-
PDF
- Split View
-
Views
-
Cite
Cite
Lenka Polanská, Anna Vičánková, Marie Nováková, Jiří Malbeck, Petre I. Dobrev, Břetislav Brzobohatý, Radomíra Vaňková, Ivana Macháčková, Altered cytokinin metabolism affects cytokinin, auxin, and abscisic acid contents in leaves and chloroplasts, and chloroplast ultrastructure in transgenic tobacco, Journal of Experimental Botany, Volume 58, Issue 3, February 2007, Pages 637–649, https://doi.org/10.1093/jxb/erl235
- Share Icon Share
Abstract
Cytokinins (CKs) are involved in the regulation of plant development including plastid differentiation and function. Partial location of CK biosynthetic pathways in plastids suggests the importance of CKs for chloroplast development. The impact of genetically modified CK metabolism on endogenous CK, indole-3-acetic acid, and abscisic acid contents in leaves and isolated intact chloroplasts of Nicotiana tabacum was determined by liquid chromatography/mass spectrometry and two-dimensional high-performance liquid chromatography, and alterations in chloroplast ultrastructure by electron microscopy. Ectopic expression of Sho, a gene encoding a Petunia hybrida isopentenyltransferase, was employed to raise CK levels. The increase in CK levels was lower in chloroplasts than in leaves. CK levels were reduced in leaves of tobacco harbouring a CK oxidase/dehydrogenase gene, AtCKX3. The total CK content also decreased in chloroplasts, but CK phosphate levels were higher than in the wild type. In a transformant overexpressing a maize β-glucosidase gene, Zm-p60.1, naturally targeted to plastids, a decrease of CK-O-glucosides in chloroplasts was found. In leaves, the changes were not significant. CK-O-glucosides accumulated to very high levels in leaves, but not in chloroplasts, of plants overexpressing a ZOG1 gene, encoding trans-zeatin-O-glucosyltransferase from Phaseolus lunatus. Manipulation of the CK content affected levels of indole-3-acetic and abscisic acid. Chloroplasts of plants constitutively overexpressing Sho displayed ultrastructural alterations including the occasional occurrence of crystalloids and an increased number of plastoglobuli. The other transformants did not exhibit any major differences in chloroplast ultrastructure. The results suggest that plant hormone compartmentation plays an important role in hormone homeostasis and that chloroplasts are rather independent organelles with respect to regulation of CK metabolism.
Introduction
The plant hormones cytokinins (CKs) are known to be involved in many processes related to plastid development and functions. Within 10 years of the discovery of kinetin (6-furfurylaminopurine) as a plant growth regulator CK (Miller et al., 1956) in cultured tobacco tissue, it was shown that kinetin could stimulate chloroplast differentiation from proplastids, including grana formation (Stetler and Laetsch, 1965). Kinetin was also found to almost double chloroplast number per cell in etiolated tobacco leaf discs incubated in the light (Boasson and Laetsch, 1969).
It has been demonstrated that exogenously applied CKs induce partial development of chloroplasts from proplastids, amyloplasts, and etioplasts, especially in darkness (Chory et al., 1994; Kusnetsov et al., 1994). For example, benzyladenine (BA) treatment of excised watermelon cotyledons accelerated degradation of reserve material and differentiation of plastids with a more developed inner membrane system, as well as increased levels of plastid pigments and enzymes (Longo et al., 1979). Moreover, applied BA accelerated the redevelopment of grana and stroma in regreening plastids, the increase of reappeared NADPH-protochlorophyllide oxidoreductase, and the contents of chlorophyll and protein in regreening tobacco leaves (Zavaleta-Mancera et al., 1999a, b).
The action of CKs in plastids seems to be mediated by the stimulation of the expression of several plastid-related genes, of both nuclear and plastid origin, in particular that of the nuclear gene for chlorophyll a/b-binding polypeptide of the light-harvesting complex and the gene coding for the small subunit of Rubisco in Nicotiana tabacum (Abdelghani et al., 1991), Arabidopsis (Chory et al., 1994), or Dianthus caryophyllus (Winiarska et al., 1994). For extensive reviews, see Schmülling et al. (1997) or Parthier (1989).
Recently, Brenner et al. (2005) identified by genome-wide expression profiling five rapidly CK-induced plastid transcripts in Arabidopsis seedlings, indicating a fast transfer of the CK signal to plastids or its direct perception there. The CK effect on gene expression may be mediated via the hormone interaction with specific proteins. In the presence of trans-zeatin, a 64 kDa chloroplast zeatin-binding protein was found to activate the chloroplast but not the nuclear genome (Lyukevich et al., 2002).
The occurrence of endogenous CKs in plastids has been proven. CKs are present in chloroplast tRNAs (Vreman et al., 1978). Zeatin-type CKs were detected in isolated spinach chloroplasts by paper chromatography and soybean callus bioassay (Davey and Van Staden, 1981). Immunolocalization of CKs revealed low labelling in the stroma of plastids of Tilia cordata embryo cotyledons and roots (Kärkönen and Simola, 1999). Eventually, CK occurrence in chloroplasts was rigorously confirmed by LCMS (liquid chromatography/mass spectrometry) which allows identification of a whole range of CK metabolites with high accuracy (Benková et al., 1999).
The importance of CKs for plastid development and function may be deduced from the partial localization of the CK biosynthetic pathway to this compartment. Kasahara et al. (2004) showed that the prenyl group of CKs may be synthesized in plastids by the methylerythritol phosphate pathway and that four isopentenyltransferases (IPTs) are localized to plastids in Arabidopsis cells. Unexpectedly, Sakakibara et al. (2005) have found that Agrobacterium IPT functions in plastids, even though it lacks a typical plastid-targeting sequence, and it prefers a substrate different from that of genuine plant IPTs. It was previously shown that the enhanced content of CKs in chloroplasts of tobacco plants overexpressing the A. tumefaciens ipt gene driven by a light-inducible promoter of a Pisum sativum small subunit of Rubisco (Pssu-ipt) correlated with highly anomalous chloroplast ultrastructure (Synková et al., 2006). Interestingly, although a clear increase in CKs was observed in chloroplasts, it remained about an order of magnitude lower than the levels found in whole leaf extracts.
In the present study, the impact of manipulation of CK metabolism on the endogenous CK pool in leaves and isolated intact chloroplasts, and on chloroplast ultrastructure in tobacco (Nicotiana tabacum L.) was evaluated. CK-overproducing plants (expressing the Sho gene coding for a Petunia hybrida IPT), plants with decreased CK levels (overexpressing CK oxidase/dehydrogenase AtCKX3 from Arabidopsis thaliana), and plants with altered CK glucoconjugation (overexpressing maize β-glucosidase Zm-p60.1 or zeatin-O-glucosyltransferase ZOG1 from Phaseolus lunatus) were compared. The simplified model of CK metabolism with highlighted enzymes involved in this study is presented in Fig. 1. Taking into account the immense cross-talk among plant hormones, the contents of auxin (IAA) and abscisic acid (ABA) were also determined.
