-
PDF
- Split View
-
Views
-
Cite
Cite
Baoli Duan, Yongqing Yang, Yanwei Lu, Helena Korpelainen, Frank Berninger, Chunyang Li, Interactions between water deficit, ABA, and provenances in Picea asperata, Journal of Experimental Botany, Volume 58, Issue 11, August 2007, Pages 3025–3036, https://doi.org/10.1093/jxb/erm160
- Share Icon Share
Abstract
The effects of exogenous abscisic acid (ABA) on the acclimation of Picea asperata to water deficit were investigated in two populations originating from wet and dry climate regions of China. Exogenous ABA was sprayed onto the leaves, and changes in plant growth and structure, gas exchange, water use efficiency (WUE), endogenous ABA content, and antioxidant enzyme levels were monitored. The results demonstrated that ABA application affected the two P. asperata populations in different ways during the water deficit. ABA application resulted in significantly lower CO2 assimilation rates (A) under water deficit in plants from the wet climate population, whereas there were no significant changes in this parameter in the dry climate population. On the other hand, ABA application significantly decreased the dry shoot biomass, stomatal conductance (gs), transpiration rate (E), and malondialdehyde (MDA) content, and it significantly increased the leaf mass per area (LMA), root/shoot ratio (Rs), fine root/total root ratio (Ft), WUE, ABA content, and the superoxide dismutase (SOD), ascorbate peroxidase (APX), and catalase (CAT) activities under water-deficit conditions in the dry climate population, whereas ABA application did not significantly affect these parameters in the wet climate population. The results clearly demonstrated that sensitivity to an exogenous ABA application is population-dependent in P. asperata. Direct evidence is presented that variation in physiological mechanisms rather than different rates of ABA absorption explain the population differentiation in the sensitivity to exogenous ABA, and that the physiological basis for the amplified response to water deficit caused by exogenous ABA, present mainly in the dry climate population, is related to internal ABA accumulation. These results provide evidence for adaptive differentiation between populations of P. asperata, and they support the expected relationship between environmental heterogeneity and the magnitude of plastic responses in plant populations.
Introduction
Abscisic acid (ABA) has been considered to be one of the main internal plant signals that trigger the numerous acclimations that plants undergo when exposed to drought (Davies and Jones, 1991; Wilkinson and Davies, 2002; Li et al., 2004). Although ABA seems to play a predominant role in the conversion of environmental signals into changes in the gene expression of plants (Rock, 2000; Zhu, 2002), all plastic responses are not mediated by it (Gibson et al., 1991). ABA has also been shown to be involved in promoting drought tolerance when applied exogenously (Wang et al., 2003; Li et al., 2004). On the other hand, increasing evidence indicates that ABA interacts with membrane phospholipids to stabilize the membranes under stress conditions (Rajasekaran and Blake, 1998; Guschina et al., 2002) and it plays a role in the enhancement of tolerance to oxidative stress by increasing the activity of antioxidant enzymes (Jiang and Zhang, 2001; Yoshida et al., 2003). However, until now, this phenomenon has not been fully illustrated.
Water use efficiency (WUE) is traditionally defined either as the ratio of dry matter accumulation to water consumption over a season (WUET) or as the ratio of net CO2 assimilation rate (A) to transpiration (E) over a period of seconds or minutes (WUEi) (Sinclair et al., 1984; Condon et al., 2004). More recently, the carbon isotope composition (δ13C) has been developed as a tool to measure WUE because of a strong correlation between δ13C and WUE under given environmental conditions (Farquhar et al., 1989; Li et al., 2000; Yin et al., 2004). Furthermore, δ13C of plant tissue provides an integrated measurement of internal physiological and external environmental properties influencing photosynthetic gas exchange over the time when the carbon is fixed (Anderson et al., 1996; Li, 2000). The effects of endogenous ABA on the physiological properties under water deficits are largely understood, whereas the details of the mechanism by which ABA modifies stress tolerance requires to be further elucidated. In particular, the effects of exogenous ABA on WUE have yet to be identified.
Especially important is the question to what extent ABA interacts with the genetic background of plants. It is known that environmental variation may influence the divergence among plant populations in the plasticity of traits (Ackerly et al., 2000; Gianoli and Gonzalez-Teuber, 2005) and also that there are large genotypic components on how plants react to drought (Tuomela, 1997; Li et al., 2000). Although previous studies have documented population differentiation (Gibson et al., 1991; Sultan and Bazzaz, 1993; Heschel and Hausmann, 2001) in the responses to drought stress, few studies have examined the role of phytohormones in the plastic responses to environmental cues in woody plant populations.
Picea asperata Mast., which is one of the most important tree species used for the production of pulp wood and timber, is a prime reforestation species in western China. It occurs in the alpine and canyon regions of the northwestern Sichuan province and southeastern Gansu province, which are both important water-limited regions (Wu and Raven, 1994). However, low survival rates and a lack of seedling vigour are reducing the long-term benefits from conifer plantations (Farnum et al., 1983). This problem can be explained by the fact that conifer seedlings raised in nurseries are often subjected to water deficit soon after planting, as the newly planted seedlings have small root systems and an imperfect root–soil contact (Grossnickle et al., 1996). ABA has recently been tested for the purpose of improving seedlings’ performance as an anti-transpirant in Pinus (Hartung and Abou-Mandour, 1996) and black spruce (Picea mariana Mill.) seedlings (Grossnickle et al., 1996). In our previous experiments on the dry and wet climate populations of P. asperata, the presence of large population differences in the adaptive responses induced by water deficit were shown, the dry-climate population being more responsive to water deficit than the wet-climate population (Duan et al., 2005). In the present study, the effects of exogenous ABA on the morphological, physiological, and biochemical characteristics, including plant growth and structure, photosynthetic gas exchange, WUE, endogenous ABA content, the activities of antioxidant enzymes, and the lipid membrane peroxidation, were investigated during water deficit in pot-grown seedlings originating from two contrasting populations of P. asperata to test the hypothesis that an ABA application improves the performance of P. asperata seedlings in dry environments. In the present study, our previous studies are extended to address the following questions: (i) Does the sensitivity to ABA application differ between the two P. asperata populations? (ii) Will ABA application improve the water deficit tolerance of P. asperata? If yes, what is the physiological basis for the increased water deficit tolerance?
Materials and methods
Plant material and experimental design
A dry climate population (Danba, 31°04’ N, 105°27’ E) and a wet climate population (Heishui, 32°39’ N, 103°06’ E) of Picea asperata Mast. were selected for this study. The mean annual precipitation is 594 mm and 833 mm, and the mean annual temperature is 14.5 °C and 9.0 °C for the dry climate population and the wet climate population, respectively. Five-year-old healthy seedlings of a uniform height (30–35 cm) were obtained from two local semi-protected nurseries with a climate similar to that of the original site of the populations. The seedlings were transplanted to 5.0 l plastic pots filled with homogenized soil and grown in a naturally lit greenhouse under semi-controlled environmental conditions at the Maoxian Field Ecological Station from 1 May to 30 September 2005 with a day temperature range of 12–28 °C, a night temperature range of 9–15 °C and the relative humidity range of 35–85%. Experimental treatments started 30 d after the seedlings were transplanted. The plants were subjected to the water deficit treatments for five months. A total of 10 g slow-release fertilizer (13% N, 10% P, 14% K) was added to each pot during the experiment.
Two water availability treatments were applied in this study. (i) A well-watered (WW) treatment: 40 pots of each population were watered to 100% of field capacity by supplying an amount of water equal to transpiration losses every four days. This kept the relative extractable soil water (REW) at around 100±6 % (mean ±SE of 10 samples). (ii) A water deficit (D) treatment: another 40 pots of each population were maintained at 25% of the field capacity by watering them every four days. In this case, the REW was kept close to 11±3% (mean ±SE of 10 samples). Evidence that soil water content did not significantly oscillate between any two watering dates (every 4 d) and the water treatments were equivalent for both populations tested is shown in Fig. 1. In each population and watering treatment, half of the seedlings were given exogenous ABA (+ABA) [(±)-cis, trans-abscisic acid, Sigma, St Louis, MO, USA] by spraying ABA onto the needles using a hand sprayer until the needles of each seedling were thoroughly wet with 10 ml of 50 μM (±) ABA per day and seedling, as described by Li et al. (2003). In addition, a surfactant was needed to promote the uptake of ABA. Another half of the seedlings were sprayed with 10 ml water per day and seedling as a control (–ABA). In other words, there was a total of four treatments: (i) well-watered, no ABA application, (ii) water deficit, no ABA application, (iii) well-watered with ABA application, and (iv) water deficit with ABA application. In each treatment, there were 20 seedlings arranged into five blocks (four seedlings per population and treatment in each block). Moreover, the locations of the five blocks in the greenhouse were randomized every 2 weeks to eliminate block effects. Evaporation from the soil surface was prevented by enclosing the pots in plastic bags, which were tied to the stems of the plants. Transpiration water loss was measured gravimetrically by weighing all the pots every 4 d. Measurements of various morphological, physiological, and biochemical parameters were performed at the end of the experiment. Moreover, since the soil never becomes waterlogged and is porous enough to allow oxygen to diffuse freely, there was no indication of root rot or root death from lack of oxygen at the end of the experiments.
