of PLDa1, a PCD marker gene encoding phospholipase D, which breaks down cell membranes (McLaughlin and Boyer, 2004), was induced in young fruits by heat with transcript levels being higher in HS than HT fruit (Fig. 8). Induction of PLD1 and another PCD gene, RIP1, occurred in maize ovaries after reduction of transcript levels of the CWIN and VIN genes Incw2 and Ivr2 under drought conditions (McLaughlin and Boyer, 2004). Importantly, down-regulation of INV genes and subsequent induction of PCD genes caused ovary abortion under drought. This phenomenon was shown to be under sucrose control since feeding sucrose recovered expression of INCW2 and Ivr2 and suppressed PCD gene expression, thus reversing abortion under drought (McLaughlin and Boyer, 2004; Boyer and McLaughlin, 2007). Most strikingly, these are the only sugar-responsive genes among many candidate genes surveyed, demonstrating that a INV-mediated sugar signalling pathway regulating PCD is the primary biochemical and molecular mechanism controlling maize ovary abortion under drought (Boyer and McLaughlin, 2007). Given phenotypic similarities in plant responses to drought and heat (Ruan et al., 2010), it is plausible to propose that higher fruit INV activities in the HT line (Figs 4 and 5) could generate higher amounts of hexoses which may account for suppressed expression of PLD1 (Fig. 8) and possibly other PCD-related genes, leading to an increased heat tolerance (Fig. 1). Further studies are required to gain a definitive answer to the causality between sucrose import, INV activity, PCD and heat tolerance in tomato ovary and fruit.
It is recognized that high expression levels of VIN (TIV1) and CWIN (Lin7) in the HT line under heat stress are associated with changes in expression of genes controlling various cellular functions (Supplementary Table S2). In particular, genes responsible for cell wall loosening, endo-1,4-β-glucanase, chitinase, and expansin, were several-fold higher in the HT line, suggesting a higher capacity for cell expansion in their fruits. Several genes involved in lipid and hormone metabolism, defence, and transcriptional regulation also changed significantly in the HT line after heat stress (Supplementary Table S2). It remains to be determined whether expression of these genes contributes to heat tolerance in tomato fruit and, if so, whether they act dependently or independently from INV-mediated sugar signalling as proposed above.
CWIN Lin7 may control CWIN activity that contributes to heat tolerance in tomato flowers and young fruits
Among the four CWIN genes, Lin5 is the most abundantly expressed in tomato fruit (Godt and Roitsch, 1997; Fridman and Zamir, 2003; see also Fig. 6). However, no difference was found in its transcript levels between the two genotypes at the control temperature or after mild and strong heat stress (Fig. 6). This finding was verified further by observations that (i) Lin5 was not differentially expressed between the two lines in our Affymetrix GeneChip analyses (Supplementary Table S2) and (ii) protein gel-blot analysis showed no difference in Lin5 abundance between the two genotypes (Fig. 7). Collectively, these results suggest that Lin5 may not be involved in heat stress response of young tomato fruit.
By contrast, expression levels of Lin7 in HT 5-day fruits were consistently higher than those of the HS line, either at the control temperature or after 24-h mild or strong heat stress (Fig. 6). Moreover, after heat stress at 36/28 °C for 24 h, expression levels of Lin7 in the HT line were 5.45-fold higher than that of the HS line, whereas under control temperature, Lin7 mRNA level in the HT fruit was only 3.43-fold higher (Supplementary Table S2). This indicates that Lin7 expression is enhanced by heat in the HT line. Such an induction was apparent in semi-quantitative RT-PCR analyses where Lin7 mRNA appeared to increase in the HT but not in the HS line, especially after strong heat stress (Fig. 6). Thus, we conclude that Lin7, but not Lin5, is the CWIN isoform that may be responsible for the higher CWIN activity in HT flowers and young fruits.
In planta CWIN activity is capped by its inhibitory protein (Jin et al., 2009; Ruan et al., 2009). The transcript level of an invertase inhibitor, INVINH1, was hardly detectable in young fruit as previously reported (Jin et al., 2009) but slightly induced under heat stress (Fig. 6). Interestingly, INVINH1 protein abundance appeared to be reduced by heat treatment for both lines (Fig. 7B). Differential regulation at transcription and translation levels, as the case for the INVINH1 (Figs 6 and 7), is not an unusual phenomenon in response to stress. For example, heat stress increased transcript, but not the encoded protein, levels of HSP101 in maize and wheat (Barnabás et al., 2008).
Overall, the HT and HS lines exhibited different sensitivities to heat stress. For the HT line, high fruit CWIN activity was maintained from 25/20 °C to 30/25 °C (Fig. 4E and G) before it was nearly halved at 36/28 °C (compare Figs 5A and 4G). By contrast, CWIN activity of HS fruit decreased significantly from 25/20 °C to 30/25 °C (Fig. 4F and H). Similarly, VIN activity in the HT line increased from 25/20 °C to 30/25 °C before it dropped at 36/28 °C, whereas VIN activity from the HS line was reduced by approximately 25% at 30/25 °C as compared to that at 25/20 °C (Fig. 4E to H; Fig.5A). Thus, HT line has a higher optimal temperature for INV activity than that of HS fruit, which correlates with its higher capacity to set fruit under heat stress (Fig. 3). Although high expression of Lin7 may contribute to high CWIN activity in fruit from HT line (see discussion above), further studies are required to elucidate the molecular basis underlying the genotypic difference of INV sensitivity in response to heat stress.
Conclusion
The data provide correlative evidence that a high capacity for sucrose import and INV activity could contribute to heat tolerance in young tomato fruit possibly through increasing glucose signalling activities repressing the PCD pathway. In conjunction with previous work (Boyer and McLaughlin, 2007; Ruan et al., 2010), these findings indicate that the INV-mediated PCD pathway through sugar signalling is conserved in reproductive organs between the eudicotyledonous species of tomato and the monocotyledonous maize in response to heat and water stress, respectively.
Supplementary material
Supplementary material is available at JXB online.
Supplementary Table S1. List of primer sequences and PCR conditions used for semi-quantitative RT-PCR analysis of INV, sHSP, and senescence genes.
Supplementary Table S2. Transcript levels of genes identified by Affymetrix microarray analyses between HT and HS lines with or without heat treatment.
Supplementary Fig. S1. Protein content of 5-day young fruit of the HT and HS lines at the control temperature (25/20 °C) and after 24 h heat stress (30/25°C). Each value is the mean ± SE from three biological replicates. No significant difference was found between the two lines under each condition (Student’s t test, P < 0.05).
Supplementary Fig. S2. Leaf photosynthetic efficiency in HT and HS lines at the control temperature or under mild heat stress. (A) Maximum photosynthetic efficiency (Fv/Fm) of the HT and HS lines. (B) Net photosynthetic rate (PN). Each value is the mean ± SE from three biological replicates. Asterisk(s) indicates a significant difference (Student’s t test, *P < 0.05; **P < 0.01).
Abbreviations
- CWIN
cell wall invertase
- VIN
vacuolar invertase
- SuSy
sucrose synthase
- PCD
programmed cell death
- CIN
cytoplasmic invertase
- HS
heat-sensitive
- HT
heat-tolerant
- EDTA
ethylenediaminetetra-acetic acid
- RT
reverse transcriptase
- sHSP
small heat-shock protein
- Fv/Fm
maximum photosynthetic efficiency
- PN
net photosynthetic rate
This work was supported by the Australian Research Council (grant DP110104931) to Y-LR and a Special Fund for International Cooperation from Zhejiang Academy of Agricultural Sciences, China, to YY.
Comments