-
PDF
- Split View
-
Views
-
Cite
Cite
Glenn E. Bartley, Betty K. Ishida, Ethylene-sensitive and insensitive regulation of transcription factor expression during in vitro tomato sepal ripening, Journal of Experimental Botany, Volume 58, Issue 8, June 2007, Pages 2043–2051, https://doi.org/10.1093/jxb/erm075
- Share Icon Share
Abstract
Tomato (Solanum lycopersicum, formerly Lycopersicon esculentum) cv. VFNT Cherry sepals, when cultured in vitro between 16 °C and 22 °C, change their genetic programme to that of ripening fruit. Previously regulation of a number of transcription factors and a putative G-protein-coupled receptor that may be involved in tomato fruit ripening and cool-temperature sepal morphogenesis had been revealed. Many of those genes such as TAG1, TM4, TM6, AP2-like (LeAP2FR), YABBY2-like (LeYAB2), and LeCOR413-PM1 have not been investigated for ethylene regulation. Ethylene-independent, regulated transcripts may be part of an early signalling process induced or de-repressed by cool temperature that causes a switch in the genetic programme of the sepals. In this paper, ethylene regulation of a number of these and other putative signalling factors are investigated during cool-temperature-induced sepal morphogenesis. 1-Methylcyclopropene was used to block ethylene-induced gene expression by interrupting the ethylene signal transduction pathway that occurs in ripening tomato fruits and presumably in ripening sepals. Transcripts of several putative transcription factors previously shown to be up-regulated during cool-temperature-induced sepal morphogenesis (TAG1, TM4, LeAP2FR) were only slightly or not induced in 1-methylcyclopropene-treated sepals, indicating either direct or indirect ethylene regulation. Two genes, VAHOX1, a homeobox domain leucine-zipper-encoding gene, and LeYAB2, a putative zinc-finger transcription factor-encoding gene, increased in treated and untreated sepals indicating regulation by cool temperatures independently of ethylene.
Introduction
Ripening is a process that transforms fruit to become more appealing to potential consumers for seed dispersal. In tomato, ripening is characterized by tissue softening, accumulation of sugars and acids, conversion of chloroplasts to lycopene-accumulating chromoplasts, and evolution of ethylene and flavour volatiles (Gray et al., 1992), and involves regulation of hundreds of expressed genes (Alba et al., 2005). At least two regulatory systems affect ripening in tomatoes, one involving regulation of ripening by ethylene is a well-investigated process that modifies transcription of many ripening-related genes (Theologis, 1992) and another involving ethylene-independent genes that are regulated through developmental signals during ripening (Vrebalov et al., 2002). Transcription factors are needed to regulate many aspects of plant and animal development and responses to stress and environmental cues (Riechmann, 2002). MADS-box transcription factors are important regulators of reproductive and vegetative development in plants (Ng and Yanofsky, 2001). A number of MADS-box genes are highly expressed early during development of tomato fruit (Bartley and Ishida, 2002; Busi et al., 2003; Fei et al., 2004). The MADS-box gene RIN (RIPENING-INHIBITOR) is an ethylene-independent developmentally regulated transcription factor proposed to be a global regulator of ripening (Vrebalov et al., 2002). A mutation in RIN results in the tomato rin (ripening-inhibitor) ripening mutant phenotype (Tigchelaar et al., 1978). RIN mutants do not ripen even upon application of exogenous ethylene. Vrebalov et al. (2002) showed that RIN expression was not significantly affected 12 h after exposure of wild-type mature green fruits to 10 ppm ethylene. RIN expression is also investigated in this paper. Previously a number of putative transcription factors whose expression is altered during tomato fruit ripening and cool-temperature sepal morphogenesis had been reported (Bartley and Ishida, 2002, 2003). Two of these factors, the MADS-box genes TAG1 (the tomato homologue of the Arabidopsis flower organ-identity gene AGAMOUS) and TM4 (a gene expressed early in flower development) are up-regulated during tomato fruit ripening and in vitro sepal ripening at cool temperatures (Pnueli et al., 1991; Ishida et al., 1998: Bartley and Ishida, 2003). TM6, another MADS-box gene involved in tomato flower development, is up-regulated in cool-temperature-cultured sepals, but is down-regulated during tomato fruit ripening (Pnueli et al., 1991; Bartley and Ishida, 2002, 2003).
Sepals of Solanum lycopersicum (formerly Lycopersicon esculentum) cv. VFNT Cherry and one or two closely related varieties, when cultured in vitro between 16 °C and 22 °C, change their developmental programme to that of ripening fruit, while those cultured at 28 °C do not (Ishida, 1991; Ishida et al., 1993, 1998). Other varieties of tomato do not exhibit this phenomenon. This cool-temperature-induced ripening process involves swelling, ethylene evolution, POLYGALACTURONASE (PG) and PHYTOENE SYNTHASE 1 (PSY1) induction, lycopene accumulation, and evolution of flavour volatiles. Accumulation of lycopene and loss of chlorophyll cause sepals to become red as in ripe tomato fruit (Fig. 1). Concurrent with lycopene accumulation, ethylene is evolved as in climacteric tomato fruit at the onset of ripening (Ishida, 1991). The mechanism of this cool-temperature morphogenesis is unknown. Regulatory genes are important components of this process.
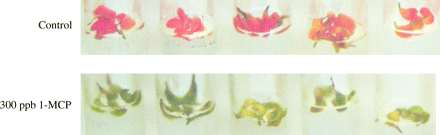
To discern the role of these putative transcriptional regulators, it is important to determine whether they are ethylene-independent or ethylene-induced. Here, is reported the use of 1-methylcyclopropene (1-MCP) to block ethylene-induced gene expression by interrupting the ethylene signal-transduction pathway that occurs in ripening tomato fruits and presumably ripening sepals. 1-MCP competes with ethylene at ethylene receptor sites, thereby preventing ethylene binding and action (Sisler and Serek, 1997). Use of 1-MCP to block ethylene action is well documented (Sisler et al., 1996; Sisler and Serek, 1997; Hoeberichts et al., 2002; Wills and Ku, 2002; Mir et al., 2004; Opiyo and Ying, 2005; Watkins, 2006). Treatment of mature green tomatoes with 1-MCP delays ripening (Mir et al., 2004). Certain ripening-related transcription factors based on their previously determined expression profiles, either cool-temperature or ripening induced, were selected to determine those not affected by ethylene and those that show ripening-associated ethylene-enhancement of transcription.