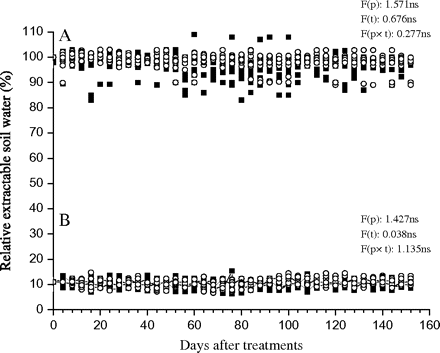
Variation of the relative extractable soil water (%) during the experiment for the (A) well-watered and (B) water-deficit treatments. The symbols represent different population: the Danba population from a dry climate region (open circles), the Heishui population from a wet climate region (filled squares). F-values and significant levels of the factorial analysis (ANOVA): F(p), the population effect; F(t), the time effect; F(p×t), the population×time interaction effect. ns, not significant.
Relative extractable soil water (REW)
The volumetric soil water content (θ) was measured every 4 d throughout the experiment by time domain reflectometry (Tektronix 1502 C, Beaverton, OR, USA) at two locations near the centre of each pot over a depth of 0–30 cm. The relative extractable soil water (REW) was calculated using the following equation, REW=(θ−θwp)/(θFC−θwp), where the subscripts WP and FC indicate the permanent wilting point and the field capacity, respectively.
Time-course of foliar 14C-ABA uptake
The uptake of ABA in needles was measured by the incorporation of 14C-ABA. The plant samples (needles) were treated with 50 μM ABA solutions containing 1.48 kBq of 14C-ABA, and incubated in 50 ml flasks with a tissue/volume ratio of 100 mg fresh weight/10 ml of solution for 24 h. At the end of the incubation, the leaf sections were rinsed with 10 ml ice-cold buffered medium containing unlabelled ABA at the same concentration used for the uptake, then further washed with water and finally blotted and placed directly into scintillation vials. Radioactivity was determined by liquid scintillation counting.
The relative water content and growth traits of the needles
For each population and treatment, current-year needles of 10 plants from five blocks (two plants from each block) were randomly selected. The relative water content (RWC) of the needles was calculated as: RWC (%)=(FW–DW)/(TW–DW)×100, where FW is the fresh weight, TW is the turgid weight after rehydrating samples sealed in darkness for 24 h in vials containing water to allow full saturation, and DW is the dry weight after oven-drying samples to constant weight at 85 °C for 24 h.
For each population and treatment, the height of all seedlings was measured every 15 d from the start to the end of the experiment. For each population and treatment, 10 plants from five blocks (two plants from each block) were randomly selected and harvested at the end of the experiment, and divided into needles, stem, and roots (coarse roots and fine roots; fine roots were defined as those with a diameter of 2 mm or less). The biomass samples were dried at 70 °C for 72 h to constant weight and weighed. Leaf area was measured using a Portable Laser Area Meter (CI-203, CID Inc, USA). The root/shoot ratio (Rs), fine root/total root ratio (Ft), and leaf mass per area (LMA) (the leaf dry weight divided by the projected leaf area of the whole seedling) were then calculated.
Gas exchange
The net CO2 assimilation rate (A), stomatal conductance (gs), and transpiration rate (E) were measured with a gas exchange system (CI-301PS, CID Inc, Vancouver, DC) equipped with a cylindrical cuvette (volume, 110 ml). The measurements took place under optimal summer conditions. The air in the leaf chamber was maintained at 23–28 °C with 36–55% of relative humidity inside the leaf chamber. The measurements were performed for current-year needles from one randomly selected seedling per block, a total of five plants for each population/watering/ABA. The projected leaf area of the sampled needles were measured with a Portable Laser Area Meter (CI-203, CID Inc). All rates of gas exchange were based on the projected leaf area. The ratio of A to E was taken as the instantaneous water use efficiency (WUEi). The measurements were conducted daily between 08.00 h and 11.00 h during a period of two weeks.
Transpiration efficiency
For each population and treatment, 10 plants (sampled from all five blocks) were randomly selected for the transpiration efficiency (WUET) measurement. WUET was determined for each seedling by dividing the total dry matter production by the cumulative amount of water used throughout the growing season. The total dry matter included needles, stems, and roots. The seedling dry matter at transplanting was estimated from the allometric relationship between the diameter and height of plants in each population, and it was subtracted from the final dry matter to estimate the total dry matter production over the course of the experiment.
Carbon isotope composition
For each population and treatment, current-year needles of 10 plants from five blocks (two plants from each block) were randomly selected for the carbon isotope analysis. Samples of 100 mg DW of plant material, oven-dried at 80 °C for 24 h, were homogenized by grinding in a ball mill. The stable carbon isotope abundance in the combusted samples was measured with a mass spectrometer (Finnegan MAT Delta-E) as described by Li et al. (2000). The overall precision of the δ-values was better than 0.1‰, as determined from repeated samples.
Quantitative analysis of ABA
For each population and treatment, current-year needles, the top of the stem, and fine roots of five plants from five blocks (one plant from each block) were randomly selected for the abscisic acid (ABA) analysis. The ABA content was analysed as described by Li et al. (2002). The samples were first weighed and then frozen in liquid nitrogen and freeze-dried. After that, samples of 30–50 mg DW of plant material were homogenized in 5 ml of 50 mM sodium phosphate buffer, pH 7.0, with 0.02% sodium diethyldithiocarbamate as antioxidant and 30 ng 2H4 ABA as an internal standard. ABA was measured by gas chromatography-mass spectrometry, as described by Johansen et al. (1986), with selective ion monitoring (SIM). Ions at 190.1 and 194.1 were monitored, and the amount of ABA in the samples was calculated using a standard curve drawn from the area ratios of known amounts of ABA and 2H4 ABA. The ABA level was calculated as μg g−1 FW.
Enzyme assays
For each population and treatment, current-year needles of five plants from five blocks (one plant from each block) were randomly selected for enzyme assays. Shoots with current-year needles were excised in the morning (between 07.00 h and 09.00 h). The samples were transported to the laboratory in darkness on a moist cloth on ice at a temperature near 0 °C. The enzymes were extracted from current-year needles using an ice-cold mortar and pestle, with 60 mg polyvinylpolypyrrolidone and 1 ml of the following optimized extraction media: superoxide dismutase (SOD) (100 mM K-phosphate buffer, pH 7.8, 0.1 mM EDTA, and 0.1% Triton X-100) and ascorbate peroxidase (APX) (50 mM K-phosphate buffer, pH 7.0, and 1 mM ascorbate). The resulting slurry was centrifuged at 12 000 g for 20 min at 4 °C. The supernatants were collected and used for the enzyme activity assays.
The total SOD activity was determined by measuring its ability to inhibit photochemical reduction of nitroblue tetrazolium (NBT) as described by Becana et al. (1986). The reaction mixture with a total volume of 3 ml contained 0.3 ml each of 20 μM riboflavin, 150 mM L-methionine, 600 μM NBT, and extracts of 0.1 ml. The reaction was started with the addition of riboflavin and carried out for 20 min under irradiance of 170 μmol photons m−2 s−1 provided by a white fluorescent lamp. The absorbance at 560 nm was determined, and the extract volume causing 50% inhibition of NBT reduction was taken as one unit of activity.
In the APX assay, conducted as described by Nakano and Asada (1981), the reaction mixture, containing 50 mM phosphate buffer (pH 7.0), 1 mM sodium ascorbate and 50 μl of the extract, was first equilibrated for 3 min. The reaction was started by the addition of 0.5 mM H2O2. The hydrogen peroxide-dependent oxidation of ascorbate was followed by a decrease in the absorbance at 290 nm.
The catalase (CAT) activity was measured spectrophotometrically, as previously described by Beers and Sizer (1952). The CAT activity was detected in 3 ml of 50 mM potassium phosphate buffer (pH 7.8) containing 3 mM H2O2. One unit was defined as the decomposition of 1 mmol H2O2 min−1 g−1 FW.