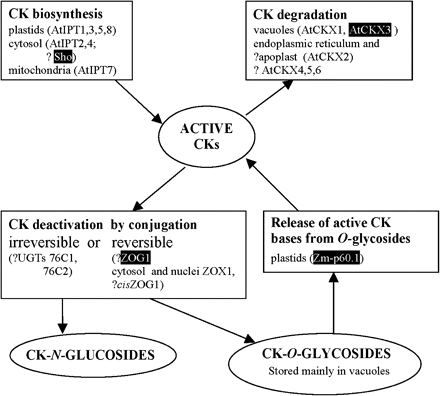
Simplified model of CK metabolism including known localization of examples of involved enzymes proved by in planta experiments. The enzymes employed in this study are highlighted. AtCKX, Arabidopsis CK oxidase/dehydrogenase (Werner et al., 2003); AtIPT, Arabidopsis isopentenyltransferase (Kasahara et al., 2004); Sho, petunia isopentenyltransferase (Zubko et al., 2002); UGT, Arabidopsis N-glucosyltransferase (Hou et al., 2004); Zm-p60.1, maize β-glucosidase (Kristoffersen et al., 2000); ZOG1, bean trans-zeatin-O-glucosyltransferase (Martin et al., 2001a); cisZOG, maize cis-zeatin-O-glucosyltransferase (Martin et al., 2001b); ZOX1, bean zeatin-O-xylosyltransferase (Martin et al., 1993); ?, unknown or uncertain localization. The interconversions among iP-, transZ-, cisZ-, and DHZ-type CKs and their bases, ribosides, and phosphates, CK transport and tissue localization are not depicted. For more details see Sakakibara (2006).
Materials and methods
Plant materials and growth conditions
Samples of mature leaves from four different types of transgenic tobacco plants with altered CK metabolism and the corresponding wild types (WTs), all in the vegetative stage, collected imediately after the dark period, were used.
1. Tobacco in vitro lines expressing an IPT homologue Sho (Shooting) gene from P. hybrida under the control of a constitutive cauliflower mosaic virus (CaMV) 35S promoter or construct with four 35S enhancer elements (Zubko et al., 2002) and WT N. tabacum L. cv. Petit Havana SR1 cultivated on solid hormone-free MS medium (Murashige and Skoog, 1962) supplemented with sucrose (30 g l−1). To achieve inducible Sho expression, a dexamethasone-inducible gene expression system (pOp6/LhGR; Šámalová et al., 2005) was employed. Sho expression was induced in 8–9-week-old transgenic plants with 20 μM dexamethasone [in 0.05% dimethylsulphoxide (DMSO), 5×50 ml per plant watered for 13 d]. As controls, transgenic plants treated with water or 0.05% DMSO and WT SR1 plants treated with water, 0.05% DMSO, or 20 μM dexamethasone in 0.05% DMSO were used. The plants were cultivated in a growth chamber (16/8 h photoperiod at 130 μE m−2 s−1, day/night temperature of 25/23 °C and relative humidity ∼80%) for 5 weeks and then transferred, before Sho induction, to a greenhouse (in summer, without additional light supplementation).
All the other plants were cultivated in a soil substrate in a growth chamber (16/8 h photoperiod at 150 μE m−2 s−1, 26/20 °C and relative humidity ∼80%).:
2. Eleven-week-old tobacco plants (35S:AtCKX3) overexpressing a gene for CK oxidase/dehydrogenase from A. thaliana under a constitutive CaMV 35S promoter (Werner et al., 2001) and 9-week-old WT N. tabacum L. cv. Samsun NN.
3. Eight- to 9-week-old tobacco plants (35S:P60) overexpressing a maize β-glucosidase Zm-p60.1 naturally targeted to plastids under a CaMV 35S promoter (Kiran et al., 2006) and WT Nicotiana tabacum L. cv. Petit Havana SR1.
4. Eight- to 9-week-old tobacco plants (35S:ZOG1) harbouring a trans-zeatin-O-glucosyltransferase gene from P. lunatus under a constitutive CaMV 35S promoter (Martin et al., 2001a) and WT N. tabacum L. cv. Wisconsin 38.
Chloroplast isolation
Intact chloroplasts were isolated and purified as described by Kiran et al. (2006). The chloroplast fraction was recovered from the homogenate of deribbed leaves in homogenization medium [0.33 M sorbitol; 50 mM TRIS–HCl, pH 7.8; 0.4 mM KCl; 0.04 mM Na2EDTA; 0.1% (w/v) bovine serum albumin; 1% (w/v) polyvinylpyrrolidone; 5 mM isoascorbic acid] by centrifugation, layered on a Percoll density gradient [40% and 80% (v/v) Percoll solution in resuspension medium (0.33 M sorbitol; 2 mM Na2EDTA; 1 mM MgCl2; 1 mM MnCl2; 50 mM HEPES, pH 7.6)] and centrifuged (1000 g; 15 min; 4 °C). Intact chloroplasts were collected at the interface of the gradient, diluted with medium, and centrifuged (1000 g; 2 min; 4 °C). The pellet was resuspended in medium. All the procedures were done at 4 °C.
Chlorophyll determination
Chlorophyll was extracted into 80% (v/v) acetone. The total chlorophyll content (a+b) was calculated from the absorbance at 652 nm of the clear extract after centrifugation (500 g, 5 min) according to Arnon (1949).
Chloroplast integrity
The integrity of chloroplasts was determined by the latency of glyceraldehyde-3-phosphate dehydrogenase according to Latzko and Gibbs (1968). Chloroplasts were incubated in a reaction mixture (0.33 M TRIS–HCl, pH 8.5; 17 mM Na2HAsO4·7H2O; 4 mM cysteine; 20 mM NaF; 40 μM NADP+). The reaction was initiated with 0.02 M glyceraldehyde-3-phosphate. Reduction of NADP+ was followed at 340 nm for 5 min. The same assay was run with chloroplasts disrupted with 0.01 M MgCl2. The percentage of intact chloroplasts was calculated from the difference between the original and disrupted sample.
Extraction and purification of IAA, ABA, and CK
The detailed procedure for hormone extraction, purification, and quantification has been described in Kiran et al. (2006). IAA, ABA, and CKs were extracted overnight at –20 °C with Bieleski solvent (Bieleski, 1964). [3H]IAA and [3H]ABA (Sigma, USA) and 12 deuterium-labelled CKs ([2H5]t-Z, [2H5]t-ZR, [2H5]t-Z7G, [2H5]t-Z9G, [2H5]t-ZOG, [2H5]t-ZROG, [2H3]DHZ, [2H3]DHZR, [2H6]iP, [2H6]iPR, [2H6]iP7G, and [2H6]iP9G; Apex Organics, UK) were added as internal standards. The extracts were purified using Sep-Pak C18 cartridges (Waters Corporation, Milford, MA, USA) and an Oasis MCX mixed mode, cation exchange, reverse-phase column (150 mg, Waters) (Dobrev and Kamínek, 2002). After a wash with 1 M HCOOH, IAA and ABA were eluted with 100% MeOH and evaporated to dryness. Further, CK phosphates were eluted with 0.34 M NH4OH in water, and CK bases, ribosides, and glucosides were eluted with 0.34 M NH4OH in 60% (v/v) MeOH. Phosphates were converted to ribosides with alkaline phosphatase. IAA and ABA were separated and quantified by two-dimensional high-performance liquid chromatography (2D-HPLC) according to Dobrev et al. (2005). Purified CK samples were analysed by an LCMS system consisting of an HTS PAL autosampler (CTC Analytics, Switzerland), Rheos 2000 quaternary pump (FLUX, Switzerland) with Csi 6200 Series HPLC oven (Cambridge Scientific Instruments, UK), and an LCQ Ion Trap mass spectrometer (Finnigan, USA) equipped with an electrospray. A 10 μl aliquot of sample was injected onto a C18 column (AQUA, 2 mm×250 mm×5 μm, Phenomenex, USA) and eluted with 0.0005% acetic acid (A) and acetonitrile (B). The HPLC gradient profile was as follows: 5 min 10% B, then increasing to 17% for 10 min, and to 46% for a further 10 min at a flow rate of 0.2 ml min−1. The column temperature was kept at 30 °C. The effluent was introduced into a mass spectrometer being operated in the positive ion, full-scan MS/MS mode. Quantification was performed using a multilevel calibration graph with deuterated CKs as internal standards. Plant hormone content is usually presented in picomoles per gram fresh weight (FW) of plant tissue. Here, hormone concentration is also expressed per unit of chlorophyll, allowing the comparison with hormone levels in isolated chloroplasts. The differences were estimated according to the value of the standard error.