Materials and methods
1-MCP treatment of plant material
Sepals from small green fruit were harvested from greenhouse-grown plants (Solanum lycopersicum cv. VFNT Cherry). Tomato sepals were excised from freshly picked green fruit 0.3–1.0 cm in diameter, disinfested with 10% CLOROX plus a few drops of Tween 20 (Sigma) for 7 min, rinsed with sterile water three times, and placed on sterile MS solidified medium, pH 5.7, in test tubes (Ishida et al., 1998). These cultures were then placed in thin layer chromatography tanks at 16–18 °C and illuminated at an average intensity of 75 μmol m−2 s−1 using Grolux lamps (GTE Sylvania, Danvers, MA, USA) for 16 h d−1. Although the manufacturer recommends a 100–300 ppb (0.1–0.3 μl l−1) 1-MCP application for tomato, the optimum concentration for the present in vitro sepal system was determined to be 300 ppb using concentrations 100, 300, 600, and 1200 ppb. One set of tanks was therefore brought to approximately 300 ppb 1-MCP using ETHYLBLOC™ (Rohm and Haas, http://www.rohmhaas.com/ethylbloc/products.html), according to the manufacturer's protocol, using a syringe to draw off gaseous 1-MCP evolved from a water and substrate mix. A second set of tanks was maintained without 1-MCP to hold control samples. Both sets of tanks were opened at the same time intervals, but only one set was treated with 1-MCP. The tanks were refreshed with 1-MCP at 5, 15, 19, 22, and 29 d. The concentration of 1-MCP in a sealed container containing plant tissue declines with time and ethylene may accumulate (Hoeberichts et al., 2002). Over time new receptors may be produced and require blocking by 1-MCP for the effect to be sustained. Sepals from both sets of tanks were harvested at 5, 29, and 35 d.
RNA extraction and relative RT-PCR
Total RNA was isolated and used in RT-PCRs, according to Bartley and Ishida (2003), except that the number of total cycles ranged from 23 to 26 depending on the primer sets involved. Oligonucleotide sequences used for relative RT-PCR can be found in Bartley and Ishida (2003) except for CNR and VAHOX1. The real-time PCR primers for CNR were also used for relative PCR (Table 1). VAHOX1RTF2 and VahOX1RTR1 primers were used for relative PCR but primers VAHOX1RTF1 and VAHOX1RTR1 were used for real-time PCR (Table 1). The sequence for PSY primers, SPS3 and PSY, can be found in Bartley and Scolnik (1993). All PCR primers except PSY and SPS3 were designed to amplify only one member of a gene family. The positive control, PHYTOENE SYNTHASE 1 (PSY1), is up-regulated during tomato fruit ripening (Bartley and Scolnik, 1993). A constitutively expressed tomato ELONGATION FACTOR 1-α gene (LeEF1-α, GenBank accession no. X14449) was used as a control (Pokalsky et al., 1989; Bartley and Ishida, 2003). As an additional control for the RT-PCR, 10-fold dilutions of each sample of the reverse-transcription reactions used in this experiment were made and PCRs were performed using the same conditions and LeEF1-α primers (Fig. 2).
No. | Transcript | 5′ Sequence 3′ | Direction | Name |
1 | PSY1 | TTTGATGAGGCAGAGAAAGGC | Forward | PSY1RTF1 |
2 | GTGAAGTTGTTGTAGTCATTGG | Reverse | PSY1RTR1 | |
3 | TAG1 | CCAACAACCATTACCCTAGACAA | Forward | TAG1RTF1 |
4 | TAATAGCAGTCTTCTTTTGTGGAT | Reverse | TAG1RTR1 | |
5 | LeAP2FR | TTAGGAGGTTCAAGCCAAGAAAT | Forward | AP2LRTF2 |
6 | TGCAGCAGTTGCAATTATCTCAT | Reverse | AP2LRTR2 | |
7 | TM4 | ACAACAACTGGACTCTCCTCACC | Forward | TM4RTF1 |
8 | AAGAACTACCTCCTTCCACTTCC | Reverse | TM4RTR1 | |
9 | TM6 | ACACAATCTTTATGCTTTTCGC | Forward | TM6RTF1 |
10 | AATGTTTAACGCCATTACTGTG | Reverse | TM6RTR1 | |
11 | RIN | CAACAAAAGGAACAATCTCTTA | Forward | RINRTF1 |
12 | TATTTGTACACTTTGCTCACCAC | Reverse | RINRTR1 | |
13 | NOR | GCCAATTTCCTGAGCCAGTTTCCT | Forward | CUC1RTF1 |
14 | TAAGAGTACCAATTCATGCCAGTA | Reverse | CUC1RTR1 | |
15 | LeCOR413-PM1 | AACACATTAGAGCATCTGGTGGA | Forward | 85295RTF1 |
16 | AAGTGGAGAATCAATGCCCAGAC | Reverse | TC85295R1 | |
17 | LEYAB2 | TTGGACTCAAGCTGGATGGCAAC | Forward | YBBY2RTF1 |
18 | TAGAGACCAATTGTTTTCTGAGG | Reverse | YBBY2RTR1 | |
19 | VAHOX1 | GCAAGAATATGCTTTCTACTGCC | Forward | VAHOX1RTF1 |
20 | ATGAATTGAGGAATAGATACGATA | Forward | VAHOX1F2 | |
21 | ATAAGTCCAGAAACCAAAACCTT | Reverse | VAHOX1RTR1 | |
22 | LeEF1-α | AAGAATGTTGACAAGAAGGAC | Forward | LEF1RTF4 |
23 | ACACTCGATGTAAATAAATAGC | Reverse | LEF1RTR5 | |
24 | PSY2 | TTGATGAGGCAGAGAAAGGTGT | Forward | PSY2RTF3 |
25 | TTGCTTCAATCTCGTCCAGTATC | Reverse | PSY2RTR3 | |
26 | CNR | GTCAGCAATGTAGCAGATTTCAT | Forward | CNRF3 |
27 | TGCCATTGATGCTGATGCTTCTT | Reverse | CNRR1 |
No. | Transcript | 5′ Sequence 3′ | Direction | Name |
1 | PSY1 | TTTGATGAGGCAGAGAAAGGC | Forward | PSY1RTF1 |
2 | GTGAAGTTGTTGTAGTCATTGG | Reverse | PSY1RTR1 | |
3 | TAG1 | CCAACAACCATTACCCTAGACAA | Forward | TAG1RTF1 |
4 | TAATAGCAGTCTTCTTTTGTGGAT | Reverse | TAG1RTR1 | |
5 | LeAP2FR | TTAGGAGGTTCAAGCCAAGAAAT | Forward | AP2LRTF2 |