Lipid peroxidation
The oxidative damage to lipids was expressed as equivalents of malondialdehyde (MDA). For each population and treatment, current-year needles of five plants from five blocks (one plant from each block) were randomly selected for the MDA analysis. Current-year needles (about 0.5 g) were homogenized in 10 ml of 10% trichloroacetic acid (TCA), and centrifuged at 12 000 g for 10 min. 2 ml of 0.6% thiobarbituric acid (TBA) in 10% TCA was added to an aliquot of 2 ml of supernatant. The mixture was heated in boiling water for 30 min, and then quickly cooled in an ice bath. After centrifugation at 10 000 g for 10 min, the absorbance of the supernatant at 440, 532, and 600 nm was determined with a spectrometer (Unicam UV-330, USA). The MDA content was determined as described by Hodges et al. (1999).
Statistical analysis
All measurements were tested by a three-way ANOVA for the effects of ABA application, water deficit, and population. Before ANOVA, data were checked for normality and the homogeneity of variances, and log-transformed to correct deviations from these assumptions when needed. The analyses were performed with the general linear ANOVA model (GLM) procedure of SPSS 11.0 (SPSS Inc., Chicago, IL). Post-hoc comparisons were tested using the Tukey's test at a significance level of P <0.05. Pearson's correlation coefficients were calculated to determine the relationships between variables.
Results
Time-course of foliar 14C-ABA uptake
The curves reported in Fig. 2 show that, under our experimental conditions, the uptake of ABA in needles is nearly linear with time in both populations. There was no evidence that significantly more 14C-ABA penetrated through the needles of the dry climate population than through the needles of the wet climate population.
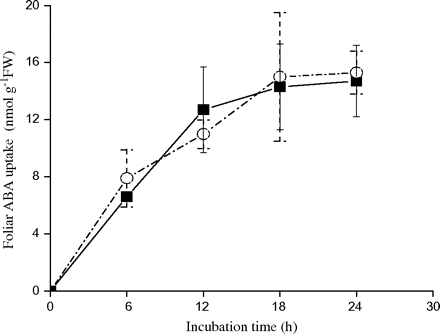
Time-course of foliar ABA uptake in two contrasting populations of P. asperata. Needles were incubated in a solution of 50 μM 14C-ABA for 24 h. The vertical lines represent SE. The values are means ±SE, n=3. The symbols represent different population: the Danba population from a dry climate region (open circles), the Heishui population from a wet climate region (filled squares).
The effects of exogenous ABA on plant growth and the relative water content of needles
Neither water deficit nor ABA application significantly affected the dry root biomass (Table 1), but the RWC of the wet climate population was lower in the water deficit condition than in the well-watered condition both with and without ABA application (Table 2). The exogenous ABA application significantly increased LMA, Rs, and Ft while it decreased the dry stem biomass of the dry climate population under water-deficit conditions (Table 2). The ABA application had a greater negative effect on height increment in the dry climate population, particularly in the water deficit treatment (Fig. 3). The dry climate population showed a significantly higher LMA, Rs, and Ft but a lower height increment and dry stem biomass than did the wet climate population under +ABA/water-deficit condition (Table 2).
Statistical significance of the F values (ANOVA) for the single and interactive effects of water deficit, ABA application, and population on different growth and physiological parameters
Parametera | Populationb | ABA applicationb | Water deficitb | ABA application× water deficitb | ABA application×populationb | Water deficit×populationb | ABA application×water deficit×populationb |
RWC | 35.9*** | 1.6ns | 97.8*** | 10.1** | 0.1 ns | 27.8*** | 0.1ns |
LMA | 12.1** | 11.8** | 4.0ns | 0.1ns | 2.8ns | 1.0ns | 1.1ns |
Height increment | 99.2*** | 7.3* | 13.5** | 3.6ns | 4.5ns | 6.5ns | 2.3ns |
Dry stem biomass | 29.9*** | 12.3** | 14.1** | 1.2ns | 0.4ns | 0.2ns | 1.0ns |
Dry root biomass | 0.6ns | 0.1ns | 0.2ns | 3.6ns | 0.8ns | 0.7ns | 0.6ns |
Rs | 42.1** | 29.2** | 26.9** | 0.1ns | 0.2ns | 0.6ns | 0.5ns |
Ft | 52.0*** | 8.9** | 2.8ns | 0.4ns | 7.7** | 0.1ns | 0.5ns |
A | 62.5*** | 13.2** | 37.1*** | 1.4ns | 4.5* | 7.1* | 0.1ns |
gs | 150.0*** | 36.7*** | 63.9*** | 18.5** | 5.0* | 17.2** | 2.8ns |
E | 86.0*** | 32.8*** | 63.3*** | 2.9ns | 0.8ns | 0.4ns | 2.3ns |
WUEi | 24.4*** | 20.0*** | 23.3*** | 8.8** | 20.2*** | 23.6*** | 11.7** |
WUET | 92.3*** | 86.8*** | 70.1*** | 0.4ns | 40.0*** | 1.6ns | 0.3ns |
δ13C | 47.7*** | 20.7** | 32.7*** | 3.8ns | 4.7* | 1.1ns | 1.8ns |
ABA leaf | 13.4** | 61.4*** | 83.9*** | 0.1 ns | 7.5* | 3.3ns | 7.5* |
ABA stem | 8.1* | 19.2** | 73.6*** | 0.2ns | 0.3ns | 3.6ns | 6.1* |
ABA root | 27.8** | 33.1*** | 93.3** | 0.7ns | 1.8ns | 5.9* | 2.1ns |
SOD | 15.2** | 31.0*** | 74.4*** | 0.2 ns | 13.4** | 2.3ns | 3.7ns |
APX | 8.4* | 13.3** | 33.4*** | 0.2ns | 5.9* | 8.4* | 2.9ns |
CAT | 5.3* | 10.1* | 18.7** | 1.6ns | 1.8ns | 8.0* | 1.8ns |
MDA | 24.9*** | 5.0* | 27.5*** | 22.4*** | 11.2** | 14.9** | 3.2ns |
Parametera | Populationb | ABA applicationb | Water deficitb | ABA application× water deficitb | ABA application×populationb | Water deficit×populationb | ABA application×water deficit×populationb |
RWC | 35.9*** | 1.6ns | 97.8*** | 10.1** | 0.1 ns | 27.8*** | 0.1ns |
LMA | 12.1** | 11.8** | 4.0ns | 0.1ns | 2.8ns | 1.0ns | 1.1ns |
Height increment | 99.2*** | 7.3* | 13.5** | 3.6ns | 4.5ns | 6.5ns | 2.3ns |
Dry stem biomass | 29.9*** | 12.3** | 14.1** | 1.2ns | 0.4ns | 0.2ns | 1.0ns |
Dry root biomass | 0.6ns | 0.1ns | 0.2ns | 3.6ns | 0.8ns | 0.7ns | 0.6ns |
Rs | 42.1** | 29.2** | 26.9** | 0.1ns | 0.2ns | 0.6ns | 0.5ns |
Ft | 52.0*** | 8.9** | 2.8ns | 0.4ns | 7.7** | 0.1ns | 0.5ns |
A | 62.5*** | 13.2** | 37.1*** | 1.4ns | 4.5* | 7.1* | 0.1ns |
gs | 150.0*** | 36.7*** | 63.9*** | 18.5** | 5.0* | 17.2** | 2.8ns |
E | 86.0*** | 32.8*** | 63.3*** | 2.9ns | 0.8ns | 0.4ns | 2.3ns |
WUEi | 24.4*** | 20.0*** | 23.3*** | 8.8** | 20.2*** | 23.6*** | 11.7** |
WUET | 92.3*** | 86.8*** | 70.1*** | 0.4ns | 40.0*** | 1.6ns | 0.3ns |
δ13C | 47.7*** | 20.7** | 32.7*** | 3.8ns | 4.7* | 1.1ns | 1.8ns |
ABA leaf | 13.4** | 61.4*** | 83.9*** | 0.1 ns | 7.5* | 3.3ns | 7.5* |
ABA stem | 8.1* | 19.2** | 73.6*** | 0.2ns | 0.3ns | 3.6ns | 6.1* |
ABA root | 27.8** | 33.1*** | 93.3** | 0.7ns | 1.8ns | 5.9* | 2.1ns |
SOD | 15.2** | 31.0*** | 74.4*** | 0.2 ns | 13.4** | 2.3ns | 3.7ns |
APX | 8.4* | 13.3** | 33.4*** | 0.2ns | 5.9* | 8.4* | 2.9ns |
CAT | 5.3* | 10.1* | 18.7** | 1.6ns | 1.8ns | 8.0* | 1.8ns |
MDA | 24.9*** | 5.0* | 27.5*** | 22.4*** | 11.2** | 14.9** | 3.2ns |
RWC, relative water content; LMA, leaf mass per area; Rs, root/shoot ratio; Ft, fine root/total root ratio; A, CO2 assimilation rate; gs, stomatal conductance; E, transpiration rate; WUEi, intrinsic water use efficiency; WUET, transpiration efficiency; δ13C, stable isotope composition; ABAleaf, ABAstem, and ABAroot, ABA contents in needles, stems, and roots, respectively; SOD, superoxide dismutase; APX, ascorbate peroxidase; CAT, catalase; MDA, malondialdehyde.
ns, Not significant; * P <0.05; ** P <0.01; *** P <0.001.