Transmission electron microscopy
Leaf blade segments (2 mm×2 mm×leaf thickness) were fixed for 2 h with 2.5% (v/v) glutaraldehyde in 0.1 M phosphate buffer, pH 7.2, followed by 2 h in 2% (w/v) osmic acid. After dehydration in a graded ethanol series up to 100% ethanol, samples were embedded via propylene oxide in low viscosity Spurr's resin. The ultrastructure was evaluated on transverse ultrathin sections of embedded objects contrasted with a saturated solution of uranyl acetate in 70% (v/v) aqueous ethanol, followed by a lead citrate solution treatment according to Reynolds (1963) using a transmission electron microscope (Philips Morgani) at an operating voltage of 70 kV.
Results
Hormone analyses
In order to investigate the role of hormone compartmentation and to study the interactions among plant hormones, the contents of CKs, free IAA, and ABA were determined in mature leaves and in isolated intact chloroplasts of four different types of transgenic tobacco with altered CK metabolism and in the corresponding WTs during the vegetative stage of plant development. The integrity of the analysed chloroplasts immediately after their isolation was >90%, as checked by the latency of the activity of the stromal enzyme glyceraldehyde-3-phosphate dehydrogenase.
Plants expressing isopentenyltransferase Sho
The total CK content in leaves after Sho induction by dexamethasone was eight times that in the corresponding WT (SR1) (Fig. 2A). The most elevated CK metabolites were phosphates (predominantly iPMP) and N-glucosides (especially iP7G). The level of physiologically active CK bases and ribosides (predominantly of iP and iPR) increased to a lesser extent. No significant difference in the content of CK-O-glucosides was observed. As CK levels did not differ significantly among WT plants treated with water, with dexamethasone in DMSO, or with DMSO solution or non-induced Sho gene-carrying plants, only the CK content of WT plants treated with water is shown. The CK elevation in induced Sho leaves was lower when the CK content was expressed per mg of chlorophyll, as the transgenics had a higher chlorophyll content than the WT (Fig. 2B). The difference between Sho transformants and the WT was much lower in isolated chloroplasts (Fig. 2C) than in leaves. Indeed, except for CK-O-glucosides, the percentage of leaf CKs sequestered in chloroplasts was lower in Sho compared with WT (see supplementary Table 1 available at JXB online). Nevertheless, the CK content of chloroplasts from plants expressing Sho was more than twice as high as that of the WT. The contents of individual CKs in leaves and isolated chloroplasts are given in supplementary Table 2 available at JXB online.
Endogenous contents of free IAA and ABA in leaves and in isolated intact chloroplasts of transgenic dexamethasone-induced Sho and WT (SR1) tobacco
Sho | WT (SR1) | |
IAA | ||
Leaves (pmol g−1 FW) | 90.7 (117.9; 63.5) | 71.2 (81.1; 61.3) |
Leaves (pmol mg−1 chlorophyll) | 73.7 (95.8; 51.6) | 74.2 (84.4; 63.9) |
Chloroplasts (pmol mg−1 chlorophyll) | 2.5 (2.8; 2.2) | 3.4 (2.8; 4.0) |
ABA | ||
Leaves (pmol g−1 FW) | 453.5 (749.1; 157.8) | 360.0 (628.1; 91.9) |
Leaves (pmol mg−1 chlorophyll) | 368.7 (609.0; 128.3) | 375.0 (654.3; 95.7) |
Chloroplasts (pmol mg−1 chlorophyll) | 16.8 (18.7; 14.9) | 12.8 (13.3; 12.2) |
Sho | WT (SR1) | |
IAA | ||
Leaves (pmol g−1 FW) | 90.7 (117.9; 63.5) | 71.2 (81.1; 61.3) |
Leaves (pmol mg−1 chlorophyll) | 73.7 (95.8; 51.6) | 74.2 (84.4; 63.9) |
Chloroplasts (pmol mg−1 chlorophyll) | 2.5 (2.8; 2.2) | 3.4 (2.8; 4.0) |
ABA | ||
Leaves (pmol g−1 FW) | 453.5 (749.1; 157.8) | 360.0 (628.1; 91.9) |
Leaves (pmol mg−1 chlorophyll) | 368.7 (609.0; 128.3) | 375.0 (654.3; 95.7) |
Chloroplasts (pmol mg−1 chlorophyll) | 16.8 (18.7; 14.9) | 12.8 (13.3; 12.2) |
Values represent the mean of two replicates (individual measurements are shown in parentheses).
Endogenous contents of free IAA and ABA in leaves and in isolated intact chloroplasts of transgenic dexamethasone-induced Sho and WT (SR1) tobacco
Sho | WT (SR1) | |
IAA | ||
Leaves (pmol g−1 FW) | 90.7 (117.9; 63.5) | 71.2 (81.1; 61.3) |
Leaves (pmol mg−1 chlorophyll) | 73.7 (95.8; 51.6) | 74.2 (84.4; 63.9) |
Chloroplasts (pmol mg−1 chlorophyll) | 2.5 (2.8; 2.2) | 3.4 (2.8; 4.0) |
ABA | ||
Leaves (pmol g−1 FW) | 453.5 (749.1; 157.8) | 360.0 (628.1; 91.9) |
Leaves (pmol mg−1 chlorophyll) | 368.7 (609.0; 128.3) | 375.0 (654.3; 95.7) |
Chloroplasts (pmol mg−1 chlorophyll) | 16.8 (18.7; 14.9) | 12.8 (13.3; 12.2) |
Sho | WT (SR1) | |
IAA | ||
Leaves (pmol g−1 FW) | 90.7 (117.9; 63.5) | 71.2 (81.1; 61.3) |
Leaves (pmol mg−1 chlorophyll) | 73.7 (95.8; 51.6) | 74.2 (84.4; 63.9) |
Chloroplasts (pmol mg−1 chlorophyll) | 2.5 (2.8; 2.2) | 3.4 (2.8; 4.0) |
ABA | ||
Leaves (pmol g−1 FW) | 453.5 (749.1; 157.8) | 360.0 (628.1; 91.9) |
Leaves (pmol mg−1 chlorophyll) | 368.7 (609.0; 128.3) | 375.0 (654.3; 95.7) |
Chloroplasts (pmol mg−1 chlorophyll) | 16.8 (18.7; 14.9) | 12.8 (13.3; 12.2) |
Values represent the mean of two replicates (individual measurements are shown in parentheses).