6 | TGCAGCAGTTGCAATTATCTCAT | Reverse | AP2LRTR2 | |
7 | TM4 | ACAACAACTGGACTCTCCTCACC | Forward | TM4RTF1 |
8 | AAGAACTACCTCCTTCCACTTCC | Reverse | TM4RTR1 | |
9 | TM6 | ACACAATCTTTATGCTTTTCGC | Forward | TM6RTF1 |
10 | AATGTTTAACGCCATTACTGTG | Reverse | TM6RTR1 | |
11 | RIN | CAACAAAAGGAACAATCTCTTA | Forward | RINRTF1 |
12 | TATTTGTACACTTTGCTCACCAC | Reverse | RINRTR1 | |
13 | NOR | GCCAATTTCCTGAGCCAGTTTCCT | Forward | CUC1RTF1 |
14 | TAAGAGTACCAATTCATGCCAGTA | Reverse | CUC1RTR1 | |
15 | LeCOR413-PM1 | AACACATTAGAGCATCTGGTGGA | Forward | 85295RTF1 |
16 | AAGTGGAGAATCAATGCCCAGAC | Reverse | TC85295R1 | |
17 | LEYAB2 | TTGGACTCAAGCTGGATGGCAAC | Forward | YBBY2RTF1 |
18 | TAGAGACCAATTGTTTTCTGAGG | Reverse | YBBY2RTR1 | |
19 | VAHOX1 | GCAAGAATATGCTTTCTACTGCC | Forward | VAHOX1RTF1 |
20 | ATGAATTGAGGAATAGATACGATA | Forward | VAHOX1F2 | |
21 | ATAAGTCCAGAAACCAAAACCTT | Reverse | VAHOX1RTR1 | |
22 | LeEF1-α | AAGAATGTTGACAAGAAGGAC | Forward | LEF1RTF4 |
23 | ACACTCGATGTAAATAAATAGC | Reverse | LEF1RTR5 | |
24 | PSY2 | TTGATGAGGCAGAGAAAGGTGT | Forward | PSY2RTF3 |
25 | TTGCTTCAATCTCGTCCAGTATC | Reverse | PSY2RTR3 | |
26 | CNR | GTCAGCAATGTAGCAGATTTCAT | Forward | CNRF3 |
27 | TGCCATTGATGCTGATGCTTCTT | Reverse | CNRR1 |
No. | Transcript | 5′ Sequence 3′ | Direction | Name |
1 | PSY1 | TTTGATGAGGCAGAGAAAGGC | Forward | PSY1RTF1 |
2 | GTGAAGTTGTTGTAGTCATTGG | Reverse | PSY1RTR1 | |
3 | TAG1 | CCAACAACCATTACCCTAGACAA | Forward | TAG1RTF1 |
4 | TAATAGCAGTCTTCTTTTGTGGAT | Reverse | TAG1RTR1 | |
5 | LeAP2FR | TTAGGAGGTTCAAGCCAAGAAAT | Forward | AP2LRTF2 |
6 | TGCAGCAGTTGCAATTATCTCAT | Reverse | AP2LRTR2 | |
7 | TM4 | ACAACAACTGGACTCTCCTCACC | Forward | TM4RTF1 |
8 | AAGAACTACCTCCTTCCACTTCC | Reverse | TM4RTR1 | |
9 | TM6 | ACACAATCTTTATGCTTTTCGC | Forward | TM6RTF1 |
10 | AATGTTTAACGCCATTACTGTG | Reverse | TM6RTR1 | |
11 | RIN | CAACAAAAGGAACAATCTCTTA | Forward | RINRTF1 |
12 | TATTTGTACACTTTGCTCACCAC | Reverse | RINRTR1 | |
13 | NOR | GCCAATTTCCTGAGCCAGTTTCCT | Forward | CUC1RTF1 |
14 | TAAGAGTACCAATTCATGCCAGTA | Reverse | CUC1RTR1 | |
15 | LeCOR413-PM1 | AACACATTAGAGCATCTGGTGGA | Forward | 85295RTF1 |
16 | AAGTGGAGAATCAATGCCCAGAC | Reverse | TC85295R1 | |
17 | LEYAB2 | TTGGACTCAAGCTGGATGGCAAC | Forward | YBBY2RTF1 |
18 | TAGAGACCAATTGTTTTCTGAGG | Reverse | YBBY2RTR1 | |
19 | VAHOX1 | GCAAGAATATGCTTTCTACTGCC | Forward | VAHOX1RTF1 |
20 | ATGAATTGAGGAATAGATACGATA | Forward | VAHOX1F2 | |
21 | ATAAGTCCAGAAACCAAAACCTT | Reverse | VAHOX1RTR1 | |
22 | LeEF1-α | AAGAATGTTGACAAGAAGGAC | Forward | LEF1RTF4 |
23 | ACACTCGATGTAAATAAATAGC | Reverse | LEF1RTR5 | |
24 | PSY2 | TTGATGAGGCAGAGAAAGGTGT | Forward | PSY2RTF3 |
25 | TTGCTTCAATCTCGTCCAGTATC | Reverse | PSY2RTR3 | |
26 | CNR | GTCAGCAATGTAGCAGATTTCAT | Forward | CNRF3 |
27 | TGCCATTGATGCTGATGCTTCTT | Reverse | CNRR1 |
No. | Transcript | 5′ Sequence 3′ | Direction | Name |
1 | PSY1 | TTTGATGAGGCAGAGAAAGGC | Forward | PSY1RTF1 |
2 | GTGAAGTTGTTGTAGTCATTGG | Reverse | PSY1RTR1 | |
3 | TAG1 | CCAACAACCATTACCCTAGACAA | Forward | TAG1RTF1 |
4 | TAATAGCAGTCTTCTTTTGTGGAT | Reverse | TAG1RTR1 | |
5 | LeAP2FR | TTAGGAGGTTCAAGCCAAGAAAT | Forward | AP2LRTF2 |
6 | TGCAGCAGTTGCAATTATCTCAT | Reverse | AP2LRTR2 | |
7 | TM4 | ACAACAACTGGACTCTCCTCACC | Forward | TM4RTF1 |
8 | AAGAACTACCTCCTTCCACTTCC | Reverse | TM4RTR1 | |
9 | TM6 | ACACAATCTTTATGCTTTTCGC | Forward | TM6RTF1 |
10 | AATGTTTAACGCCATTACTGTG | Reverse | TM6RTR1 | |
11 | RIN | CAACAAAAGGAACAATCTCTTA | Forward | RINRTF1 |
12 | TATTTGTACACTTTGCTCACCAC | Reverse | RINRTR1 | |
13 | NOR | GCCAATTTCCTGAGCCAGTTTCCT | Forward | CUC1RTF1 |
14 | TAAGAGTACCAATTCATGCCAGTA | Reverse | CUC1RTR1 | |
15 | LeCOR413-PM1 | AACACATTAGAGCATCTGGTGGA | Forward | 85295RTF1 |
16 | AAGTGGAGAATCAATGCCCAGAC | Reverse | TC85295R1 | |
17 | LEYAB2 | TTGGACTCAAGCTGGATGGCAAC | Forward | YBBY2RTF1 |
18 | TAGAGACCAATTGTTTTCTGAGG | Reverse | YBBY2RTR1 | |
19 | VAHOX1 | GCAAGAATATGCTTTCTACTGCC | Forward | VAHOX1RTF1 |
20 | ATGAATTGAGGAATAGATACGATA | Forward | VAHOX1F2 | |
21 | ATAAGTCCAGAAACCAAAACCTT | Reverse | VAHOX1RTR1 | |
22 | LeEF1-α | AAGAATGTTGACAAGAAGGAC | Forward | LEF1RTF4 |
23 | ACACTCGATGTAAATAAATAGC | Reverse | LEF1RTR5 | |
24 | PSY2 | TTGATGAGGCAGAGAAAGGTGT | Forward | PSY2RTF3 |
25 | TTGCTTCAATCTCGTCCAGTATC | Reverse | PSY2RTR3 | |
26 | CNR | GTCAGCAATGTAGCAGATTTCAT | Forward | CNRF3 |
27 | TGCCATTGATGCTGATGCTTCTT | Reverse | CNRR1 |
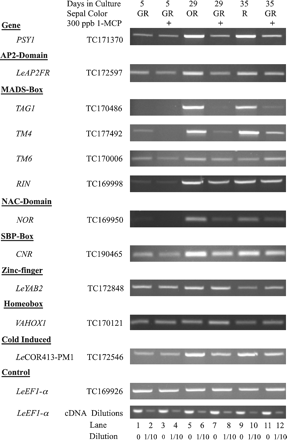
RT-PCR of transcripts regulated during cool temperature ripening in untreated sepals and sepals treated with 1-MCP. GR, green; OR, orange/red; R, red; +, 1-MCP treatment. At the bottom is a control PCR of LeEF1-α indicating relative abundance of mRNAs. Odd-numbered lanes contain samples obtained exactly as in the reactions above, while the even-numbered lanes were the same reverse transcription reaction samples that were diluted 10-fold and used in the PCR.