Statistical significance of the F values (ANOVA) for the single and interactive effects of water deficit, ABA application, and population on different growth and physiological parameters
Parametera | Populationb | ABA applicationb | Water deficitb | ABA application× water deficitb | ABA application×populationb | Water deficit×populationb | ABA application×water deficit×populationb |
RWC | 35.9*** | 1.6ns | 97.8*** | 10.1** | 0.1 ns | 27.8*** | 0.1ns |
LMA | 12.1** | 11.8** | 4.0ns | 0.1ns | 2.8ns | 1.0ns | 1.1ns |
Height increment | 99.2*** | 7.3* | 13.5** | 3.6ns | 4.5ns | 6.5ns | 2.3ns |
Dry stem biomass | 29.9*** | 12.3** | 14.1** | 1.2ns | 0.4ns | 0.2ns | 1.0ns |
Dry root biomass | 0.6ns | 0.1ns | 0.2ns | 3.6ns | 0.8ns | 0.7ns | 0.6ns |
Rs | 42.1** | 29.2** | 26.9** | 0.1ns | 0.2ns | 0.6ns | 0.5ns |
Ft | 52.0*** | 8.9** | 2.8ns | 0.4ns | 7.7** | 0.1ns | 0.5ns |
A | 62.5*** | 13.2** | 37.1*** | 1.4ns | 4.5* | 7.1* | 0.1ns |
gs | 150.0*** | 36.7*** | 63.9*** | 18.5** | 5.0* | 17.2** | 2.8ns |
E | 86.0*** | 32.8*** | 63.3*** | 2.9ns | 0.8ns | 0.4ns | 2.3ns |
WUEi | 24.4*** | 20.0*** | 23.3*** | 8.8** | 20.2*** | 23.6*** | 11.7** |
WUET | 92.3*** | 86.8*** | 70.1*** | 0.4ns | 40.0*** | 1.6ns | 0.3ns |
δ13C | 47.7*** | 20.7** | 32.7*** | 3.8ns | 4.7* | 1.1ns | 1.8ns |
ABA leaf | 13.4** | 61.4*** | 83.9*** | 0.1 ns | 7.5* | 3.3ns | 7.5* |
ABA stem | 8.1* | 19.2** | 73.6*** | 0.2ns | 0.3ns | 3.6ns | 6.1* |
ABA root | 27.8** | 33.1*** | 93.3** | 0.7ns | 1.8ns | 5.9* | 2.1ns |
SOD | 15.2** | 31.0*** | 74.4*** | 0.2 ns | 13.4** | 2.3ns | 3.7ns |
APX | 8.4* | 13.3** | 33.4*** | 0.2ns | 5.9* | 8.4* | 2.9ns |
CAT | 5.3* | 10.1* | 18.7** | 1.6ns | 1.8ns | 8.0* | 1.8ns |
MDA | 24.9*** | 5.0* | 27.5*** | 22.4*** | 11.2** | 14.9** | 3.2ns |
Parametera | Populationb | ABA applicationb | Water deficitb | ABA application× water deficitb | ABA application×populationb | Water deficit×populationb | ABA application×water deficit×populationb |
RWC | 35.9*** | 1.6ns | 97.8*** | 10.1** | 0.1 ns | 27.8*** | 0.1ns |
LMA | 12.1** | 11.8** | 4.0ns | 0.1ns | 2.8ns | 1.0ns | 1.1ns |
Height increment | 99.2*** | 7.3* | 13.5** | 3.6ns | 4.5ns | 6.5ns | 2.3ns |
Dry stem biomass | 29.9*** | 12.3** | 14.1** | 1.2ns | 0.4ns | 0.2ns | 1.0ns |
Dry root biomass | 0.6ns | 0.1ns | 0.2ns | 3.6ns | 0.8ns | 0.7ns | 0.6ns |
Rs | 42.1** | 29.2** | 26.9** | 0.1ns | 0.2ns | 0.6ns | 0.5ns |
Ft | 52.0*** | 8.9** | 2.8ns | 0.4ns | 7.7** | 0.1ns | 0.5ns |
A | 62.5*** | 13.2** | 37.1*** | 1.4ns | 4.5* | 7.1* | 0.1ns |
gs | 150.0*** | 36.7*** | 63.9*** | 18.5** | 5.0* | 17.2** | 2.8ns |
E | 86.0*** | 32.8*** | 63.3*** | 2.9ns | 0.8ns | 0.4ns | 2.3ns |
WUEi | 24.4*** | 20.0*** | 23.3*** | 8.8** | 20.2*** | 23.6*** | 11.7** |
WUET | 92.3*** | 86.8*** | 70.1*** | 0.4ns | 40.0*** | 1.6ns | 0.3ns |
δ13C | 47.7*** | 20.7** | 32.7*** | 3.8ns | 4.7* | 1.1ns | 1.8ns |
ABA leaf | 13.4** | 61.4*** | 83.9*** | 0.1 ns | 7.5* | 3.3ns | 7.5* |
ABA stem | 8.1* | 19.2** | 73.6*** | 0.2ns | 0.3ns | 3.6ns | 6.1* |
ABA root | 27.8** | 33.1*** | 93.3** | 0.7ns | 1.8ns | 5.9* | 2.1ns |
SOD | 15.2** | 31.0*** | 74.4*** | 0.2 ns | 13.4** | 2.3ns | 3.7ns |
APX | 8.4* | 13.3** | 33.4*** | 0.2ns | 5.9* | 8.4* | 2.9ns |
CAT | 5.3* | 10.1* | 18.7** | 1.6ns | 1.8ns | 8.0* | 1.8ns |
MDA | 24.9*** | 5.0* | 27.5*** | 22.4*** | 11.2** | 14.9** | 3.2ns |
RWC, relative water content; LMA, leaf mass per area; Rs, root/shoot ratio; Ft, fine root/total root ratio; A, CO2 assimilation rate; gs, stomatal conductance; E, transpiration rate; WUEi, intrinsic water use efficiency; WUET, transpiration efficiency; δ13C, stable isotope composition; ABAleaf, ABAstem, and ABAroot, ABA contents in needles, stems, and roots, respectively; SOD, superoxide dismutase; APX, ascorbate peroxidase; CAT, catalase; MDA, malondialdehyde.
ns, Not significant; * P <0.05; ** P <0.01; *** P <0.001.