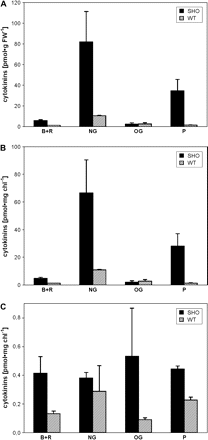
Cytokinin content (B+R, free bases and ribosides; NG, N-glucosides; OG, O-glucosides; P, phosphates) in leaves (per fresh weight A and per unit of chlorophyll B) and in isolated intact chloroplasts (C) of transgenic dexamethasone-induced Sho (SHO, black) and control SR1 (WT, striped) tobacco. Error bars display the SE.
On a FW basis, the higher CK content in Sho leaves compared with the WT was accompanied by a slightly higher content of IAA and ABA (Table 1). These differences were not significant when expressed per unit of chlorophyll. Chloroplasts of Sho plants had lower IAA, but higher ABA content than chloroplasts of WT plants.
Plants expressing CK oxidase/dehydrogenase AtCKX3
The constitutive overexpression of AtCKX3 significantly delayed development of transgenic plants; consequently, hormone contents and chloroplast ultrastructure were analysed in transgenic plants that were older than the corresponding WT (SNN) plants. The total CK content was reduced in leaves (Fig. 3A) to about one-half of that in the WT. Particularly altered were the levels of CK-N-glucosides and, to a lesser extent, bases and ribosides. The chlorophyll content was reduced in transgenic plants, so that the differences between transgenic and WT tobacco are reduced when expressed per unit of chlorophyll (Fig. 3B). The CK content was changed to a lesser extent in isolated intact chloroplasts (Fig. 3C). Further, when AtCKX3 and WT were compared, the percentage of leaf CK bases and ribosides remained almost unchanged, whilst those of phosphates sequestered in chloroplasts increased; the values for CK-glucosides dropped significantly (see supplementary Table 1 available at JXB online).

Cytokinin content (B+R, free bases and ribosides; NG, N-glucosides; OG, O-glucosides; P, phosphates) in leaves (per fresh weight A and per unit of chlorophyll B) and in isolated intact chloroplasts (C) of transgenic 35S:AtCKX3 (CKX, black) and control SNN (WT, striped) plants. Error bars display the SE.
The content of IAA was not significantly changed in AtCKX3 leaves (Table 2) compared with WT when expressed per FW, but it was higher when expressed per unit of chlorophyll. The ABA level was lower in leaves of transgenic tobacco. No significant changes of IAA or ABA levels were observed in chloroplasts (Table 2).
Endogenous contents of free IAA and ABA in leaves and in isolated intact chloroplasts of transgenic 35S:AtCKX3 and WT (SNN) tobacco
35S:AtCKX3 | WT (SNN) | |
IAA | ||
Leaves (pmol g−1 FW) | 125.0 (177.4; 72.6) | 122.1 (147.3; 96.9) |
Leaves (pmol mg−1 chlorophyll) | 200.5 (279.1; 121.8) | 114.4 (151.6; 77.2) |
Chloroplasts (pmol mg−1 chlorophyll) | 5.2 (4.3; 6.1) | 5.2 (9.9; 0.4) |
ABA | ||
Leaves (pmol g−1 FW) | 359.5 (447.7; 271.3) | 1102.3 (822.9; 1381.6) |
Leaves (pmol mg−1 chlorophyll) | 579.9 (704.4; 455.4) | 974.1 (847.2; 1100.9) |
Chloroplasts (pmol mg−1 chlorophyll) | 68.0 (44.0; 92.0) | 58.8 (106.6; 10.9) |
35S:AtCKX3 | WT (SNN) | |
IAA | ||
Leaves (pmol g−1 FW) | 125.0 (177.4; 72.6) | 122.1 (147.3; 96.9) |
Leaves (pmol mg−1 chlorophyll) | 200.5 (279.1; 121.8) | 114.4 (151.6; 77.2) |
Chloroplasts (pmol mg−1 chlorophyll) | 5.2 (4.3; 6.1) | 5.2 (9.9; 0.4) |
ABA | ||
Leaves (pmol g−1 FW) | 359.5 (447.7; 271.3) | 1102.3 (822.9; 1381.6) |
Leaves (pmol mg−1 chlorophyll) | 579.9 (704.4; 455.4) | 974.1 (847.2; 1100.9) |
Chloroplasts (pmol mg−1 chlorophyll) | 68.0 (44.0; 92.0) | 58.8 (106.6; 10.9) |
Values represent the mean of two replicates (individual measurements are shown in parentheses).
Endogenous contents of free IAA and ABA in leaves and in isolated intact chloroplasts of transgenic 35S:AtCKX3 and WT (SNN) tobacco
35S:AtCKX3 | WT (SNN) | |
IAA | ||
Leaves (pmol g−1 FW) | 125.0 (177.4; 72.6) | 122.1 (147.3; 96.9) |
Leaves (pmol mg−1 chlorophyll) | 200.5 (279.1; 121.8) | 114.4 (151.6; 77.2) |
Chloroplasts (pmol mg−1 chlorophyll) | 5.2 (4.3; 6.1) | 5.2 (9.9; 0.4) |
ABA | ||
Leaves (pmol g−1 FW) | 359.5 (447.7; 271.3) | 1102.3 (822.9; 1381.6) |
Leaves (pmol mg−1 chlorophyll) | 579.9 (704.4; 455.4) | 974.1 (847.2; 1100.9) |
Chloroplasts (pmol mg−1 chlorophyll) | 68.0 (44.0; 92.0) | 58.8 (106.6; 10.9) |
35S:AtCKX3 | WT (SNN) | |
IAA | ||
Leaves (pmol g−1 FW) | 125.0 (177.4; 72.6) | 122.1 (147.3; 96.9) |
Leaves (pmol mg−1 chlorophyll) | 200.5 (279.1; 121.8) | 114.4 (151.6; 77.2) |
Chloroplasts (pmol mg−1 chlorophyll) | 5.2 (4.3; 6.1) | 5.2 (9.9; 0.4) |
ABA | ||
Leaves (pmol g−1 FW) | 359.5 (447.7; 271.3) | 1102.3 (822.9; 1381.6) |
Leaves (pmol mg−1 chlorophyll) | 579.9 (704.4; 455.4) | 974.1 (847.2; 1100.9) |
Chloroplasts (pmol mg−1 chlorophyll) | 68.0 (44.0; 92.0) | 58.8 (106.6; 10.9) |
Values represent the mean of two replicates (individual measurements are shown in parentheses).