Real-time PCR
cDNAs resulting from reverse transcription of the above total RNAs and cDNAs from a previous experiment, 24 d of culture at 16 °C or 28 °C (Bartley and Ishida, 2003), were diluted 10-fold and 1 μl aliquots were used in real-time PCRs with SYBR Green Supermix (BIO-RAD), according to the manufacturer's protocols with these changes: (i) reactions were performed in 25 μl total volume; (ii) an MX3000P (Stratagene) instrument was used to perform the PCR. PCR conditions were 5 min at 95 °C followed by 40 cycles of incubation at 94 °C×15 s, 55 °C×1 min, and 72 °C×30 s. Primer efficiencies were determined using dilution curves of cDNA and ranged from 94 % to 81.7 %. Relative quantitation was performed by normalization to the elongation factor 1-alpha transcript as in Livak and Schmittgen (2001). Only one time point was investigated to conserve materials and reagents as each real-time RT-PCR experiment was performed in triplicate. This time point in the 1-MCP experiment also showed the greatest difference in PSY1, TAG1, and TM4 gene expression when comparing treated and untreated sepals with relative PCR. It should be noted here that the transcript of only one particular EF1-α member (TC169926) of a gene family was used for normalization because other members of the gene families may vary in their abundance (Bartley and Ishida, 2003). PHYTOENE SYNTHASE 2 (PSY2) was included as an example of a gene that is not up-regulated during ripening (Bartley and Scolnik, 1993). Negative controls to determine the extent of DNA contamination were identical concentrations of total RNAs that were not reverse transcribed. A negative control was run for each primer set. In each case the no-reverse transcription control signal was achieved only after nine or more cycles more than the samples that were transcribed. The results from the real-time PCR analysis are shown in Table 2. Sequences of the primers for real-time PCR are presented in Table 1. The 1-MCP experiment and the previous experiment are not directly comparable because of the different culture conditions, one in capped test tubes in TLC tanks (1-MCP) and the other not in tanks (16 °C versus 28 °C) (Bartley and Ishida, 2003).
Gene | Fold difference untreated/treated after 29 d of culture | Fold difference cool/normal after 24 d of culture |
Orange/green | 16 °C/28 °C | |
PSY1 | 41.1±5.0 | 30.7±3.4 |
PSY2 | 1.2±0.3 | 1.2±0.1 |
TAG1 | 12.2±0.3 | 15.4±1.6 |
TM4 | 15.0±1.2 | 7.2±1.2 |
TM6 | 1.6±0.1 | 2.8±0.3 |
LeCOR413-PM1 | 3.5±1.0 | 4.5±0.4 |
LeYAB2 | 1.4±0.2 | 3.5±0.2 |
RIN | 3.0±0.4 | 2.3±0.6 |
CNR | 3.0±0.2 | 2.0±0.2 |
LeAP2FR | 12.5±2.0 | 15.6±3.0 |
VAHOX1 | 1.0±0.2 | 1.9±0.3 |
NOR | 9.6±0.2 | 3.2±0.6 |
Gene | Fold difference untreated/treated after 29 d of culture | Fold difference cool/normal after 24 d of culture |
Orange/green | 16 °C/28 °C | |
PSY1 | 41.1±5.0 | 30.7±3.4 |
PSY2 | 1.2±0.3 | 1.2±0.1 |
TAG1 | 12.2±0.3 | 15.4±1.6 |
TM4 | 15.0±1.2 | 7.2±1.2 |
TM6 | 1.6±0.1 | 2.8±0.3 |
LeCOR413-PM1 | 3.5±1.0 | 4.5±0.4 |
LeYAB2 | 1.4±0.2 | 3.5±0.2 |
RIN | 3.0±0.4 | 2.3±0.6 |
CNR | 3.0±0.2 | 2.0±0.2 |
LeAP2FR | 12.5±2.0 | 15.6±3.0 |
VAHOX1 | 1.0±0.2 | 1.9±0.3 |
NOR | 9.6±0.2 | 3.2±0.6 |
Data normalized for the LEF1-α transcript. Values are means ±standard deviation.
Gene | Fold difference untreated/treated after 29 d of culture | Fold difference cool/normal after 24 d of culture |
Orange/green | 16 °C/28 °C | |
PSY1 | 41.1±5.0 | 30.7±3.4 |
PSY2 | 1.2±0.3 | 1.2±0.1 |
TAG1 | 12.2±0.3 | 15.4±1.6 |
TM4 | 15.0±1.2 | 7.2±1.2 |
TM6 | 1.6±0.1 | 2.8±0.3 |
LeCOR413-PM1 | 3.5±1.0 | 4.5±0.4 |
LeYAB2 | 1.4±0.2 | 3.5±0.2 |
RIN | 3.0±0.4 | 2.3±0.6 |
CNR | 3.0±0.2 | 2.0±0.2 |
LeAP2FR | 12.5±2.0 | 15.6±3.0 |
VAHOX1 | 1.0±0.2 | 1.9±0.3 |
NOR | 9.6±0.2 | 3.2±0.6 |
Gene | Fold difference untreated/treated after 29 d of culture | Fold difference cool/normal after 24 d of culture |
Orange/green | 16 °C/28 °C | |
PSY1 | 41.1±5.0 | 30.7±3.4 |
PSY2 | 1.2±0.3 | 1.2±0.1 |
TAG1 | 12.2±0.3 | 15.4±1.6 |
TM4 | 15.0±1.2 | 7.2±1.2 |
TM6 | 1.6±0.1 | 2.8±0.3 |
LeCOR413-PM1 | 3.5±1.0 | 4.5±0.4 |
LeYAB2 | 1.4±0.2 | 3.5±0.2 |
RIN | 3.0±0.4 | 2.3±0.6 |
CNR | 3.0±0.2 | 2.0±0.2 |
LeAP2FR | 12.5±2.0 | 15.6±3.0 |
VAHOX1 | 1.0±0.2 | 1.9±0.3 |
NOR | 9.6±0.2 | 3.2±0.6 |
Data normalized for the LEF1-α transcript. Values are means ±standard deviation.