Characteristics of plant growth and structure, the measurements of photosynthetic gas exchange, the activities of antioxidant enzymes, and the level of lipid peroxidation in two contrasting P. asperata populations, as affected by exogenous ABA application
Parametera | Populationb | −ABAb | +ABAb | −ABAb | +ABAb |
Well-watered | Well-watered | Water deficit | Water deficit | ||
RWC (%) | Heishui | 88.9±2.1 a | 87.1±1.5 a | 68.8±1.1 b | 70.0±2.3 b |
Danba | 76.7±2.3 b | 76.4±1.7 b | 67.2±1.0 b | 73.1±2.3 b | |
LMA (g m−2) | Heishui | 91.1±4.1 a | 97.8±6.5 a | 95.7±2.3 a | 98.7±1.0 a |
Danba | 96.2±3.4 a | 106.4±2.2 ab | 100.6±6.0 a | 118.3±2.1 b | |
Dry stem biomass (g) | Heishui | 22.23±0.68 a | 20.45±0.84 a | 20.11±1.49 a | 18.16±1.11 a |
Danba | 18.67±0.92 a | 17.34±0.62 a | 17.34±0.71 a | 13.17±0.78 b | |
Dry root biomass (g) | Heishui | 10.80±0.38 a | 11.72±0.09 a | 11.70±0.67 a | 11.81±0.42 a |
Danba | 11.17±0.62 a | 11.98±0.24 a | 11.97±0.55 a | 11.00±0.56 a | |
Rs | Heishui | 0.42±0.01 a | 0.49±0.02 ab | 0.48±0.03 ab | 0.54±0.02 b |
Danba | 0.50±0.01 ab | 0.57±0.01 b | 0.57±0.01 b | 0.67±0.01 c | |
Ft | Heishui | 0.29±0.02 a | 0.29±0.02 a | 0.31±0.02 a | 0.32±0.02 a |
Danba | 0.36±0.03 a | 0.43±0.02 ab | 0.37±0.01 a | 0.47±0.01 b | |
A (μmol m−2 s−1) | Heishui | 7.02±0.06 a | 6.29±0.31 a | 5.81±0.30 a | 4.75±0.32 b |
Danba | 5.01±0.08 b | 4.98±0.19 b | 4.69±0.07 b | 4.23±0.19 b | |
gs (mmol m−2 s−1) | Heishui | 374.8±8.3 a | 362.2±8.3 a | 350.8±19.5 a | 303.0±6.7 ab |
Danba | 299.9±11.4 b | 281.0±8.5 b | 243.2±20.0 b | 109.9±12.4 c | |
E (mmol m−2 s−1) | Heishui | 3.91±0.19 a | 3.28±0.16 a | 3.16±0.08 a | 2.65±0.14 ab |
Danba | 2.94±0.20 b | 2.59±0.09 b | 2.38±0.11 b | 1.36±0.07 c | |
SOD (units g−1 Fw) | Heishui | 196.00±9.64 a | 212.00±8.36 a | 310.70±7.60 b | 339.35±11.09 b |
Danba | 205.67±22.70 a | 327.33±31.94 b | 325.01±8.98 b | 506.61±36.44 c | |
APX (units g−1 FW) | Heishui | 10.14±0.25 a | 10.51±0.14 a | 10.77±0.38 a | 10.79±0.26 a |
Danba | 10.03±0.08 a | 10.62±0.19 a | 11.02±0.11 a | 12.32±0.14 b | |
CAT (units g−1 FW) | Heishui | 0.69±0.02 a | 0.72±0.01 a | 0.73±0.01 a | 0.75±0.03 a |
Danba | 0.68±0.08 a | 0.73±0.02 a | 0.76±0.05 a | 0.89±0.00 b | |
MDA (nmol g−1 FW) | Heishui | 5.17±0.13 a | 6.76±0.05 a | 8.00±0.19 b | 8.60±0.04 b |
Danba | 5.26±0.62 a | 6.52±0.20 a | 7.13±0.13 b | 5.31±0.27 a |
Parametera | Populationb | −ABAb | +ABAb | −ABAb | +ABAb |
Well-watered | Well-watered | Water deficit | Water deficit | ||
RWC (%) | Heishui | 88.9±2.1 a | 87.1±1.5 a | 68.8±1.1 b | 70.0±2.3 b |
Danba | 76.7±2.3 b | 76.4±1.7 b | 67.2±1.0 b | 73.1±2.3 b | |
LMA (g m−2) | Heishui | 91.1±4.1 a | 97.8±6.5 a | 95.7±2.3 a | 98.7±1.0 a |
Danba | 96.2±3.4 a | 106.4±2.2 ab | 100.6±6.0 a | 118.3±2.1 b | |
Dry stem biomass (g) | Heishui | 22.23±0.68 a | 20.45±0.84 a | 20.11±1.49 a | 18.16±1.11 a |
Danba | 18.67±0.92 a | 17.34±0.62 a | 17.34±0.71 a | 13.17±0.78 b | |
Dry root biomass (g) | Heishui | 10.80±0.38 a | 11.72±0.09 a | 11.70±0.67 a | 11.81±0.42 a |
Danba | 11.17±0.62 a | 11.98±0.24 a | 11.97±0.55 a | 11.00±0.56 a | |
Rs | Heishui | 0.42±0.01 a | 0.49±0.02 ab | 0.48±0.03 ab | 0.54±0.02 b |
Danba | 0.50±0.01 ab | 0.57±0.01 b | 0.57±0.01 b | 0.67±0.01 c | |
Ft | Heishui | 0.29±0.02 a | 0.29±0.02 a | 0.31±0.02 a | 0.32±0.02 a |
Danba | 0.36±0.03 a | 0.43±0.02 ab | 0.37±0.01 a | 0.47±0.01 b | |
A (μmol m−2 s−1) | Heishui | 7.02±0.06 a | 6.29±0.31 a | 5.81±0.30 a | 4.75±0.32 b |
Danba | 5.01±0.08 b | 4.98±0.19 b | 4.69±0.07 b | 4.23±0.19 b | |
gs (mmol m−2 s−1) | Heishui | 374.8±8.3 a | 362.2±8.3 a | 350.8±19.5 a | 303.0±6.7 ab |
Danba | 299.9±11.4 b | 281.0±8.5 b | 243.2±20.0 b | 109.9±12.4 c | |
E (mmol m−2 s−1) | Heishui | 3.91±0.19 a | 3.28±0.16 a | 3.16±0.08 a | 2.65±0.14 ab |
Danba | 2.94±0.20 b | 2.59±0.09 b | 2.38±0.11 b | 1.36±0.07 c | |
SOD (units g−1 Fw) | Heishui | 196.00±9.64 a | 212.00±8.36 a | 310.70±7.60 b | 339.35±11.09 b |
Danba | 205.67±22.70 a | 327.33±31.94 b | 325.01±8.98 b | 506.61±36.44 c | |
APX (units g−1 FW) | Heishui | 10.14±0.25 a | 10.51±0.14 a | 10.77±0.38 a | 10.79±0.26 a |
Danba | 10.03±0.08 a | 10.62±0.19 a | 11.02±0.11 a | 12.32±0.14 b | |
CAT (units g−1 FW) | Heishui | 0.69±0.02 a | 0.72±0.01 a | 0.73±0.01 a | 0.75±0.03 a |
Danba | 0.68±0.08 a | 0.73±0.02 a | 0.76±0.05 a | 0.89±0.00 b | |
MDA (nmol g−1 FW) | Heishui | 5.17±0.13 a | 6.76±0.05 a | 8.00±0.19 b | 8.60±0.04 b |
Danba | 5.26±0.62 a | 6.52±0.20 a | 7.13±0.13 b | 5.31±0.27 a |
RWC, relative water content; LMA, leaf mass per area; Rs, root/shoot ratio; Ft, fine root/total root ratio; A, CO2 assimilation rate; gs, stomatal conductance; E, transpiration rate; SOD, superoxide dismutase; APX, ascorbate peroxidase; CAT, catalase; MDA, malondialdehyde.
Heishui is a population from a wet climate region; Danba is a population from a dry climate region. The values within a row or column not sharing the same letters are significantly different (P <0.05) according to Tukey's test. The values are means ±SE, n=10 for RWC, LMA, dry stem biomass, dry root biomass, Rs and Ft; n=5 for A, gs, E, SOD, APX, CAT, and MDA.