Plants expressing β-glucosidase Zm-p60.1
Compared with WT (SR1) leaves, a trend towards an increase in CK bases and ribosides and CK-N-glucosides was observed in leaves (Fig. 4A, B) of tobacco overexpressing maize β-glucosidase Zm-p60.1 targeted to plastids. A dramatic decrease in the levels of CK-O-glucosides remained restricted to the CK pool found in chloroplasts (Fig. 4C). Interestingly, a moderate decrease was also observed for CK-N-glucosides in the chloroplast fraction. The trend towards an increase in CK bases and ribosides observed in whole leaf extracts was also seen in the chloroplast fraction. Accordingly, the percentage of leaf CK-O- and N-glucosides sequestered in chloroplasts was significantly lower in the transgenic plants than in WT plants, while the values changed only marginally for CK bases and ribosides, and for phosphates (see supplemetary Table 1 available at JXB online).
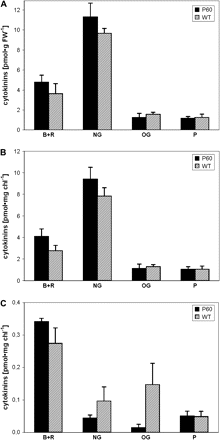
Cytokinin content (B+R, free bases and ribosides; NG, N-glucosides; OG, O-glucosides; P, phosphates) in leaves (per fresh weight A and per unit of chlorophyll B) and in isolated intact chloroplasts (C) of transgenic 35S:Zm-p60.1 (P60, black) and control SR1 (WT, striped) tobacco. Error bars display the SE.
The IAA measurements revealed a significantly lower level of IAA in leaves and in chloroplasts of transgenic plants compared with the WT IAA level (Table 3). The ABA content was reduced in Zm-p60.1 leaves compared with WT (Table 3). In chloroplasts, the difference in ABA level was less pronounced.
Endogenous contents of free IAA and ABA in leaves and in isolated intact chloroplasts of transgenic 35S:Zm-p60.1 and WT (SR1) tobacco
35S:Zm-p60.1 | WT (SR1) | |
IAA | ||
Leaves (pmol g−1 FW) | 85.7 (95.1; 76.2) | 144.0 (146.5; 141.5) |
Leaves (pmol mg−1 chlorophyll) | 60.3 (66.9; 53.6) | 135.2 (137.5; 132.8) |
Chloroplasts (pmol mg−1 chlorophyll) | 0.8 (1.4; 0.2) | 1.2 (2.1; 0.3) |
ABA | ||
Leaves (pmol g−1 FW) | 481.6 (486.9; 476.3) | 839.0 (785.9; 892.1) |
Leaves (pmol mg−1 chlorophyll) | 338.7 (342.4; 334.9) | 787.5 (737.6; 837.4) |
Chloroplasts (pmol mg−1 chlorophyll) | 23.7 (21.5; 25.8) | 26.3 (40.4; 12.1) |
35S:Zm-p60.1 | WT (SR1) | |
IAA | ||
Leaves (pmol g−1 FW) | 85.7 (95.1; 76.2) | 144.0 (146.5; 141.5) |
Leaves (pmol mg−1 chlorophyll) | 60.3 (66.9; 53.6) | 135.2 (137.5; 132.8) |
Chloroplasts (pmol mg−1 chlorophyll) | 0.8 (1.4; 0.2) | 1.2 (2.1; 0.3) |
ABA | ||
Leaves (pmol g−1 FW) | 481.6 (486.9; 476.3) | 839.0 (785.9; 892.1) |
Leaves (pmol mg−1 chlorophyll) | 338.7 (342.4; 334.9) | 787.5 (737.6; 837.4) |
Chloroplasts (pmol mg−1 chlorophyll) | 23.7 (21.5; 25.8) | 26.3 (40.4; 12.1) |
Values represent the mean of two replicates (individual measurements are shown in parentheses).
Endogenous contents of free IAA and ABA in leaves and in isolated intact chloroplasts of transgenic 35S:Zm-p60.1 and WT (SR1) tobacco
35S:Zm-p60.1 | WT (SR1) | |
IAA | ||
Leaves (pmol g−1 FW) | 85.7 (95.1; 76.2) | 144.0 (146.5; 141.5) |
Leaves (pmol mg−1 chlorophyll) | 60.3 (66.9; 53.6) | 135.2 (137.5; 132.8) |
Chloroplasts (pmol mg−1 chlorophyll) | 0.8 (1.4; 0.2) | 1.2 (2.1; 0.3) |
ABA | ||
Leaves (pmol g−1 FW) | 481.6 (486.9; 476.3) | 839.0 (785.9; 892.1) |
Leaves (pmol mg−1 chlorophyll) | 338.7 (342.4; 334.9) | 787.5 (737.6; 837.4) |
Chloroplasts (pmol mg−1 chlorophyll) | 23.7 (21.5; 25.8) | 26.3 (40.4; 12.1) |
35S:Zm-p60.1 | WT (SR1) | |
IAA | ||
Leaves (pmol g−1 FW) | 85.7 (95.1; 76.2) | 144.0 (146.5; 141.5) |
Leaves (pmol mg−1 chlorophyll) | 60.3 (66.9; 53.6) | 135.2 (137.5; 132.8) |
Chloroplasts (pmol mg−1 chlorophyll) | 0.8 (1.4; 0.2) | 1.2 (2.1; 0.3) |
ABA | ||
Leaves (pmol g−1 FW) | 481.6 (486.9; 476.3) | 839.0 (785.9; 892.1) |
Leaves (pmol mg−1 chlorophyll) | 338.7 (342.4; 334.9) | 787.5 (737.6; 837.4) |
Chloroplasts (pmol mg−1 chlorophyll) | 23.7 (21.5; 25.8) | 26.3 (40.4; 12.1) |
Values represent the mean of two replicates (individual measurements are shown in parentheses).
Plants expressing zeatin-O-glucosyltransferase ZOG1
When a ZOG1 gene was constitutively expressed in tobacco, CK-O-glucosides accumulated in leaves (Fig. 5A). The level of t-ZOG was two orders of magnitude higher in leaves of transformants than in those of WT (W38). No significant difference in the content of CK-N-glucosides, and only marginal reduction of the remaining CK forms was observed. These differences reduced further when the CK content was expressed per unit of chlorophyll (Fig. 5B), while that of CK-O-glucosides was more profound, as the chlorophyll content was lower in ZOG1 than in WT. By contrast, in chloroplasts, very low levels of CK-O-glucosides were found (Fig. 5C). The predominant CK forms were bases and ribosides, the contents of which, as well as that of CK phosphates, were higher in ZOG1 chloroplasts than in WT chloroplasts. Indeed, out of the transgenic plants analysed, only in the plants overexpressing ZOG1 was the percentage of leaf CK bases and ribosides sequestered in chloroplasts significantly higher in the transgenic plants than in WT plants (see supplementary Table 1 available at JXB online).
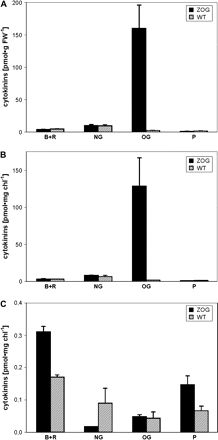
Cytokinin content (B+R, free bases and ribosides; NG, N-glucosides; OG, O-glucosides; P, phosphates) in leaves (per fresh weight A and per unit of chlorophyll B) and in isolated intact chloroplasts (C) of transgenic 35S:ZOG1 (ZOG, black) and control W38 (WT, striped) tobacco. Error bars display the SE.