Ethylene measurement
Ethylene produced by sepal tissues was collected by sealing culture tubes, as described by Ishida (2000). Gas samples collected for approximately 1 h at 23 °C were injected into a Hewlett-Packard gas chromatograph Model 6890 equipped with a ¼-inch stainless-steel column (Porapak-N, 80–100 mesh, Alltech Associates, Inc., Deerfield, IL, USA), a flame ionization detector, and an HP 3396 Series II integrator (Hewlett-Packard). Plant tissues were weighed under aseptic conditions in a laminar-flow hood after each series of ethylene measurements and then returned to treatment tanks until the next set of measurements.
Gene identification and database mining
Previously the tomato expressed sequence tag (EST) database entitled Tomato Gene Index (LGI) at TIGR (The Institute for Genomic Research) was used to confirm sequence identity and to browse for genes having interesting regulation during fruit ripening (Bartley and Ishida, 2002). This database is now located at the Harvard Computational Biology and Functional Genomics Laboratory website, http://compbio.dfci.harvard.edu/tgi/cgi-bin/tgi/gimain.pl?gudb=tomato. TCs (tentative consensus sequences) are cDNA sequences assembled from overlapping ESTs common to that transcript. TC numbers change over time, but a search using the original number will generally find the correct database entry. For purposes of consistency with previous work and to complement the TIGR databases, at least two TC numbers for each gene in a list were included (Table 3). In some cases, notably PSY1 and TM4, more than one accession exists because of sequence anomalies and errors in the original cloned sequences, causing misclassification of the ESTs associated with these genes. The most representative form is used in the text and in Fig. 2, but in Table 3 some alternative accessions are listed. Additionally, some confusion exists in the literature in regard to the MADS-box genes TM4 and TM6 (Pnueli et al., 1991; Lozano et al., 1998; Fei et al., 2004). In Table 3TM4 and TM6 are shown with their aliases TDR4 and TDR6 because their GenBank files still contain that annotation although the references do not (Pnueli et al., 1991; Lozano et al., 1998).
Gene | GenBank accession | Current TC number | Previous TC numbers |
PSY1 | M84744 | TC171370, TC178429, TC169935 | TC84644, TC115970, TC115971, TC116252, TC115968, TC153853, TC153852, TC153854 |
PSY2 | L23424 | TC 183738 | TC120273, TC158116 |
LeAP2FL | N/A | TC180296 | TC154703 |
LeAP2FR | N/A | TC172597, TC178186 | TC85031, TC124194, TC124195, TC162117 |
TAG1 | L26295, AY098733 | TC170486, TC190126 | TC89786, TC124766, TC154658 |
TM4 (TDR4) | X60757, AY098732, AY306155 | TC177492, TC173952 | TC94405, TC125305, TC126125, TC154847, TC158381 |
TM6 (TDR6) | X60759, Y098734 | TC170006 | TC90108, TC117238, TC154953 |
RIN | AF448522 | TC169998 | TC91451, TC126704, TC164766 |
CNR | DQ672601 | TC190465 | TC125032, TC162962 |
NOR | AY573802 | TC169950 | TC149932, TC117875, TC155746 |
VAHOX1 | X94947 | TC170121 | TC124192/TC94020, TC154081 |
LeYAB2 | N/A | TC172848, TC170528 | TC89502, TC124135, TC154049 |
LeCOR413-PM1 | AW039062 | TC172546 | TC85295, TC116832, TC154633 |
LeEF1-α | X14449, X53043 | TC169926, TC172095 | TC98347, TC123771, TC161869 |
Gene | GenBank accession | Current TC number | Previous TC numbers |
PSY1 | M84744 | TC171370, TC178429, TC169935 | TC84644, TC115970, TC115971, TC116252, TC115968, TC153853, TC153852, TC153854 |
PSY2 | L23424 | TC 183738 | TC120273, TC158116 |
LeAP2FL | N/A | TC180296 | TC154703 |
LeAP2FR | N/A | TC172597, TC178186 | TC85031, TC124194, TC124195, TC162117 |
TAG1 | L26295, AY098733 | TC170486, TC190126 | TC89786, TC124766, TC154658 |
TM4 (TDR4) | X60757, AY098732, AY306155 | TC177492, TC173952 | TC94405, TC125305, TC126125, TC154847, TC158381 |
TM6 (TDR6) | X60759, Y098734 | TC170006 | TC90108, TC117238, TC154953 |
RIN | AF448522 | TC169998 | TC91451, TC126704, TC164766 |
CNR | DQ672601 | TC190465 | TC125032, TC162962 |
NOR | AY573802 | TC169950 | TC149932, TC117875, TC155746 |
VAHOX1 | X94947 | TC170121 | TC124192/TC94020, TC154081 |
LeYAB2 | N/A | TC172848, TC170528 | TC89502, TC124135, TC154049 |
LeCOR413-PM1 | AW039062 | TC172546 | TC85295, TC116832, TC154633 |
LeEF1-α | X14449, X53043 | TC169926, TC172095 | TC98347, TC123771, TC161869 |
The TC number in the fourth column is the older number used in previous papers, and the third column is the most recent TIGR database accession number. Underlined TCs are used in the text in case of multiple current accessions. N/A, none available in the non-redundant GenBank database.
Gene | GenBank accession | Current TC number | Previous TC numbers |
PSY1 | M84744 | TC171370, TC178429, TC169935 | TC84644, TC115970, TC115971, TC116252, TC115968, TC153853, TC153852, TC153854 |
PSY2 | L23424 | TC 183738 | TC120273, TC158116 |
LeAP2FL | N/A | TC180296 | TC154703 |
LeAP2FR | N/A | TC172597, TC178186 | TC85031, TC124194, TC124195, TC162117 |
TAG1 | L26295, AY098733 | TC170486, TC190126 | TC89786, TC124766, TC154658 |
TM4 (TDR4) | X60757, AY098732, AY306155 | TC177492, TC173952 | TC94405, TC125305, TC126125, TC154847, TC158381 |
TM6 (TDR6) | X60759, Y098734 | TC170006 | TC90108, TC117238, TC154953 |
RIN | AF448522 | TC169998 | TC91451, TC126704, TC164766 |
CNR | DQ672601 | TC190465 | TC125032, TC162962 |
NOR | AY573802 | TC169950 | TC149932, TC117875, TC155746 |
VAHOX1 | X94947 | TC170121 | TC124192/TC94020, TC154081 |
LeYAB2 | N/A | TC172848, TC170528 | TC89502, TC124135, TC154049 |
LeCOR413-PM1 | AW039062 | TC172546 | TC85295, TC116832, TC154633 |
LeEF1-α | X14449, X53043 | TC169926, TC172095 | TC98347, TC123771, TC161869 |
Gene | GenBank accession | Current TC number | Previous TC numbers |
PSY1 | M84744 | TC171370, TC178429, TC169935 | TC84644, TC115970, TC115971, TC116252, TC115968, TC153853, TC153852, TC153854 |
PSY2 | L23424 | TC 183738 | TC120273, TC158116 |
LeAP2FL | N/A | TC180296 | TC154703 |
LeAP2FR | N/A | TC172597, TC178186 | TC85031, TC124194, TC124195, TC162117 |
TAG1 | L26295, AY098733 | TC170486, TC190126 | TC89786, TC124766, TC154658 |
TM4 (TDR4) | X60757, AY098732, AY306155 | TC177492, TC173952 | TC94405, TC125305, TC126125, TC154847, TC158381 |
TM6 (TDR6) | X60759, Y098734 | TC170006 | TC90108, TC117238, TC154953 |
RIN | AF448522 | TC169998 | TC91451, TC126704, TC164766 |
CNR | DQ672601 | TC190465 | TC125032, TC162962 |
NOR | AY573802 | TC169950 | TC149932, TC117875, TC155746 |
VAHOX1 | X94947 | TC170121 | TC124192/TC94020, TC154081 |
LeYAB2 | N/A | TC172848, TC170528 | TC89502, TC124135, TC154049 |
LeCOR413-PM1 | AW039062 | TC172546 | TC85295, TC116832, TC154633 |
LeEF1-α | X14449, X53043 | TC169926, TC172095 | TC98347, TC123771, TC161869 |
The TC number in the fourth column is the older number used in previous papers, and the third column is the most recent TIGR database accession number. Underlined TCs are used in the text in case of multiple current accessions. N/A, none available in the non-redundant GenBank database.