Characteristics of plant growth and structure, the measurements of photosynthetic gas exchange, the activities of antioxidant enzymes, and the level of lipid peroxidation in two contrasting P. asperata populations, as affected by exogenous ABA application
Parametera | Populationb | −ABAb | +ABAb | −ABAb | +ABAb |
Well-watered | Well-watered | Water deficit | Water deficit | ||
RWC (%) | Heishui | 88.9±2.1 a | 87.1±1.5 a | 68.8±1.1 b | 70.0±2.3 b |
Danba | 76.7±2.3 b | 76.4±1.7 b | 67.2±1.0 b | 73.1±2.3 b | |
LMA (g m−2) | Heishui | 91.1±4.1 a | 97.8±6.5 a | 95.7±2.3 a | 98.7±1.0 a |
Danba | 96.2±3.4 a | 106.4±2.2 ab | 100.6±6.0 a | 118.3±2.1 b | |
Dry stem biomass (g) | Heishui | 22.23±0.68 a | 20.45±0.84 a | 20.11±1.49 a | 18.16±1.11 a |
Danba | 18.67±0.92 a | 17.34±0.62 a | 17.34±0.71 a | 13.17±0.78 b | |
Dry root biomass (g) | Heishui | 10.80±0.38 a | 11.72±0.09 a | 11.70±0.67 a | 11.81±0.42 a |
Danba | 11.17±0.62 a | 11.98±0.24 a | 11.97±0.55 a | 11.00±0.56 a | |
Rs | Heishui | 0.42±0.01 a | 0.49±0.02 ab | 0.48±0.03 ab | 0.54±0.02 b |
Danba | 0.50±0.01 ab | 0.57±0.01 b | 0.57±0.01 b | 0.67±0.01 c | |
Ft | Heishui | 0.29±0.02 a | 0.29±0.02 a | 0.31±0.02 a | 0.32±0.02 a |
Danba | 0.36±0.03 a | 0.43±0.02 ab | 0.37±0.01 a | 0.47±0.01 b | |
A (μmol m−2 s−1) | Heishui | 7.02±0.06 a | 6.29±0.31 a | 5.81±0.30 a | 4.75±0.32 b |
Danba | 5.01±0.08 b | 4.98±0.19 b | 4.69±0.07 b | 4.23±0.19 b | |
gs (mmol m−2 s−1) | Heishui | 374.8±8.3 a | 362.2±8.3 a | 350.8±19.5 a | 303.0±6.7 ab |
Danba | 299.9±11.4 b | 281.0±8.5 b | 243.2±20.0 b | 109.9±12.4 c | |
E (mmol m−2 s−1) | Heishui | 3.91±0.19 a | 3.28±0.16 a | 3.16±0.08 a | 2.65±0.14 ab |
Danba | 2.94±0.20 b | 2.59±0.09 b | 2.38±0.11 b | 1.36±0.07 c | |
SOD (units g−1 Fw) | Heishui | 196.00±9.64 a | 212.00±8.36 a | 310.70±7.60 b | 339.35±11.09 b |
Danba | 205.67±22.70 a | 327.33±31.94 b | 325.01±8.98 b | 506.61±36.44 c | |
APX (units g−1 FW) | Heishui | 10.14±0.25 a | 10.51±0.14 a | 10.77±0.38 a | 10.79±0.26 a |
Danba | 10.03±0.08 a | 10.62±0.19 a | 11.02±0.11 a | 12.32±0.14 b | |
CAT (units g−1 FW) | Heishui | 0.69±0.02 a | 0.72±0.01 a | 0.73±0.01 a | 0.75±0.03 a |
Danba | 0.68±0.08 a | 0.73±0.02 a | 0.76±0.05 a | 0.89±0.00 b | |
MDA (nmol g−1 FW) | Heishui | 5.17±0.13 a | 6.76±0.05 a | 8.00±0.19 b | 8.60±0.04 b |
Danba | 5.26±0.62 a | 6.52±0.20 a | 7.13±0.13 b | 5.31±0.27 a |
Parametera | Populationb | −ABAb | +ABAb | −ABAb | +ABAb |
Well-watered | Well-watered | Water deficit | Water deficit | ||
RWC (%) | Heishui | 88.9±2.1 a | 87.1±1.5 a | 68.8±1.1 b | 70.0±2.3 b |
Danba | 76.7±2.3 b | 76.4±1.7 b | 67.2±1.0 b | 73.1±2.3 b | |
LMA (g m−2) | Heishui | 91.1±4.1 a | 97.8±6.5 a | 95.7±2.3 a | 98.7±1.0 a |
Danba | 96.2±3.4 a | 106.4±2.2 ab | 100.6±6.0 a | 118.3±2.1 b | |
Dry stem biomass (g) | Heishui | 22.23±0.68 a | 20.45±0.84 a | 20.11±1.49 a | 18.16±1.11 a |
Danba | 18.67±0.92 a | 17.34±0.62 a | 17.34±0.71 a | 13.17±0.78 b | |
Dry root biomass (g) | Heishui | 10.80±0.38 a | 11.72±0.09 a | 11.70±0.67 a | 11.81±0.42 a |
Danba | 11.17±0.62 a | 11.98±0.24 a | 11.97±0.55 a | 11.00±0.56 a | |
Rs | Heishui | 0.42±0.01 a | 0.49±0.02 ab | 0.48±0.03 ab | 0.54±0.02 b |
Danba | 0.50±0.01 ab | 0.57±0.01 b | 0.57±0.01 b | 0.67±0.01 c | |
Ft | Heishui | 0.29±0.02 a | 0.29±0.02 a | 0.31±0.02 a | 0.32±0.02 a |
Danba | 0.36±0.03 a | 0.43±0.02 ab | 0.37±0.01 a | 0.47±0.01 b | |
A (μmol m−2 s−1) | Heishui | 7.02±0.06 a | 6.29±0.31 a | 5.81±0.30 a | 4.75±0.32 b |
Danba | 5.01±0.08 b | 4.98±0.19 b | 4.69±0.07 b | 4.23±0.19 b | |
gs (mmol m−2 s−1) | Heishui | 374.8±8.3 a | 362.2±8.3 a | 350.8±19.5 a | 303.0±6.7 ab |
Danba | 299.9±11.4 b | 281.0±8.5 b | 243.2±20.0 b | 109.9±12.4 c | |
E (mmol m−2 s−1) | Heishui | 3.91±0.19 a | 3.28±0.16 a | 3.16±0.08 a | 2.65±0.14 ab |
Danba | 2.94±0.20 b | 2.59±0.09 b | 2.38±0.11 b | 1.36±0.07 c | |
SOD (units g−1 Fw) | Heishui | 196.00±9.64 a | 212.00±8.36 a | 310.70±7.60 b | 339.35±11.09 b |
Danba | 205.67±22.70 a | 327.33±31.94 b | 325.01±8.98 b | 506.61±36.44 c | |
APX (units g−1 FW) | Heishui | 10.14±0.25 a | 10.51±0.14 a | 10.77±0.38 a | 10.79±0.26 a |
Danba | 10.03±0.08 a | 10.62±0.19 a | 11.02±0.11 a | 12.32±0.14 b | |
CAT (units g−1 FW) | Heishui | 0.69±0.02 a | 0.72±0.01 a | 0.73±0.01 a | 0.75±0.03 a |
Danba | 0.68±0.08 a | 0.73±0.02 a | 0.76±0.05 a | 0.89±0.00 b | |
MDA (nmol g−1 FW) | Heishui | 5.17±0.13 a | 6.76±0.05 a | 8.00±0.19 b | 8.60±0.04 b |
Danba | 5.26±0.62 a | 6.52±0.20 a | 7.13±0.13 b | 5.31±0.27 a |
RWC, relative water content; LMA, leaf mass per area; Rs, root/shoot ratio; Ft, fine root/total root ratio; A, CO2 assimilation rate; gs, stomatal conductance; E, transpiration rate; SOD, superoxide dismutase; APX, ascorbate peroxidase; CAT, catalase; MDA, malondialdehyde.
Heishui is a population from a wet climate region; Danba is a population from a dry climate region. The values within a row or column not sharing the same letters are significantly different (P <0.05) according to Tukey's test. The values are means ±SE, n=10 for RWC, LMA, dry stem biomass, dry root biomass, Rs and Ft; n=5 for A, gs, E, SOD, APX, CAT, and MDA.
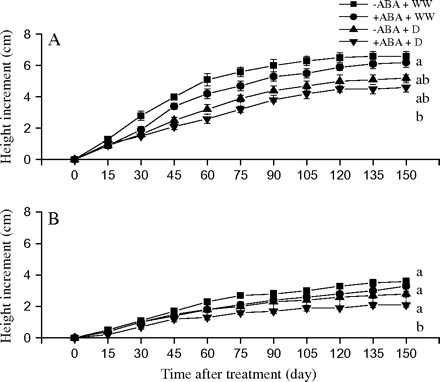
Height increment in two contrasting populations of P. asperata as affected by exogenous ABA application. (A) The Heishui population from a wet climate region; (B) the Danba population from a dry climate region. The vertical lines represent SE. The values are means ±SE, n=20. Treatments: –ABA+WW, non-ABA application+well-watered; +ABA+WW, ABA application+well-watered; –ABA+D, non-ABA application+water deficit; +ABA+D, ABA application+water deficit. Different letters on the right denote statistical differences (P <0.05) according to the Tukey's test.
The effects of exogenous ABA on photosynthetic gas exchange
In the dry climate population, the exogenous ABA application significantly decreased gs and E under water-deficit conditions, whereas the ABA application decreased A only in the seedlings from the wet climate population under water deficit (Table 2). Compared with the wet climate population, the dry climate population had significantly lower A, gs, and E values in all treatments, except in A under +ABA/water-deficit conditions (Table 2). The value of gs correlated negatively with the ABA content of the needles (Fig. 4A; r= –0.523, F=6.082, df=1, P=0.02 for the wet climate population and r= –0.741, F=9.011, df=1, P=0.003 for the dry climate population), while gs correlated negatively with the ABA content of the stem (Fig. 4B; r= –0.799, F=11.914, df=1, P=0.002 for the wet climate population and r= −0.939, F=134.133, df=1, P=0.000 for the dry climate population).