In leaves of transgenic plants, a 2-fold higher IAA content was detected (Table 4). The ABA level was not significantly affected in ZOG1 leaves on a FW basis, but was higher when expressed per unit of chlorophyll. IAA and ABA contents were higher in ZOG1 chloroplasts than in WT chloroplasts.
Endogenous contents of free IAA and ABA in leaves and in isolated intact chloroplasts of transgenic 35S:ZOG1 and WT (W38) tobacco
35S:ZOG1 | WT (W38) | |
IAA | ||
Leaves (pmol g−1 FW) | 88.8 (102.1; 75.5) | 44.2 (38.4; 50.0) |
Leaves (pmol mg−1 chlorophyll) | 77.2 (88.8; 65.6) | 24.0 (20.8; 27.1) |
Chloroplasts (pmol mg−1 chlorophyll) | 1.5 (1.8; 1.1) | 1.0 (0.5; 1.4) |
ABA | ||
Leaves (pmol g−1 FW) | 528.1 (662.4; 393.8) | 523.3 (566.9; 479.6) |
Leaves (pmol mg−1 chlorophyll) | 459.2 (575.9; 342.4) | 284.0 (307.7; 260.3) |
Chloroplasts (pmol mg−1 chlorophyll) | 13.1 (20.9; 5.2) | 10.1 (15.0; 5.2) |
35S:ZOG1 | WT (W38) | |
IAA | ||
Leaves (pmol g−1 FW) | 88.8 (102.1; 75.5) | 44.2 (38.4; 50.0) |
Leaves (pmol mg−1 chlorophyll) | 77.2 (88.8; 65.6) | 24.0 (20.8; 27.1) |
Chloroplasts (pmol mg−1 chlorophyll) | 1.5 (1.8; 1.1) | 1.0 (0.5; 1.4) |
ABA | ||
Leaves (pmol g−1 FW) | 528.1 (662.4; 393.8) | 523.3 (566.9; 479.6) |
Leaves (pmol mg−1 chlorophyll) | 459.2 (575.9; 342.4) | 284.0 (307.7; 260.3) |
Chloroplasts (pmol mg−1 chlorophyll) | 13.1 (20.9; 5.2) | 10.1 (15.0; 5.2) |
Values represent the mean of two replicates (individual measurements are shown in parentheses).
Endogenous contents of free IAA and ABA in leaves and in isolated intact chloroplasts of transgenic 35S:ZOG1 and WT (W38) tobacco
35S:ZOG1 | WT (W38) | |
IAA | ||
Leaves (pmol g−1 FW) | 88.8 (102.1; 75.5) | 44.2 (38.4; 50.0) |
Leaves (pmol mg−1 chlorophyll) | 77.2 (88.8; 65.6) | 24.0 (20.8; 27.1) |
Chloroplasts (pmol mg−1 chlorophyll) | 1.5 (1.8; 1.1) | 1.0 (0.5; 1.4) |
ABA | ||
Leaves (pmol g−1 FW) | 528.1 (662.4; 393.8) | 523.3 (566.9; 479.6) |
Leaves (pmol mg−1 chlorophyll) | 459.2 (575.9; 342.4) | 284.0 (307.7; 260.3) |
Chloroplasts (pmol mg−1 chlorophyll) | 13.1 (20.9; 5.2) | 10.1 (15.0; 5.2) |
35S:ZOG1 | WT (W38) | |
IAA | ||
Leaves (pmol g−1 FW) | 88.8 (102.1; 75.5) | 44.2 (38.4; 50.0) |
Leaves (pmol mg−1 chlorophyll) | 77.2 (88.8; 65.6) | 24.0 (20.8; 27.1) |
Chloroplasts (pmol mg−1 chlorophyll) | 1.5 (1.8; 1.1) | 1.0 (0.5; 1.4) |
ABA | ||
Leaves (pmol g−1 FW) | 528.1 (662.4; 393.8) | 523.3 (566.9; 479.6) |
Leaves (pmol mg−1 chlorophyll) | 459.2 (575.9; 342.4) | 284.0 (307.7; 260.3) |
Chloroplasts (pmol mg−1 chlorophyll) | 13.1 (20.9; 5.2) | 10.1 (15.0; 5.2) |
Values represent the mean of two replicates (individual measurements are shown in parentheses).
Ultrastructure observations
The most striking anomaly in chloroplast ultrastructure was the occasional occurrence of crystalloid structures in chloroplasts of in vitro-cultivated plants harbouring the Sho gene under transcriptional control of four 35S enhancers (Fig. 6A, detailed view in Fig. 6B) as well as the 35S promoter. However, some chloroplasts from these plants did not show apparent changes in the structure compared with the WT (Fig. 6C). Chloroplasts from Sho-overexpressing plants contained more plastoglobuli than WT chloroplasts (Fig. 6D). The crystalloids were never present in chloroplasts of WT plants cultivated in vitro. After induction of the Sho gene expression, no crystalloids were found, only an increased grana stacking was observed (Fig. 6E). The crystalloids did not appear in chloroplasts of WT plants treated either with water (Fig. 6F), with dexamethasone in DMSO, or with DMSO solution, or in chloroplasts of non-induced plants carrying the Sho gene.
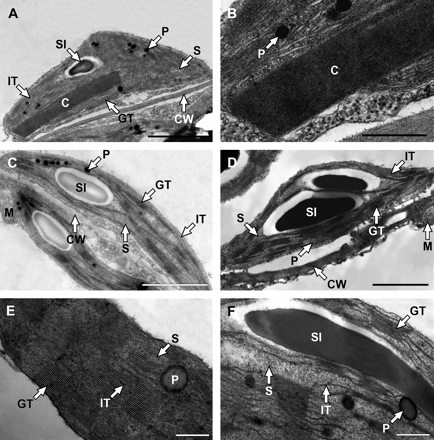
Transmission electron micrographs of representative chloroplast cross-sections taken from the intact leaves of tobacco expressing the Sho gene and four 35S enhancer elements (A, C) with detail of crystalloid (B) and control SR1 (D) tobacco growing under sterile conditions; and chloroplast cross-section detail taken from the leaf of transgenic dexamethasone-induced Sho (E) and control SR1 (F) tobacco cultivated in a greenhouse. C, crystalloid; CW, cell wall; GT, granal thylakoids; IT, intergranal thylakoids; M, mitochondrion; P, plastoglobuli; S, stroma; SI, starch inclusion. Bar=2 μm in A, C and D; and 500 nm in B, E and F.
Representative chloroplasts from the other types of transgenic tobacco and corresponding WTs are shown in Fig. 7. The most obvious feature observed was the different starch accumulation. The chloroplasts of tobacco overexpressing AtCKX3 (Fig. 7A) and Zm-p60.1 (Fig. 7C) have less starch inclusions than chloroplasts of WT SNN (Fig. 7B) and SR1 (Fig. 7D), respectively. In contrast, the chloroplasts of ZOG1 transformants (Fig. 7E) contained more starch inclusions than WT W38 chloroplasts (Fig. 7F). With increasing starch content, the shape of chloroplasts altered from lens-shaped to more loaf-like.