Results and discussion
1-MCP treatment
During ripening tomato fruit tissue becomes soft, accumulates certain sugars and acids, chloroplasts change to lycopene-accumulating chromoplasts, and evolution of ethylene and flavour volatiles occurs (Gray et al., 1992). In a cool-temperature, in vitro culture system, VFNT Cherry tomato sepals change their developmental programme to that of fruit (Ishida, 1991). During sepal morphogenesis at cool temperatures, 16–22 °C, sepals swell, lose their chlorophyll, accumulate lycopene, produce ethylene, change their sugar content, emit flavour volatiles associated with ripening tomato fruit, and their cell walls break down (Ishida, 1991). However, during in vitro culture at 28 °C these changes do not occur.
Hoeberichts et al. (2002) showed that mature green tomato fruit treated with 150 nl l−1 1-MCP for 20 h resulted in delayed softening, colour development (lycopene accumulation), ethylene evolution, and suppression of transcription of PSY1. Opiyo and Ying (2005) established 0.11 μl l−1 (110 ppb) as the point of saturation of ethylene receptors in mature green cherry tomato fruit. In the present experiment, sepals cultured at 16 °C and treated with 300 ppb 1-MCP remained green throughout the 35 d of in vitro culture. By contrast, untreated control sepals cultured at 16 °C became red within 35 d (Fig. 1). Ethylene evolution was measured in control (red) and treated sepals (green) and it was found that treatment with 1-MCP decreased evolution of ethylene to approximately 10% that of the control (data not shown). The steady-state mRNA levels of various transcription factors shown by Bartley and Ishida (2003) to increase during ripening and cool temperature in vitro culture of sepals were analysed by relative and real-time RT-PCR for evidence of regulation by ethylene (Fig. 2, Table 2). These transcription factors and one putative G-protein-coupled-receptor (GPCR), LeCOR413-PM1, were chosen because of their previously discovered up-regulation during cool temperature culture or high expression during tomato fruit ripening (Bartley and Ishida, 2002, 2003).
Gene expression analysis
Through database mining and RT-PCR, Bartley and Ishida (2003) showed up-regulation of a number of transcription factors and carotenoid biosynthesis genes during cool temperature sepal morphogenesis and tomato fruit ripening. In those studies, transcripts for PSY1, TAG1, TM4, RIN, an APETELA2-like (LeAP2FR) fruit predominant transcription factor (TC172597), and a putative GPCR LeCOR413-PM1 (TC172546) all increased dramatically in abundance during cool temperature sepal morphogenesis, but not significantly at 28 °C, except RIN which increased at both temperatures, and all increased during tomato fruit ripening. However, in that study, it was not determined whether these genes were induced independently by cool temperature or because of the concurrent evolution of ethylene. In the present study, as expected, the positive control gene, PSY1, an enzyme that catalyses the formation of phytoene, a colourless precursor to the carotenoid lycopene, was up-regulated within 29 d of culture in untreated sepals, but only slightly in 1-MCP-treated sepals (Fig. 2). Similarly, the AP2-like, LeAP2FR, putative ripening-related transcription factor, TC172597, also increased dramatically in control sepals, but not in treated sepals at 29 d. APETALA2 (AP2) in Arabidopsis is a B function gene according to the ABC model of floral development. AP2 negatively regulates AGAMOUS (AG) in sepals and petals (Bowman et al., 1991). Previously two AP2-like transcripts, TC172597 (LeAP2FR) and TC180296 (LeAP2FL) (flower predominant), were identified, and induction of LeAP2FR during cool-temperature culture and ripening of tomato fruit was shown (Bartley and Ishida, 2002, 2003). In tomato, perhaps this AP2 gene has gained additional functions. The present results indicate ethylene regulation of LeAP2FR (Table 2, Fig. 2). Of the MADS-box genes analysed, TAG1 and TM4 were up-regulated in the control sepals. The high induction of TAG1 and TM4 in untreated sepals and much lower expression in sepals treated with 1-MCP indicates ethylene involvement in the regulation of these genes. Real-time RT-PCR confirmed these results (Table 2). TM6 arose from a gene duplication event in the APETALA3 (AP3) gene lineage and diverged functionally from the AP3 function in floral development (Kramer et al., 1998; de Martino et al., 2006). In Arabidopsis, the MADS-box gene AP3 plays a key role in petal and stamen formation. TM6 is induced in cool temperature-grown tomato sepals (Bartley and Ishida, 2003). In this experiment, TM6 transcripts, as shown by RT-PCR, are more abundant in the untreated sepals at 29 d of culture, but appear to be less abundant in the untreated sepals at 35 d of culture (Fig. 2). Real-time PCR showed clear up-regulation of expression of TM6 by cool temperature but the difference in expression, 1.6-fold, of TM6 in untreated sepals at 29 d is not conclusive for ethylene involvement (Table 2). During tomato fruit ripening, TM6 clearly shows a unique pattern of expression, high in mature green tomato fruit and declining during ripening (Bartley and Ishida, 2002, 2003). TAG1, TM4, and TM6 are also induced in tomato flowers during cold-temperature (17 °C day, 7 °C night) growth of tomato plants causing abnormal flower formation (Lozano et al., 1998). However, ethylene involvement in this process was not investigated in that experiment. In view of the present results, it seems possible that ethylene may also have a role in cool-temperature induction of TAG1 and TM4 in tomato flowers.
The data in the present study and previous experiments indicate that the MADS-box gene, RIN, has both a developmental and ethylene component to its expression profile (Table 2, Fig. 2; Vrebalov et al., 2002). In those previous experiments (Bartley and Ishida, 2003), RIN was clearly induced at 28 °C while other MADS-box genes were either not induced or were only slightly induced. Indeed, in the present RT-PCR analysis, while RIN was induced in untreated sepals, it was also induced, but to a lesser degree, in 1-MCP-treated sepals (Fig. 2). Real-time PCR indicated possible ethylene involvement in RIN regulation (Table 2). This ethylene involvement may require factors that were not present in mature green fruit exposed to ethylene by Vrebalov et al. (2002). Additional experiments are needed to confirm this possible ethylene involvement.