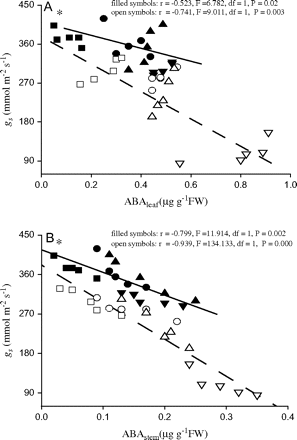
Relationships between the stomatal conductance (gs) and (A) the ABA content of needles, and (B) the ABA content of stems in plants from the dry climate population (open symbols) and wet climate population (filled symbols) of P. asperata as affected by an exogenous ABA application. The symbols represent different treatments: non-ABA application+well-watered (open squares), non-ABA application+water deficit (open triangles), ABA application+well-watered (open circles), ABA application+water deficit (open inverse triangles). Asterisk represents significant difference (P <0.01) between both the populations in their responsiveness of stomatal conductance to internal ABA tested by analysing the significant difference between slopes of regression line (Gebauer et al., 1996).
The effects of exogenous ABA on WUE
The dry climate population displayed a greater sensitivity of WUE to the ABA application than did the wet climate population (a significant population×ABA application effect; Table 1). The interaction between ABA application×water deficit×population was also significant in the case of WUEi (Table 1), showing that when exposed to both ABA and to water limitation, the dry climate population had a significantly higher WUEi. In the dry climate population, the exogenous ABA application significantly increased WUET, WUEi, and δ13C under water-deficit conditions (Fig. 5). However, the exogenous ABA application did not significantly affect these parameters in the wet climate population (Fig. 5). Compared with the wet climate population, the dry climate population possessed higher WUEi, WUET, and δ13C values under+ABA/water-deficit conditions (Fig. 5).
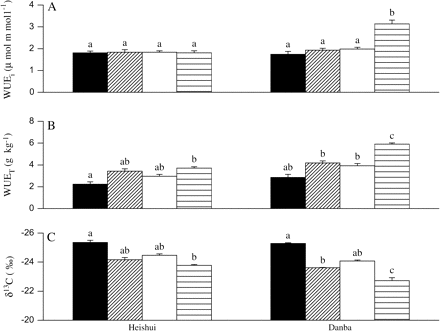
The instantaneous water use efficiency (WUEi) (A), the transpiration efficiency (WUET) (B), and the carbon isotope composition (δ13C) (C) in two contrasting populations of P. asperata as affected by an exogenous ABA application. Heishui, the population from a wet climate region; Danba, the population from a dry climate region. The values not sharing the same letters are significantly different (P <0.05) according to Tukey's test. The vertical lines represent SE. The values are means ±SE, n=5 for WUEi, n=10 for WUET and δ13C. Treatments: non-ABA application+well-watered (black area), non-ABA application+water deficit (diagonal lined area), ABA application+well-watered (white area), ABA application+water deficit (transverse lined area).
The effects of exogenous ABA on enzyme activities, and on the ABA and MDA contents
In the dry climate population, the exogenous ABA application significantly increased SOD activities and the ABA content of the needles under both well-watered and water-deficit conditions, and also significantly increased APX and CAT activities, and stem and root ABA contents, while it decreased the MDA content under water deficit conditions (Table 2; Fig. 6). However, the exogenous ABA application did not significantly affect these parameters in the wet climate population (Fig. 6). Compared with the wet climate population, the dry climate population showed significantly higher SOD activities under +ABA/water deficit and +ABA/well-watered conditions (Table 2), and it also showed significantly higher needle, stem and root ABA contents, and APX and CAT activities, and a lower MDA content under +ABA/water deficit conditions (Fig. 6; Table 2). In addition, the ABA content of the needles was consistently higher than that of the roots and stem.
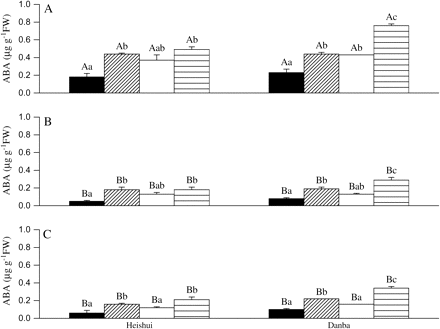
The ABA content in needles (A), stems (B), and roots (C) in two contrasting P. asperata populations as affected by an exogenous ABA application. Heishui, the population from a wet climate region; Danba, the population from a dry climate region. The lower case letters refer to differences within traits between the treatments. The capital letters refer to differences in the ABA content between the organs under the same treatments within the same population. The values not sharing the same letters are significantly different (P <0.05) according to Tukey's test. The vertical lines represent SE. The values are means ±SE, n=5. Treatments: non-ABA application+well-watered (black area), non-ABA application+water deficit (diagonal lined area), ABA application+well-watered (white area), ABA application+water deficit (transverse lined area).
Discussion
The present study provided experimental evidence of distinct differences in ABA-induced acclimation to water deficit between different populations of P. asperata. The exogenous ABA application significantly affected LMA, dry shoot biomass, Rs, Ft, gs, E, WUE, ABA accumulation, and the activities of antioxidant enzymes under water-deficit conditions in the dry climate population. However, the wet climate population was hardly affected by the ABA application concerning the measured variables, except for A. Out of the 13 traits measured in the dry climate population, eight traits were statistically different from all other treatments. Such results suggest that the difference in plant sensitivity to ABA treatments is associated with differences in the response to water deficit. Such intraspecific variability in response patterns is under genetic control, as environmental differences within the growth chamber were negligible and our experimental design minimized the effects of a possible non-homogenous environment in the growth chambers.
In this study, no significant effect of water deficit on LMA was found in either population during the treatments. It may be that the length of the experiment (5 months) did not allow enough time to elucidate the LMA responses of P. asperata to the water-deficit treatments. The two populations are characterized by discrete ‘flushes’ of shoot growth, as previously proposed by Crookshanks et al. (1998) for Pinus sylvestris. The ABA application resulted in a lower height increment, particularly under water-deficit conditions. Similar results have been observed in other studies (Li et al., 2004; Yin et al., 2004). High Rs in ABA-applied plants from the dry climate population under water deficit can account for the reductions in shoot biomass rather than for the effects on root growth as shown in Table 2. Considerable attention has been given to the possibility that an increase in the content of ABA in water deficit-stressed plants may be causally related to growth inhibition (Munns and Cramer, 1996; Li et al., 2004). However, a recent study indicates that ABA maintains rather than inhibits shoot growth, probably via the suppression of ethylene accumulation (Sharp, 2002; LeNoble et al., 2004). If this is the case, it can be presumed that one reason why shoot growth was inhibited while root growth was maintained in the dry climate population was that the ABA levels were sufficient to prevent ethylene-induced root growth inhibition, but were insufficient for the maximal elongation of the shoot (Sharp, 2002). Treatment with ABA significantly increased Ft in seedlings from the dry climate population under water deficit but not in the wet climate population. Such differences in carbon allocation within the root systems of the two populations imply different root architecture to optimize resource acquisition through changing root development (Crookshanks et al., 1998). The absence of a higher fine root growth in the water-deficit treatment compared with other treatments is not consistent with some of the literature, which supports the concept of a shift towards increased fine-root growth with increasing water deficit exposure (Tomlinson and Anderson, 1998; Yin et al., 2005). However, it is consistent with the study by Xiong et al. (2006) that reported that a decrease in fine root growth is an adaptive trait concerning tolerance to water deficit due to the urgent need for drought-stressed plants to increase the uptake of water by allocating more resources to the growth of primary roots. Such conflicting results indicate that the responses may be highly species-specific (Pallardy and Rhoads, 1993; Joslin et al., 2000). On average, when comparing the dry climate population with the wet climate population, the former has a higher plasticity of LMA and biomass allocation as a response to the ABA application.
The ABA application resulted in a significantly lower A in water deficit-stressed plants originating from the wet climate population, whereas there were no significant changes in this parameter in the dry climate population. Other studies concerning the effects of exogenously applied ABA on photosynthesis have produced various results, ranging from increases (Rajasekaran and Blake, 1998) to no change (Bradford et al., 1983) and to decreases (Li et al., 2004; Yin et al., 2004). Such variation in results can be explained by the fact that the ABA effects on stress tolerance may depend on the extent and duration of the dehydration as well as on the sensitivity of the particular species to drought (Gibson et al., 1991; Rajasekaran and Blake, 1998; Li et al., 2004; Yin et al., 2004). In addition, it was found that the stomata of the plants from the dry climate population closed earlier than those in the plants from the wet climate population and that stomatal closure was more complete when the plants were exposed to dry conditions. The variable gs is more strongly correlated with ABA in the stem than in the leaves. Therefore, ABA in the leaves may be less important in determining gs. This further strengthens the suggestion by Jia and Zhang (1999) that the concentration of ABA in the xylem is the most important determinant of stomatal function, and that population differences in ABA in the stem largely explain population variability in stomatal responses. Moreover, the lack of a change in RWC in the dry climate population may also further strengthen the view of the importance of internal regulation in reactions to water deficit.