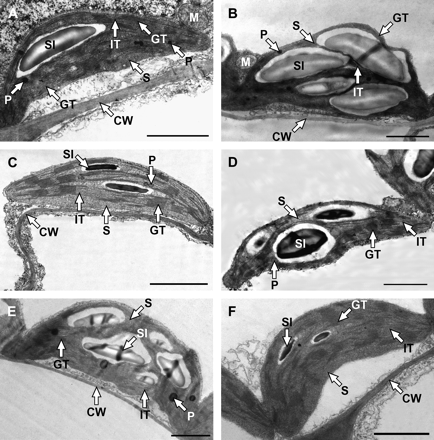
Transmission electron micrographs of representative chloroplast cross-sections taken from the intact leaves of transgenic 35S:AtCKX3 (A) and control SNN (B), transgenic 35S:Zm-p60.1 (C) and control SR1 (D), transgenic 35S:ZOG1 (E) and control W38 (F) tobacco. CW, cell wall; GT, granal thylakoids; IT, intergranal thylakoids; M, mitochondrion; P, plastoglobuli; S, stroma; SI, starch inclusion. Bar=2 μm.
Discussion
Hormone analyses
In order to elucidate the degree of chloroplast CK autonomy, the impact of altered CK metabolism on the CK pool in isolated intact chloroplasts and in bulk leaf tissue was compared. Bearing in mind hormone cross-talk, the effect of altered CK metabolism on IAA and ABA levels was also followed. The presence of these hormones in chloroplasts was demonstrated in earlier studies through the use of immunocytochemical (Sossountzov et al., 1986; Ohmiya and Hayashi, 1992; Kärkönen and Simola, 1999) or fractionation techniques, followed by HPLC or LCMS analysis (Sandberg et al., 1990; Benková et al., 1999). Chloroplasts were found to be the compartment where most of the ABA in leaf tissue is formed by the methyl-erythritol phosphate pathway (Milborrow and Lee, 1998). This pathway also provides most of the prenyl group of CKs (Kasahara et al., 2004). Nordström et al. (2004) demonstrated de novo CK synthesis in Arabidopsis shoots and tobacco leaves, and suggested that the presence of chloroplasts might be a prerequisite for the iPMP-independent pathway of CK biosynthesis.
Plants expressing isopentenyltransferase Sho
Numerous studies of plants transformed with the ipt gene, encoding an IPT from Agrobacterium tumefaciens, have demonstrated enhanced biosynthesis of endogenous CKs, especially of the Z-type (Redig et al., 1996; Motyka et al., 2003). Recently, genuine plant IPT genes (AtIPT1–9) were identified in the A. thaliana genome (Kakimoto, 2001; Takei et al., 2001) and their homologue Sho in P. hybrida (Zubko et al., 2002). The overexpression of the plant homologue AtIPT8 (Sun et al., 2003), as well as Sho (Zubko et al., 2002), in contrast to the bacterial ipt, leads to profound accumulation of the iP-type of CKs. A dexamethasone-inducible gene expression system (pOp6/LhGR; Šámalová et al., 2005) was used to drive Sho expression in transgenic tobacco. Following Sho induction in leaf extracts, major increases were observed in iP7G and iPMP, which is in line with previous results reported for petunia and tobacco overexpressing Sho constitutively (Zubko et al., 2002). The CK content was also increased in chloroplasts, although to a relatively lower extent. This is in accordance with preliminary results of feeding experiments (data not shown) showing that this IPT preferentially utilizes mevalonate as a substrate, indicating its cytosolic localization. In a recent analysis of Pssu-ipt tobacco (Synková et al., 2006), similarly to Sho plants, CK levels increased in chloroplasts to much lower levels compared with leaf extracts, indicating that the main CK pool accumulates outside chloroplasts in both Z- and iP-type CK-overproducing plants.
The content of IAA in leaves of Sho-expressing plants did not differ significantly from that in WT, whereas the chloroplasts contained a lower level of IAA than WT chloroplasts. In ipt tobacco, Eklöf et al. (1997, 2000) found lower levels of IAA and reduced rates of IAA synthesis and turnover. This has led to the suggestion that CKs might down-regulate IAA levels. Recently, a decrease in auxin biosynthesis following an increase in CK levels achieved by induction of ipt was observed in Arabidopsis, however, with a significant delay. Thus, the decrease was hypothesized to be mediated through an altered development rather than through a direct CK–auxin cross-talk (Nordström et al., 2004).
A higher ABA content was found in chloroplasts and in leaves (when expressed per FW) of Sho plants compared with WT. After overexpression of bacterial ipt, both a decrease (Synková et al., 1999; Chang et al., 2003) and an increase (Macháčková et al., 1997) in ABA content was reported.
Plants expressing CK oxidase/dehydrogenase AtCKX3
The CK oxidase/dehydrogenase selectively cleaves unsaturated N6 side chains of Z, iP, and their corresponding ribosides, while CK phosphates, O-glucosides, and CKs with saturated side chains are not substrates for CK oxidase/dehydrogenase (Armstrong, 1994). It was shown that overexpression of AtCKX genes in tobacco and Arabidopsis plants resulted in a reduced content of endogenous CKs and a strongly altered phenotype, with dwarfed shoot habit, enhanced root growth, and delayed flowering and senescence (Werner et al., 2001, 2003). More abundant Z-derived CKs were more strongly reduced than were iP derivatives in 35S:AtCKX1 and 35S:AtCKX2 Arabidopsis, in contrast to 35S:AtCKX1 and 35S:AtCKX2 tobacco where the more profound changes were in iP-type CKs. To our knowledge, the CK content has never been measured in any 35S:AtCKX3 transgenic plant. These plants are phenotypically identical to 35S:AtCKX1 and the enzyme is ultimately targeted to the same compartment, the vacuole, as proved by in planta experiments exploring an AtCKX–green fluorescent protein (GFP) fusion. Nevertheless, the apparent Km value for AtCKX3 against iP was 14 times higher than that for AtCKX1 (Werner et al., 2003). It has been shown that the ectopic expression of AtCKX3 reduced the CK levels in leaves of adult tobacco plants and also influenced the CK levels in chloroplasts. In leaves, the most strongly reduced CK metabolites were N-glucosides. It is obvious that after a tremendous increase of the activity of an important CK catabolic pathway (side chain cleavage by oxidase/dehydrogenase), the activity of the other CK deactivation pathway (N-glucosylation) was suppressed. In young tobacco seedlings with very low N-glucosylation activity, oxidase/dehydrogenase overexpression had only a marginal effect on the level of N-glucosides (Werner et al., 2001). This discrepancy could be explained by the different developmental stages of the analysed tobacco plants. In 35S:AtCKX1 and 35S:AtCKX2 Arabidopsis seedlings, Z9G and iPG were reduced compared with WT.
No significant differences were observed between the IAA levels, expressed on a FW basis, in leaves of adult AtCKX3 and WT tobacco plants. This finding contrasts with the results of Werner et al. (2003), who found reduced levels of IAA in 35S:AtCKX1 and 35S:AtCKX2 Arabidopsis seedlings. However, the authors speculate that CKs need not directly regulate IAA metabolism, but that the different tissue composition, i.e. reduced size of the shoot apical meristem with fewer meristematic cells and reduced cell production in leaves, might lead to a lowering of IAA-producing shoot tissue in transgenic plants.