The ripening regulator NOR (NON-RIPENING), a NAC (NO APICAL MERISTEM (NAM)/ATAF1/2/CUC2) domain transcription factor, was recently cloned (Giovannoni et al., 2004). A mutation in this gene causes a reduction in tomato fruit ripening similar to the rin mutation (Tigchelaar et al., 1978). Real-time RT-PCR results indicate NOR is affected by blocking ethylene perception (Table 2). Another ripening regulator, CNR (COLORLESS NON-RIPENING), an SBP-box (SQUAMOSA promoter-binding protein-like) gene, was also recently cloned (Manning et al., 2006). An epigenetic mutation in CNR results in colourless fruits (Manning et al., 2006). RIN is highly up-regulated in the mutant cnr but TM4(TDR4) is very reduced (Eriksson et al., 2004). Although Giovannoni (2004) suggested that CNR is downstream of or in a different regulatory network from RIN, not enough is known to draw definite conclusions. Expression of CNR appears similar to RIN (Tables 2, 4, Fig. 2).
Gene and family | Accession number | In vitro sepals | Fruit ripening | ||
16 °C | 28 °C | Ethylene | |||
MADS box | |||||
TAG1 | L26295 | +++ | No effect | +++ | +++ |
TM4 | X60757 | +++ | + | +++ | +++ |
TM6 | X60759 | ++ | No effect | + | – |
RIN | AF448522 | + | +++ | + | +++ |
SBP box | |||||
CNR | DQ672601 | + | +++a | + | +++ |
NAC domain | |||||
NOR | AY573802 | ++ | ++ a | +++ | +++ |
AP2 domain | |||||
LeAP2FR | TC172597 | +++ | + | +++ | +++ |
Homeobox | |||||
VAHOX1 | X94947 | ++ | + a | No effect | +++ |
Zinc finger | |||||
LeYAB2 | TC172848 | ++ | + | No effect | +++ |
GPCR | |||||
LeCOR413-PM1 | X14449 | +++ | + | +++ | +++ |
Gene and family | Accession number | In vitro sepals | Fruit ripening | ||
16 °C | 28 °C | Ethylene | |||
MADS box | |||||
TAG1 | L26295 | +++ | No effect | +++ | +++ |
TM4 | X60757 | +++ | + | +++ | +++ |
TM6 | X60759 | ++ | No effect | + | – |
RIN | AF448522 | + | +++ | + | +++ |
SBP box | |||||
CNR | DQ672601 | + | +++a | + | +++ |
NAC domain | |||||
NOR | AY573802 | ++ | ++ a | +++ | +++ |
AP2 domain | |||||
LeAP2FR | TC172597 | +++ | + | +++ | +++ |
Homeobox | |||||
VAHOX1 | X94947 | ++ | + a | No effect | +++ |
Zinc finger | |||||
LeYAB2 | TC172848 | ++ | + | No effect | +++ |
GPCR | |||||
LeCOR413-PM1 | X14449 | +++ | + | +++ | +++ |
This table is a compilation of data from this study, data-mining of EST databases, and the following references: Bartley and Ishida (2002, 2003); Giovannoni et al. (2004); Manning et al. (2006). The number of plus/up (+) signs or minus/down (–) signs represents the relative strength of expression.
RT-PCR not shown.
Gene and family | Accession number | In vitro sepals | Fruit ripening | ||
16 °C | 28 °C | Ethylene | |||
MADS box | |||||
TAG1 | L26295 | +++ | No effect | +++ | +++ |
TM4 | X60757 | +++ | + | +++ | +++ |
TM6 | X60759 | ++ | No effect | + | – |
RIN | AF448522 | + | +++ | + | +++ |
SBP box | |||||
CNR | DQ672601 | + | +++a | + | +++ |
NAC domain | |||||
NOR | AY573802 | ++ | ++ a | +++ | +++ |
AP2 domain | |||||
LeAP2FR | TC172597 | +++ | + | +++ | +++ |
Homeobox | |||||
VAHOX1 | X94947 | ++ | + a | No effect | +++ |
Zinc finger | |||||
LeYAB2 | TC172848 | ++ | + | No effect | +++ |
GPCR | |||||
LeCOR413-PM1 | X14449 | +++ | + | +++ | +++ |
Gene and family | Accession number | In vitro sepals | Fruit ripening | ||
16 °C | 28 °C | Ethylene | |||
MADS box | |||||
TAG1 | L26295 | +++ | No effect | +++ | +++ |
TM4 | X60757 | +++ | + | +++ | +++ |
TM6 | X60759 | ++ | No effect | + | – |
RIN | AF448522 | + | +++ | + | +++ |
SBP box | |||||
CNR | DQ672601 | + | +++a | + | +++ |
NAC domain | |||||
NOR | AY573802 | ++ | ++ a | +++ | +++ |
AP2 domain | |||||
LeAP2FR | TC172597 | +++ | + | +++ | +++ |
Homeobox | |||||
VAHOX1 | X94947 | ++ | + a | No effect | +++ |
Zinc finger | |||||
LeYAB2 | TC172848 | ++ | + | No effect | +++ |
GPCR | |||||
LeCOR413-PM1 | X14449 | +++ | + | +++ | +++ |
This table is a compilation of data from this study, data-mining of EST databases, and the following references: Bartley and Ishida (2002, 2003); Giovannoni et al. (2004); Manning et al. (2006). The number of plus/up (+) signs or minus/down (–) signs represents the relative strength of expression.
RT-PCR not shown.
Homeobox genes are involved in a number of developmental processes in plants (Chan et al., 1998). Leucine zipper-containing homeobox genes are specific to plants. One class I homeobox domain leucine-zipper (HD-ZIP) gene, VAHOX1, is highly expressed in tomato fruit and up-regulated in cool-temperature, in vitro culture, but is not affected by ripening-related ethylene accumulation (Tables 2, 4) (Bartley and Ishida, 2002). VAHOX1 is expressed in phloem tissue of tomato during phases of secondary growth in more mature areas of the stem and root where secondary vascularization and lignification occur (Tornero et al., 1996). Indeed, analysis of EST occurrence in various libraries in the TIGR Tomato Gene Index shows the highest occurrence of VAHOX1 (TC170121) in nutrient-deficient roots, 0.19% and 0.03% in roots at fruit set (Quackenbush et al., 2000). Interestingly, the next highest occurrence of VAHOX1 transcripts is 0.18% in breaker-stage fruits In addition, cDNA microarray results located in the Tomato Expression Database (TED) (http://ted.bti.cornell.edu/cgi-bin/array/profile.cgi?arrayID=1–1–8.4.17.12&cultivar=1) (Fei et al., 2006) reveal an expression profile indicative of ripening association. The present real-time PCR experiments indicate a nearly 2-fold increase in VAHOX1 transcript during cold-induced sepal transformation. However, control and treated sepals at 29 d of culture show little difference in expression, indicating lack of ethylene involvement (Table 2, Fig. 2). If VAHOX1 is necessary for vascularization, high expression during early stages of tomato fruit development is not surprising. However, expression during breaker and red ripe stages of tomato fruit may indicate other functions for this transcription factor. Recently, in pepper (Capsicum annuum L.), an HD-ZIP protein was found to be associated with the cold-inducible (4 °C) transcription factor, CaCBF1B (Kim et al., 2004).