Significant interaction effects detected between population and ABA application on WUEi, WUET, and δ13C indicate that there is not only plasticity in WUE in the response to the ABA application but that differences exist between the populations for their plastic responses, as previously detected in oak species (Ponton et al., 2002). There is now strong evidence that the plant hormone ABA plays an important role in the regulation of stomatal behaviour of plants under water deficit (Liu et al., 2003, 2005), and that it may result in an increase in the level of WUEi (A/E). This is due to the non-linear relationship observed between stomatal conductance and photosynthesis: partial stomatal closure decreases the transpiration of the leaves while the photosynthetic rate is less affected (Cowan, 1977; Turner, 1997; Liu et al., 2005). The results from the present study support this idea, particularly for the dry climate population. Further, as a consequence of a greater ABA-related reduction in transpiration than in dry matter accumulation in response to drought, the long-term WUE, as indicated by WUET and δ13C in the present study, was improved. Although not significant, the tendency is similar in the wet climate population as well. However, the increased WUE come at the cost of reduced growth. Perhaps the paradox can be explained if the effect of exogenous ABA being an integrated response throughout the plant concerning water conservation is considered. On the basis of the data presented here, field trials of the anti-transpirant properties of these compounds are recommended in order to minimize plant maintenance costs and to reduce the risk of water deficit stress during transplantation shock. Moreover, WUET and δ13C increased to a significantly greater degree in the plants from the dry climate population than they did in plants from the wet climate population, which predicts the presence of a more conservative water use pattern in the dry climate population. The result is consistent with the generally reported better performance of dry climate populations in stress-prone habitats (Li and Wang, 2003; Yin et al., 2005), but in contrast to studies that have reported that higher WUE may not necessarily be associated with the aridity of the habitat (Searson et al., 2004). At the site of the dry population, a drought cue may indicate a prolonged period of soil moisture deficit. Since P. asperata is deeply rooted and largely free from competition for water with the adjacent shrubs, it is likely that surface moisture conserved by the dry climate population would be available later in the growing season. In other words, water conservation by plants from the dry climate population with high WUE may confer a fitness advantage in water-limited habitats (Li and Wang, 2003).
The increase in ABA contents in needles, stems, and roots, caused by desiccation, was further enhanced by the ABA treatment in the dry climate population but not in the wet climate population. Moreover, in both populations the ABA content of the needles was consistently higher than that of the stem and roots. These results strongly suggest that ABA accumulation induced by water deficit is triggered differently in needle and root tissues. This suggestion is supported by many earlier studies that have shown that the responses of gene expression to stress are organ- or tissue-specific (Jia et al., 2002). The high level of ABA in needles may be a consequence of both in situ synthesis and an import from the roots (Stoll et al., 2000). Moreover, tissue-specific differences in the rates of metabolism could also influence the pattern of relative ABA content found in the tissues following the treatment. Further, owing to its rapid deactivation by photoisomerization (Abrams, 1999), it was assumed that any exogenous ABA left outside the cuticle would diffuse out. Thus, the high level of ABA in needles was unlikely to be due to contamination by exogenous ABA that remained outside the cuticle. In addition, the higher ABA accumulation observed in the dry climate population indicates that it has a greater ability than does the wet climate population to synthesize ABA and optimize its performance under stressful conditions. ABA increased in response to exogenous ABA application in all plant tissues in the dry climate population, this result at least rules out the possibility that an increase in one pool, for example, the needle, was at the expense of another pool, for example, the root-tip region. Further insight into the higher accumulation of ABA in the dry climate population can be gained from checking other possible ways in which an increase in endogenous ABA concentrations might occur in response to exogenous ABA application, for example, as a result of higher ABA biosynthesis rate, or a lower catabolic rate of free ABA, or the conversion of a conjugated form to free ABA.
In the present study, these results indicated that the ABA application can enhance antioxidant systems and, consequently, tolerance to water deficit stress. This effect was stronger in the dry climate population than in the wet climate population. This fact is reinforced by the lower level of lipid peroxidation in the dry climate population compared with the wet climate population. It is possible that the prevention of lipid peroxidation in the dry climate population is due to a reduction in ROS production by protecting the membranous organelles, mitochondria and chloroplasts (Alscher et al., 2002). On the other hand, in the wet climate population, slight increases in SOD activities may cause increased production rates of ROS, which remain unattended due to a failure of other defences to adjust correspondingly, and, thus, increased lipid peroxidation (Schwanz and Polle, 2001). Also, the predominance of APX over CAT was observed in the present study. In all, these results suggest that the ROS produced by SOD in the cells are mainly eliminated by APX.
The observed difference in the response to the ABA exposure between the two populations of P. asperata may have resulted from stronger selection pressures present in the dry climate population. It is possible that the adaptation of the dry climate population has involved the evolution of physiological traits that facilitate a greater plasticity to environmental variability, allowing a better exploitation of resources compared with the wet climate population when soil moisture is limited. On the other hand, since the cuticular membrane is considered to be the prime barrier to foliar uptake (Arteca and Tsai, 1987), direct evidence is presented of the ABA uptake, determined by the accumulation of radioactivity in samples of leaf segments incubated in 50 μM 14C-ABA (Fig. 2). Population differentiation in the sensitivity to ABA in P. asperata is not related to differences in the amount of ABA absorbed, as the uptake and retention of ABA over time were similar in the needles of both populations tested. However, it is difficult to exclude the alternative that ABA metabolism, subsequent to penetration, is responsible for the lack of response to foliar sprays in the wet climate population. It is suggested that physiological mechanisms rather than the rates of ABA absorption explain the population differentiation in the sensitivity to ABA. Three interpretations can be visualized for the above results: (i) the quantities of ABA that do penetrate may be insufficient to induce the desired response in the wet climate population; (ii) the wet climate populations may produce higher levels of compounds antagonistic to ABA (Sharma et al., 2005); and (iii) differential sensitivity of the receptors may play a role in determining the responses to ABA application (Quarrie, 1991). Additional research is required to explore these possibilities. In any case, the wet climate population was relatively unresponsive to the ABA spray. These results suggest that ABA application may exert its regulatory effect through an increase in the internal ABA content, which serves as a trigger for all the above symptoms in the dry climate population. Besides, an increase in the internal ABA content may be required to evolve in response to ABA exposure, and that may not exist in the wet climate population in which the ability to increase water deficit tolerance is not fully expressed. Therefore, the physiological basis for the increase in the dehydration tolerance after an ABA treatment is related to the internal ABA accumulation, and the protective effect of ABA is due to stomatal control and the ability to enhance the elimination system for ROS, as measured in terms of antioxidant enzymes.
In conclusion, the experimental data presented here clearly indicate that exogenous ABA amplified the response to water deficit mainly in the dry climate population. The response is attributable to strong stomatal closure and to the greater plasticity of LMA and biomass allocation, as well as to higher levels of ABA contents and antioxidant enzyme activities, possibly predominantly APX activities. These results also suggest that it is feasible to anticipate the involvement of ABA in the manipulation of stomatal aperture and in the improvement of WUE under water-limited conditions in the dry climate population. However, the ABA exposure during water deficit had no significant effect on most of the measured variables in the wet climate population. On the basis of the above results, it is concluded that sensitivity to ABA and acclimation to water deficit is population-dependent in P. asperata. This work also highlights the importance of considering intraspecific variability before making conclusions regarding the role of ABA application. Moreover, perhaps the greatest remaining challenge is to estimate whether water conservation becomes a real benefit for the ABA-treated dry climate population in natural conditions. Undoubtedly, environmental conditions outdoors is very complex, and whether our results can hold in the field may depend on the presence of concurrent environmental factors such as water availability. The potential for the responses to ABA application that occur under field conditions needs to be thoroughly evaluated to address the questions raised in the present work. The potential benefits or carrying out this work are, however, considerable, both for reforestation and afforestation projects with drier conditions.
The research was supported by the Outstanding Young Scientist Program of the National Natural Science Foundation of China (No. 30525036) and the China National Key Program of the International Cooperation for Science and Technology (No. 2005DFA30620).
Comments