A lower ABA level was found in AtCKX3 leaves compared with WT leaves, which might be linked to prolonged life span and retarded senescence of transgenic plants. Brugière et al. (2003) demonstrated induction of maize CKX1 gene expression in leaf discs by ABA, suggesting a role for this hormone in lowering CK concentrations under different abiotic stresses.
Plants expressing β-glucosidase Zm-p60.1
Maize β-glucosidase Zm-p60.1 was the first plant enzyme shown to release active CKs from CK-O- and N3-glucosides in vivo (Brzobohatý et al., 1993). Recently, it was shown that ectopic overexpression of Zm-p60.1 can perturb CK homeostasis in transgenic tobacco (Kiran et al., 2006). Higher levels of CK bases and ribosides in upper leaves and internodes of transgenic plants were found. The present results are consistent with those of the previous report. Moreover, it was found that a tendency to higher accumulation of CK bases and ribosides is also apparent in chloroplasts. The dramatic decrease in CK-O-glucoside levels reported here for chloroplasts isolated from Zm-p60.1 plants corresponded well with the plastid/chloroplast location of Zm-p60.1 (Kristoffersen et al., 2000) and a strong decrease in apparent Km for t-ZOG when the values obtained for the enzyme purified in vitro and present in isolated chloroplasts were compared (Kiran et al., 2006).
Lower IAA levels were determined in leaves as well as isolated chloroplasts of Zm-p60.1 plants compared with WT. This agrees with previous results, where a steeper fall in IAA gradient from high to low from youngest leaves downward, and a decreased IAA content in the apex and first internodes in Zm-p60.1 tobacco plants was found (Kiran et al., 2006). CK effects might be partly mediated by elevation of ethylene biosynthesis (Genkov et al., 2003). Ethylene treatment was shown to stimulate the oxidative decarboxylation of IAA (Winer et al., 2000). Thus, the elevated contents of active CKs in Zm-p60.1 plants could lead to lower levels of free IAA.
ABA measurements revealed a lower ABA content in Zm-p60.1 leaves than in WT. This finding is in contrast to those of a previous study (Kiran et al., 2006), when the tendency of increased ABA accumulation especially in older leaves was observed.
Plants expressing trans-zeatin-O-glucosyltransferase ZOG1
Tobacco transformed with the ZOG1 gene from P. lunatus under the control of the CaMV 35S promoter was constructed by Martin et al. (2001a). They found a strongly elevated t-ZOG content with no substantial changes in the level of other CK metabolites. In the present study, an order of magnitude higher accumulation of ZOG in ZOG1 leaves was detected, which may have been caused by the analysis of older material in the present case and different cultivation conditions. The elevated CK-O-glucoside concentrations in bulk leaf tissue did not significantly influence the content of these metabolites in intact chloroplasts isolated from the transgenic plants. This indicates that CK-O-glucosides are accumulated mainly outside chloroplasts, which agrees with the previous observation that transgenic plants overexpressing Zm-p60.1 can accumulate high levels of t-ZOG when grown on a medium supplemented with t-Z despite high Zm-p60.1 β-glucosidase activity in chloroplasts (Kiran et al., 2006). The vacuole has been proposed as the main storage compartment for CK-O-glucosides, based on feeding experiments in Chenopodium rubrum (Fußeder and Ziegler, 1988).
A 2-fold higher IAA content was observed in ZOG1 leaves compared with WT. Martin et al. (2001a) reported that IAA does not serve as a substrate for zeatin-O-glucosyltransferase and therefore excluded changes in IAA glucosylation as triggering the observed variations in morphology of transformants (e.g. aerial roots, multiple shoots, more compact stature than WT).
Ultrastructure observations
Considerable changes were observed in the chloroplast ultrastructure of tobacco constitutively expressing the Sho gene, including an increased number of plastoglobuli and the appearance of crystalloids in plants grown in vitro. These crystalloids were not found in other CK metabolism transformants analysed in this work and their corresponding WTs. In another type of transgenic tobacco, with strongly elevated endogenous CKs, Pssu-ipt, anomalies in chloroplast ultrastructure together with crystalloid occurrence were also found, and the distinct crystalline structures were hypothesized to be formed by 2D crystals of light-harvesting complex proteins (Synková et al., 2006).
When dexamethasone-induced Sho plants were analysed, increased grana stacking was noticed. Likewise, an increase in grana stacking was observed in chloroplasts of transgenic ipt tobacco (Čatský et al., 1993) and after CK treatment (Wilhelmová and Kutík, 1995; Salopek-Sondi et al., 2002).
Except for Sho tobacco, marked changes in the ultrastructure of chloroplasts were not found in the other CK transformants analysed. Only a difference in starch accumulation, associated with a change in chloroplast shape, was perceived. This finding is in accordance with data showing that exogenous CK application may increase starch accumulation in chloroplasts (Wilhelmová and Kutík, 1995; Stoynova et al., 1996). The involvement of endogenous CKs in starch formation and amyloplast development was proved by Miyazawa et al. (2002) in tobacco BY-2 cells.
In conclusion, a highly non-uniform distribution of CKs was found between chloroplasts and bulk leaf tissue in transgenic tobacco lines with several distinct alterations of CK metabolism, indicating that chloroplasts are relatively independent organelles with respect to the regulation of CK metabolism. Thus, it is evident that the estimation of overall CK content in plant tissue is not sufficient to assess its level at a particular site of action and that different compartmentation of individual CK metabolites should be taken into account. CK glucosides do not usually accumulate in chloroplasts, while active forms of CKs, bases, and ribosides are often found in them. In line with previous reports, the present data suggest a complex nature of mutual interactions between CKs, IAA, and ABA that apparently cannot be described by a simple model based on the current state of the art in this field. Thus, Werner et al. (2003) and Nordström et al. (2004) suggested that the effect of CKs on IAA levels might be mediated through altered development. Very variable data can be found in the literature on the interaction of CKs and ABA. CKs are known to counteract many processes induced by ABA (e.g. senescence or stomata closure). Elevated CK content thus might be balanced by higher ABA levels, which is also the case for Sho plants. However, the CK–ABA cross-talk does not seem to be straightforward. It probably strongly depends on the actual physiological conditions of the studied material including environmental factors and/or tissue specificity.
Supplementary data
Supplementary data available at JXB online are Table 1 showing the percentage of leaf CKs sequestered to chloroplasts and Table 2 listing the CK contents in leaves and isolated chloroplasts.
Abbreviations
- ABA
abscisic acid
- BA
benzyladenine
- CaMV
cauliflower mosaic virus
- CK
cytokinin
- DMSO
dimethylsulphoxide
- FW
fresh weight
- IAA
indole-3-acetic acid
- IPT
isopentenyltransferase
- LCMS
liquid chromatography/mass spectrometry
- WT
wild type
We are very grateful to Professor Peter Meyer (Sho), Professor Thomas Schmülling (AtCKX3), and Professors Machteld and David Mok (ZOG1) for kind donation of transgenic material, and to Professor David Morris for critical reading of the manuscript. This work was supported by grant nos 206/03/0369 and 206/06/1306 (Grant Agency of the Czech Republic) and IAA600040612 (Grant Agency of the Academy of Sciences of the Czech Republic).
Comments