A putative zinc-finger transcription factor, a YABBY2-like gene (LeYAB2) TC172848, was investigated in the present study. YABBY family genes are expressed in a polar manner in lateral organs of Arabidopsis, soybean (Glycine max), snapdragon (Antirrhinum majus), and tomato (Siegfried et al., 1999; Kim et al., 2003; Thibaud-Nissen et al., 2003; Golz et al., 2004; Zhang et al., 2004). In Arabidopsis, YABBY family genes specify abaxial cell fate in all lateral organs (Siegfried et al., 1999). One member of the YABBY family, CRABS CLAW, is expressed in and promotes abaxial cell fate of cells of the gynoecium in Arabidopsis (Bowman and Smyth, 1999). In tomato, the YABBY-B gene was cloned using the Arabidopsis YABBY gene, INNER NO OUTER (Kim et al., 2003). YABBY-B is expressed in abaxial regions of the leaf primordium (Kim et al., 2003). LeYAB2 (TC172848) was chosen previously for its high expression in pericarp during tomato ripening (Bartley and Ishida, 2002). Gene expression of LeYAB2 was previously found to increase during cool-temperature (16 °C), in vitro culture but not at 28 °C and also increased in breaker/turning-stage fruit (Bartley and Ishida, 2003). Indeed, this gene was induced after 2 d exposure to 16 °C during in vitro culture, but TAG1, TM4, and LeAP2FR were not. The expression of LeYAB2 appeared nearly identical in both untreated and treated sepals (Fig. 2). Real-time PCR results indicate cool temperature regulation but not significant ethylene involvement in the regulation of this gene (Table 2). It is possible that this gene may determine the abaxial fate of pericarp cells, but it may also function in ripening.
LeCOR413-PM1 (TC172546) is a transcript that codes for a protein very similar to WCOR413, a low temperature-induced protein in wheat (Triticum aestivum) (Allard et al., 1998). The function of this protein is unknown, but sequence analysis indicates several transmembrane helices, suggesting that it stabilizes the plasma membrane during cold stress (Allard et al., 1998). Recently, Breton et al. (2003), by using bioinformatics and signal peptide prediction software, proposed that the WCOR413 family of proteins including LeCOR413-PM1 may be membrane-bound GPCRs located in the plasma (-PM1, -PM2) or thylakoid (-TM1) membranes. They further suggest that these proteins are plant specific and are associated with environmental stress signalling and cold acclimation. These authors found the barley (Hordeum vulgare) and rye (Secale cereale) WCOR413-PM1 homologues were also induced by cold acclimation and the Arabidopsis thaliana homologue was induced by cold, dehydration, and abscisic acid (Breton et al., 2003). However, the ligand for these putative receptors remains unknown. Garwe et al. (2003) recently cloned the same gene from the resurrection plant (Xerophyta viscosa Baker) and investigated its regulation. This gene, XVSAP1, was induced by heat (42 °C), salt (100 mM NaCl), high light, and cold (4 °C). The WCOR413 family in tomato consists of three known members, LeCOR413-PM1 (TC172546, GenBank AW039062), LeCOR413-PM2 (TC174333, GenBank BG642925), and LeCOR413-TM1 (TC177286, GenBank AW034114) (Breton et al., 2003). In animals, GPCRs are involved in recognizing and transducing messages such as light, Ca2+, odorants, amino acids, nucleotides, peptides, and proteins (Bockaert and Pin, 1999). It has been shown that LeCOR413-PM1 was highly induced during cool-temperature, in vitro culture and during tomato fruit ripening (Bartley and Ishida, 2003). In the previous in vitro experiment LeCOR413-PM1 was only slightly induced at 28 °C, indicating little if any developmental regulation. LeCOR413-PM1 shows an ethylene-induced expression pattern (Fig. 2, Table 2). However, a cold-induced or ethylene-independent component to this regulation is also indicated by an increase in expression even in 1-MCP-treated sepals from 5 d to 29 d of culture (Fig. 2). Real-time PCR confirmed ethylene involvement in the regulation of this gene. Therefore, this gene might participate in a signalling pathway during cool-temperature sepal morphogenesis and during tomato fruit development, but further investigation is needed.
In conclusion, a number of putative regulatory genes are affected either directly or indirectly by ethylene during cool-temperature sepal morphogenesis. The results of the present study indicate ethylene involvement in regulation of certain transcription factors and one putative GPCR during cool temperature-induced ripening of tomato sepals. The positive control PSY1 is regulated by ethylene (Maunders et al., 1987), but this correlation with LeAP2FR, TAG1, TM4, or LeCOR413-PM1 has not previously been made. This correlation with ethylene reception may indicate the importance of these transcripts in ripening. Considering the similarity in expression of PSY1 and these transcription factors in treated and untreated sepals, it is possible one (or more) of these genes has a more specific influence on carotenoid production. Ectopic expression of TAG1 in transgenic plants caused sepals to become fruit-like (Pnueli et al., 1994). RIN, CNR, and NOR are all induced even in treated sepals but these sepals do not complete the sepal ripening process, i.e. lycopene accumulation, indicating the need for a functional ethylene reception system as in fruit. In addition, two ethylene-independent, cool-temperature-regulated transcripts, VAHOX1 and LeYAB2, have been found. Both of these genes are induced early at 16 °C by 2 d in a study involving cool temperature versus 28 °C in vitro culture (Bartley and Ishida, 2003; GE Bartley and BK Ishida, unpublished data). However, it is still unknown whether VAHOX1 and LeYAB2 are initiators in this process. It has also been shown that two transcripts, LeCOR413-PM1 and TM6, are affected by both ethylene and cool-temperature. Although the function of LeCOR413-PM1 is not known, this type of regulation during ripening and cool-temperature in vitro culture of sepals may indicate the importance of this gene. Interestingly, the present results also reveal that ripening regulators RIN and CNR are affected slightly by blocking ethylene perception but NOR expression is highly affected (Fig. 2, Tables 2, 4). Further investigation is required to assess fully the relationship of the components of the signalling pathways involved in cool-temperature sepal morphogenesis and tomato fruit ripening. However, elucidation of function of particular genes may be difficult in some cases. Transgenic plants constructed using the TM4 gene in antisense orientation showed no obvious fruit phenotype (Eriksson et al., 2004). This result may indicate possible redundancy of MADS-box genes in tomato fruit. Dissecting and understanding the ripening process will lead to more precise engineering of nutrient accumulation in tomato fruit in the future.
Abbreviations
- EST
expressed sequence tag
- GPCR
G-protein-coupled receptor
- 1-MCP
1-methylcyclopropene
- TCs
tentative consensus sequences
The authors would like to thank Drs Grace Chen, Yong-sic Hwang, Christopher Mau, and Dominic Wong for their critical analysis of the manuscript. The authors would also like to thank Dr HL Warner (Rohm and Haas Company) for the gift of ETHYLBLOC™.
Comments